Indoor thermal comfort studies based on physiological parameter measurement and questionnaire i
浙江台州传统民居冬季室内热舒适评价研究
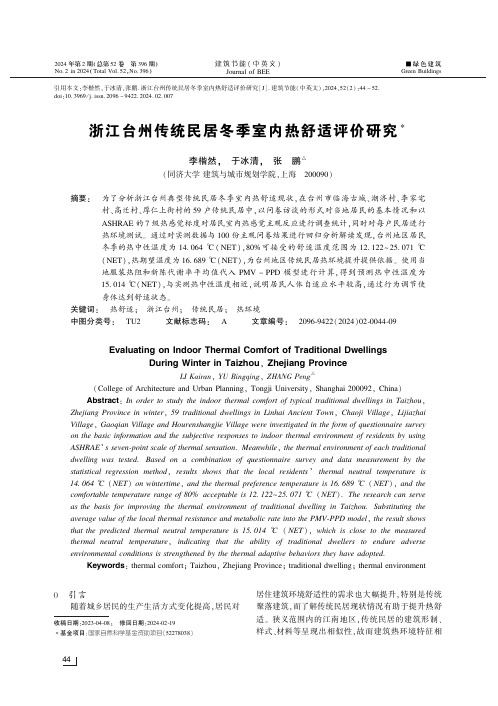
as the basis for improving the thermal environment of traditional dwelling in Taizhou. Substituting the
During Winter in Taizhouꎬ Zhejiang Province
LI Kairanꎬ YU Bingqingꎬ ZHANG Peng △
( College of Architecture and Urban Planningꎬ Tongji Universityꎬ Shanghai 200092ꎬ China)
的热舒适性需要进一步改善
[3]
ꎮ 为了提升传统民居
的冬季热舒适度ꎬ在现有建筑的基础上无论是安装集
中供暖系统还是加保温层提升墙体保温性能的方式ꎬ
都需要了解室内热中性温度和舒适阈值ꎮ
受温度下限ꎬ居民自适应水平较高 [11ꎬ12] ꎮ Chengcheng
现在江南地区的西递、志峰、俞源村以及南京小西湖
片区的传统民居中ꎬ居民对高湿度的容忍度和对寒冷
适研究已积累部分成果ꎮ 通过将南阳、上海、湖北随
对西递徽州传统民居的室内热环境测试数据分析得
州 / 咸宁、罗田、岳阳、台州仙居、粤东农村住宅的冬季
出ꎬ建筑围护结构保温性密闭性差ꎬ建筑保温防寒效
果差ꎬ冬季室内阴冷潮湿
ꎮ 黄志甲等人分析造成徽
[1]
州民居热不舒适的主要原因为室内温度远低于舒适
温度范围ꎬ且相对湿度过高
上海某办公楼VAV空调系统设计
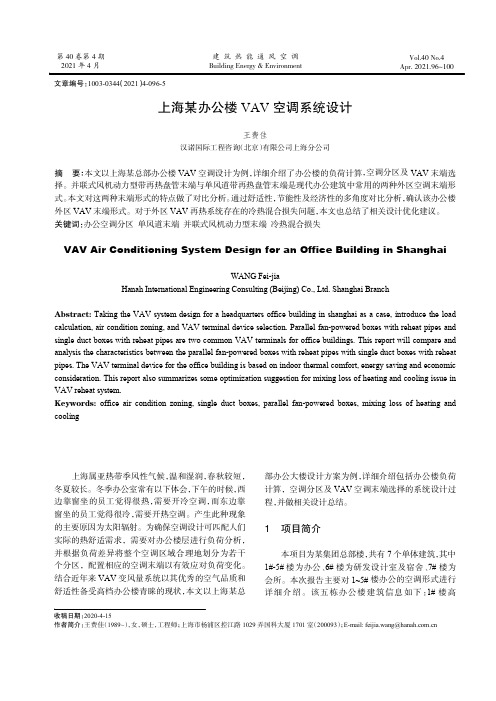
上海某办公楼 VAV 空调系统设计王费佳汉诺国际工程咨询 (北京) 有限公司上海分公司摘 要: 本文以上海某总部办公楼 VAV 空调设计为例, 详细介绍了办公楼的负荷计算, 空调分区及 VAV 末端选 择。
并联式风机动力型带再热盘管末端与单风道带再热盘管末端是现代办公建筑中常用的两种外区空调末端形式。
本文对这两种末端形式的特点做了对比分析。
通过舒适性, 节能性及经济性的多角度对比分析, 确认该办公楼 外区VAV 末端形式。
对于外区VAV 再热系统存在的冷热混合损失问题, 本文也总结了相关设计优化建议。
关键词: 办公空调分区 单风道末端 并联式风机动力型末端 冷热混合损失VAV Air Conditioning System Design for an Office Building in ShanghaiWANG FeijiaHanah International Engineering Consulting (Beijing)Co.,Ltd.Shanghai BranchAbstract: Taking the VAV system design for a headquarters office building in shanghai as a case,introduce the load calculation,air condition zoning,and VAV terminal device selection.Parallel fanpowered boxes with reheat pipes and single duct boxes with reheat pipes are two common VAV terminals for office buildings.This report will compare and analysis the characteristics between the parallel fanpowered boxes with reheat pipes with single duct boxes with reheat pipes.The VAV terminal device for the office building is based on indoor thermal comfort,energy saving and economic consideration.This report also summarizes some optimization suggestion for mixing loss of heating and cooling issue in VAV reheat system.Keywords: office air condition zoning,single duct boxes,parallel fanpowered boxes,mixing loss of heating and cooling收稿日期: 2020415 作者简介: 王费佳 (1989~), 女, 硕士, 工程师; 上海市杨浦区控江路 1029弄国科大厦 1701室 (200093); Email:*********************.cn上海属亚热带季风性气候,温和湿润, 春秋较短, 冬夏较长。
既有建筑节能改造和对策研究04-外文翻译
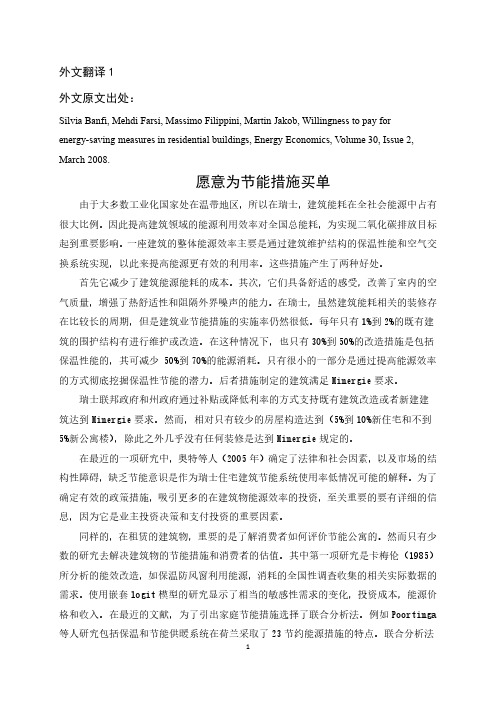
外文翻译1外文原文出处:Silvia Banfi,Mehdi Farsi,Massimo Filippini,Martin Jakob,Willingness to pay forenergy-saving measures in residential buildings,Energy Economics,Volume30,Issue2, March2008.愿意为节能措施买单由于大多数工业化国家处在温带地区,所以在瑞士,建筑能耗在全社会能源中占有很大比例。
因此提高建筑领域的能源利用效率对全国总能耗,为实现二氧化碳排放目标起到重要影响。
一座建筑的整体能源效率主要是通过建筑维护结构的保温性能和空气交换系统实现,以此来提高能源更有效的利用率。
这些措施产生了两种好处。
首先它减少了建筑能源能耗的成本。
其次,它们具备舒适的感受,改善了室内的空气质量,增强了热舒适性和阻隔外界噪声的能力。
在瑞士,虽然建筑能耗相关的装修存在比较长的周期,但是建筑业节能措施的实施率仍然很低。
每年只有1%到2%的既有建筑的围护结构有进行维护或改造。
在这种情况下,也只有30%到50%的改造措施是包括保温性能的,其可减少50%到70%的能源消耗。
只有很小的一部分是通过提高能源效率的方式彻底挖掘保温性节能的潜力。
后者措施制定的建筑满足Minergie要求。
瑞士联邦政府和州政府通过补贴或降低利率的方式支持既有建筑改造或者新建建筑达到Minergie要求。
然而,相对只有较少的房屋构造达到(5%到10%新住宅和不到5%新公寓楼),除此之外几乎没有任何装修是达到Minergie规定的。
在最近的一项研究中,奥特等人(2005年)确定了法律和社会因素,以及市场的结构性障碍,缺乏节能意识是作为瑞士住宅建筑节能系统使用率低情况可能的解释。
为了确定有效的政策措施,吸引更多的在建筑物能源效率的投资,至关重要的要有详细的信息,因为它是业主投资决策和支付投资的重要因素。
有关仿生建筑的英语作文
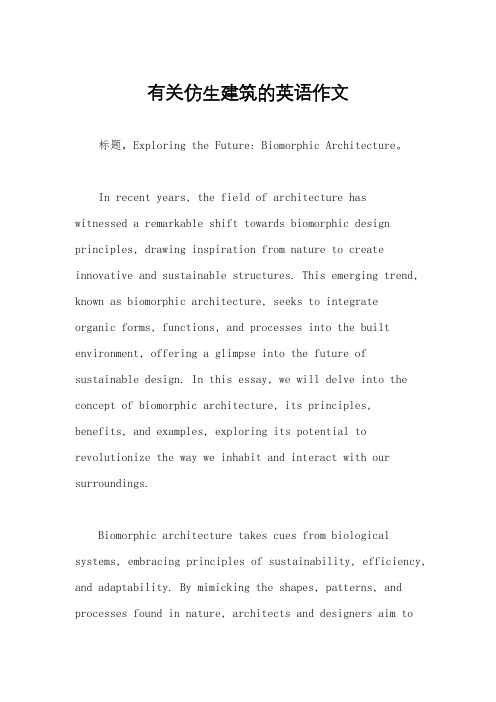
有关仿生建筑的英语作文标题,Exploring the Future: Biomorphic Architecture。
In recent years, the field of architecture haswitnessed a remarkable shift towards biomorphic design principles, drawing inspiration from nature to create innovative and sustainable structures. This emerging trend, known as biomorphic architecture, seeks to integrateorganic forms, functions, and processes into the built environment, offering a glimpse into the future of sustainable design. In this essay, we will delve into the concept of biomorphic architecture, its principles, benefits, and examples, exploring its potential to revolutionize the way we inhabit and interact with our surroundings.Biomorphic architecture takes cues from biological systems, embracing principles of sustainability, efficiency, and adaptability. By mimicking the shapes, patterns, and processes found in nature, architects and designers aim tocreate buildings that harmonize with their environmentwhile enhancing the well-being of occupants. This approach not only reduces the environmental impact of constructionbut also fosters a deeper connection between humans and the natural world.One of the key principles of biomorphic architecture is biomimicry, the practice of emulating nature's designs and strategies to solve human challenges. From the lotus-inspired roof of the Singapore ArtScience Museum to the termite mound-inspired Eastgate Centre in Zimbabwe, biomimetic designs have proven to be both aesthetically striking and functionally efficient. By studying the waysin which organisms regulate temperature, optimize structure, and conserve resources, architects can develop innovative solutions for energy-efficient building design, ventilation systems, and material selection.Moreover, biomorphic architecture emphasizes the use of sustainable materials and construction techniques,prioritizing renewable resources and minimizing waste. Bamboo, for example, is a versatile and rapidly renewablematerial that has been used in projects such as the Green School in Bali, showcasing its strength, flexibility, and eco-friendly properties. Similarly, 3D printing technology has enabled the creation of intricate structures inspired by natural forms, such as the "Hy-Fi" tower constructed from organic mushroom-based materials.In addition to environmental benefits, biomorphic architecture prioritizes human well-being by creating spaces that promote health, comfort, and productivity. Biophilic design principles, which integrate natural elements into the built environment, have been shown to reduce stress, increase productivity, and enhance creativity. Features such as green roofs, indoor gardens, and natural lighting not only improve air quality and thermal comfort but also foster a sense of connection to the outdoors, supporting overall health and well-being.Furthermore, biomorphic architecture encourages a holistic approach to urban planning, envisioning cities as living ecosystems that interact harmoniously with their inhabitants and surroundings. Concepts such as verticalgardens, urban forests, and pedestrian-friendly design aim to create sustainable, resilient urban environments that prioritize human-scale development and biodiversity. Projects like the Bosco Verticale in Milan and the High Line in New York City exemplify this approach, transforming disused urban spaces into vibrant green corridors that promote biodiversity and community engagement.In conclusion, biomorphic architecture represents a paradigm shift in the field of design, harnessing the power of nature to create sustainable, resilient, and human-centered built environments. By embracing biomimicry, sustainable materials, and biophilic design principles, architects and designers can pave the way for a more harmonious relationship between humanity and the natural world. As we continue to face pressing challenges such as climate change, resource depletion, and urbanization, biomorphic architecture offers a beacon of hope for a more sustainable and regenerative future. Through innovation, collaboration, and a deep respect for the wisdom of nature, we can unlock the full potential of biomorphic architecture to shape a better world for generations to come.。
室内热舒适性问题
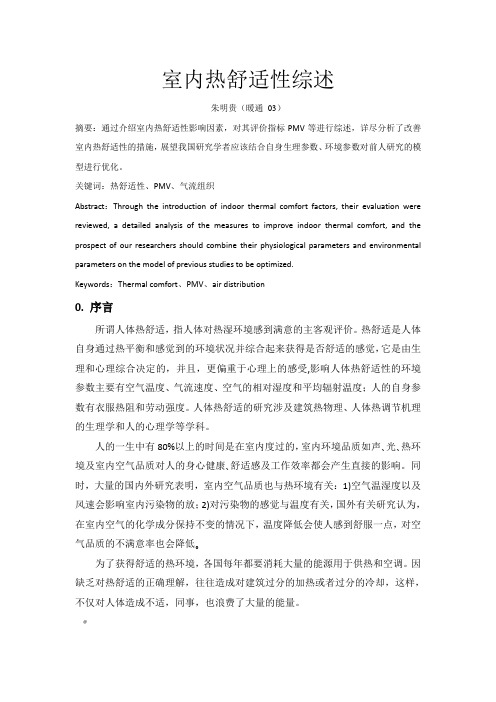
室内热舒适性综述朱明贵(暖通03)摘要:通过介绍室内热舒适性影响因素,对其评价指标PMV等进行综述,详尽分析了改善室内热舒适性的措施,展望我国研究学者应该结合自身生理参数、环境参数对前人研究的模型进行优化。
关键词:热舒适性、PMV、气流组织Abstract:Through the introduction of indoor thermal comfort factors, their evaluation were reviewed, a detailed analysis of the measures to improve indoor thermal comfort, and the prospect of our researchers should combine their physiological parameters and environmental parameters on the model of previous studies to be optimized.Keywords:Thermal comfort、PMV、air distribution0.序言所谓人体热舒适,指人体对热湿环境感到满意的主客观评价。
热舒适是人体自身通过热平衡和感觉到的环境状况并综合起来获得是否舒适的感觉,它是由生理和心理综合决定的,并且,更偏重于心理上的感受,影响人体热舒适性的环境参数主要有空气温度、气流速度、空气的相对湿度和平均辐射温度;人的自身参数有衣服热阻和劳动强度。
人体热舒适的研究涉及建筑热物理、人体热调节机理的生理学和人的心理学等学科。
人的一生中有80%以上的时间是在室内度过的,室内环境品质如声、光、热环境及室内空气品质对人的身心健康、舒适感及工作效率都会产生直接的影响。
同时,大量的国内外研究表明,室内空气品质也与热环境有关:1)空气温湿度以及风速会影响室内污染物的放;2)对污染物的感觉与温度有关,国外有关研究认为,在室内空气的化学成分保持不变的情况下,温度降低会使人感到舒服一点,对空气品质的不满意率也会降低。为了获得舒适的热环境,各国每年都要消耗大量的能源用于供热和空调。
冬季外窗和外墙冷辐射的热舒适限值

128暖通空调HV&AC2021年第51卷第5期[引用本文]王昭俊,徐云艳,苏小文.冬季外窗和外墙冷辐射的热舒适限值[J].暖通空调,2021,51(5) :128_132|| 冬季外窗和外墙冷辖射的热舒适限值*哈尔滨工业大学寒地城乡人居环境科学与技术工业和信息化部重点实验室王昭俊☆徐云艳苏小文摘要严寒和寒冷地区冬季建筑外墙和外窗的内表面温度较低,形成的冷辐射会引起人体 局部热不舒适。
为了研究冷壁面温度引起热不舒适的限值,基于人工气候室的实验研究数据,研 究了冷辐射最不利工况下的人体热反应。
结果表明:小腿对外墙和外窗冷辐射最敏感,建立了最 不利工况下人体全身热感觉和局部热感觉的相关性模型;当室内空气温度为19 X:,且受试者距离 外墙和外窗1m时,20名受试者的平均热感觉投票值为一0.82,即为20%不满意率的下限值;该 最不利工况下外墙和外窗内表面温度与室内空气温度之差的限值为2.6 X:。
关键词冷辐射冷表面温度限值局部热感觉不满意率建筑热工设计Comfort limit for cold radiation from cold walls andwindows in winterBy Wong Zhoojun^ ,Xu Yunyon ond Su XioowenA bstract Local human thermal discomfort may exist because of cold radiation from a cold surface,such as a cold external wall or window with lower inner surface temperature in severe cold and cold zones in winter. In order to study the limit of cold surface temperature causing discom fort,analyses the human thermal responses under the most unfavorable cold radiant condition based on the experimental data of an artificial climate chamber. The results show that the calf of subjects is the most sensitive part to cold radiation o f external walls and windows. Establishes a correlation model of the overall and local thermal sensations of the human body under the most unfavorable conditions. When the indoor air temperature is 19C and the subjects are 1 m away from an external wall and an external window, the average thermalsensation vote of 20 subjects is -0.82,which is the lower limit of 20% o f dissatisfied percentage. The limit o f the difference is 2. 6 °C between the indoor air temperature and the inner surface temperature of the external wall and the external window under this condition.Keywords cold radiation,limit of cold surface temperature, local thermal sensation, dissatisfied percentage, building thermal design★ Harbin Institute of Technology,Harbin,China〇引言随着生活水平的提高,人们对提升人居环境的 热舒适更加关注。
冬季西安农村住宅室内热舒适研究
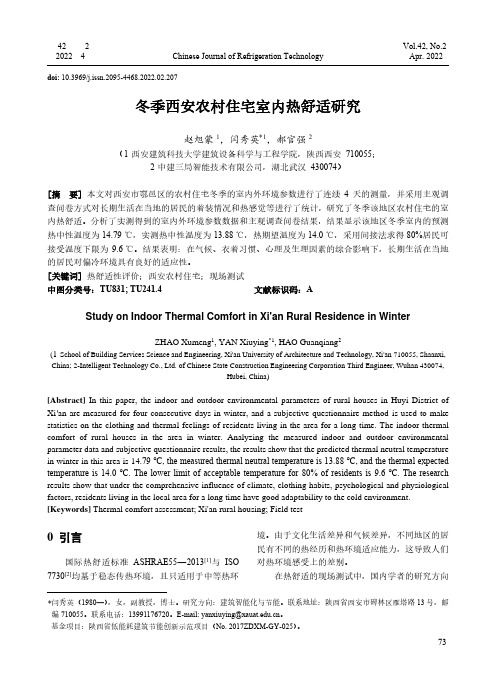
doi: 10.3969/j.issn.2095-4468.2022.02.207冬季西安农村住宅室内热舒适研究赵旭蒙1,闫秀英*1,郝官强2(1-西安建筑科技大学建筑设备科学与工程学院,陕西西安 710055;2-中建三局智能技术有限公司,湖北武汉 430074)[摘 要] 本文对西安市鄠邑区的农村住宅冬季的室内外环境参数进行了连续4天的测量,并采用主观调查问卷方式对长期生活在当地的居民的着装情况和热感觉等进行了统计,研究了冬季该地区农村住宅的室内热舒适。
分析了实测得到的室内外环境参数数据和主观调查问卷结果,结果显示该地区冬季室内的预测热中性温度为14.79 ℃,实测热中性温度为13.88 ℃,热期望温度为14.0 ℃,采用间接法求得80%居民可接受温度下限为9.6 ℃。
结果表明:在气候、衣着习惯、心理及生理因素的综合影响下,长期生活在当地的居民对偏冷环境具有良好的适应性。
[关键词] 热舒适性评价;西安农村住宅;现场测试 中图分类号:TU831; TU241.4文献标识码:AStudy on Indoor Thermal Comfort in Xi'an Rural Residence in WinterZHAO Xumeng 1, YAN Xiuying *1, HAO Guanqiang 2(1-School of Building Services Science and Engineering, Xi'an University of Architecture and Technology, Xi'an 710055, Shaanxi, China; 2-Intelligent Technology Co., Ltd. of Chinese State Construction Engineering Corporation Third Engineer, Wuhan 430074,Hubei, China)[Abstract] In this paper, the indoor and outdoor environmental parameters of rural houses in Huyi District of Xi 'an are measured for four consecutive days in winter, and a subjective questionnaire method is used to make statistics on the clothing and thermal feelings of residents living in the area for a long time. The indoor thermal comfort of rural houses in the area in winter. Analyzing the measured indoor and outdoor environmental parameter data and subjective questionnaire results, the results show that the predicted thermal neutral temperature in winter in this area is 14.79 ℃, the measured thermal neutral temperature is 13.88 ℃, and the thermal expected temperature is 14.0 ℃. The lower limit of acceptable temperature for 80% of residents is 9.6 ℃. The research results show that under the comprehensive influence of climate, clothing habits, psychological and physiological factors, residents living in the local area for a long time have good adaptability to the cold environment. [Keywords] Thermal comfort assessment; Xi'an rural housing; Field test*闫秀英(1980—),女,副教授,博士。
暖通毕业设计外文翻译
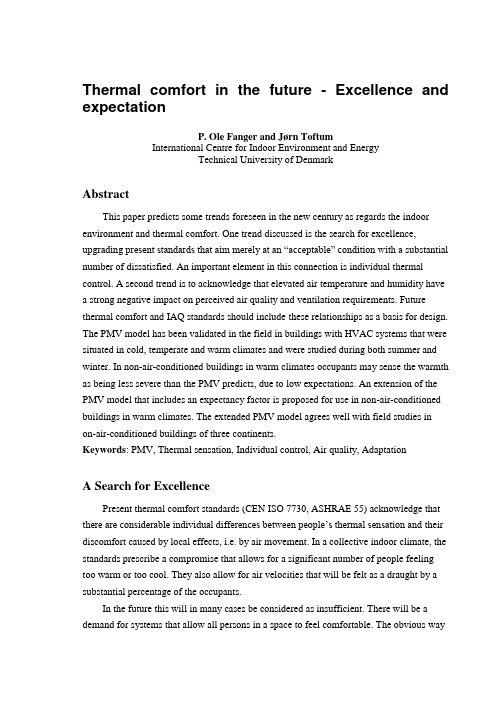
Thermal comfort in the future - Excellence and expectationP. Ole Fanger and Jørn ToftumInternational Centre for Indoor Environment and EnergyTechnical University of DenmarkAbstractThis paper predicts some trends foreseen in the new century as regards the indoor environment and thermal comfort. One trend discussed is the search for excellence, upgrading present standards that aim merely at an “acceptable” condition with a substantial number of dissatisfied. An important element in this connection is individual thermal control. A second trend is to acknowledge that elevated air temperature and humidity have a strong negative impact on perceived air quality and ventilation requirements. Future thermal comfort and IAQ standards should include these relationships as a basis for design. The PMV model has been validated in the field in buildings with HVAC systems that were situated in cold, temperate and warm climates and were studied during both summer and winter. In non-air-conditioned buildings in warm climates occupants may sense the warmth as being less severe than the PMV predicts, due to low expectations. An extension of the PMV model that includes an expectancy factor is proposed for use in non-air-conditioned buildings in warm climates. The extended PMV model agrees well with field studies inon-air-conditioned buildings of three continents.Keywords: PMV, Thermal sensation, Individual control, Air quality, AdaptationA Search for ExcellencePresent thermal comfort standards (CEN ISO 7730, ASHRAE 55) acknowledge that there are considerable individual differences between people’s thermal sensation and their discomfort caused by local effects, i.e. by air movement. In a collective indoor climate, the standards prescribe a compromise that allows for a significant number of people feeling too warm or too cool. They also allow for air velocities that will be felt as a draught by a substantial percentage of the occupants.In the future this will in many cases be considered as insufficient. There will be a demand for systems that allow all persons in a space to feel comfortable. The obvious wayto achieve this is to move from the collective climate to the individually controlled local climate. In offices, individual thermal control of each workplace will be common. The system should allow for individual control of the general thermal sensation without causing any draught or other local discomfort. We know the range of operative temperatures required in a workplace to satisfy nearly everybody (Wyon 1996; Fanger 1970) and we know the sensitivity to draught from a wide range of studies. A search for excellence involves providing all persons in a space with the means to feel thermally comfortable without compromise.Thermal Comfort and IAQPresent standards treat thermal comfort and indoor air quality separately, indicating that they are independent of each other. Recent research documents that this is not true (Fang et al. 1999; Toftum et al. 1998). The air temperature and humidity combined in the enthalpy have a strong impact on perceived air quality, and perceived air quality determines the required ventilation in ventilation standards. Research has shown that dry and cool air is perceived as being fresh and pleasant while the same composition of air at an elevated temperature and humidity is perceived as stale and stuffy. During inhalation it is the convective and evaporative cooling of the mucous membrane in the nose that is essential for the fresh and pleasant sensation. Warm and humid air is perceived as being stale and stuffy due to the lack of nasal cooling. This may be interpreted as a local warm discomfort in the nasal cavity. The PMV model is the basis for existing thermal comfort standards. It is quite flexible and allows for the determination of a wide range of air temperatures and humidities that result in thermal neutrality for the body as a whole. But the inhaled air would be perceived as being very different within this wide range of air temperatures and humidities. An example: light clothing and an elevated air velocity or cooled ceiling, an air temperature of 28ºC and a relative humidity of 60% may givePMV=0, but the air quality would be perceived as stale and stuffy. A simultaneous request for high perceived air quality would require an air temperature of 20-22oC and a modest air humidity. Moderate air temperature and humidity decrease also SBS symptoms (Krogstad et al. 1991, Andersson et al. 1975) and the ventilation requirement, thus saving energy during the heating season. And even with air-conditioning it may be beneficial and save energy during the cooling season.PMV model and the adaptive modelThe PMV model is based on extensive American and European experiments involving over a thousand subjects exposed to well-controlled environments (Fanger 1970). The studies showed that the thermal sensation is closely related to the thermal load on the effector mechanisms of the human thermoregulatory system. The PMV model predicts the thermal sensation as a function of activity, clothing and the four classical thermal environmental parameters. The advantage of this is that it is a flexible tool that includes all the major variables influencing thermal sensation. It quantifies the absolute and relative impact of these six factors and can therefore be used in indoor environments with widely differing HVAC systems as well as for different activities and different clothing habits. The PMV model has been validated in climate chamber studies in Asia (de Dear et al. 1991; Tanabe et al. 1987) as well as in the field, most recently in ASHRAE’s worldwide research in buildings with HVAC systems that were situated in cold, temperate and warm climates and were studied during both summer and winter (Cena et al. 1998; Donini et al. 1996; de Dear et al. 1993a; Schiller et al. 1988). The PMV is developed for steady-state conditions but it has been shown to apply with good approximation at the relatively slow fluctuations of the environmental parameters typically occurring indoors. Immediately after an upward step-wise change of temperature, the PMV model predicts well the thermal sensation, while it takes around 20 min at temperature down-steps (de Dear et al. 1993b).Field studies in warm climates in buildings without air-conditioning have shown, however, that the PMV model predicts a warmer thermal sensation than the occupants actually feel (Brager and de Dear 1998). For such non-air-conditioned buildings an adaptive model has been proposed (de Dear and Brager 1998). This model is a regression equation that relates the neutral temperature indoors to the monthly average temperature outdoors. The only variable is thus the average outdoor temperature, which at its highest may have an indirect impact on the human heat balance. An obvious weakness of the adaptive model is that it does not include human clothing or activity or the four classical thermal parameters that have a well-known impact on the human heat balance and therefore on the thermal sensation. Although the adaptive model predicts the thermal sensation quite well for non-air-conditioned buildings of the 1900’s located in warm parts of the world, the question remains as to how well it would suit buildings of new types in the future where the occupants have a different clothing behaviour and a different activity pattern.Why then does the PMV model seem to overestimate the sensation of warmth in nonair-conditioned buildings in warm climates? There is general agreement thatphysiological acclimatization does not play a role. One suggested explanation is that openable windows in naturally ventilated buildings should provide a higher level of personal control than in air-conditioned buildings. We do not believe that this is true in warm climates. Although an openable window sometimes may provide some control of air temperature and air movement, this applies only to the persons who work close to a window. What happens to persons in the office who work far away from the window? And in warm climates, the normal strategy in naturally ventilated buildings is to cool the building during the night and then close the windows some time during the morning when the outdoor temperature exceeds the indoor temperature. Another obstacle is of course traffic noise, which makes open windows in many areas impossible. We believe that in warm climates air-conditioning with proper thermostatic control in each space provides a better perceived control than openable windows.Another factor suggested as an explanation to the difference is the expectations of the occupants. We think this is the right factor to explain why the PMV overestimates the thermal sensation of occupants in non-air-conditioned buildings in warm climates. These occupants are typically people who have been living in warm environments indoors and outdoors, maybe even through generations. They may believe that it is their “destiny” to live in environments where they feel warmer than neutral. If given a chance they may not on average prefer an environment that is different from that chosen by people who are used to air-conditioned buildings. But it is likely that they would judge a given warm environment as less severe and less unacceptable than would people who are used toair-conditioning. This may be expressed by an expectancy factor, e, to be multiplied with PMV to reach the mean thermal sensation vote of the occupants of the actualnon-air-conditioned building in a warm climate. The factor e may vary between 1 and 0.5. It is 1 for air-conditioned buildings. For non-air-conditioned buildings, the expectancy factor is assumed to depend on the duration of the warm weather over the year and whether such buildings can be compared with many others in the region that are air-conditioned. If the weather is warm all year or most of the year and there are no or few otherair-conditioned buildings, e may be 0.5, while it may be 0.7 if there are many other buildings with air-conditioning. For non-air-conditioned buildings in regions where the weather is warm only during the summer and no or few buildings have air-conditioning, the expectancy factor may be 0.7 to 0.8, while it may be 0.8 to 0.9 where there are many air-conditioned buildings. In regions with only brief periods of warm weather during the summer, the expectancy factor may be 0.9 to 1. Table 1 proposes a first rough estimationof ranges for the expectancy factor corresponding to high, moderate and low degrees of expectation.A second factor that contributes erroneously to the difference between the PMV and actual thermal sensation votes in non-air-conditioned buildings is the estimated activity. In many field studies in offices, the metabolic rate is estimated on the basis of a questionnaire identifying the percentage of time the person was sedentary, standing, or walking. This mechanistic approach does not acknowledge the fact that people, when feeling warm, unconsciously tend to slow down their activity. They adapt to the warm environment by decreasing their metabolic rate. The lower pace in warm environments should be acknowledged by inserting a reduced metabolic rate when calculating the PMV.To examine these hypotheses further, data were downloaded from the database of thermal comfort field experiments (de Dear 1998). Only quality class II data obtained in non-air-conditioned buildings during the summer period in warm climates were used in the analysis. Data from four cities (Bangkok, Brisbane, Athens, and Singapore) were included, representing a total of more than 3200 sets of observations (Busch 1992, de Dear 1985, Baker 1995, de Dear et al. 1991). The data from these four cities with warm climates were also used for the development of the adaptive model (de Dear and Brager 1998).For each set of observations, recorded metabolic rates were reduced by 6.7% for every scale unit of PMV above neutral, i.e. a PMV of 1.5 corresponded to a reduction in the metabolic rate of 10%. Next, the PMV was recalculated with reduced metabolic rates using ASHRAE’s thermal comfort tool (Fountain and Huizenga 1997). The resulting PMV values were then adjusted for expectation by multiplication with expectancy factors estimated to be 0.9 for Brisbane, 0.7 for Athens and Singapore and 0.6 for Bangkok. As an average for each building included in the field studies, Figure 1 and Table 2 compare the observed thermal sensation with predictions using the new extended PMV model for warm climates.Figure 1. Thermal sensation in non-air-conditioned buildings in warm climates.Comparison of observed mean thermal sensation with predictions made using the new extension of the PMV model for non-air-conditioned buildings in warm climates. The linesare based on linear regression analysis weighted according to the number of responsesTable 2. Non-air-conditioned buildings in warm climates.Comparison of observed thermal sensation votes and predictions made using the newextension of the PMV model.The new extension of the PMV model for non-air-conditioned buildings in warmclimates predicts the actual votes well. The extension combines the best of the PMV andthe adaptive model. It acknowledges the importance of expectations already accounted forby the adaptive model, while maintaining the PMV model’s classical thermal parametersthat have direct impact on the human heat balance. It should also be noted that the newPMV extension predicts a higher upper temperature limit when the expectancy factor islow. People with low expectations are ready to accept a warmer indoor environment. Thisagrees well with the observations behind the adaptive model.Further analysis would be useful to refine the extension of the PMV model, and additional studies in non-air-conditioned buildings in warm climates in different parts of the world would be useful to further clarify expectation and acceptability among occupants. It would also be useful to study the impact of warm office environments on work pace and metabolic rate.ConclusionsThe PMV model has been validated in the field in buildings with HVAC systems, situated in cold, temperate and warm climates and studied during both summer and winter. In non-air-conditioned buildings in warm climates, occupants may perceive the warmth as being less severe than the PMV predicts, due to low expectations.An extension of the PMV model that includes an expectancy factor is proposed for use in non-air-conditioned buildings in warm climates.The extended PMV model agrees well with field studies in non-air-conditioned buildings in warm climates of three continents.A future search for excellence will demand that all persons in a space be thermally comfortable. This requires individual thermal control.Thermal comfort and air quality in a building should be considered simultaneously. A high perceived air quality requires moderate air temperature and humidity. AcknowledgementFinancial support for this study from the Danish Technical research Council is gratefully acknowledged.ReferencesAndersson, L.O., Frisk, P., Löfstedt, B., Wyon, D.P., (1975), Human responses to dry, humidified and intermittently humidified air in large office buildings. Swedish Building Research Document Series, D11/75.ASHRAE 55-1992: Thermal environmental conditions for human occupancy. American Society of Heating, Refrigerating and Air-conditioning Engineers, Inc.Baker, N. and Standeven, M. (1995), A Behavioural Approach to Thermal Comfort Assessment in Naturally Ventilated Buildings. Proceedings from CIBSE National Conference, pp 76-84.Brager G.S., de Dear R.J. (1998), Thermal adaptation in the built environment: a literature review. Energy and Buildings, 27, pp 83-96.Busch J.F. (1992), A tale of two populations: thermal comfort in air-conditioned and naturally ventilated offices in Thailand. Energy and Buildings, vol. 18, pp 235-249.CEN ISO 7730-1994: Moderate thermal environments - Determination of the PMV and PPD indices and specification of the conditions for thermal comfort. International Organization for Standardization, Geneva.Cena, K.M. (1998), Field study of occupant comfort and office thermal environments in a hot-arid climate. (Eds. Cena, K. and de Dear, R.). Final report, ASHRAE 921-RP, ASHRAE Inc., Atlanta.de Dear, R., Fountain, M., Popovic, S., Watkins, S., Brager, G., Arens, E., Benton, C., (1993a), A field study of occupant comfort and office thermal environments in a hot humid climate. Final report, ASHRAE 702 RP, ASHRAE Inc., Atlanta.de Dear, R., Ring, J.W., Fanger, P.O. (1993b), Thermal sensations resulting from sudden ambient temperature changes. Indoor Air, 3, pp 181-192.de Dear, R. J., Leow, K. G. and Foo, S.C. (1991), Thermal comfort in the humid tropics: Field experiments in air-conditioned and naturally ventilated buildings in Singapore. International Journal of Biometeorology, vol. 34, pp 259-265.de Dear, R.J. (1998), A global database of thermal comfort field experiments. ASHRAE Transactions, 104(1b), pp 1141-1152.de Dear, R.J. and Auliciems, A. (1985), Validation of the Predicted Mean Vote model of thermal comfort in six Australian field studies. ASHRAE Transactions, 91(2), pp 452- 468. de Dear, R.J., Brager G.S. (1998), Developing an adaptive model of thermal comfort and preference. ASHRAE Transactions, 104(1a), pp 145-167.de Dear, R.J., Leow, K.G., and Ameen, A. (1991), Thermal comfort in the humid tropics - Part I: Climate chamber experiments on temperature preferences in Singapore. ASHRAE Transactions 97(1), pp 874-879.Donini, G., Molina, J., Martello, C., Ho Ching Lai, D., Ho Lai, K., Yu Chang, C., La Flamme, M., Nguyen, V.H., Haghihat, F. (1996), Field study of occupant comfort and office thermal environments in a cold climate. Final report, ASHRAE 821 RP, ASHRAE Inc., Atlanta.Fang, L., Clausen, G., Fanger, P.O. (1999), Impact of temperature and humidity on chemical and sensory emissions from building materials. Indoor Air, 9, pp 193-201. Fanger, P.O. (1970), Thermal comfort. Danish Technical Press, Copenhagen, Denmark. Fouintain, M.E. and Huizenga, C. (1997), A thermal sensation prediction tool for use by the profession. ASHRAE Transactions, 103(2), pp 130-136.Humphreys, M.A. (1978), Outdoor temperatures and comfort indoors. Building Research and Practice, 6(2), pp 92-105.Krogstad, A.L., Swanbeck, G., Barregård, L., et al. (1991), Besvär vid kontorsarbete med olika temperaturer i arbetslokalen - en prospektiv undersökning (A prospective study of indoor climate problems at different temperatures in offices), Volvo Truck Corp., Göteborg, Sweden.Schiller, G.E., Arens, E., Bauman, F., Benton, C., Fountain, M., Doherty, T. (1988) A field study of thermal environments and comfort in office buildings. Final report, ASHRAE 462 RP, ASHRAE Inc., Atlanta.Tanabe, S., Kimura, K., Hara, T. (1987), Thermal comfort requirements during the summer season in Japan. ASHRAE Transactions, 93(1), pp 564-577.Toftum, J., Jørgensen, A.S., Fanger, P.O. (1998), Upper limits for air humidity for preventing warm respiratory discomfort. Energy and Buildings, 28(3), pp 15-23.Wyon, D.P. (1996) Individual microclimate control: required range, probable benefits and current feasibility. Proceedings of Indoor Air ’96, vol. 1, pp 1067-1072未来的热舒适性——优越性和期望值P. Ole Fanger 和Jørn Toftum国际中心室内环境与能源丹麦科技大学摘要本文预期一些可在新世纪所预见的关于热舒适的室内环境的趋势。