ABSTRACT HAPTIC RENDERING FOR INTERNAL CONTENT OF AN IMPLICIT OBJECT
基于心流理论的沉浸式VR旅游产品设计的科学研究

基于心流理论的沉浸式VR旅游产品设计的科学研究心流理论是指个体在从事某项活动时,完全投入毫无分心的心理状态。
在这种状态下,个体会体验到极度放松、愉悦、流畅,时间流失的感觉。
心流理论的提出者Csikszentmihalyi认为,心流状态不仅提升了个体的幸福感,还能够提高工作和学习效率。
沉浸式虚拟现实(VR)旅游是指通过虚拟现实技术,让用户感受到身临其境的旅行体验。
在VR旅游中,用户可以自由移动、观察景物和与虚拟环境进行互动,通过视觉、听觉、触觉等感官体验,达到类似于真实旅游的感受。
基于心流理论的沉浸式VR旅游产品设计,可以有效提升用户的体验和满足感。
本文旨在通过科学研究,探讨如何设计这样的产品。
需要充分利用虚拟现实技术的优势,创造逼真、丰富的虚拟环境。
在设计景点或场景时,应注重细节,力求让用户感觉到身临其境。
还可以通过增加互动元素,让用户参与到故事或任务中,增加用户的投入感。
设计过程中应注重用户的流动体验,即用户在沉浸式VR旅游中的心流状态。
为了达到这一目的,可以采取以下策略:一是提供适当的挑战和难度,让用户感到兴奋和满足;二是为用户提供及时的反馈,让用户了解自己的表现,并根据反馈进行调整;三是设计清晰的目标和任务,让用户有明确的方向和动力。
还可以利用虚拟现实技术的优势,创造独特的体验和感受。
在设计中可以加入音乐、声音效果等,让用户更深度地沉浸于虚拟环境中;还可以与其他用户进行互动,创造社交性的体验,增加用户的参与感和满足感。
对于沉浸式VR旅游产品设计的科学研究,我们还需要进行用户实验和评估。
通过实际测试和用户反馈,评估产品的效果和用户的体验。
还需要不断改进和优化产品设计,以满足用户的需求和期望。
介绍全息技术英语作文

介绍全息技术英语作文Holographic technology is a cutting-edge innovationthat has revolutionized the way we perceive and interact with visual content. It creates three-dimensional images using light, and has a wide range of applications in various industries, including entertainment, healthcare, education, and more.One of the most exciting aspects of holographic technology is its potential to transform the entertainment industry. With holographic displays, performers can create stunning visual effects and immersive experiences for their audiences. This technology has the power to bring virtual characters and worlds to life in a way that was previously unimaginable.In the field of healthcare, holographic technology is being used to revolutionize medical imaging and training. Doctors can now use holographic images to better understand complex medical conditions, plan surgeries, and educatepatients about their treatment options. This has the potential to improve patient care and outcomes significantly.In the education sector, holographic technology is being used to create immersive learning experiences for students. By using holographic images and simulations, educators can bring abstract concepts to life and make learning more engaging and interactive. This can help students better understand and retain information, leading to improved academic performance.Another exciting application of holographic technology is in the field of design and engineering. Engineers and designers can use holographic images to visualize and manipulate 3D models of their projects, allowing them to make more informed decisions and streamline the design process. This has the potential to improve the efficiency and quality of their work significantly.Overall, holographic technology has the potential to revolutionize the way we interact with visual content invarious industries. Its ability to create immersive and interactive experiences makes it a powerful tool for entertainment, healthcare, education, design, and more. As the technology continues to advance, we can expect to see even more exciting applications and innovations in the future.。
基于心流理论的沉浸式VR旅游产品设计的科学研究
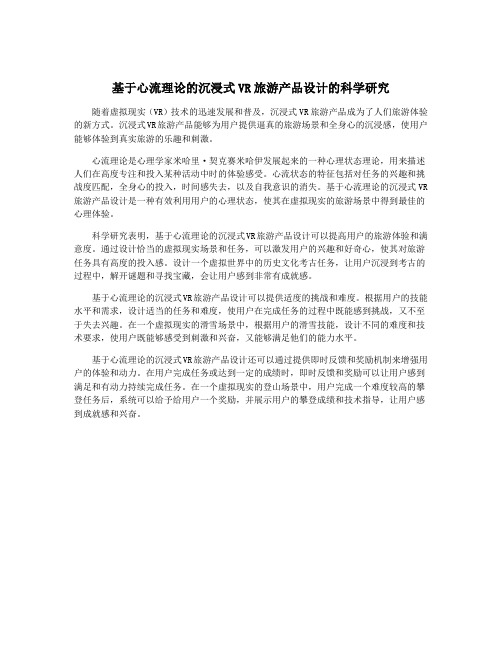
基于心流理论的沉浸式VR旅游产品设计的科学研究随着虚拟现实(VR)技术的迅速发展和普及,沉浸式VR旅游产品成为了人们旅游体验的新方式。
沉浸式VR旅游产品能够为用户提供逼真的旅游场景和全身心的沉浸感,使用户能够体验到真实旅游的乐趣和刺激。
心流理论是心理学家米哈里·契克赛米哈伊发展起来的一种心理状态理论,用来描述人们在高度专注和投入某种活动中时的体验感受。
心流状态的特征包括对任务的兴趣和挑战度匹配,全身心的投入,时间感失去,以及自我意识的消失。
基于心流理论的沉浸式VR 旅游产品设计是一种有效利用用户的心理状态,使其在虚拟现实的旅游场景中得到最佳的心理体验。
科学研究表明,基于心流理论的沉浸式VR旅游产品设计可以提高用户的旅游体验和满意度。
通过设计恰当的虚拟现实场景和任务,可以激发用户的兴趣和好奇心,使其对旅游任务具有高度的投入感。
设计一个虚拟世界中的历史文化考古任务,让用户沉浸到考古的过程中,解开谜题和寻找宝藏,会让用户感到非常有成就感。
基于心流理论的沉浸式VR旅游产品设计可以提供适度的挑战和难度。
根据用户的技能水平和需求,设计适当的任务和难度,使用户在完成任务的过程中既能感到挑战,又不至于失去兴趣。
在一个虚拟现实的滑雪场景中,根据用户的滑雪技能,设计不同的难度和技术要求,使用户既能够感受到刺激和兴奋,又能够满足他们的能力水平。
基于心流理论的沉浸式VR旅游产品设计还可以通过提供即时反馈和奖励机制来增强用户的体验和动力。
在用户完成任务或达到一定的成绩时,即时反馈和奖励可以让用户感到满足和有动力持续完成任务。
在一个虚拟现实的登山场景中,用户完成一个难度较高的攀登任务后,系统可以给予给用户一个奖励,并展示用户的攀登成绩和技术指导,让用户感到成就感和兴奋。
《《治愈创造力》(节选)英汉翻译实践报告》范文

《《治愈创造力》(节选)英汉翻译实践报告》篇一《治愈创造力》英汉翻译实践报告(节选)一、引言在全球化的大背景下,翻译成为了一种跨越语言和文化的桥梁,尤其在当下数字化和信息化的时代,翻译的需求与日俱增。
本次实践报告的主题是“治愈创造力”的翻译,本篇为该实践报告的节选部分。
在此,我们将探讨在翻译过程中所遇到的挑战、翻译策略的选择及效果,并分享实践过程中的感悟与反思。
二、原文背景及翻译任务介绍《治愈创造力》一书旨在探讨如何通过创造力的培养和激发来达到治愈心灵的目的。
本书内容涉及心理学、艺术、文学等多个领域,旨在为读者提供一种全新的思考方式和生活方式。
翻译任务的目标是将这本书从英文翻译成中文,以便更多的中文读者能够了解和体验这本书的内容。
三、翻译过程中的挑战与策略1. 词汇选择与理解在翻译过程中,遇到了大量专业词汇和地道的表达方式。
针对这些问题,我们首先进行了充分的词汇准备,查阅了大量的专业文献和词典。
在理解原文的基础上,我们尽量选择最贴切的词汇来传达原文的意思。
同时,我们注意到了不同领域词汇的灵活运用,确保译文既符合专业要求,又符合中文的表达习惯。
2. 句式结构调整英文句子结构与中文句子结构存在较大差异。
在翻译过程中,我们针对不同的句式结构进行了调整,以适应中文的表达习惯。
例如,对于长句,我们进行了拆分和重组,使其更符合中文的逻辑顺序;对于复杂的从句结构,我们采用了顺译和倒译的方法,使译文更加流畅自然。
3. 文化背景的传达本书涉及心理学、艺术等多个领域,其中包含大量的文化背景信息。
为了确保译文的准确性,我们在翻译过程中注重文化背景的传达。
我们通过查阅相关资料和文献,了解西方文化和东方文化的差异,以确保在翻译过程中能够准确传达原文的文化内涵。
四、翻译效果评估与反思经过我们的努力,《治愈创造力》中文版已经顺利出版并得到了广大读者的好评。
在翻译过程中,我们采用了多种翻译策略和方法,取得了较好的翻译效果。
首先,在词汇选择上,我们尽可能选择了最贴切的词汇来传达原文的意思;其次,在句式结构上,我们进行了适当的调整和重组;最后,在文化背景的传达上,我们注重了中西文化的差异和融合。
全息投影手环的作文英语
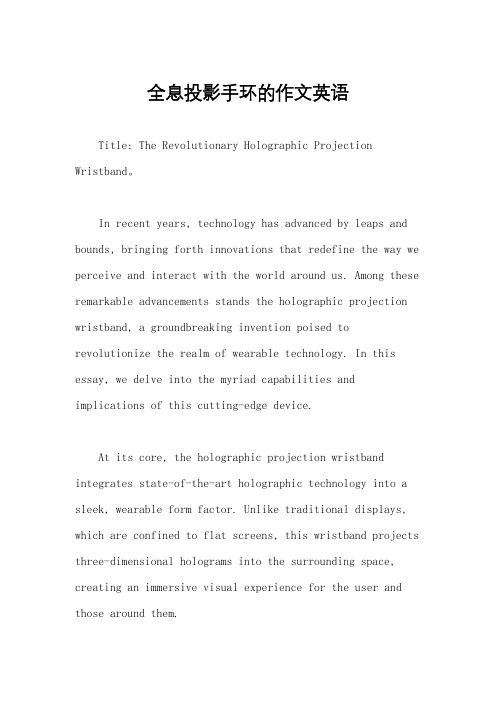
全息投影手环的作文英语Title: The Revolutionary Holographic Projection Wristband。
In recent years, technology has advanced by leaps and bounds, bringing forth innovations that redefine the way we perceive and interact with the world around us. Among these remarkable advancements stands the holographic projection wristband, a groundbreaking invention poised to revolutionize the realm of wearable technology. In this essay, we delve into the myriad capabilities and implications of this cutting-edge device.At its core, the holographic projection wristband integrates state-of-the-art holographic technology into a sleek, wearable form factor. Unlike traditional displays, which are confined to flat screens, this wristband projects three-dimensional holograms into the surrounding space, creating an immersive visual experience for the user and those around them.One of the most notable features of the holographic projection wristband is its versatility. Whether it's for entertainment, communication, education, or productivity, the wristband offers a wide range of applications limited only by imagination. Imagine being able to watch your favorite movies or concerts in full 3D, with holographic images appearing as if they were right in front of you. Furthermore, the wristband could serve as a communication tool, allowing users to engage in holographic video calls with friends, family, or colleagues from anywhere in the world.In the realm of education, the holographic projection wristband holds immense potential to revolutionize learning experiences. Imagine students being able to visualize complex concepts in three dimensions, bringing subjectslike biology, physics, and history to life in ways never before possible. With interactive holographic simulations, learning becomes not only more engaging but also more effective, as students gain a deeper understanding of abstract concepts through immersive experiences.Moreover, the wristband could significantly enhance productivity in various fields. For professionals such as architects, engineers, and designers, being able tovisualize and manipulate 3D models directly in front ofthem could streamline the design process and foster greater creativity. In business settings, presentations could become more dynamic and engaging, as speakers use holographic visuals to convey their ideas with impact.Beyond its practical applications, the holographic projection wristband also has the potential to reshape entertainment and social interactions. Imagine attendinglive events where holographic performers interact with the audience in real-time, blurring the lines between thevirtual and the physical worlds. Additionally, thewristband could enable new forms of social media, where users share holographic experiences and engage with each other in immersive virtual environments.Of course, with any new technology, there are also considerations regarding privacy, security, and ethical use.As holographic projections become more commonplace in everyday life, it will be essential to establish clear guidelines and regulations to protect users' privacy and prevent misuse of the technology.In conclusion, the holographic projection wristband represents a paradigm shift in wearable technology, offering a glimpse into a future where interactions with digital content are no longer confined to screens but seamlessly integrated into our surroundings. With its vast potential for entertainment, education, productivity, and social interaction, the wristband heralds a new era of immersive experiences limited only by our imagination. As we continue to push the boundaries of technological innovation, the possibilities presented by the holographic projection wristband are truly limitless.。
基于心流理论的沉浸式VR旅游产品设计的科学研究
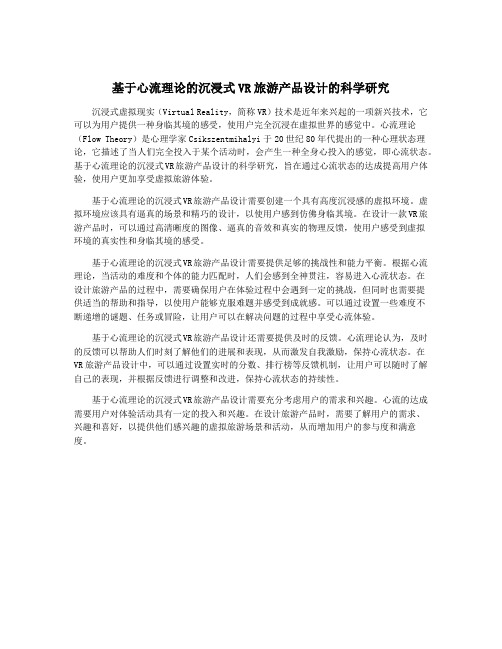
基于心流理论的沉浸式VR旅游产品设计的科学研究沉浸式虚拟现实(Virtual Reality,简称VR)技术是近年来兴起的一项新兴技术,它可以为用户提供一种身临其境的感受,使用户完全沉浸在虚拟世界的感觉中。
心流理论(Flow Theory)是心理学家Csikszentmihalyi于20世纪80年代提出的一种心理状态理论,它描述了当人们完全投入于某个活动时,会产生一种全身心投入的感觉,即心流状态。
基于心流理论的沉浸式VR旅游产品设计的科学研究,旨在通过心流状态的达成提高用户体验,使用户更加享受虚拟旅游体验。
基于心流理论的沉浸式VR旅游产品设计需要创建一个具有高度沉浸感的虚拟环境。
虚拟环境应该具有逼真的场景和精巧的设计,以使用户感到仿佛身临其境。
在设计一款VR旅游产品时,可以通过高清晰度的图像、逼真的音效和真实的物理反馈,使用户感受到虚拟环境的真实性和身临其境的感受。
基于心流理论的沉浸式VR旅游产品设计需要提供足够的挑战性和能力平衡。
根据心流理论,当活动的难度和个体的能力匹配时,人们会感到全神贯注,容易进入心流状态。
在设计旅游产品的过程中,需要确保用户在体验过程中会遇到一定的挑战,但同时也需要提供适当的帮助和指导,以使用户能够克服难题并感受到成就感。
可以通过设置一些难度不断递增的谜题、任务或冒险,让用户可以在解决问题的过程中享受心流体验。
基于心流理论的沉浸式VR旅游产品设计还需要提供及时的反馈。
心流理论认为,及时的反馈可以帮助人们时刻了解他们的进展和表现,从而激发自我激励,保持心流状态。
在VR旅游产品设计中,可以通过设置实时的分数、排行榜等反馈机制,让用户可以随时了解自己的表现,并根据反馈进行调整和改进,保持心流状态的持续性。
基于心流理论的沉浸式VR旅游产品设计需要充分考虑用户的需求和兴趣。
心流的达成需要用户对体验活动具有一定的投入和兴趣。
在设计旅游产品时,需要了解用户的需求、兴趣和喜好,以提供他们感兴趣的虚拟旅游场景和活动,从而增加用户的参与度和满意度。
基于心流理论的沉浸式VR旅游产品设计的科学研究

基于心流理论的沉浸式VR旅游产品设计的科学研究一、心流理论概述心流理论是由心理学家米哈里·契克塞米哈伊在20世纪70年代提出的,它描述了一种个体在全神贯注、专注于特定活动时所体验到的心理状态。
心流状态具有以下特点:专注、挑战与技能平衡、目标明确、即时反馈、感知掌控力、自我消失等。
心流状态既能带来情感上的愉悦,又能提高工作效率,改善工作质量。
二、沉浸式VR旅游产品沉浸式虚拟现实旅游产品是指利用虚拟现实技术,使用户在虚拟的环境中产生身临其境的感觉,达到一种仿佛置身于真实场景的效果。
沉浸式VR旅游产品在景区体验、旅游宣传、文化传播等方面具有广泛的应用前景。
三、基于心流理论的沉浸式VR旅游产品设计1. 专注体验:沉浸式VR旅游产品需要具有引导用户进入心流状态的能力,让用户在沉浸的虚拟环境中能够集中注意力,忘却外界的干扰。
通过精心设计的场景和互动,引导用户进入心流状态,从而获得更加深刻的体验。
2. 挑战与技能平衡:沉浸式VR旅游产品应该在保证用户体验挑战的让用户感到自己具备足够的技能来完成旅游体验的任务。
这种挑战与技能的平衡将激发用户的兴趣和动力,让他们更愿意投入到沉浸式VR旅游产品中去。
3. 目标明确:沉浸式VR旅游产品应该设定明确的目标和任务,并给予用户清晰的导航和提示,避免用户迷失在虚拟环境中。
用户在完成目标的过程中,可以体验到成就感和满足感,从而更容易进入心流状态。
4. 即时反馈:沉浸式VR旅游产品的设计应该及时给予用户反馈,让用户知道他们的行为和决策所带来的结果。
这种即时反馈能够增强用户参与感和体验感,让用户更好地投入到沉浸式VR旅游产品中。
5. 感知掌控力:沉浸式VR旅游产品应该给予用户一定的自由度和控制权,让用户在虚拟环境中感到自己的行为和决策可以影响场景的发展和结果。
这种感知掌控力可以增强用户的参与感和投入感,促进用户进入心流状态。
6.自我消失:沉浸式VR旅游产品的设计应该尽量减少干扰因素,让用户能够全神贯注地投入到虚拟环境中。
人机交互中的社会临场感研究——以弹幕短视频为例

◎2023年第3期◎*本文系广东省哲学社会科学“十三五”规划学科共建项目“社交媒体数据驱动的用户应急信息行为模式研究”(项目编号:GD20XTS02)研究成果。
人机交互中的社会临场感研究——以弹幕短视频为例*李晶,薛晨琦,宋昊阳摘要人机交互已经成为当今信息交互的主要场景,社会临场感被证明是在人机交互过程中具有重要影响的一种用户心理体验,但已有文献缺乏对社会临场感的量化研究以及影响机理的揭示。
弹幕短视频是一种最常见的人机交互应用,弹幕为创造社会临场感提供了载体。
文章以B 站的科普视频为研究对象,利用Python 工具收集视频弹幕共299,994条,采用数据挖掘及文本分析法,从情感、认知两个维度出发,计算每个视频的社会临场感水平,在此基础上分析视频的社会临场感水平与用户视频使用行为的关联关系。
研究结果表明:短视频的社会临场感水平与视频播放量、用户点赞量、用户投币量以及视频弹幕总数之间存在正相关关系。
研究结论为拓展人机交互领域的社会临场感理论,以及基于用户视角促进科普类视频的分享与传播提供启示和参考。
关键词人机交互社会临场感弹幕信息分析文本分析引用本文格式李晶,薛晨琦,宋昊阳.人机交互中的社会临场感研究:以弹幕短视频为例[J].图书馆论坛,2023,43(3):141-150.Research on Social Presence in Human-Computer Interaction :Taking Short Videos with Bullet Screen as a CaseLI Jing ,XUE Chenqi &SONG HaoyangAbstract Human-computer interaction has become the main scenario of information interaction at present.Socialpresence has been proved to be a user psychological experience that has an important influence in human-computer interaction.However ,there are few quantitative researches on social presence and its influence mechanism.Short video with bullet screen is one of the most common human-computer interaction applications.Bullet screen provides a carrier for creating a sense of social presence.This article takes the popular science videos at Bilibili asthe research object ,uses python tools to collect a total of 299,994bullet comments (or ,real-time comments )from the videos ,and employs data mining and text analysis methods to calculate the social presence level of each video from the emotional and cognitive dimensions.On this basis ,the relationship between the level of social presence of the video and the user's video use behavior is analyzed.The results show that there is a significant positive correlation between the level of video ’s social presence and the volume of video views ,user likes ,coin-loading and bullet comments.This study provides inspiration and reference for extending the theory of social presence to the field of human-computer interaction ,and promoting the sharing and dissemination of popularscience videos from the user's perspective.Keywords Human-Computer Interaction ;social presence ;information analysis of bullet comments ;textanalysis1410引言随着计算机迅速发展,“面对面”的信息交互逐渐被人机交互(Human-Computer Inter-action,HCI)取代,计算机媒介沟通(Comput-er-Mediated Communication,CMC)时代随之到来,依托计算终端开展一对多的“虚拟”交互成为日常。
- 1、下载文档前请自行甄别文档内容的完整性,平台不提供额外的编辑、内容补充、找答案等附加服务。
- 2、"仅部分预览"的文档,不可在线预览部分如存在完整性等问题,可反馈申请退款(可完整预览的文档不适用该条件!)。
- 3、如文档侵犯您的权益,请联系客服反馈,我们会尽快为您处理(人工客服工作时间:9:00-18:30)。
HAPTIC RENDERING FORINTERNAL CONTENT OF AN IMPLICIT OBJECTThavida Maneewarn Blake HannafordDepartment of Electrical Engineering Department of Electrical Engineering University of Washington University of Washington/BRL /BRL Duane W. Storti Mark A. GanterDepartment of Mechanical Engineering Department of Mechanical Engineering University of Washington University of Washingtonstorti@ ganter@ABSTRACTThe haptic rendering algorithms for object with implicit surface representation are proposed. The basic rendering algorithm for a regular two-dimensional manifold is used to represent a solid volumetric object. For applications where information about the internal content of an object is concerned, the haptic rendering algorithm for internal content of an implicit object is developed. The proposed algorithm is derived from the concept of state transition via ‘port’ using manifold with boundary representation. Manifold with boundary is constructed from implicit primitives. The advantage of implicit surface representation in haptic applications has been emphasized. Both algorithms were successfully implemented in the haptic interface system and were applied to various implicit models. INTRODUCTIONBased on experience in the physical world, it is natural for humans to perceive objects as being more realistic as more of the senses are provided the feedback normally associated with interactions with a physical object. Since the goal of any CAD/CAM system is to provide a virtual representation of a part, assembly, or environment that is perceived to be as real as possible.In this paper, we concentrate on haptic feedback which provides the user with tactile sensations that either simulate interaction with the real version of the virtual object or which are designed to assist in communicating characteristics of the object. In particular, we focus on haptic rendering, the process of creating a synthetic force to replicate the reaction force associated with contacting an object.Previously introduced haptic rendering algorithms can be classified according to their reliance on standard surface representations. Zilles [13] introduced the "god-object" approach that allows polyhedral model to be rendered by restricting the surface contact point from passing through the object. Thompson [12] proposed a related algorithm that supports direct haptic rendering of NURBS surfaces.Some algorithms employ a local approximation of the surface to reduce computation time. Adachi [1] and Mark [4] use a slowly changing planar approximation as an intermediate representation of a surface. Zilles [13], Morgenbesser and Srinivasan [7] and Ruspini [10] applied a technique called ‘force shading’, analogous to Phong shading in computer graphics, to render surface curvature by interpolating surface normal vectors of polyhedral facets.Methods that do not rely directly on surface representation of a geometric model have also been suggested, such as a potential field method due to Massie and Salisbury [5] and a voxel-based method [2] that determines haptic interaction forces based on the current position of the user interaction point. Voxel-based methods are suited for rendering large volumetric objects, but they are memory intensive and may not be applied to objects with feature sizes comparable to voxel sizes.Implicit surface representationImplicit surfaces are two-dimensional, geometric shapes that exist in three-dimensional space. The surface is implicitly described by an analytic function S(p) as S(p) = 0. The use of implicit surfaces in computer graphics was initially introduced in scan-line rendering of quadric surfaces (Davis et al. [3] and Mittelman [6]). The implicit function characterizes volume as well as surface, since value of S(p) indicates the proximity between point P and the surface. Implicit functions can not only be used to describe basic geometric shapes. The model of complicate shapes can be achieved by applying appropriate blending operations to basic implicit primitive [9]. The use of implicit surface representation in geometric modeling has increased due to its unique properties. These properties include simple collision detection between a point and the surface that can be achieved from function evaluation. If S(p)<0, point P is enclosed inside the surface. If S(p)>0, point P is outside the surface and if S(p) = 0, point P is on the surface. The gradient of S(p) is proportional to the surface normal vector pointing in outward direction. The gradient of S(p) can be described by)/)(,/)(,/)(()(zpSypSxpSpS∂∂∂∂∂∂=∇The surface normal vector indicates the direction of a responsive force from the implicit model. The gradient of an implicit equation can be calculated both analytically and numerically. Anotherinteresting property is that the inside/outside can simply be reversed by sign inversion of S(p). This property is very useful in a rendering process for some class of objects, which will be discussed in the following section.The haptic rendering of implicit surfaces was first introduced by Salisbury and Tar[13]. They proposed a method for rendering solid objects represented by regular two-manifold and extended to address the specific problem of rendering finites solid cones or cylinders created from intersecting half-spaces with an implicit solid. However,their method only applies to the surfaces enclosing solid volumetric objects regardless of the internal content that may be confined inside the surfaces.In geometric modeling, the non-regularized manifolds such as ‘manifold with boundary’ and ‘non-manifold’ are also concerned. The non-regularized manifold description can be applied to objects that consist of non-uniform or composite materials, mostly used in biomedical or electronics applications. Manifold with boundary is a surface that locally approximated either by a disk or by a half-disk. The manifold with boundary represents object that contains openings or dangling edges. The haptic rendering technique proposed in this paper can be applied to more general classes of objects in addition to the solid volumetric object described by the two-manifold. We would like to emphasize the advantage of implicit representation in haptic rendering where the object interior can be haptically displayed. One example described here is to simulate the effect of having non-solid substance confined in the space enclosed by implicit surfaces, such as liquid in a cup. The algorithm is very simple to implement and is not computationally expensive, which make it appropriate to be performed in real-time. The model can be well described by a small number of implicit equations. Thus only small memory space is required in this method unlike in voxel-based method or in polyhedral model-based method.Figure 1.1 A cup that contains liquid inside can be described by manifold with boundary constructed from implicit primitives.IMPLICIT HAPTIC RENDERING ALGORITHMThe haptic rendering algorithm for objects defined by implicit surfaces can be described in two parts, the rendering algorithms for a regular two-dimensional manifold and the rendering algorithm for internal content of an object using manifold with boundary description.Although the rendering algorithm for regular two-dimensional manifold proposed here has several aspects that are quite similar to previous work by Salisbury and Tar [11], our implementation details are different from theirs in various points. For instance, the Lagrangian multiplier is used, in the method introduced by Salisbury and Tar, inorder to find the surface contact point which minimize distance between the seed point (the probe point that is inside the surface for the first time) and the point on the surface. The problem of using the closest point on the surface instead of the actual surface crossing point can be illustrated in figure (2.1). When the probe point approaches the convex surface, the surface contact point is established at )(k P o ′which is the nearest point from )(k P instead of the actual surface crossing point at )(k P o . The responsive force that is calculated from )(k P o ′ may be incorrect and it may cause an undesirable effect. In our algorithm, the binary search is used to establish the surface contact point at the crossing point between a line segment and the surface, which will provide better accuracy. Details of our haptic rendering algorithm for a regular two-dimension manifold will be described in the following section.Figure 2.1 A situation where surface contact point can be incorrectly established at the closest point between the seed point and the implicit surface.Rendering a regular two-dimensional manifoldThe rendering algorithm for regular two-dimensionalmanifold is an important basis for more complicate rendering algorithm that will be presented in the next section. Two-dimension manifold is often used to describe the surface enclosing solid objects where the point-set inside the surface is entirely separate from the outside space. When the probe point is contacting with solid objects,the responsive force is a force that prevents a probe point from penetrating into the surface. The responsive force is defined by an equation )())()((k BV k P k P K f i o −−=, where K representsstiffness and B represents damping of a solid object. o P (k) is the surface contact point and i P (k) is the interaction point inside the surface. V(k) is velocity of the probe point.Figure 2.2 A responsive force resulted from contacting the surface)(k P o )(k P i ))()((BV k P k P K f i o −−=)(k P o )(k P o )(k P )1(−k PThe algorithm for calculating the responsive force can be described as followed. )(k P o and )(k P i , which are used in force calculation, are found from algorithm I and II, depends on the condition of the current position (P(k)) and the previous position (P(k-1)) of the probe point input. The collision occurs when the probe point is inside the surface for the first time which means P(k-1) is outside the surface (0))1((>−k P S ) and P(k) is inside the surface (0))((<k P S ). When the collision occurs, algorithm I is used to define the new interaction points )(k P o and )(k P i . After the collision and if P(k) still be inside the surface, algorithm II will be used to define the next interaction points. Note that, in the special case where point )(k P o which is calculated from algorithm II is too far outside the surface ( ε>))((k P S o ), the new interaction points will have to be recalculated as the surface crossing point on the line segment from )(k P o to )(k P i using algorithm I.Algorithm I is performed when the probe point P(k) collideswith the surface for the first time. The surface contact point )(k P o is established at the point where the surface crosses the line segment between point P(k) and P(k-1). The implicit function S(p) evaluates zero at )(k P o . Since the update rate in haptic rendering process is very high, only a small movement is permitted within one interval (i.e.distance between P(k) and P(k-1) is small). The surface crossing point where 0))((=k P S o can be found using binary search along the segment between P(k) and P(k-1). (Figure 2.3)Figure 2.3. The surface contact point )(k P o is found on the line segment between P(k) and P(k-1)After the surface contact point )(k P o is founded, theinteraction point inside the surface )(k P i is established by mapping the probe point input P(k) onto the surface normal vector at )(k P o so that the direction of force vector calculated from )(k P o and )(k P i is appropriately defined with respect to the implicit surface.Figure 2.4 The interaction point inside the surface )(k P i is established from P(k) and the surface normal at )(k P o It is also important to make sure that )(k P o is not inside the surface.Therefore, when implicit function evaluated at )(k P o is less than zero, )(k P o has to be moved outside the surface by a very small distance (specified by α as shown in algorithm I) in the direction of surface normal evaluated at )(k P o .Algorithm I: Find the new interaction point%% Find Po(k)If P(k) is inside the object for the first time%% Binary search for the interaction point on the surface DoPo(k) = (P(k)+P(k-1))/2;Until S(Po(k)) < ε or MAX_N iter.%% Find Pi(k)%% N(P(k)) is the surface normal vector at point P(k)))()(()(k P k P k vP o −=))())(())(())(())(()((()()(k vP k P N k P N k P N k P N k vP k P k P o o o o i −⋅⋅+=%%check if Po(k) is inside the surface then modify Po(k)if S(Po(k)) < 0))(())(())(()()(k P N k P N k P S k P k P o o o o o α−=return Po(k) and Pi(k)When the probe point is still inside a surface after the first collision with the surface, Algorithm II is used to move the interaction point on the surface from the previous state )1(−k P o to the new position on the surface based on the movement of probe point P(k-1) to P(k). The movement of the probe point inside the surface is used to calculated the interaction point )(k P o , by mapping the vector from P(k-1) to P(k)onto the tangent plane at )1(−k P o . )(k P o is modified by a small distance in the direction of the surface normal evaluated at )1(−k P o to guarantee that )(k P o will not be inside the surface.(k P P(k)P(k-1))(k P i is also calculated in the same way as in Algorithm I so that the direction of reactive force is defined correctly with respect to the implicit surface.Figure 2.5 The surface contact point )(k P o is established based on the movement of probe point inside the surfaceAlgorithm II: Find the next interaction point %% Find Po(k)))1()(()(−−=k P k P k V )))1(())1(()1((())1(())1(())1(())1(()()(()1()(−−−⋅−−−⋅−−⋅−+−=k P N k P N k P S k P N k P N k P N k P N k V k V k P k P o o o o o o o o o α%% Find Pi(k)))()(()(k P k P k vP o −=))())(())(())(())(()((()()(k vP k P N k P N k P N k P N k vP k P k P o o o o i −⋅⋅+=return Po(k) and Pi(k)Rendering the internal content of an implicit objectNormally, a solid model is represented by a closed surfaceseparated the outside space from the inside. In the regular solid model,no information about what contains inside the surface is available. In practice, internal content of an object often provides significant amount of useful information, such as property of composite material that contained inside the object or a special form of internal structure of the object. The ability to sense internal content of an object becomes more important in several applications especially in biomedical and electronics applications.The internal content of geometric model can be described bymanifold with boundary. The reason that manifolds with boundary are used is so that the transition between internal and external space can only occur within the restrictive region. Using the manifold with boundary representation, the opening region bounded by the manifold boundary is defined as ‘port’ where the transition between spaces takes place. In other word, internal content of an object can only be displayed after the probe point has entered a defined region called ‘port’. The concept of traveling through ‘port’ allows interior and exterior force of the geometric model to be distinctively displayed via state transition.Manifold with boundary is constructed from implicitsurface primitives. Implicit surface representation is required because implicit function posses unique properties that the surface can be simply reversed by a sign inversion and inside/outside can be easily determined by evaluating an implicit function. The exterior force is defined to simulate the responsive force from contacting the exterior surface of a geometric model. Haptic rendering process for the exterior surface can be performed in the same way as for a regular two-dimensional manifold surface described in the previous section.Internal content can be displayed by various techniques depends on material property or the arrangement of the internal structure. For example, viscous effect can be used to represent non-solid viscous substance that confined inside the implicit surface or a spatial periodic oscillation can be used to represent a grid-like structure of internal material.As a basic example, the manifold with boundaryconstructed from an implicit half-plane and a sphere is demonstrated (as shown in figure 2.6). The state transition conditions for these two implicit primitives are shown in Table I. Four distinctive regions are defined in the state transition table. State transition is only performed when the probe point is traveling through the ‘port’ which defined as an intersect region between a half-plane and a sphere. If the probe point is inside both surfaces (a sphere and a half-plane), the exterior surface of a sphere will be displayed unless the probe point has entered ‘port’ in the previous state. In that case, the internal content of a sphere will be displayed instead. In this example, a sphere container that contains non-solid viscous substance is simulated. The exterior force is calculated as the responsive force from contacting the outer shell of a sphere container. The interior force is a combination of the viscous resisting force and contacting force from the surface interior.The viscous resisting force is proportional to velocity of the probe point, which can be described by the equation )(k V B F v v −= , wherev B is the viscous coefficient of a simulated non-solid substance andV(k) is the probe point velocity. The contacting force from the surface interior is calculated in the same way as the responsive force from contacting a regular two-manifold, except that the reversed implicit function of sphere is used instead. At each servo loop, collision detection between the probe point and implicit surfaces is performed for each surface in the same way as described in the method for a regular two-manifold. The proper responsive force is calculated based on the status of the probe point as shown in the state transition table.Figure 2.6. Manifold with boundary that represents a sphere container, constructed from a sphere and a half-plane.V (k)P(k-1)State Sphere Half-plane1IN IN If (the previous state is 2)ENTER_PORTElseIf (ENTER_PORT has occurred)Find a viscous resisting forceElseFind a contact force of sphere-exterior surface2IN OUT If (the previous state is 1)EXIT_PORT3OUT IN If (ENTER_PORT has occurred)Find a contact force of reversed -sphere surfaceElseF = 04OUT OUTF = 0Table I. State transition table for 2 implicit primitivesMore complex example is illustrated in figure 2.7. Compositematerial is confined inside a cylinder tube. In the case where n implicitsurfaces are required to describe a model, 2n distinctive regions areintroduced. Different material properties will be displayed as the probepoint passes through each layer. Although state transition table for thismodel will be much more complicate than in the previous example, it isbased on the concept where conditions can be tested iteratively. (i.e. theprobe point cannot enter port 2,3,4 unless it has entered port 1,therefore it is not necessary to test every conditions at each servo loop.)The rendering algorithm for complex internal structure within ageometrical model can be simplified by applying this concept of stateFigure 2.7 A cylinder tube that contains composite material can bemodeled using state transition via ‘port’ concept.State transition via ‘port’ can also be applied to an applicationwhere the ‘flow direction’ is concerned. The flow direction means thatonly a certain direction is allowed to travel within a defined space.According to the state transition via ‘port’ paradigm, arbitrary regioncan not be accessed unless the probe point has traveled through theprior region defined by a sequence of ‘port’.Objects with complicate shape can be modeled bothexternally and internally by applying constructing techniques to basicimplicit primitives as we has discussed earlier. The haptic renderingalgorithm proposed here emphasizes the advantage of implicit surfacerepresentation in haptic applications where the object interior can behaptically displayed as well as the exterior surface.HAPTIC INTERFACE SYSTEMThe implicit haptic rendering algorithm described above wasimplemented in the haptic interface system at Biorobotics LaboratoryUniversity of Washington using Excalibur, the 3 degree-of-freedomhaptic linear haptic display device (shown in figure 3.1). TheExcalibur was designed and manufactured by HapticTechnologies,Inc. of Seattle, WA. The user can provide the positional input bymoving the handgrip that connects to light linkage elements. The 3-dimensional force feedback is generated from motors mounted on thebase of the mechanism. The Excalibur is using steel cabletransmission that enables high forces and high rigidity in the three-axis translation motions. The workspace of the device is300x300x200 mm3 with the maximum force of 100 N and theposition resolution of 0.008 mm.The haptic rendering algorithm is implemented on a 266 MHzPentium II processor PC that controlled the Excalibur in real-timewith the update rate of 1 KHz.Figure 3.1. Excalibur, the 3 degree-of-freedom haptic linear hapticdisplay deviceSeveral examples of implicit models were hapticallyrendered using the methods described in the previous section. Theseexamples include1.Basic shapes such as sphere, ellipsoid, cube and heart.2.Metaballs: 2-5 blended spheres.3.CSG: union, intersection and difference of basic primitives suchas ellipsoid and cube.4.Implicit container: A sphere container with the opening on thetop (Figure 3) that contains liquid with adjustable viscouscoefficient.The user was able to correctly recognize the shape ofrendered objects by using tactile senses alone without graphicalinterface. The proposed algorithm is simple and computationallyinexpensive enough to be performed within 1 millisecond timeinterval. However, undesirable oscillation sometimes occurs in highconvex curvature region. Applying the appropriate adjustment to thenormal vector calculation procedure may alleviate this problem. Thebounding box or localization technique should be implemented in thealgorithm so that more complex model which consists of a largenumber of primitives can be haptically displayed in real-time.CONCLUSIONSThe implicit surface representation is well suited for hapticapplications, especially for modeling the object consisting of quadricprimitives. Constructing techniques in geometric modeling allow complex shapes to be haptically displayed as well as basic shapes. The haptic rendering methods for a regular two-dimensional manifold and for the internal content of an object were presented in this paper. The properties of implicit surface representation are suitable to be applied to haptic rending process for both classes of objects. The new concept of state transition via ‘port’ model is introduced so that the internal content of an object can be rendered haptically. Haptic rendering of various implicit models was demonstrated including the combination of geometric primitives and the model of implicit container that contains non-solid viscous substance. Unfortunately, implicit surface representation is still not widely used in geometric modeling compare to other well-established methods such as parametric surface representation or boundary representation. This factor may prevent the implicit haptic rendering technique to be applied to the existing geometric modeling system. Hopefully, as the attention of implicit surface modeling in graphics increases, it will consequently bring more interest into implicit haptic rendering applications in the near future. REFERENCES[1] Y.Adachi, T.Kumano and K.Ogino, Intermediate representation for stiff virtual objects, Proc. Virtual Reality Annual Intl. Symp., (Research Triangle Park, NC, Mar 11-15, 1995),203-210.[2] R.S. Avila and L.M Sobierajski, A haptic interaction method for volume visualization, Visualization ’96 Proc. (October, 1996).[3] J.R.Davis, R.Nagel and W.Guber, A model making and display technique for 3D pictures, Proc.7th Annual Meeting ofUAIDE,(1968),47-72.[4] W.R.Mark, S.C.Randolph, M.Finch, J.M.Van Verth, andR.M.Taylor III, Adding force feedback to graphics systems: issues and solutions, Proc. SIGGRAPH 96, (New Orleans, Aug 4-91994),447-452.[5] T.Massie and K.Salisbury, The PHANToM haptic interface: a device for probing virtual objects, Proc. Of ASME Winter Annual Meeting, Symp. Haptic Interfaces for Virtual Environment and Teleoperator Systems, (Chicago, IL, Nov. 1994).[6] P.Mittelman, Computer graphics at MAGI, Computer Graphics 83, (London, Online Publications, 1983), 291-301.[7] H.B. Morgenbesser and M.A. Srinivasan, Force shading for haptic shape perception, Proc.ASME Winter Annual Meeting, (1996).[8] H. Nishimura, M. Hirai, T. Kawai, T. Kawata, I. Shirakawa and K. Omura, Object modeling by distribution function and a method of image generation, Trans. Inst. Of Elec. And Comm. Eng. Of Japan, vol. J68-D, no. 4, (1985), 718-725.[9] A. Ricci, A constructive geometry for computer graphics, The Computer Journal, Vol.16, No.2, (1973), 157-160.[10] D. Ruspini, K.Kolarov and O.Khatib, Robust Haptic Display of Graphical Environments, in: J.K.Salisbury and M.A. Srinivasan, ed., Proc. Of the First PHANToM Users Group Workshop, M.I.T. Artificial Intelligence Laboratory Technical Report AITR-1596, (1996).[11] K.Salisbury and C. Tar, Haptic rendering of surfaces defined by implicit functions, Proc. ASME Dyn. Sys. and Control Div., DSC-Vol.61,(1997),61-67.[12] T.V.Thompson II, D.E.Johnson and E. Cohen, Direct haptic rendering of sculptured models, Proc. Sym. Interactive 3D Graphics, (Providence, RI, April 27-30, 1997) 1-10.[13] C. Zilles and K. Salisbury, A constraint-based god-object method for haptic display, Proc.IROS-95, Vol.3, (Pittsburgh, Aug 6-9, 1995), 146-151.。