Functional Expression and Purification
Protein Expression and purification
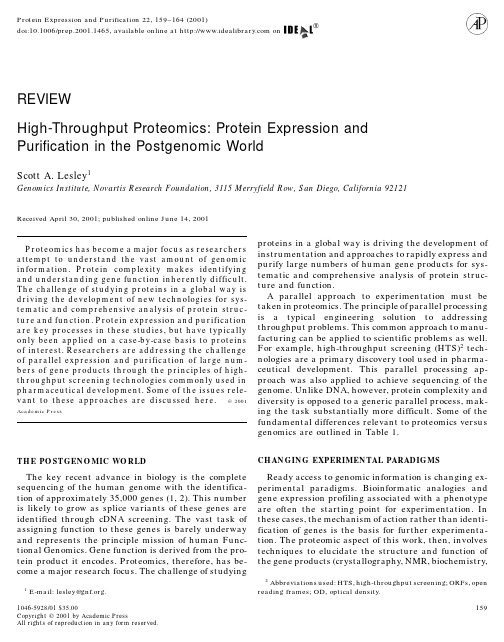
Protein Expression and Purification22,159–164(2001)160SCOTT A.LESLEYTABLE1Genomic versus Proteomic TechnologiesGenomic technologies(DNA)Proteomic technologies(protein) Identification Determined experimentally,bioinformatics Predicted from genomic informationFunction1-dimensional information storage3-dimensional organization of chemicalfunctionalitiesBuilding blocks4bases20ϩamino acidsDetection sensitivity PCR amplification techniques Direct detection methodsSynthetic approaches Cheap and efficient oligonucleotide synthesis Limited capacity of peptide synthesismethods combined with PCRSequence determination500–700bases common by automated sequencing Direct sequencing difficult,mass spectrometry Purification Generic methods Generic methods require modification of proteinthrough gene fusionAnalysis methods Typically employ enzymes,hybridization Chemical,biophysical,biochemicaland mutagenesis),interactions between proteins(two-are of primary interest and typically are expressed in hybrid),and global protein changes(2D gels and LC–a bacterial host.Often this approach leads to problems MS).Purified protein is often required in these studies associated with expression levels and proper folding of and defines the outputs of any parallel expression and the protein of interest.Flexibility in expression options purification process.is a key parameter.Pichia or baculovirus expressionsystems can offer effective alternatives to bacterial sys-tems.Each expression scenario requires a specific vec-GENE CLONING FOR EXPRESSIONtor.Recloning cDNAs into each of these specific vectors Determining gene function through genomics typi-is extremely labor-intensive.Recombinatorial cloning cally starts from a query of a database.Sequence infor-methods provide an opportunity to minimize the effort mation for the3.9billion bases of sequence from the required for alternate expression.human genome is now available(1,2).Access and inter-Two systems are commonly used for recombinatorial pretation of this information often require sophisticated cloning and shown in Fig.1.The cre–lox recombination bioinformatics software outside the scope of this dis-system described by Elledge utilizes a single recombina-cussion.Public archives such as the Unigene Data-tion to introduce the gene of interest into a recipient base(/)or TIGR(http://vector(3).This is an in vitro reaction combined with a /)provide bioinformatic access to many in-genetic selection for the recombinant vector.In this way teresting plete genomic sequence infor-the gene of interest is cloned once into a donor vector mation is now available through Celera(http://and can then be moved into any of a number of recipient /index.cfm).Future annotation of fullplasmids for expression in different hosts or to utilize sequence information will greatly expand the access todifferent purification tags.A similar system utilizes full-length cDNA sequences.The first requirement is converting this genomic in-lambda Int/Xis/IHF recombination at att sites(4)to formation into an actual cDNA clone of that gene.Am-achieve transfer of open reading frames(ORFs).This plification of full-length cDNAs via PCR is the typical system has the advantage of a precise ORF transfer to first step.Both reverse transcriptase and amplification the expression vector rather than the cointegrant vector polymerases typically are lacking in proofreading activ-product of the cre–lox system.With either system,the ity.Care must be taken to limit the number of steps of primary limitation is that translational fusion of the amplification and to use proofreading enzymes where recombination sites is typically required to maintain possible to minimize the probability of introducing un-the flexibility and utility of the recombination method wanted mutations.Tissue selection for cDNA librariesfor expression.In those cases,such as crystallography, also is an important consideration for attempting towhere translational fusions are potentially detrimental isolate genes as they must be expressed within thatto the protein,a conventional cloning approach is library source for successful amplification.This infor-more appropriate.mation is often obtained from cDNA or oligonucleotideRegardless of the cloning method,parallel expression expression arrays.and purification requires utilization of purification Amplified gene products are cloned into appropriatetags.Many options exist for this purpose.A comprehen-vectors for expression.Depending on the source of thesive review is beyond the scope of this article.A list of gene,the host,and the end use of the protein,manydifferent vectors may be appropriate.Eukaryotic genes some commonly used tags is shown in Table2.By farHIGH-THROUGHPUT PROTEOMICS161FIG.1.Strategies of recombinatorial cloning.Individual cDNAs are cloned into a donor vector that can then be recombined into any number of recipient vectors through recombinatorial cloning.One option is to form a cointegrant plasmid through Cre-mediated recombination across a lox site.In the second scenario,a cDNA is flanked by phage lambda att sites which direct recombination into an expression vector through the use of the INT/XIS/IHF proteins.In these ways,a single donor clone can easily be transferred into any number of recipient vectors. the most common fusion is the histidine tag for purifica-analysis studies into small-scale for analysis of expres-tion on metal-chelate resins.This tag provides a sub-sion levels and properties and into large-scale for use stantial purification handle while being relatively un-with many of the proteomic applications.obtrusive as a fusion partner.Beyond purification,Small-scale expression is most useful for identifying translational fusions often provide a means to enhance those clones which express recombinant protein to high expression.The larger fusion tags such as thioredoxin levels and for evaluating the folding state of the protein. and GST often are superior in this respect.Crude expression testing is typically done by simpleSDS–PAGE analysis of whole cells.Evaluation of thefolding state is typically done by centrifugal fraction-RECOMBINANT EXPRESSION OF PROTEINation of a lysate,requiring a more gentle lysis proce-dure.Several lysozyme and mild detergent methods are Bacterial expression is most common for recombinantcommercially available for this purpose.proteins because of its ease of use and the high levelsof protein obtained.It is useful to divide expression For large-scale production for many applications,TABLE2Common Purification TagsBasis of purification Elution Reference Small tagsHistidine tag Metal affinity resin Imidizole(5)S-tag Interaction with S-protein Temperature(6) Calmodulin-binding protein Interaction with Calmodulin Calcium(7) Large tagsGlutathione S-transferase Glutathione agarose Reduced glutathione(8) Thioredoxin Phenylarsine oxide resin-Mercaptoethanol(9) Biotinylation domain Monomeric avidin resin Biotin(10) Maltose binding protein Amylose resin Maltose(11) Chitin binding protein/intein Chitin affinity Thiol(12)162SCOTT A.LESLEYtens of milligrams of protein typically are required.PURIFICATION STRATEGYEven with common bacterial expression levels,500–Proteins are highly diverse in their properties mak-1000ml of culture typically is required to provide these ing generic methods of purification difficult.Purifica-amounts.While such methods are commonplace in labo-tion tags such as described in Table2are a typical ratories,systems for parallel processing large numbers solution for purifying proteins in parallel.His-tag fu-of cultures at this level are not commercially available.sions are very common and provide a single-step chro-By developing instrumentation and optimizing media matographic purification that yields protein of suffi-and aeration conditions for high-density cell growth,cient purity for most applications.In addition,the his-our laboratory can parallel process96cultures at this tag sequence requires the addition of only six amino scale.Optical density(OD)values at600nm can reach acids to the recombinant protein,reducing the likeli-a value of40with logarithmic growth through at least hood that such a fusion will adversely affect gene func-30OD units.These cell densities allow us to producetion.A typical purification strategy is outlined in Fig.2. sufficient cell mass with65-ml culture volumes to yieldtens of milligrams of recombinant protein,sufficient for PURIFICATION AUTOMATIONmost applications.Such instrumentation is not com-Parallel processing typically involves instrumenta-monly available,but common shaking incubators cantion for automation.Lysis methods such as sonication or substitute with larger volumes.using a French press are not simple automation tasks. Recombinant expression of proteins is achievedLikewise,centrifugation is not easily integrated into through induction of a strong promoter system.Manyautomation due to problems of locating and indexing options exist in this regard including tac,T7,lambdathe rotor position.Automation of this process typically P L,and ara B promoters.It is important for parallelinvolves protocol modifications.This can easily be processing that growth and induction characteristicsachieved in small-scale methods.are consistent.For this reason,it is important to retainOn small-scale,parallel processing usually involves tight repression of expression and have a simple induc-use of a96-well plate format.Lysis is typically achieved tion procedure for high-level expression.For T7sys-using a combination of lysozyme and freeze–thaw cy-tems,the lac operator and T7lysozyme(pLysS)provide cles.Phage lysozymes are more effective than hen egg an extra level of repression.The arabinose promoter is white lysozyme for this purpose and can be combined tightly repressed in the absence of inducer and is our with nucleases to reduce viscosity and facilitate re-preferred system for parallel growth.With all of the moval of cell debris at the low g forces commonly used promoters listed,recombinant expression levels of10–with microtiter plates.Alternatively,nonionic deter-50%of total cell protein are common.gents can be employed for nondenaturing lysis.FIG.2.Generalized purification strategy of recombinant fusion protein.A common purification strategy is shown here.Proteins are purified from fermentation cultures by affinity purification.Isolated cell pellets are resuspended in an appropriate lysis buffer and disrupted by high-intensity sonication.Cell walls and insoluble debris are pelleted by centrifugation and the soluble supernatant containing the recombinant fusion protein is applied to chromatography resin containing an immobilized metal for affinity purification.Fractions containing the recombinant protein can be used directly or further purified using conventional chromatographic techniques.HIGH-THROUGHPUT PROTEOMICS163TABLE3Robotic Systems with Capabilities Adaptable to ProteinPurificationManu-facturer Instrument WebsiteQiagen BioRobot3000/Tomtec Quadra96/Matrix PlateMate /Hamilton Microlab4200/Beckman Biomek2000/Packard PlateTrak /index2.htmGilson Nebula215/index.htmlRobotic systems for nucleic acid purification are rela-tively commonplace and have recently been adapted forprotein purification.The Qiagen BioRobot3000per-forms multiple functions relevant to protein purifica-tion.It provides aspirate,dispense,pipet,vacuum fil-tration,and plate-shaking functions on a relativelycompact platform.These functions can be adapted to FIG.4.SDS–PAGE analysis of purified protein.Metal affinity chro-perform cell lysis and chromatography steps from1–2matography yields highly purified protein from a single chromato-graphic separation.This gel shows typical yields and purity obtained ml of bacterial culture.Specialized96-well plates clearfrom parallel purification using an automated purification system. cell debris via vacuum filtration and are also used toSuch proteins have been incorporated directly in successful crystalli-retain resin for chromatographic separations.The Wal-zation trials.Ten-microliter samples of12ml protein eluates from Ni-lac Quadra96also has most of these capacities and resin were separated by10%SDS–PAGE.Samples are recombinant can parallel process96or384samples.Both of thesefusions of thioredoxin to human proteins as indicated by accessionnumber.systems have been used with success in our laboratoryfor small-scale protein purification of proteins in micro-titer plates.Table3lists some robotic systems that maybe applied to small-scale protein purification.providing the throughput needed for proteomic studies Despite the difficulties,large-scale protein purifica-involving tens of thousands of proteins.We are cur-tion also can be automated.In our laboratory we simul-rently able to process approximately96–192proteins taneously process96bacterial cultures of65–70ml.per day with this system with average yields of around Instrumentation for processing96parallel cultures at10mg of purified protein.Affinity purification results that scale required development of custom roboticsin recombinant protein that is typically80–90%pure shown in Fig.3.These robotics incorporate liquid aspi-(see Fig.4)which is sufficient for most applications. rate and dispense,centrifugation,and sonication capa-Subsequent purification is sometimes necessary,for ex-bilities required for purification.Automation is key to ample,in protein crystallography,and is achieved usingFIG.3.Protein purification automation.Custom robotics for performing the purification strategy outlined in Fig.2are shown.(a)The instrument has capacity for automated liquid aspiration and dispensing,sonication,centrifugation,and fractionation.Ninety-six cultures are processed in parallel,giving up to10–50mg of purified protein per culture.(b)Expanded view of aspirate/dispense/sonicate head accessing rotor.164SCOTT A.LESLEYREFERENCESstandard ion-exchange and size-exclusion chromatog-raphy.Automation of these methods is relatively1.Venter,J.C.et al.(2001)The sequence of the human genome. straightforward employing standard FPLC and au-Science291,1304–1351.tosampler instrumentation.2.International Human Genome Sequencing Consortium(2001)Initial sequencing and analysis of the human genome.Nature SUMMARY409,860–921.3.Liu,Q.,Li,M.Z.,Leibham,D.,Cortez,D.,and Elledge,S.J. Determining gene function and understanding the(1999)The univector plasmid-fusion system,a method for rapid relationships and interactions of the gene products are construction of recombinant DNA without restriction enzymes.a global effort in biological studies.The approach toCurr.Biol.8,1300–1309.performing this immense task is driven by the availabil- 4.Hatley,J.L.,Temple,G.F.,and Brasch,M.A.(2000)DNA cloningity of genomic information.To utilize this informationusing in vivo site-specific recombination.Genome Res.10,pp.1788–1795.for experimentation,however,significant effort isneeded to actually isolate and express proteins from5.Petty,K.J.(1996)Metal-chelate affinity chromatography,in“Current Protocols in Molecular Biology,”Vol.2,Wiley,New York. the genes of interest for study.The complexity of this6.Kim,J.S.,and Raines,R.T.(1993)Ribonuclease S-peptide as a effort is compounded by the large number of gene prod-carrier in fusion proteins.Protein Sci.2,348–356.ucts comprising the proteome.Parallel processing and7.Stofko-Hahn,R.E.,.Carr,D.W,and Scott,J.D.(1992)A single generic methods are required to achieve a systematicstep purification for recombinant proteins.FEBS Lett.302, and thorough evaluation of gene function.274–278.Experimental uses of proteins for structural and8.Smith,D.B.,and Johnson,K.S.(1988)Single-step purification functional studies typically require milligram amounts of polypeptides expressed in Escherichia coli as fusions within purified form.Unlike genomic technologies that pri-glutathione S-transferase.Gene67,31–40.marily involve the study of nucleic acids,proteomic9.Lu,Z.,DiBlasio-Smith,E.A.,Grant,K.L.,Warne,N.W.,LaVallie,studies focus on proteins.Proteins are by nature much E.R.,Collins-Racie,L.A.,Follettie,M.T.,Williamson,M.J.,more diverse in composition and properties than nucleicand McCoy,J.M.(1996)Histidine patch thioredoxins.Mutantforms of thioredoxin with metal chelating affinity that provide acids.In many ways,this makes them more interestingfor convenient purifications of thioredoxin fusion proteins.J. but also less amenable to generic methods and technolo-Biol.Chem.271,5059–5065.gies for parallel processing.Nonetheless,methods and10.Cronan,J.E.(1990)Biotination of proteins in vivo.A post-trans-instrumentation are currently available to meet this lational modification to label,purify,and study proteins.J.Biol.ing these advances will allow a systematic Chem.265,10327–33.effort at understanding biological pathways at the11.Maina,C.V.,Riggs,P.D.,Grandea,A.G.,Slatko,B.E.,Moran,molecular level.L.S.,Tagliamonte,J.A.,McReynolds,L.A.,and Guan,C.D.(1988)An Escherichia coli vector to express and purify foreign ACKNOWLEDGMENTS proteins by fusion to and separation from maltose-binding pro-tein.Gene74,365–373.The author acknowledges the help of Marc Nasoff,Heath Klock,Dan McMullan,Tanya Shin,Juli Vincent,Mike Hornsby,Mark12.Chong S.,Mersha F.B.,Comb D.G.,Scott M.E.,Landry D.,Vence L.M.,Perler F.B.,Benner J.,Kucera R.B.,Hirvonen C. Knuth,Loren Miraglia,and Jeremiah Gilmore for their contributionsto the high-throughput cloning and expression efforts.He also recog- A.,Pelletier J.J.,Paulus H.,and Xu M.Q.(1997)Single-column nizes Bob Downs,Mark Weselak,Andy Meyer,and Jim Mainquistpurification of free recombinant proteins using a self-cleavable and the rest of the GNF engineering staff for their contributions to affinity tag derived from a protein splicing element.Gene192, the custom robotics that make this effort possible.271–281.。
微生物资源及开发利用

nature
Vol 467|2 September 10| doi:10.1038 /nature 09354
Bacterial charity work leads to population-wide resistance Henry H. Lee, Michael N. Molla, Charles R. Cantor & James J. Collins
to understand microbial communities. …individual host properties
such未a来s b医od师y m或a可ss依in据de肠x, a型ge的, o区r g划en,de量r 身can打n造ot 病exp患la饮in 食the清o单bserved ente以rot及yp开es处…方,甚至至为抗生素寻求替代品。
Enterotypes of the human gut microbiome
…identify three robust clusters (referred to as enterotypes hereafter)
that人ar类e n肠ot道na微tio生n 物or 系co统nti可ne拥nt 有spe特ci定fic的…分Th类is —ind肠ic型ates further the
othf比a1n2人4th类Eeu基hruo因mpe组aann大gien1nd5ei0vc倍iodmu。apllse…m.eTnth,e…geOnveesre9t,9~%15o0f
times larger the genes are
ba超ct过er9ia9l%, in为d细ica菌tin。g that the entire cohort harbours between
羟基磷灰石_mrna纯化_解释说明以及概述
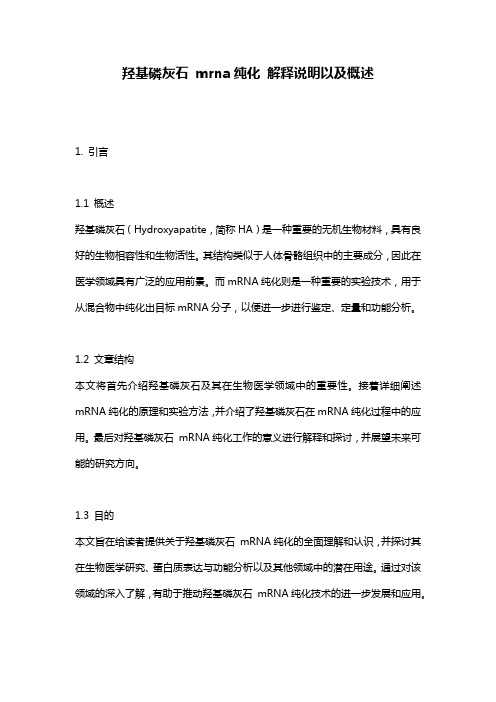
羟基磷灰石mrna纯化解释说明以及概述1. 引言1.1 概述羟基磷灰石(Hydroxyapatite,简称HA)是一种重要的无机生物材料,具有良好的生物相容性和生物活性。
其结构类似于人体骨骼组织中的主要成分,因此在医学领域具有广泛的应用前景。
而mRNA纯化则是一种重要的实验技术,用于从混合物中纯化出目标mRNA分子,以便进一步进行鉴定、定量和功能分析。
1.2 文章结构本文将首先介绍羟基磷灰石及其在生物医学领域中的重要性。
接着详细阐述mRNA纯化的原理和实验方法,并介绍了羟基磷灰石在mRNA纯化过程中的应用。
最后对羟基磷灰石mRNA纯化工作的意义进行解释和探讨,并展望未来可能的研究方向。
1.3 目的本文旨在给读者提供关于羟基磷灰石mRNA纯化的全面理解和认识,并探讨其在生物医学研究、蛋白质表达与功能分析以及其他领域中的潜在用途。
通过对该领域的深入了解,有助于推动羟基磷灰石mRNA纯化技术的进一步发展和应用。
2. 羟基磷灰石mRNA纯化2.1 羟基磷灰石介绍羟基磷灰石(Hydroxyapatite,HA)是一种常见的生物无机材料,具有良好的生物相容性和生物活性。
它主要由钙离子、磷酸盐以及羟基离子组成,并具有类似骨骼与牙釉质的化学成分和晶体结构。
由于其特殊的理化性质,羟基磷灰石被广泛应用于医药领域。
2.2 mRNA纯化原理mRNA(messenger RNA)是DNA转录过程中的一个重要产物,承担了将DNA 上的遗传信息转移到蛋白质合成过程中的功能。
在细胞内,mRNA通常与许多其他RNA种类混合存在,并且其含量非常低。
为了从细胞中纯化出高纯度的mRNA样品用于进一步实验或分析,需要使用合适的方法进行纯化。
羟基磷灰石作为一种亲和层析材料可以选择性地结合并保留带有多聚A核苷酸序列(polyA tail)的mRNA。
这是因为多数真核细胞的mRNA 3'末端都存在连续六个以上的A核苷酸,而DNA中则不含有或只含有很少的这种序列。
镍柱纯化蛋白说明书

Instruction ManualProBond TM Purification SystemFor purification of polyhistidine-containing recombinant proteinsCatalog nos. K850-01, K851-01, K852-01, K853-01, K854-01,R801-01, R801-15Version K2 September200425-0006iiTable of ContentsKit Contents and Storage (iv)Accessory Products (vi)Introduction (1)Overview (1)Methods (2)Preparing Cell Lysates (2)Purification Procedure—Native Conditions (7)Purification Procedure—Denaturing Conditions (11)Purification Procedure—Hybrid Conditions (13)Troubleshooting (15)Appendix (17)Additional Protocols (17)Recipes (18)Frequently Asked Questions (21)References (22)Technical Service (23)iiiKit Contents and StorageTypes of Products This manual is supplied with the following products:Product CatalogNo.ProBond™ Purification System K850-01ProBond™ Purification System with Antibodywith Anti-Xpress™ Antibody K851-01with Anti-myc-HRP Antibody K852-01with Anti-His(C-term)-HRP Antibody K853-01with Anti-V5-HRP Antibody K854-01ProBond™ Nickel-Chelating Resin (50 ml) R801-01ProBond™ Nickel Chelating Resin (150 ml) R801-15ProBond™Purification System Components The ProBond™ Purification System includes enough resin, reagents, and columns for six purifications. The components are listed below. See next page for resin specifications.Component Composition Quantity ProBond™ Resin 50% slurry in 20% ethanol 12 ml5X NativePurification Buffer250 mM NaH2PO4, pH 8.02.5 M NaCl1 × 125 ml bottleGuanidinium LysisBuffer6 M Guanidine HCl20 mM sodium phosphate, pH 7.8500 mM NaCl1 × 60 ml bottleDenaturingBinding Buffer8 M Urea20 mM sodium phosphate, pH 7.8500 mM NaCl2 × 125 ml bottlesDenaturing WashBuffer8 M Urea20 mM sodium phosphate, pH 6.0500 mM NaCl2 × 125 ml bottlesDenaturing ElutionBuffer8 M Urea20 mM NaH2PO4, pH 4.0500 mM NaCl1 × 60 ml bottleImidazole 3 M Imidazole,20 mM sodium phosphate, pH 6.0500 mM NaCl1 × 8 ml bottlePurificationColumns10 ml columns 6Continued on next pageivKit Contents and Storage, ContinuedProBond™Purification System with Antibody The ProBond™ Purification System with Antibody includes resin, reagents, and columns as described for the ProBond™ Purification System (previous page) and 50 µl of the appropriate purified mouse monoclonal antibody. Sufficient reagents are included to perform six purifications and 25 Western blots with the antibody.For more details on the antibody specificity, subclass, and protocols for using the antibody, refer to the antibody manual supplied with the system.Storage Store ProBond™ resin at +4°C. Store buffer and columns at room temperature.Store the antibody at 4°C. Avoid repeated freezing and thawing of theantibody as it may result in loss of activity.The product is guaranteed for 6 months when stored properly.All native purification buffers are prepared from the 5X Native PurificationBuffer and the 3 M Imidazole, as described on page 7.The Denaturing Wash Buffer pH 5.3 is prepared from the Denaturing WashBuffer (pH 6.0), as described on page 11.Resin and ColumnSpecificationsProBond™ resin is precharged with Ni2+ ions and appears blue in color. It isprovided as a 50% slurry in 20% ethanol.ProBond™ resin and purification columns have the following specifications:• Binding capacity of ProBond™ resin: 1–5 mg of protein per ml of resin• Average bead size: 45–165 microns• Pore size of purification columns: 30–35 microns• Recommended flow rate: 0.5 ml/min• Maximum flow rate: 2 ml/min• Maximum linear flow rate: 700 cm/h• Column material: Polypropylene• pH stability (long term): pH 3–13• pH stability (short term): pH 2–14ProductQualificationThe ProBond™ Purification System is qualified by purifying 2 mg of myoglobinprotein on a column and performing a Bradford assay. Protein recovery mustbe 75% or higher.vAccessory ProductsAdditionalProductsThe following products are also available for order from Invitrogen:Product QuantityCatalogNo.ProBond™ Nickel-Chelating Resin 50 ml150 mlR801-01R801-15Polypropylene columns(empty)50 R640-50Ni-NTA Agarose 10 ml25 ml R901-01 R901-15Ni-NTA Purification System 6 purifications K950-01 Ni-NTA Purification Systemwith Antibodywith Anti-Xpress™ Antibody with Anti-myc-HRP Antibody with Anti-His(C-term)-HRP Antibodywith Anti-V5-HRP Antibody 1 kit1 kit1 kit1 kitK951-01K952-01K953-01K954-01Anti-myc Antibody 50 µl R950-25 Anti-V5 Antibody 50 µl R960-25 Anti-Xpress™ Antibody 50 µl R910-25 Anti-His(C-term) Antibody 50 µl R930-25 InVision™ His-tag In-gel Stain 500 ml LC6030 InVision™ His-tag In-gelStaining Kit1 kit LC6033Pre-Cast Gels and Pre-made Buffers A large variety of pre-cast gels for SDS-PAGE and pre-made buffers for your convenience are available from Invitrogen. For details, visit our web site at or contact Technical Service (page 23).viIntroductionOverviewIntroduction The ProBond™ Purification System is designed for purification of 6xHis-tagged recombinant proteins expressed in bacteria, insect, and mammalian cells. Thesystem is designed around the high affinity and selectivity of ProBond™Nickel-Chelating Resin for recombinant fusion proteins containing six tandemhistidine residues.The ProBond™ Purification System is a complete system that includespurification buffers and resin for purifying proteins under native, denaturing,or hybrid conditions. The resulting proteins are ready for use in many targetapplications.This manual is designed to provide generic protocols that can be adapted foryour particular proteins. The optimal purification parameters will vary witheach protein being purified.ProBond™ Nickel-Chelating Resin ProBond™ Nickel-Chelating Resin is used for purification of recombinant proteins expressed in bacteria, insect, and mammalian cells from any 6xHis-tagged vector. ProBond™ Nickel-Chelating Resin exhibits high affinity and selectivity for 6xHis-tagged recombinant fusion proteins.Proteins can be purified under native, denaturing, or hybrid conditions using the ProBond™ Nickel-Chelating Resin. Proteins bound to the resin are eluted with low pH buffer or by competition with imidazole or histidine. The resulting proteins are ready for use in target applications.Binding Characteristics ProBond™ Nickel-Chelating Resin uses the chelating ligand iminodiacetic acid (IDA) in a highly cross-linked agarose matrix. IDA binds Ni2+ ions by three coordination sites.The protocols provided in this manual are generic, and may not result in 100%pure protein. These protocols should be optimized based on the bindingcharacteristics of your particular proteins.Native VersusDenaturingConditionsThe decision to purify your 6xHis-tagged fusion proteins under native ordenaturing conditions depends on the solubility of the protein and the need toretain biological activity for downstream applications.• Use native conditions if your protein is soluble (in the supernatant afterlysis) and you want to preserve protein activity.• Use denaturing conditions if the protein is insoluble (in the pellet afterlysis) or if your downstream application does not depend on proteinactivity.• Use hybrid protocol if your protein is insoluble but you want to preserveprotein activity. Using this protocol, you prepare the lysate and columnsunder denaturing conditions and then use native buffers during the washand elution steps to refold the protein. Note that this protocol may notrestore activity for all proteins. See page 14.1MethodsPreparing Cell LysatesIntroduction Instructions for preparing lysates from bacteria, insect, and mammalian cellsusing native or denaturing conditions are described below.Materials Needed You will need the following items:• Native Binding Buffer (recipe is on page 8) for preparing lysates undernative conditions• Sonicator• 10 µg/ml RNase and 5 µg/ml DNase I (optional)• Guanidinium Lysis Buffer (supplied with the system) for preparing lysatesunder denaturing conditions• 18-gauge needle• Centrifuge• Sterile, distilled water• SDS-PAGE sample buffer• Lysozyme for preparing bacterial cell lysates• Bestatin or Leupeptin, for preparing mammalian cell lysatesProcessing Higher Amount of Starting Material Instructions for preparing lysates from specific amount of starting material (bacteria, insect, and mammalian cells) and purification with 2 ml resin under native or denaturing conditions are described in this manual.If you wish to purify your protein of interest from higher amounts of starting material, you may need to optimize the lysis protocol and purification conditions (amount of resin used for binding). The optimization depends on the expected yield of your protein and amount of resin to use for purification. Perform a pilot experiment to optimize the purification conditions and then based on the pilot experiment results, scale-up accordingly.Continued on next page2Preparing Bacterial Cell Lysate—Native Conditions Follow the procedure below to prepare bacterial cell lysate under native conditions. Scale up or down as necessary.1. Harvest cells from a 50 ml culture by centrifugation (e.g., 5000 rpm for5 minutes in a Sorvall SS-34 rotor). Resuspend the cells in 8 ml NativeBinding Buffer (recipe on page 8).2. Add 8 mg lysozyme and incubate on ice for 30 minutes.3. Using a sonicator equipped with a microtip, sonicate the solution on iceusing six 10-second bursts at high intensity with a 10-second coolingperiod between each burst.Alternatively, sonicate the solution on ice using two or three 10-secondbursts at medium intensity, then flash freeze the lysate in liquid nitrogen or a methanol dry ice slurry. Quickly thaw the lysate at 37°C andperform two more rapid sonicate-freeze-thaw cycles.4. Optional: If the lysate is very viscous, add RNase A (10 µg/ml) andDNase I (5 µg/ml) and incubate on ice for 10–15 minutes. Alternatively,draw the lysate through a 18-gauge syringe needle several times.5. Centrifuge the lysate at 3,000 ×g for 15 minutes to pellet the cellulardebris. Transfer the supernatant to a fresh tube.Note: Some 6xHis-tagged protein may remain insoluble in the pellet, and can be recovered by preparing a denatured lysate (page 4) followed bythe denaturing purification protocol (page 12). To recover this insolubleprotein while preserving its biological activity, you can prepare thedenatured lysate and then follow the hybrid protocol on page 14. Notethat the hybrid protocol may not restore activity in all cases, and should be tested with your particular protein.6. Remove 5 µl of the lysate for SDS-PAGE analysis. Store the remaininglysate on ice or freeze at -20°C. When ready to use, proceed to theprotocol on page 7.Continued on next page3Preparing Bacterial Cell Lysate—Denaturing Conditions Follow the procedure below to prepare bacterial cell lysate under denaturing conditions:1. Equilibrate the Guanidinium Lysis Buffer, pH 7.8 (supplied with thesystem or see page 19 for recipe) to 37°C.2. Harvest cells from a 50 ml culture by centrifugation (e.g., 5000 rpm for5 minutes in a Sorvall SS-34 rotor).3. Resuspend the cell pellet in 8 ml Guanidinium Lysis Buffer from Step 1.4. Slowly rock the cells for 5–10 minutes at room temperature to ensurethorough cell lysis.5. Sonicate the cell lysate on ice with three 5-second pulses at high intensity.6. Centrifuge the lysate at 3,000 ×g for 15 minutes to pellet the cellulardebris.Transfer the supernatant to a fresh tube.7. Remove 5 µl of the lysate for SDS-PAGE analysis. Store the remaininglysate on ice or at -20°C. When ready to use, proceed to the denaturingprotocol on page 11 or hybrid protocol on page 13.Note: To perform SDS-PAGE with samples in Guanidinium Lysis Buffer, you need to dilute the samples, dialyze the samples, or perform TCAprecipitation prior to SDS-PAGE to prevent the precipitation of SDS.Harvesting Insect Cells For detailed protocols dealing with insect cell expression, consult the manual for your particular system. The following lysate protocols are for baculovirus-infected cells and are intended to be highly generic. They should be optimized for your cell lines.For baculovirus-infected insect cells, when the time point of maximal expression has been determined, large scale protein expression can be carried out. Generally, the large-scale expression is performed in 1 liter flasks seeded with cells at a density of 2 × 106 cells/ml in a total volume of 500 ml and infected with high titer viral stock at an MOI of 10 pfu/cell. At the point of maximal expression, harvest cells in 50 ml aliquots. Pellet the cells by centrifugation and store at -70°C until needed. Proceed to preparing cell lysates using native or denaturing conditions as described on the next page.Continued on next page4Preparing Insect Cell Lysate—Native Condition 1. Prepare 8 ml Native Binding Buffer (recipe on page 8) containingLeupeptin (a protease inhibitor) at a concentration of 0.5 µg/ml.2. After harvesting the cells (previous page), resuspend the cell pellet in8 ml Native Binding Buffer containing 0.5 µg/ml Leupeptin.3. Lyse the cells by two freeze-thaw cycles using a liquid nitrogen or dryice/ethanol bath and a 42°C water bath.4. Shear DNA by passing the preparation through an 18-gauge needle fourtimes.5. Centrifuge the lysate at 3,000 ×g for 15 minutes to pellet the cellulardebris.Transfer the supernatant to a fresh tube.6. Remove 5 µl of the lysate for SDS-PAGE analysis. Store remaining lysateon ice or freeze at -20°C. When ready to use, proceed to the protocol on page 7.Preparing Insect Cell Lysate—Denaturing Condition 1. After harvesting insect cells (previous page), resuspend the cell pellet in8 ml Guanidinium Lysis Buffer (supplied with the system or see page 19for recipe).2. Pass the preparation through an 18-gauge needle four times.3. Centrifuge the lysate at 3,000 ×g for 15 minutes to pellet the cellulardebris. Transfer the supernatant to a fresh tube.4. Remove 5 µl of the lysate for SDS-PAGE analysis. Store remaining lysateon ice or freeze at -20° C. When ready to use, proceed to the denaturingprotocol on page 11 or hybrid protocol on page 13.Note: To perform SDS-PAGE with samples in Guanidinium Lysis Buffer, you need to dilute the samples, dialyze the samples, or perform TCAprecipitation prior to SDS-PAGE to prevent the precipitation of SDS.Continued on next pagePreparing Mammalian Cell Lysate—Native Conditions For detailed protocols dealing with mammalian expression, consult the manual for your particular system. The following protocols are intended to be highly generic, and should be optimized for your cell lines.To produce recombinant protein, you need between 5 x 106and 1 x 107 cells. Seed cells and grow in the appropriate medium until they are 80–90% confluent. Harvest cells by trypsinization. You can freeze the cell pellet in liquid nitrogen and store at -70°C until use.1. Resuspend the cell pellet in 8 ml of Native Binding Buffer (page 8). Theaddition of protease inhibitors such as bestatin and leupeptin may benecessary depending on the cell line and expressed protein.2. Lyse the cells by two freeze-thaw cycles using a liquid nitrogen or dryice/ethanol bath and a 42°C water bath.3. Shear the DNA by passing the preparation through an 18-gauge needlefour times.4. Centrifuge the lysate at 3,000 ×g for 15 minutes to pellet the cellulardebris. Transfer the supernatant to a fresh tube.5. Remove 5 µl of the lysate for SDS-PAGE analysis. Store the remaininglysate on ice or freeze at -20° C. When ready to use, proceed to theprotocol on page 7.Preparing Mammalian Cell Lysates—Denaturing Conditions For detailed protocols dealing with mammalian expression, consult the manual for your particular system. The following protocols are intended to be highly generic, and should be optimized for your cell lines.To produce recombinant protein, you need between 5 x 106and 1 x 107 cells. Seed cells and grow in the appropriate medium until they are 80–90% confluent. Harvest cells by trypsinization. You can freeze the cell pellet in liquid nitrogen and store at -70°C until use.1. Resuspend the cell pellet in 8 ml Guanidinium Lysis Buffer (suppliedwith the system or see page 19 for recipe).2. Shear the DNA by passing the preparation through an 18-gauge needlefour times.3. Centrifuge the lysate at 3,000 ×g for 15 minutes to pellet the cellulardebris. Transfer the supernatant to a fresh tube.4. Remove 5 µl of the lysate for SDS-PAGE analysis. Store the remaininglysate on ice or freeze at -20° C until use. When ready to use, proceed to the denaturing protocol on page 11 or hybrid protocol on page 13.Note: To perform SDS-PAGE with samples in Guanidinium Lysis Buffer, you need to dilute the samples, dialyze the samples, or perform TCAprecipitation prior to SDS-PAGE to prevent the precipitation of SDS.Purification Procedure—Native ConditionsIntroduction In the following procedure, use the prepared Native Binding Buffer, NativeWash Buffer, and Native Elution Buffer, columns, and cell lysate preparedunder native conditions. Be sure to check the pH of your buffers before starting.Buffers for Native Purification All buffers for purification under native conditions are prepared from the5X Native Purification Buffer supplied with the system. Dilute and adjust the pH of the 5X Native Purification Buffer to create 1X Native Purification Buffer (page 8). From this, you can create the following buffers:• Native Binding Buffer• Native Wash Buffer• Native Elution BufferThe recipes described in this section will create sufficient buffers to perform one native purification using one kit-supplied purification column. Scale up accordingly.If you are preparing your own buffers, see page 18 for recipe.Materials Needed You will need the following items:• 5X Native Purification Buffer (supplied with the system or see page 18 forrecipe)• 3 M Imidazole (supplied with the system or see page 18 for recipe)• NaOH• HCl• Sterile distilled water• Prepared ProBond™ columns with native buffers (next page)• Lysate prepared under native conditions (page 2)Imidazole Concentration in Native Buffers Imidazole is included in the Native Wash and Elution Buffers to minimize the binding of untagged, contaminating proteins and increase the purity of the target protein with fewer wash steps. Note that, if your level of contaminating proteins is high, you may add imidazole to the Native Binding Buffer.If your protein does not bind well under these conditions, you can experiment with lowering or eliminating the imidazole in the buffers and increasing the number of wash and elution steps.Continued on next page1X Native Purification Buffer To prepare 100 ml 1X Native Purification Buffer, combine:• 80 ml of sterile distilled water• 20 ml of 5X Native Purification Buffer (supplied with the system or see page 18 for recipe)Mix well and adjust pH to 8.0 with NaOH or HCl.Native Binding Buffer Without ImidazoleUse 30 ml of the 1X Native Purification Buffer (see above for recipe) for use as the Native Binding Buffer (used for column preparation, cell lysis, and binding).With Imidazole (Optional):You can prepare the Native Binding Buffer with imidazole to reduce the binding of contaminating proteins. (Note that some His-tagged proteins may not bind under these conditions.).To prepare 30 ml Native Binding Buffer with 10 mM imidazole, combine: • 30 ml of 1X Native Purification Buffer• 100 µl of 3 M Imidazole, pH 6.0Mix well and adjust pH to 8.0 with NaOH or HCl.Native Wash Buffer To prepare 50 ml Native Wash Buffer with 20 mM imidazole, combine:• 50 ml of 1X Native Purification Buffer• 335 µl of 3 M Imidazole, pH 6.0Mix well and adjust pH to 8.0 with NaOH or HCl.Native Elution Buffer To prepare 15 ml Native Elution Buffer with 250 mM imidazole, combine:• 13.75 ml of 1X Native Purification Buffer• 1.25 ml of 3 M Imidazole, pH 6.0Mix well and adjust pH to 8.0 with NaOH or HCl.Continued on next pageDo not use strong reducing agents such as DTT with ProBond™ columns. DTTreduces the nickel ions in the resin. In addition, do not use strong chelatingagents such as EDTA or EGTA in the loading buffers or wash buffers, as thesewill strip the nickel from the columns.Be sure to check the pH of your buffers before starting.PreparingProBond™ ColumnWhen preparing a column as described below, make sure that the snap-off capat the bottom of the column remains intact. To prepare a column:1. Resuspend the ProBond™ resin in its bottle by inverting and gentlytapping the bottle repeatedly.2. Pipet or pour 2 ml of the resin into a 10-ml Purification Columnsupplied with the kit. Allow the resin to settle completely by gravity(5-10 minutes) or gently pellet it by low-speed centrifugation (1 minuteat 800 ×g). Gently aspirate the supernatant.3. Add 6 ml of sterile, distilled water and resuspend the resin byalternately inverting and gently tapping the column.4. Allow the resin to settle using gravity or centrifugation as described inStep 2, and gently aspirate the supernatant.5. For purification under Native Conditions, add 6 ml Native BindingBuffer (recipe on page 8).6. Resuspend the resin by alternately inverting and gently tapping thecolumn.7. Allow the resin to settle using gravity or centrifugation as described inStep 2, and gently aspirate the supernatant.8. Repeat Steps 5 through 7.Storing PreparedColumnsTo store a column containing resin, add 0.02% azide or 20% ethanol as apreservative and cap or parafilm the column. Store at room temperature.Continued on next pagePurification Under Native Conditions Using the native buffers, columns and cell lysate, follow the procedure below to purify proteins under native conditions:1. Add 8 ml of lysate prepared under native conditions to a preparedPurification Column (page 9).2. Bind for 30–60 minutes using gentle agitation to keep the resinsuspended in the lysate solution.3. Settle the resin by gravity or low speed centrifugation (800 ×g), andcarefully aspirate the supernatant. Save supernatant at 4°C forSDS-PAGE analysis.4. Wash with 8 ml Native Wash Buffer (page 8). Settle the resin by gravityor low speed centrifugation (800 ×g), and carefully aspirate thesupernatant. Save supernatant at 4°C for SDS-PAGE analysis.5. Repeat Step 4 three more times.6. Clamp the column in a vertical position and snap off the cap on thelower end. Elute the protein with 8–12 ml Native Elution Buffer (seepage 2). Collect 1 ml fractions and analyze with SDS-PAGE.Note: Store the eluted fractions at 4°C. If -20°C storage is required, addglycerol to the fractions. For long term storage, add protease inhibitors to the fractions.If you wish to reuse the resin to purify the same recombinant protein, wash the resin with 0.5 M NaOH for 30 minutes and equilibrate the resin in a suitable binding buffer. If you need to recharge the resin, see page 17.Purification Procedure—Denaturing ConditionsIntroduction Instructions to perform purification using denaturing conditions with prepareddenaturing buffers, columns, and cell lysate are described below.Materials Needed You will need the following items:• Denaturing Binding Buffer (supplied with the system or see page 19 forrecipe)• Denaturing Wash Buffer, pH 6.0 (supplied with the system or see page 19 forrecipe) and Denaturing Wash Buffer, pH 5.3 (see recipe below)• Denaturing Elution Buffer (supplied with the system or see page 20 forrecipe)• Prepared ProBond™ columns with Denaturing buffers (see below)• Lysate prepared under denaturing conditions (page 11)Preparing the Denaturing Wash Buffer pH 5.3 Using a 10 ml aliquot of the kit-supplied Denaturing Wash Buffer (pH 6.0), mix well, and adjust the pH to 5.3 using HCl. Use this for the Denaturing Wash Buffer pH 5.3 in Step 5 next page.Be sure to check the pH of your buffers before starting. Note that thedenaturing buffers containing urea will become more basic over time. PreparingProBond™ ColumnWhen preparing a column as described below, make sure that the snap-off capat the bottom of the column remains intact.If you are reusing the ProBond™ resin, see page 17 for recharging protocol.To prepare a column:1. Resuspend the ProBond™ resin in its bottle by inverting and gentlytapping the bottle repeatedly.2. Pipet or pour 2 ml of the resin into a 10-ml Purification Columnsupplied with the kit. Allow the resin to settle completely by gravity(5-10 minutes) or gently pellet it by low-speed centrifugation (1 minuteat 800 ×g). Gently aspirate the supernatant.3. Add 6 ml of sterile, distilled water and resuspend the resin byalternately inverting and gently tapping the column.4. Allow the resin to settle using gravity or centrifugation as described inStep 2, and gently aspirate the supernatant.5. For purification under Denaturing Conditions, add 6 ml of DenaturingBinding Buffer.6. Resuspend the resin by alternately inverting and gently tapping thecolumn.7. Allow the resin to settle using gravity or centrifugation as described inStep 2, and gently aspirate the supernatant. Repeat Steps 5 through 7.Continued on next pagePurification Procedure—Denaturing Conditions, ContinuedPurification Under Denaturing Conditions Using the denaturing buffers, columns, and cell lysate, follow the procedure below to purify proteins under denaturing conditions:1. Add 8 ml lysate prepared under denaturing conditions to a preparedPurification Column (page 11).2. Bind for 15–30 minutes at room temperature using gentle agitation (e.g.,using a rotating wheel) to keep the resin suspended in the lysatesolution. Settle the resin by gravity or low speed centrifugation (800 ×g), and carefully aspirate the supernatant.3. Wash the column with 4 ml Denaturing Binding Buffer supplied with thekit by resuspending the resin and rocking for two minutes. Settle theresin by gravity or low speed centrifugation (800 ×g), and carefullyaspirate the supernatant. Save supernatant at 4°C for SDS-PAGEanalysis. Repeat this step one more time.4. Wash the column with 4 ml Denaturing Wash Buffer, pH 6.0 supplied inthe kit by resuspending the resin and rocking for two minutes. Settle the resin by gravity or low speed centrifugation (800 ×g), and carefullyaspirate the supernatant. Save supernatant at 4°C for SDS-PAGEanalysis. Repeat this step one more time.5. Wash the column with 4 ml Denaturing Wash Buffer pH 5.3 (see recipeon previous page) by resuspending the resin and rocking for 2 minutes.Settle the resin by gravity or low speed centrifugation (800 ×g), andcarefully aspirate the supernatant. Save supernatant at 4°C for SDS-PAGE analysis. Repeat this step once more for a total of two washes with Denaturing Wash Buffer pH 5.3.6. Clamp the column in a vertical position and snap off the cap on thelower end. Elute the protein by adding 5 ml Denaturing Elution Buffersupplied with the kit. Collect 1 ml fractions and monitor the elution bytaking OD280readings of the fractions. Pool the fractions that contain the peak absorbance and dialyze against 10 mM Tris, pH 8.0, 0.1% Triton X-100 overnight at 4°C to remove the urea. Concentrate the dialyzedmaterial by any standard method (i.e., using 10,000 MW cut-off, low-protein binding centrifugal instruments or vacuum concentrationinstruments).If you wish to reuse the resin to purify the same recombinant protein, wash the resin with 0.5 M NaOH for 30 minutes and equilibrate the resin in a suitable binding buffer. If you need to recharge the resin, see page 17.。
人重组粒细胞集落刺激因子在大肠杆菌中的过表达说明书

IJMSVol 28, No.3, September 2003131Overexpression of Recombinant Human Granulocyte Colony-Stimulating Factor in E. coliAbstractBakground: Granulocyte colony-stimulating factor (G-CSF) is a cytokine that stimulates hematopoiesis and induces proliferation and differentiation of granulocyte progenitor cells as well as production of bone marrow neutrophilic granulocyte colonies. Nowadays, hu-man recombinant G-CSF(hr G-CSF) is used for the treatment of chemotherapy- and radiotherapy-induced neutropenia, and also in patients with bone marrow transplantation.Methods: A cDNA of human G-CSF (hG-CSF) was synthesized by PCR from recombinant cloning vector, with two altered nucleotides for increasing mRNA stability and overexpression, then inserted into a pET expression vector under the control of T7 promoter and cloned in E. coli strain BL21 (DE3).Results: After culture and induction of recombinant E. coli with IPTG, we achieved a high level expression of the hG-CSF, where it represented approximately 35% of the total protein as determined by SDS-PAGE and confirmed by western blotting with polyclonal and monoclonal hG-CSF antibodies.Conclusion: rhG-CSF was produced in a significantly high quantity with a yield of 35% of total protein as determined by SDS-PAGE. Since it is easily obtained by simple purification steps, it may be cost-effective, even on an industrial scale. Iran J Med Sci 2003; 28(3):131-134.Keywords • Granulocyte colony stimulating factor, recombinant • recombinant proteins • escherichia coli.Introductionranulocyte colony-stimulating factor (G-CSF) is a hemato-poietic growth factor which stimulates the proliferation and differentiation of neutrophil precursor cells as well as someof the functional properties of mature neutrophil granulocytes.1 It has been shown that G-CSF has a dramatic effect in the treatment of leukopenia, AIDS, MDS and bone marrow transplantation. It has also been reported that hrG-CSF plays an important role in modifying clinical infections secondary to chemotherapy .2 A single G-CSF gene per haploid genome exists on human chromosomeGOriginal ArticleArchive of SIDFallah M J, Akbari B, Saeedinia A.R, et al132 17 in region q21-q22. The gene consists of about 2500 nuclotides and is split by four introns.3 More than 80% of the G-CSF mRNA produced in human carcinoma cells including squamous carcinoma CHU-2 and bladder carcinoma 5637 cell lines en-code a protein of 204 amino acids (G-CSFb), while the remaining mRNA encode a protein of 207 amino acids (G-CSFa). These two different human G-CSF mRNAs are generated by alternative use of the 3' donor sequence of the intron 2 of the G-CSF gene. The N-terminal 30 amino. acids of G-CSFa and G-CSFb are the signal sequence for secretion of G-CSF. Mature G-CSFb, consists of 174 amino acids, has a molecular weight Of 18,671 and is at least 20 times more potent in colony stimulating activity than that consisting of 177 amino acids.1,4 Human G-CSF is O-glycosylated at Thr redisue (in the three amino acid deleted version) with a struc-ture of N —acetyl-neuraminic acid α(2-6)[galactose β(1-3)] N-acetylgalactosamine. The sugar moiety of the human G-CSF is not necessary for biological activity because human recombinant G-CSF pro-duced in E. coli is as active as the recombinant molecule produced in mouse cells.4,5 Several re-ports are available which point to the use of cell lines for synthesis of G-CSF cDNA.6, 7 Because the sequence bias of genes in nature and its correla-tion with tRNA are significantly different between procaryotes and eucaryotes, there is a limitation for the expression of human cDNA in E. coli system. Recently, however, the entire synthetic gene was used in order to increase the expression level of hrG-CSF.8 Also, the codon-anticodon interaction seems to be so sticky that it interferes with the translation of hG-CSF in E. coli, due to the abun-dance of GC rich codons in 5' end of hG-CSF cDNA.7 In this report, we used peripheral blood monocytes as RNA source for cDNA synthesis and its overexpression in E. coli , by altering the se-quence at the 5' end of the G-CSF-coding region and decreasing the G+C content without altering the predicted amino acids sequence.Materials and MethodsPlasmid, Bacterial strain and Reagents: pET23a was kindly provided by Biotechnology division of Pasteur Institute of Iran. E. coli Top10F' and BL21(DE3) were purchasted from Cinnagen (Iran). Restriction endonucleases, T4 DNA ligase and chemical reagent were purchased from Roche. Primers were synthesised by GENE SET OLIGUS (France).Fig 1: Structure of expression vector (pET) [pETG-CSF construct]Archive of SIDOverexpression of recombinant human granulocyte colony-stimulating factor in E. coli133DNA Recombinant Technology: Extraction of plas-mid, digestion, isolation, ligation, transformation, identification, PCR were performed as described elsewhere.9Construction of Expression Vector: For subcloning of cDNA without signal sequence, pBluescript II sk containing 650 bp fragment, previously con-structed, was used as template in PCR by the fol-lowing:CATATGACACCCCTAGGCCCTGCC as forward primer; and GAATTCATTAGGGCTGGGCAAGGT as reverse primer.PCR product was 540 bp hG-CSF cDNA without signal sequence. Then, 540 bp fragment inserted into pET23a expression vector under control of T7 promoter.Recombinant Human G-CSF Expression: Compe-tent E. coli BL21(DE3) cells were transformed with pET23a expression vector containing the hG-CSF cDNA. E. coli cells were grown in shaker flasks at 37ºc, in LB broth medium until the absorbance of 0.7 at 600 nm was reached. 10 μl IPTG (100 mM) was then added, to induce the production of hG-CSF. After 4 h, the cells were harvested by cen-trifugation at 3000 rpm for 5 min. SDS-PAGE was performed and for confirmation of rhG-CSF band in gel, western blotting with polyclonal and mono-clonal human G-CSF antibody were performed. In western blot, rabbit polyclonal antibody was used at1/1000 concentration and mouse monoclonal anti-body at 2.5 μg/ml.ResultsUsing PCR human G-CSF cDNA was obtained from previously constructed recombinant cloningvector of pBluescript SK-GCSF. 10The pBluescript containing 650 bp fragment was used as template in PCR with two primers into which Nde I and EcoR I sites were introduced . To increase mRNA stability and overexpression the forward primer was altered in two nucleotides. PCR product was 540 bp hG-CSF cDNA without signal sequence. The latter was inserted to pET23a expression vector under the control of T7 promoter (Fig 1). pETG-CSF recombinant vector transferred to E. coli BL21(DE3) strain and the transformant bacteria grown at 37˚C and induced by IPTG. The cell pellets were collected and lysed for SDS-PAGE (Fig 2). As shown in Figure 2. BL21(DE3) expressed a 18.6 KD molecular weight of G-CSF at a level of 35% of total cell protein as measured by densitometric scanning with photodoc and total lab software and Vilber lurmat Gel docu-mentation. The rhG-CSF was expressed as inclu-sion bodies. Western blotting with monoclonal and polyclonal hG-CSF antibodies confirmed the G-CSF band in gel (result not shown).DiscussionThe human G-CSF was formally first been applied to the leukopenia in US in 1991. At present, the hG-CSF is the most widely-used and clinically ef-fective haematopoietic growth factors. The ran-domized studies using rhG-CSF versus placebo after chemotherapy for cancers resulted in faster neutrophil recovery, less severe neutropenia, and infections reduced.2We constructed the procaryotic expression vec-tor pET23a containing human G-CSFb cDNA, and achieved high level expression of the hG-CSF in E. coli , which represented at least 35% of the total protein as determined by SDS-PAGE. The hG-CSF was expressed as inclusion bodies in E. coli . We used monocytes of peripheral Blood whereas Shu 2 and Nagata 3 used cell lines for RNA extrac-tion and cDNA synthesis . According to Delvin et al 7, a decrease in the G+C content of the 5' end of the coding region can increase the G-CSF expres-sion. Therefore, they altered 3-5 nucleotides in this region and reported 17% and 6.5% of the total pro-tein in the pL and trpP expression Systems whereas alteration of two nucleotides yielded 35% of the total protein of recombinant E. coli . Accord-ing to Kang et al.8, the limitation for the expression of human cDNA in E. coli system accounts for theFig 2: SDS-PAGE of E.coli BL-21 Recombinant strains. Lones, from left to right: 1. molecular weight marker (Kilodalton); 2. G-CSF (Filgrastim-Neupogen); 3. bacteria with plasmid 4 hours after induction; 4. bacteria with plasmid before induction; 5. bacteria without plasmid.Archive of SIDFallah M J, Akbari B, Saeedinia A.R, et al134 significant differences between sequence bias of genes in nature and their correlation with tRNA in procaryotes and eucaryotes. Therefore, they used the entire synthetic G-CSF genes and obtained 500-600 mg/lit rhG-CSF. We cultured recombinant E. coli in fermentor and produced 1.2 g/lit rhG-CSF. In conclusion, rhG-CSF was obtained in a signifi-cantly high quantity and the yield was 35% of total protein as determined by SDS-PAGE. Since it is easily obtained by simple purification steps, it may be cost- effective, even at an industrial scale.AcknowledgmentWe thank Vahid Sadeghi for the presentation of materials and regents and also Hossein Ali Sami for the editorial work.References1Nagata SH: Gene structure and function of granulocyte colony-stimulating factor. Bio es-says 1989; 10(4):113-7.2Shu zh, Qinong Y: Expression of cDNA for rhuG-CSF in E. coli and characterization of the Protein. Chin J Cancer Res 1998 ;(10):256-9. 3Nagata SH, Tsuchiya M, Asano S, et al: The chromosomal gene structure and two mRNAs for human granulocyte colony stimulating fac-tor. EMBO J 1986; 5(3):575-81.4Nagata SH, Fukunaga R: Granulocyte colony-stimulating factor and its receptor. Prog Growth Factor Res 1991; 3(2):131-41.5 Hoglund M: Glycosilated and non-glycosilatedrecombinant huG-CSF. What is the difference? Med Oncol 1998; 15:229-33.6 Souza LM, Boone TC, Gabrilove J, et al: Re-combinant human granulocyte colony stimulat-ing factor: effects on normal and leukemia myeloid cells. Science 1986; 232(4746):61-5.7 Devlin PE, Drummond RJ, Toy P, et al: Altera-tion of amino-terminal codons of huG-CSF in-creases expression levels and allows efficient processing by methionine aminopeptidase in E. coli. Gene 1988; 65(1):13-22.8 Kang SH, Park CI, Park JH, et al: high levelexpressionn and simple purification of recom-binant human granulocyte colony stimulating factor in E. coli. Biotechnol Lett 1995; 17(7): 687-92.9 Sambrook J, Russell DW: Molecular cloning: alaboratory manual. Cold Spring Harbor Labora-tory, 1989.10 Saeedinia A, Sadeghizadeh M, Maghsoudi N,et al: Construction and cloning of human granulocyte colony stimulating factor (hG-CSF) cDNA. Modarres J M S 2003; 5(1).Archive of SID。
重组融合蛋白MBP-BSH在大肠杆菌中的表达及其纯化、功能鉴定
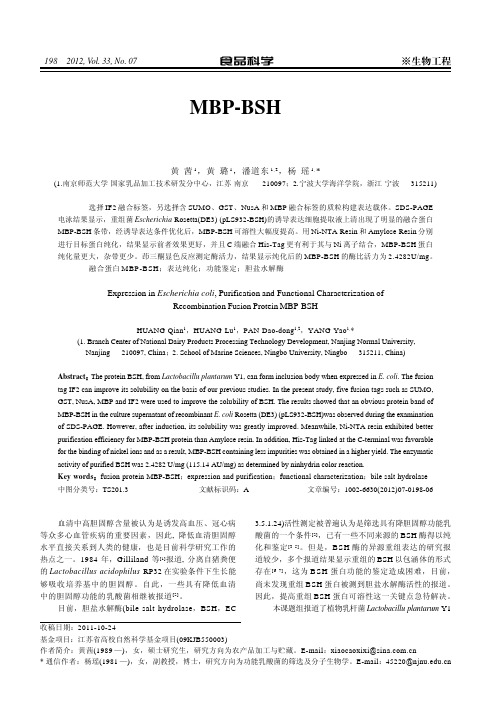
注:Apr. 具有氨苄青霉素抗性;Kanr. 具有卡那霉素抗性;m-MBP. 具有 MBP 双突变 A317V/I322V。
来源
实验室筛选保藏 实验室保藏 实验室保藏
日本 TaKaRa 公司 尚广东博士馈赠 尚广东博士馈赠 尚广东博士馈赠 尚广东博士馈赠 尚广东博士馈赠 尚广东博士馈赠 尚广东博士馈赠
purification efficiency for MBP-BSH protein than Amylose resin. In addition, His-Tag linked at the C-terminal was favorable
for the binding of nickel ions and as a result, MBP-BSH containing less impurities was obtained in a higher yield. The enzymatic
限制性内切酶 BamHⅠ和 XhoⅠ、250bp DNA Ladder Marker、λ/HindIII DNA Marker、pMD18-TTA 克 隆试剂盒、质粒提取试剂盒和 DNA 凝胶回收纯化试剂 盒 宝生物工程(大连)有限公司;Protein Marker Mid Range、氨苄青霉素、卡那霉素 上海生工生物工程技 术有限公司;酵母粉、胰蛋白胨 英国 Oxoid 公司; T4 DNA 连接酶 美国 New England Biolabs 公司;Amylose Resin、Ni-NTA Resin 德国 Novagen 公司。 1.3 bsh 基因的克隆及测序
大肠杆菌系统蛋白表达纯化流程
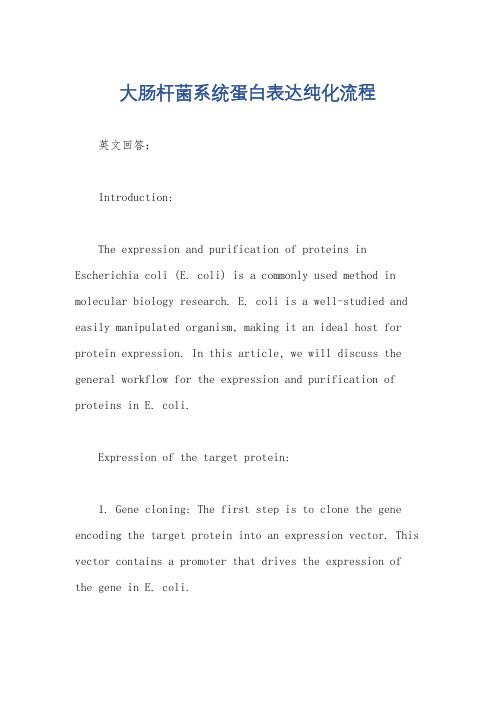
大肠杆菌系统蛋白表达纯化流程英文回答:Introduction:The expression and purification of proteins in Escherichia coli (E. coli) is a commonly used method in molecular biology research. E. coli is a well-studied and easily manipulated organism, making it an ideal host for protein expression. In this article, we will discuss the general workflow for the expression and purification of proteins in E. coli.Expression of the target protein:1. Gene cloning: The first step is to clone the gene encoding the target protein into an expression vector. This vector contains a promoter that drives the expression of the gene in E. coli.2. Transformation: The recombinant expression vector is then introduced into E. coli cells through a process called transformation. This results in the production of many E. coli cells carrying the target gene.3. Expression induction: The transformed E. coli cells are grown in a suitable culture medium until they reach a specific growth phase. At this point, expression of the target gene is induced by adding a chemical inducer or by changing the growth conditions.4. Protein expression: The induced E. coli cells produce the target protein, which can either be present in the soluble fraction or form insoluble aggregates called inclusion bodies.Protein purification:1. Cell lysis: The E. coli cells are harvested by centrifugation and then lysed to release the proteins. Various methods can be used for cell lysis, such as sonication, freeze-thaw cycles, or enzymatic digestion.2. Removal of cell debris: The cell lysate is then clarified by centrifugation to remove cell debris and insoluble material. The resulting supernatant contains the target protein along with other cellular components.3. Protein purification: Different purification techniques can be employed to isolate the target protein from the crude lysate. These techniques include affinity chromatography, ion exchange chromatography, size exclusion chromatography, and hydrophobic interaction chromatography. The choice of purification method depends on the properties of the target protein.4. Protein concentration: After purification, thetarget protein is often in a dilute solution. Concentration can be achieved by using techniques such as ultrafiltration or precipitation with ammonium sulfate.5. Protein characterization: The purified protein should be characterized to confirm its identity and purity. Techniques such as SDS-PAGE, western blotting, and massspectrometry can be used for protein analysis.Conclusion:The expression and purification of proteins in E. coli is a well-established and widely used technique in molecular biology research. The workflow involves gene cloning, protein expression, cell lysis, protein purification, concentration, and characterization. By following this general procedure, researchers can obtain purified proteins for further analysis and functional studies.中文回答:简介:大肠杆菌(E. coli)中的蛋白表达和纯化是分子生物学研究中常用的方法。
人补体C3功能片段rC3B的克隆表达和活性鉴定
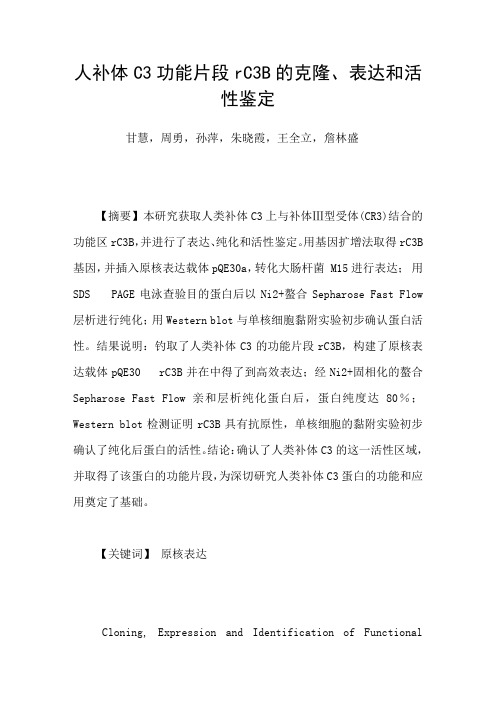
人补体C3功能片段rC3B的克隆、表达和活性鉴定甘慧,周勇,孙萍,朱晓霞,王全立,詹林盛【摘要】本研究获取人类补体C3上与补体Ⅲ型受体(CR3)结合的功能区rC3B,并进行了表达、纯化和活性鉴定。
用基因扩增法取得rC3B 基因,并插入原核表达载体pQE30a,转化大肠杆菌 M15进行表达;用SDS PAGE电泳查验目的蛋白后以Ni2+螯合Sepharose Fast Flow 层析进行纯化;用Western blot与单核细胞黏附实验初步确认蛋白活性。
结果说明:钓取了人类补体C3的功能片段rC3B,构建了原核表达载体pQE30rC3B并在中得了到高效表达;经Ni2+固相化的螯合Sepharose Fast Flow亲和层析纯化蛋白后,蛋白纯度达80%;Western blot检测证明rC3B具有抗原性,单核细胞的黏附实验初步确认了纯化后蛋白的活性。
结论:确认了人类补体C3的这一活性区域,并取得了该蛋白的功能片段,为深切研究人类补体C3蛋白的功能和应用奠定了基础。
【关键词】原核表达Cloning, Expression and Identification of FunctionalFragment rC3B of Human Complement C3 in E. ColiAbstract This study was purposed to verify the binding part of human complement C3 to complement receptorⅢ(CRⅢ) in monocytes, the peptide rC3B, including the binding site, was expressed, purified and identified. rC3B, the binding part of human complement C3 to CRⅢ, was selected by computer aided modeling and summarizing researches published. Then, rC3B gene fragment was amplified by PCR, and cloned into prokaryotic vector pQE30a. The fusion protein rC3B was expressed in M15 and purified by Ni2+chelating affinity chromatography. The activity of rC3B was identified by Western blot and adherence assay with monocytes. The results showed that rC3B fragment was obtained, and a prokaryotic expression vector pQE30rC3B was constructed. rC3B was efficiently expressed and purified. In Western blot, the target protein showed the activity of binding with C3 antibody, while the purified protein showed the activity of adherence with monocytes. It is concluded that the recombinant C3B was obtained and identified, and this study lay the basis for the further functional analysis of C3.Key words complement C3; rC3B gene; prokaryote expression;monocyteJ Exp Hematol 2007; 15(4):827-832补体C3蛋白要紧由肝细胞和巨噬细胞产生。
- 1、下载文档前请自行甄别文档内容的完整性,平台不提供额外的编辑、内容补充、找答案等附加服务。
- 2、"仅部分预览"的文档,不可在线预览部分如存在完整性等问题,可反馈申请退款(可完整预览的文档不适用该条件!)。
- 3、如文档侵犯您的权益,请联系客服反馈,我们会尽快为您处理(人工客服工作时间:9:00-18:30)。
Accepted ManuscriptFunctional Expression and Purification of CYP93C20a Plant Membrane-As‐sociated Cytochrome P450 from Medicago truncatulaZhenzhan Chang, Xiaoqiang Wang, Risheng Wei, Zhouying Liu, Hong Shan,Guizhen Fan, Hongli HuPII:S1046-5928(10)00322-0DOI:10.1016/j.pep.2010.11.012Reference:YPREP 3835To appear in:Protein Expression and PurificationReceived Date:7 April 2010Revised Date:16 November 2010Please cite this article as: Z. Chang, X. Wang, R. Wei, Z. Liu, H. Shan, G. Fan, H. Hu, Functional Expression and Purification of CYP93C20a Plant Membrane-Associated Cytochrome P450 from Medicago truncatula, Protein Expression and Purification (2010), doi: 10.1016/j.pep.2010.11.012This is a PDF file of an unedited manuscript that has been accepted for publication. As a service to our customers we are providing this early version of the manuscript. The manuscript will undergo copyediting, typesetting, and review of the resulting proof before it is published in its final form. Please note that during the production process errors may be discovered which could affect the content, and all legal disclaimers that apply to the journal pertain.Functional Expression and Purification of CYP93C20 a PlantMembrane-Associated Cytochrome P450 from Medicago truncatulaZhenzhan Chang , Xiaoqiang Wang, Risheng Wei, Zhouying Liu, Hong Shan,Guizhen Fan, Hongli HuDepartment of Biophysics, Peking University Health Science Center, 38 Xue Yuan Road, Beijing 100191, ChinaAddress correspondence to Dr. Zhenzhan Chang: Department of Biophysics, Peking University Health Science Center, Xue Yuan Road 38, Beijing 100191, China. Tel. +86 10 82805369; Fax: +86 10 82801444; E-mail: changz@Abbreviations used: IFS, isoflavone synthase; ALA, 5-aminolevulinic acid; IPTG, isopropyl -D-1-thiogalactopyranoside; MALDI-TOF-MS, matrix-assisted laser desorption ionization-time of flight-mass spectrometry; SDS-PAGE, sodium dodecyl sulfate polyacrylamide gel electrophoresis; NADPH, nicotinamide adenine dinucleotide phosphate reduced form; HPLC, high performance liquid chromatography.Abstract Plants possess very large numbers of biosynthetic cytochrome P450 enzymes. In spite of the importance of these enzymes for the synthesis of bioactive plant secondary metabolites, only two plant P450 structures has been obtained to date. Isoflavone synthase (IFS) is a membrane-associated cytochrome P450 enzyme catalyzing the entry-point reaction into isoflavonoid biosynthesis. IFS from the model legume Medicago truncatula (CYP93C20) was engineered by deleting the membrane-spanning domain and inserting a hydrophilic polypeptide in the N-terminus and a four histidine tag at the C-terminus. The truncated form exhibited dramatically enhanced expression and solubility. The engineered enzyme was expressed in Escherichia coli XL1-blue cells and was purified by Ni 2+-NTA affinity chromatograph and size-exclusion chromatograph. The purified enzyme was characterized by enzyme assay, reduced carbon monoxide difference spectroscopy and peptide mass fingerprinting. The engineered soluble enzyme exhibited the same activity as the full length membrane-associated enzyme expressed in yeast. These studies suggest an approach for engineering plant membrane-associated P450s with enhanced expression and solubility for mechanistic and structural studies. Keywords : cytochrome P450; isoflavone synthase; functional expression; purification Introduction异黄酮蒺藜状苜蓿Cytochromes P450 (P450s) are external monooxygenases encoded by a superfamily of genes ubiquitously distributed in different organisms from all biological kingdoms. The P450s reactions are extremely diverse and contribute to the biotransformation of drugs, the异型生物质bioconversion of xenobiotics, the biosynthesis of physiologically important compounds such as steroids, fatty acids, fat-soluble vitamins and bile acids, the metabolism of chemical carcinogens, as well as the degradation of herbicides and insecticides [1]. Eukaryotic P450s are expressed at low levels, sometimes as P420 forms, the biologically inactive forms which possess ferrous CO Soret absorption at 420 nm [2]. Therefore their crystallization and structure determination are challenging. Progress on structural studies of mammalian P450 enzymes have been significantly advanced by the use of E. coli as a heterologous expression system. Efforts to decrease the hydrophobicity and thus increase the solubility of mammalian P450s have primarily focused on deletion of the N-terminal membrane anchor with addition of a His-tag at the C-terminus to aid in protein purification. Williams et al.crystallized and determined the structure of the first membrane-bound P450 (2C5). They expressed a soluble monomeric form compatible with growing diffraction-quality crystals by truncating the N-terminus and introducing mutations into a peripheral membrane-binding site in the F-G loop region [3]. Success in crystallographic studies of other mammalian P450s, (e.g. 2B4, 3A4, 2C8 and 2A6) [4, 5, 6, 7] and plant P450 CYP74A [8], suggested that removal of the N-terminal transmembrane domain with addition of a C-terminal His-tag is sufficient to crystallize these enzymes, without need for additional mutations in the F-G loop region.Several widely used expression systems have been developed to produce catalytically active plant P450s in bacteria, yeast and insect cells, with the yeast expression system being themost frequently used [9]. Plant P450 expression studies in E. coli have focused on the CYP74 [10, 11, 12, 13, 14] and CYP79 [15, 16, 17] families. Arabidopsis CYP90B1 [18], flavonoid 3’, 5’-hydroxylase (F 3’5’H) [19] and carotene hydroxylases (CYP97 family) [20] have also been expressed in E. coli. However, only a few plant P450s (eg. CYP74A1, CYP74C3, CYP74A2, CYP74A) have been produced in E. coli at the milligram scale necessary for crystallization studies [13, 14, 21, 8] and so far only two crystal structures of plant P450s (CYP74A2 and CYP74A) have been determined [22, 8].Here we describe the membrane-associated CYP93C20 IFS from the model legume Medicago truncatula utilizing molecular engineering techniques. We were able to obtain soluble CYP93C20 protein in milligram quantities that is functionally characterized, highly active, homogeneous and detergent-free. The CYP93C20 protein obtained is prepared for crystallographic studies. Our results describe a general methodology for molecular engineering and purification of membrane-associated plant P450 enzymes.Materials and methodsChemicalsOligonucleotides for cloning, site-directed mutagenesis and DNA sequencing were purchased from IDT (Coralville, IA, USA). Liquiritigenin was purchased from Indofine chemical company, Inc. (Hillsborough, NJ, USA), NADPH-P450 oxidoreductase (Human, recombinant) from Calbiochem-EMD Biosciences (La Jolla, CA, USA), NADPH, ALA and sodium cholate hydrate from Sigma-Aldrich (St. Louis, MO, USA), IPTG from Promega (Madison, WI, USA) and Ni2+-NTA affinity resin from Qiagen (Valencia, CA, USA).Generation of recombinant CYP93C20 constructsAlignment of amino acid sequences of CYP93C20 from Medicago truncatula with mammalian P4502C5 and 2B4 [3, 4] was performed using ClustalW (Fig. 1). Based on the sequence alignment analysis, four truncated forms of CYP93C20 were designed and generated using the templates and primers listed in Table 1. CYP93C20 cDNA encoding M. truncatula IFS (GenBank: AY167424) served as an initial template for polymerase chain reaction (PCR). The forward 5’-primer was designed to introduce an Nde I restriction site and to delete codons for residues 2-18 followed by a sequence corresponding to codons for residues 19-24 of CYP93C20. The reverse 3’-primer includes an Xba I restriction site, the termination codon TTA, codons for four histidines, and a sequence complementary to codons for residues 522-517 of CYP93C20. The PCR products were digested with Nde I and Xba I, and subcloned into pCW ori+ expression vector. This cloning strategy generated the construct that we call pCW ori+ 2-18ifsH which contained 4xHis tag at the C-terminus and deleted the N-terminal 17 amino acids.The construct pCW ori+ 2-34ifsH (with further deletion of N-terminal amino acids 19-34) was generated using the QuikChange site-directed mutagenesis strategy (Stratagene) with pCW ori+ 2-18ifsH as template and the set of complementary primers listed in Table 1.To generate constructs with insertion of a short charged, hydrophilic polypeptide at the N-terminus, site-directed mutagenesis was performed using pCW ori+ 2-34ifsH as template with the primers shown in Table 1. This strategy replaced the N-terminal transmembrane signal anchor domain (residues 1-34) with an optimized sequence encodingthe peptide sequence MAKKTSSKGKL and inserted four codons for histidine upstream of the stop codon to facilitate purification of the enzyme. The resulting plasmid was termed pCW ori+ 2-34 10ifsH.In order to determine how the C-terminus sequence of CYP93C20 affects the expression and purification of CYP93C20, we deleted amino acids ARAGVADKLLSS utilizing site-directed mutagenesis with 2-34 10ifsH as the template with primers in Table 1 to generate a construct pCW ori+ 2-34 10ifs 12H.Optimization of expression of recombinant CYP93C20 in E. coliThe construct pCW ori+ 2-34 10ifsH was transformed into BL21(DE3) competent cells to screen expression conditions for recombinant CYP93C20. One colony of BL21(DE3)/ pCW ori+ 2-34 10ifsH was inoculated into 50 ml LB medium containing 100 g/ml ampicillin and incubated at 37o C and 250 rpm; 100 ml overnight cultures were started by inoculating with 0.5 ml aliquots of overnight culture. Induction of recombinant CYP93C20 was initiated at OD600 = 0.5 by adding IPTG to a final concentration of 0.5 mM or 1 mM; ALA was supplemented as a precursor for heme synthesis to a final concentration of 0.5 mM or 1 mM. Incubation was continued with shaking at 190 rpm at 16, 28 or 30o C. The expression level was assessed at 24, 32, 48 and 72 h by SDS-PAGE analysis.To select the best E. coli strains for expression of recombinant CYP93C20, the construct pCW ori+ 2-34 10ifsH was transformed into different competent cell lines. One liter batches of LB medium fortified with suitable antibiotics for BL21(DE3) cells (100 g/ml ampicillin ), XL1-blue cells (100 g/ml ampicillin and 12.5 g/ml tetracycline), JM109 cells(100 g/ml ampicillin), Rosetta (DE3) cells (50 g/ml chloramphenicol) and DH5 cells (100 g/ml ampicillin) were started in 2-liter culture flasks at 37o C and 250 rpm. At OD600= 0.4~0.6, IPTG was added to a final concentration of 1mM to induce expression. ALA was supplemented at a final concentration of 0.5 mM and imidazole was added to a final concentration of 5 mM at the time of induction to stabilize holoprotein. Cultures were grown for an additional 48 h at 28o C and 190 rpm. The cultures were placed on ice for 15 min and the cells harvested by centrifugation at 5000×g for 10 min at 4°C. Enzyme was purified by Ni-NTA affinity chromatography from individual culture extracts. The expression level was compared by SDS-PAGE analysis.The construct pCW ori+ 2-34 10ifs 12H was also transformed into XL1-blue competent cells for determining its expression level in E. coli.UV-visible and carbon monoxide difference spectra of recombinant CYP93C20 UV-visible and carbon monoxide difference spectroscopy was performed using a dual-beam scanning Shimadzu UV-visible spectrophotometer (Model UV-1601; Shimadzu, Milton Keynes, U.K.) A 200 ml culture of XL1-blue cells transformed with pCW ori+ 2-34 10ifsH was prepared as described above. The cells were harvested and resuspended in 10 ml cell lysis buffer (500 mM potassium phosphate pH 7.4, 250 mM KCl, 1 mM PMSF, 10% glycerol), followed by sonication for 10 X 20 s with 40 s intervals on ice and centrifugation at 29000 g for 45 min.The reduced-CO difference spectrum of the supernatant containing CYP93C20 was obtained according to a published method [23]. Briefly, 3 ml of the supernatant wasreduced with a few mg of sodium dithionite, and equal volumes of this preparation were distributed into sample and reference quartz cuvettes (0.7 ml) for baseline correction. CO was gently bubbled through the sample cuvette for 1 min prior to recording the difference spectrum from 500 to 400 nm.Peptide mass fingerprintingIn-gel digestion, MALDI-TOF-MS and database searching were performed according to a reported method [24]. Briefly, protein bands were excised from the SDS-PAGE gel, washed and destained completely with 50% acetonitrile in 50 mM ammonium bicarbonate. The gel pieces were then dehydrated with acetonitrile and dried in a vacuum centrifuge. Digestion was performed overnight with bovine trypsin (10 ng/ l), stopped by addition of 10% formic acid, and the supernatant recovered. The gel pieces were extracted once with 25 l 50% acetonitrile and once with 100% acetonitrile. The supernatant was combined and dried in a vacuum centrifuge. Peptides were suspended in a 1:1 (v/v) solution of 2% formic acid in acetonitrile, mixed 1:1 (v/v) with matrix (10 mg ml-1 –cyano-4-hydroxycinnamic acid in the same solution), and spotted on a MALDI plate. MALDI-TOF-MS peptide maps were analyzed by a database search against NCBInr (release January 9, 2005) using MS-Fit () with the following parameters: Mass accuracy, 100 ppm; missed cleavage, 1; possible modifications, oxidation of Met.Purification of recombinant CYP93C20Large scale (usually 4 liters) expression with XL1-blue or BL21(DE3) cells transformed with pCW ori+ 2-34 10ifsH was carried out to produce recombinant CYP93C20.A 5 ml overnight culture was inoculated into 1 liter of LB medium containing 100 g/ml ampicillin and 12.5 g/ml tetracycline, maintaining the same expression conditions as used to screen competent cells.Red cell pellets were resuspended in lysis buffer (500 mM potassium phosphate, 0.25 M NaCl, 10 mM -mercaptoethanol, 10% glycerol, 10 mM imidazole, 0.25% sodium cholate, pH 7.4). Lysozyme (0.2 mg/ml), Dnase I (1.2 g/ml), MgSO4 (2 mM) and phenylmethanesulfonyl fluoride (1 mM) were added. The cells were incubated and stirred for 30 min at 4°C and homogenized by passing through a French press cell homogenizer, followed by centrifugation at 29,000 g for 1 h. The supernatant containing the CYP93C20 was applied to a Ni2+-NTA column previously equilibrated with 20 column volumes of equilibration buffer/wash buffer A (500 mM potassium phosphate, pH 7.4, 0.5 M NaCl, 10 mM -mercaptoethanol, 10% glycerol). The column was washed with: (1), 100 column volumes of equilibration buffer/wash buffer A; (2), 100 column volumes of wash buffer B (10 mM potassium phosphate, pH 7.4, 0.5 M NaCl, 10 mM -mercaptoethanol, 20 mM imidazole, 10% glycerol) and (3), 20 column volumes of wash buffer C (10 mM potassium phosphate, pH 7.4, 0.5 M NaCl, 10 mM -mercaptoethanol, 40 mM imidazole, 10% glycerol). The protein was then eluted with elution buffer (10 mM potassium phosphate, pH7.4, 0.5 M NaCl, 10 mM -mercaptoethanol, 250 mM imidazole, 10% glycerol). Fractions with the highest concentration of protein were pooled. Finally the protein was purified by gel filtration on a HiLoad 16/60 Superdex 200 column eluting in gel filtration buffer (10 mM potassiumphosphate, pH 7.2, 0.5 M NaCl, 1 mM DTT, 0.2 mM EDTA, 10% glycerol). The red-colored fractions were pooled and concentrated with a Vivaspin 20 concentrator (30 kDa molecular-weight cut-off, Stonehouse, UK).Assay of enzyme activity of recombinant CYP93C20Transformation of yeast WAT11 cells with the empty pYeDP60 vector and pYeDP60-IFS construct 51865 and preparation of microsomes were conducted as previously described [23]. E. coli-expressed recombinant CYP93C20 (20 M), NADPH (1 mM) and liquiritigenin (80 M) were mixed in reaction buffer (0.1 M K2HPO4, 0.4 M sucrose, 0.5 mM glutathione, pH 8.0) with approximately 1 mg of microsomes containing Arabidopsis thaliana(ATR1) NADPH-P450 reductase prepared from yeast WAT11 cells transformed with pYeDP60 vector, or 0.7 units of human NADPH-P450 oxidoreductase, and incubated overnight at 16 o C. Microsomes (1 mg) prepared from yeast WAT11 cells transformed with pYeDP60-IFS construct 51865 were incubated with liquiritigenin in reaction buffer as positive control.Following incubation, reactions were stopped and extracted three times with one volume of ethyl acetate. Ethyl acetate extracts were combined, dried under a stream of N2, and the residues resuspended in 100 l methanol. HPLC analysis was performed on an Agilent HP1100 HPLC equipped with an auto sampler, quaternary pump, and diode array detector. Solvent A was 1% aqueous phosphoric acid and solvent B was acetonitrile. Reaction samples (40 l) were applied to an ODS reverse-phase column (5 m particle size, 4.6 x 250 mm) and eluted in 1% (v/v) phosphoric acid with an increasing gradient ofacetonitrile (0-5 min, 5%; 5-10 min, 5-10%; 10-25 min, 10-17%; 25-30 min, 17-23%; 30-65 min, 23-50%; 65-69 min, 50-100%) at a flow rate of 1 ml min-1. The eluants were monitored at 235, 254 and 270 nm. Isoflavonoid products were identified by comparing retention times and UV spectra with those of authentic standards.Results and discussionEffect of N-terminal and C-terminal modifications on protein expressionThe first eukaryotic P450 structure solved was that of mammalian P450 2C5 (Williams, 2000), which was engineered to delete the N-terminal membrane-spanning helix ( 3-21) and to mutate a peripheral membrane–binding site to facilitate protein expression. For solving the structure of rabbit P450 2B4, the N-terminal transmembrane domain was truncated ( 3-21) and several N-terminal residues were mutated to facilitate expression.CYP93C20 from M. truncatula is also a membrane-associated P450, and modification of its amino acid sequence is also required to enhance solubility and achieve high-level expression level. To design truncation forms of CYP93C20, its amino acid sequence was aligned with those of two structurally characterized mammalian P450s (2C5 and 2B4) [25, 3, 4, 26]. The alignment showed that the amino acid sequence identity between M. truncatula CYP93C20 and the two mammalian P450s had about 20% homology, but they share many conserved features such as the heme binding motif (Fig. 1). A conserved proline-rich region with a PXXP motif is also present in the N-terminus between the signal-anchor and catalytic domain, and previous work on cytochrome P450 2C2 and others suggested its importance inthe formation of a functional cytochrome P450 across species from humans to plants [27, 28, 29]. Based on the sequence alignment, three truncated forms of CYP93C20 were designed to delete the putative transmembrane domain ( 2-18, 2-24, 2-29) and keep the proline-rich motif. All constructs had low level or no expression in E. coli. Additionally, reduced-CO difference spectra shown the proteins existed predominately as inactive cytochrome P420 (data not shown).The construct 2-34ifsH was designed to remove the N-terminal residues 2-34. Subsequently, 2-34 10ifsH was generated to incorporate the small peptide AKKTSSKGKL in the N-terminus of 2-34ifsH by referring to the method for generateing the construct which was successful in solubilization of cytochrome 2B4 [26]. 2-34 10ifsH was transformed into BL21(DE3) cells for expression. The expression conditions were optimized with respect to temperature (28o C), time (48h), aeration (190 rpm), and concentrations of IPTG (1 mM) and ALA (0.5 mM).The optimized growth conditions were applied to cultures of different E. coli strains transformed with 2-34 10ifsH. SDS-PAGE analysis (Fig. 2) indicated that XL1-blue, BL21 (DE3), DH5 and Rosetta (DE3) cells gave higher level expression of the target protein than JM109 cells. However, Rosetta (DE3) and DH5 cells expressed more non-target proteins than with the other cells. XL1-blue and BL21 (DE3) cells were therefore chosen for large scale expression of CYP93C20.To analyze the effects of the C-terminal sequence on protein expression/stability, the C-terminally truncated form pCW ori+ 2-34 10ifs 12H was expressed in XL1-blue cells. The cells were less pink in color than those transformed with pCW ori+ 2-34 10ifsH withoutthe C-terminal truncation. Further purification trials showed that the protein did not bind well to Ni2+-NTA beads, suggesting that residues at the C-terminus of CYP93C20 are important for the enzyme to form the correct conformation.Identification of recombinant CYP93C20 by peptide mass fingerprintingTo confirm that the protein expressed and purified from E. coli was recombinant CYP93C20, peptide mass fingerprint analysis was performed with a mass spectrometry with bands excised from the Coomassie-stained SDS-PAGE gel. High quality MALDI-TOF-MS peptide maps were obtained (Fig. 3), and analyzed by a database search against NCBInr. Two proteins were successfully identified. Band 1 from the SDS-PAGE (Fig.2) was identified as chaperone Hsp70 with 34 peptides and sequence coverage of 59%. Band 2, the major band in the SDS-PAGE, was identified as isoflavone synthase from Medicago truncatula with 13 peptides and sequence coverage of 29%.Spectral properties of recombinant engineered CYP93C20The UV-visible spectrum of CYP93C20 showed a Soret band at 391 nm and major features at 508 and 545 nm (Fig. 4). The reduced-CO difference spectrum had a characteristic peak at 450 nm; however, under the present culture conditions, a peak for cytochrome P420, the denatured form of CYP93C20, was also observed.The reduced-CO difference spectrum of microsomes from yeast expressing the full-length CYP93C20 also exhibited a major peak at 450 nm; however, after the microsomes had been kept in the spectrophotometer for 3 min, or if the enzyme remained at roomtemperature for 5-10 min prior to reduction, a peak at 420 nm would be observed. Very similar culture conditions have been used in our laboratory to express another plant P450 enzyme, allene oxide synthase, in E. coli with no appreciable degradation to cytochrome P420. Our observations suggest that CYP93C20 is inherently unstable when expressed either as the full-length protein in yeast or as an engineered form in E. coli, and may require additional factors and more careful handling to maintain it in the active P450 form during expression and purification.Purification of recombinant engineered CYP93C20The engineered CYP93C20 pCW ori+ 2-34 10ifsH expressed in XL1-blue or BL21(DE3) cells was purified using two chromatography steps. Chromatography on Ni2+-NTA column appeared to be an efficient purification step and led to a significant enrichment of the P450. Subsequent gel filtration chromatography resulted in nearly homogeneous CYP93C20, which appeared as a single band of SDS-PAGE analysis (Fig. 5).The gel filtration profile using a HiLoad 16/60 Superdex 200 preparative column showed that engineered CYP93C20 was a monomer, with a molecular weight value matching that calculated based on its primary sequence. The red-colored fractions were pooled and used for crystallization of CYP93C20. In some circumstances after gel filtration, a small proportion of soluble aggregated protein was also observed (Fig. 6). The polymer had the same purity, but with very low heme content and was not used for crystallization.Expression of the construct pCW ori+ 2-34 10ifsH in E. coli XL1-blue or BL21(DE3) cells and subsequent purification by Ni2+-NTA affinity chromatography yielded ~25 mg CYP93C20 per liter of culture medium as assayed by the Bradford method [30].Enzymatic activity of recombinant engineered CYP93C20To determine whether the recombinant engineered CYP93C20 retains full catalytic activity, enzymatic activity assays were performed using liquiritigenin as substrate, in the presence of NADPH and NADPH-P450 reductase (either as microsomes from yeast expressing the Arabidopsis reductase, or commercial human P450 oxidoreductase). Reaction products were separated by HPLC and their identities confirmed by comparison of retention times and UV spectra to those of authentic standards.When E. coli expressed recombinant CYP93C20 was incubated with yeast microsomes containing plant P450 reductase, liquiritigenin, and NADPH, a major product, daidzein, was observed, along with a smaller amount of 2,7,4’-trihydroxyisoflavanone (Fig. 7b). This indicated that the engineered CYP93C20 converted liquiritigenin to 2,7,4’-trihydroxyisoflavanone, and was subsequently dehydrated non-enzymatically to daidzein. Similar amounts of daidzein were produced under these conditions when yeast-expressed full-length CYP93C20 was incubated with liquiritigenin and NADPH (Fig. 7a, b). Daidzein was also formed when the soluble engineered CYP93C20 was incubated with liquiritigenin, NADPH and human NADPH-P450 oxidoreductase, but at levels less than 10% of those produced when using the Arabidopsis reductase (Fig. 7c). Thus, the solubleengineered CYP93C20 maintained full catalytic activity when the plant P450 reductase was supplied as the electron donor from NADPH.ConclusionsOverall, our work has demonstrated that it is possible to optimize construct design and expression system to generate large amounts of soluble, active IFS. These approaches should be applicable to other plant P450s. The success in CYP93C20 expression, purification will facilitate future structural studies to understand how IFS carries out the novel aryl-ring migration for conversion of flavanone to 2-hydroxyisoflavanone, and to reveal the molecular basis of the catalysis and exquisite substrate specificity of IFS and other plant biosynthetic P450s.AcknowledgementsWe thank Dr. Z. Lei for assistance with the MALDI-TOF-MS peptide fingerprint analysis, J. Lin for initial work on cloning and expression, Dr. B. Deavours for help with HPLC analysis, Dr. L. Pedersen (NIEHS, NIH) for kindly providing pCWori+ vector, Dr. F. W. Dahlquist (Institute of Molecular Biology, University of Oregon) for permission to use pCWori+, Drs. R. A. Dixon and C.C. Yin for critical reading of the manuscript. This work was supported by grant 30970572 from the National Natural Science Foundation of China.Figure LegendsFig. 1. Alignment of amino acid sequences of CYP93C20 from Medicago truncatula with rabbit P450s 2C5 and 2B4. Box A indicates the proline-rich region (Kemper, 2004). Box B indicates the highly conserved “I” helix suggested to be involved in O2 binding by P450s, and box C highlights the heme binding motif (Graham-Lorence, 1996). Conserved residues are highlighted. Sequence analysis was performed using ClustalW search on DeCypher Protein Sequences.Fig. 2. SDS-PAGE analysis of engineered CYP93C20 expressed in different E. coli competent cells. BLs, XLs, JMs, DHs, RSs indicated the supernatant of BL21(DE3), XL1-blue, JM109, DH5 , Rosetta (DE3), respectively; BLp, XLp, JMp, DHp, RSp indicated the protein of each cell which was purified with Ni2+-NTA affinity column. Bands 1 and 2 were excised for protein identification by peptide mass fingerprinting.Fig. 3. Peptide mass spectrums obtained using MALDI-TOF-MS. Mass spectral peaks are labeled with monoisotopic mass-to-charge ratio (m/z) values used for database searching. IFS (A) and chaperone Hsp70 (B) were successfully identified using the NCBInr database.Fig. 4. UV-visible absorption spectrum (A) and CO difference spectrum (B) of supernatant of engineered CYP93C20 expressed in E. coli XL1-blue cells.Fig. 5. SDS-PAGE analysis of the purification of CYP93C20 expressed in XL1 blue cells. Lanes 1-3, fractions eluted from Ni2+-NTA with 250 mM imidazole; Lane 4, cell supernatant; Lane 5, fraction from gel filtration chromatography; Lane 7, Pre-stained protein standardsFig. 6. Gel filtration chromatography of CYP93C20 on a HiLoad 16/60 Superdex 200 column. Elution was monitored at 280 nm at a flow rate of 1.0 ml/min.Fig. 7. HPLC elution profiles of enzyme assay mixtures. a, yeast microsomes from pYeDP60-IFS 51865 strain incubated with liquiritigenin and NADPH; b, CYP93C20 incubated with liquiritigenin, NADPH and yeast microsome from pYeDP60 control strain (expressing Arabidopsis P450 reductase); c, CYP93C20 incubated with liquiritigenin, NADPH and human NADPH-P450 oxidoreductase; d, Liquiritigenin standard. L, liquiririgenin; D, Daidzein; H, 2,7,4’-trihydroxyisoflavanone.。