The activation of c + a non-basal slip in Magnesium alloys
腺苷激活A1_与A2A_受体诱导睡眠的研究进展

Advances in Clinical Medicine 临床医学进展, 2023, 13(2), 2083-2092 Published Online February 2023 in Hans. https:///journal/acm https:///10.12677/acm.2023.132291腺苷激活A 1与A 2A 受体诱导睡眠的研究进展蒋单栋1,俞 盈2,任思齐3,邹 莹4,么春艳2*1杭州医学院临床医学院,浙江 杭州 2杭州医学院食品科学与工程学院,浙江 杭州3杭州医学院药学院,浙江 杭州4浙江中医药大学附属第二医院,浙江 杭州收稿日期:2023年1月14日;录用日期:2023年2月8日;发布日期:2023年2月16日摘 要睡眠–觉醒是一个涉及多系统、多中枢的生理过程,这一变化过程可通过脑内多种神经递质和内源性睡眠物质共同作用而实现。
作为一种核苷,腺苷是目前发现的最强的内源性促眠物质之一,激活A 1和A 2A 受体诱导睡眠,其中又以A 2A 受体占主导作用,A 1受体在不同脑区表现出区域特异性。
作为中枢腺苷受体拮抗剂,咖啡因通过纹状体伏隔核壳区的A 2A 受体发挥觉醒效能。
为更好地理解腺苷对睡眠的调节机制以及为新型失眠药的研发提供思路,本文对腺苷的代谢和睡眠稳态、A 1、A 2A 受体对睡眠调节作用的差异、咖啡因与A 2A 受体的关系等内容进行了系统描述。
关键词腺苷,睡眠,咖啡因,腺苷A 1受体,腺苷A 2A 受体Advances in Sleep Induction by Adenosine Activation of A 1 and A 2A ReceptorsShandong Jiang 1, Ying Yu 2, Siqi Ren 3, Ying Zou 4, Chunyan Yao 2*1School of Clinical Medicine, Hangzhou Medical College, Hangzhou Zhejiang 2Institute of Food Science and Engineering, Hangzhou Medical University, Hangzhou Zhejiang 3School of Pharmacy, Hangzhou Medical College, Hangzhou Zhejiang 4The Second Affiliated Hospital of Zhejiang Chinese Medical University, Hangzhou Zhejiang Received: Jan. 14th , 2023; accepted: Feb. 8th , 2023; published: Feb. 16th , 2023*通讯作者。
生理学重要名词解释英文版

Internal environment:内环境 refers to the liquid surrounding the cells in the body of multicellular animals, that is extracellular fluid.Homeostasis稳态: refers to a state of relatively constant of physical and chemical properties of internal environment, such as temperature, pH, osmotic pressure and all kinds of liquid ingredients in the body, and so on.positive feedback正反馈: A change in a condition leads to responses from the effectors which a mplifies that changenegative feedback负反馈: A change in a condition leads to responses from the effectors which counteracts that changefacilitated diffusion via carrier经载体易化扩散: Water soluble small molecules and ions under the carrier protein mediated, cross the plasma membrane follow concentration gradientfacilitated diffusion via ion channel经通道易化扩散: All kinds of charged ions under the channel proteins mediated, cross the plasma membrane follow concentration gradient and potential gradientPrimary active transport原发性主动转运:making direct use of energy derived from ATP to transport the ions across the cell membraneSecondary active transport次级主动转运:The ion gradients established by primary active transport permits the transport of other substances against their concentration gradientsresting potential静息电位: A potential difference across the membranes of inactive cells, with the inside of the cell negative relative to the outside of the cell action potential动作电位: Some of the cells (excitable cells) are capable to rapidly reverse their resting membrane potential from negative resting values to slightly positive values. This transient and rapid change in membrane potential is called an action potentialExcitation-contraction coupling兴奋收缩耦联: the mediation process of striated muscle cells generate action potentials and muscle filament contraction and relaxation.Hematocrit血细胞比容: The capacity percentage of the blood cells in the blood erythrocyte sedimentation rate红细胞沉降率: The distance that red blood cells settle in a tube of blood in one hourHemostasis止血: Small damaged blood vessels stop bleeding after a few minutes automaticallyBlood coagulation血凝固: blood change from sol to illiquid gel stateBlood group血型: The type of specific antigen on the surface of blood cells effective refractory period:premature systole期前收缩: if ventricle is stimulated after ventricular muscle effective refractory period, before The next sinoatrial node excitement arrive, it will produce a contraction in advance.compensatory pause代偿间歇: A longer ventricular diastolic after premature systole.Atrioventricular delay房室延搁: Excitement slowly spread in atrioventricular junction and take longer time.Cardiac cycle心动周期: A cycle of heart contraction and relaxationStroke volume每搏输出量: One side of the ventricular injection volume of blood by a heart throbEjection fraction射血分数: The percentage stroke volume account for ventricularend-diastolic volumeCardiac output心输出量: The blood volume inject by one side of the ventricular per minuteCardiac index心指数: calculate cardiac output by unit surface areasystolic pressure收缩压: The highest blood pressure at mid ventricular systolic . diastolic pressure舒张压: The lowest blood pressure at ventricular end-diastolic. pulse pressure脉压: Systolic blood pressure minus diastolic blood pressure central venous pressure中心静脉压: The blood pressure in right atrium and chest cavity vena cavaMicrocirculation: Blood circulation between arteriole and micro veinEffective filtration pressure有效滤过压 :the pressure difference between filtration and reabsorptionRespiration: The process of gas exchange between the body and its environment vital capacity肺活量: After inhalation complete, the largest gas exhaled from the lungsforced vital capacity用力肺活量: After inhalation complete, exhale the largest gas from the lungs as fast as possibleforced expiratory volume用力呼气量: After inhalation complete, the gas exhaled from the lungs in a certain timeAlveolar Ventilation肺泡通气量: amount of inhaled the fresh air in the alveoli per minute.Pulmonary stretch reflex牵张反射:The reflection of inspiratory inhibit or inhale excited caused by pulmonary inflation and pulmonary deflation.Digestion消化: break down of food into small molecular components small enough to absorb.Mechanical digestion and chemical digestion.Absorption吸收: the small molecules that formed by digestion across the digestion tract mucosa go into blood and lymph.Small wave小波: the spontaneous rhythmic, subthreshold depolarization of the cell membrane of the gastrointestinal tract that characterizes the underlying electrical activity of the bowel.胃液主要成分1.HCl ,parietal cell ,acid sterilization. Activation of pepsinogen, promotion of secretin secretion. Assisted effect of Fe and Ca absorption.2.Pepsinogen胃蛋白酶, chief cell, active in stomach, initially by H ions and then by active pepsin, autocatalytic activation. Pepsin an endopeptidase, which attacks peptide bonds in the interior of large protein molecules.3.Mucus粘液, neck cell and goblet cell, lubrication of the mucosal surface. Protection of the tissue from mechanical damage by food particle.4.intrinsic factor内因子, parietal cell. The intrinsic factor binds to vit B12 and facilitated its absorption.Stimulate gastric secretion刺激胃液分泌ACH gastrin histamine/ somatostatin Digestion phase gastric secretion消化期胃液分泌 Cephalic gastric intestinal ph aseRegulation inhibitory gastric juice secretion胃液分泌抑制性调节1.HCl:inhibite G cell release gastrin;stimulate D cell release somatostatin;in the gastric antrum,inhibition of G cells,release of SST;in the duodenum ,release of secretin,bulbogastrone.2,fat:initiating release of enterogastrone.3,hepertonic solution:entero-gastric reflex.Receptive relaxation 容受性舒张:stimulation of receptor reflex muscle relax in the fundus and stomach body when chew and swallow.Pancreatic juice composition effect胰液成分作用:pancreatic amylase,pancreatic lipase, trypsin,chymotrypsin,HCO3 bicarbonate balance the HCl in duodenum. Prot ect intestinal mucosa TPS and chymolase, pancreatic lipase, pancreatic amylase. Enterohepatic circulation of bile salt.Bile salts were emptied into the small intestine with hepatic bile, about 95% is absorbed into the blood in the terminal ileum, and then synthesizing bile again after the hepatic vein to the liver, then empty into intestine.Physiological functions of bile胆汁生理作用1.Emulsifying or detergent function of bile salts.2.Help in the absorption of: fatly acid, lmonoglycerides, cholesterol, other lipids Peristalsis蠕动: the rhythmic waves of muscular relaxation and contraction are called peristalsis.Receptive relaxation 容受性舒张: stimulation of food on pharynx and esophagus produce relaxation of the lower esophageal sphincter and stomach.Gastric emptying胃排空: the process that the gastric contents are delivered to the duodenum.Thermal equivalent of food热价: calories liberated by 1g food oxidized in body. (kJ/g)Thermal equivalent of oxygen氧热价: heat production by consuming one liter of oxygen to oxidize a specific type of blood. (kJ/L)Respiratory quotient(RQ)呼吸商: in the process of oxidizing food, the ratio of CO2 produced to O2 used。
(细胞生物学专业优秀论文)组蛋白乙酰化修饰调控果蝇热休克基因表达和寿..
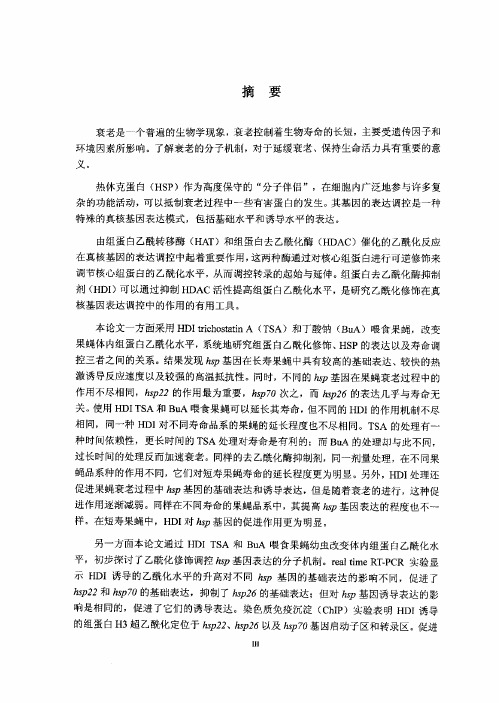
摘要衰老是一个普遍的生物学现象,衰老控制着生物寿命的长短,主要受遗传因子和环境因素所影响。
了解衰老的分子机制,对于延缓衰老、保持生命活力具有重要的意义。
热休克蛋白(HSP)作为高度保守的“分子伴侣”,在细胞内广泛地参与许多复杂的功能活动,可以抵制衰老过程中一些有害蛋白的发生。
其基因的表达调控是一种特殊的真核基因表达模式,包括基础水平和诱导水平的表达。
由组蛋白乙酰转移酶(HAT)和组蛋白去乙酰化酶(HDAC)催化的乙酰化反应在真核基因的表达调控中起着重要作用,这两种酶通过对核心组蛋白进行可逆修饰来调节核心组蛋白的乙酰化水平,从而调控转录的起始与延伸。
组蛋白去乙酰化酶抑制剂(HDI)可以通过抑制HDAC活性提高组蛋白乙酰化水平,是研究乙酰化修饰在真核基因表达调控中的作用的有用工具。
本论文一方面采用HDItrichostatinA<TSA)和丁酸钠(BuA)喂食果蝇,改变果蝇体内组蛋白乙酰化水平,系统地研究组蛋白乙酰化修饰、HSP的表达以及寿命调控三者之间的关系。
结果发现hsp基因在长寿果蝇中具有较高的基础表达、较快的热激诱导反应速度以及较强的高温抵抗性。
同时,不同的hsp基因在果蝇衰老过程中的作用不尽相同,hsp22的作用最为重要,hsp70次之,而hsp26的表达几乎与寿命无关。
使用HDITSA和BuA喂食果蝇可以延长其寿命,但不同的HDI的作用机制不尽相同,同一种HDI对不同寿命品系的果蝇的延长程度也不尽相同。
TSA的处理有一种时间依赖性,更长时间的TSA处理对寿命是有利的;而BuA的处理却与此不同,过长时间的处理反而加速衰老。
同样的去乙酰化酶抑制剂,同一剂量处理,在不同果蝇品系种的作用不同,它们对短寿果蝇寿命的延长程度更为明显。
另外,HDI处理还促进果蝇衰老过程中hsp基因的基础表达和诱导表达,但是随着衰老的进行,这种促进作用逐渐减弱。
同样在不同寿命的果蝇品系中,其提高hsp基因表达的程度也不一样。
生物专业英语词汇-常用前缀

生物专业英语词汇 - 常用前缀第一节表示无,抗,非的前缀一.a-,an-无,非。
无可以进一步理解为离开,除去,脱去等1. 无abacteria 无菌的,atony 无力,anemia 贫血(无血之意)。
abiosis 无生命,abraehia 无臂畸形,adaerya 无泪,asaetylia 无指(趾)畸形,asendrie无树突的,adermotrophia 皮肤萎缩(即无皮肤营养之意),adiaphoresis 无汗症。
2. 否定asymmetercal 不对称的,asynergy 不协调,astnthests 不连接,aststole 心搏停止(心脏不收缩)。
atactic 共济失调的(不协调),astnchronous 不同步的,askyllabia 拼音不能。
3. 离开aspiration 吸引(即吸除之意),aberrent 迷走的(即离开正常途径的)。
4. an-在元音前用an-anaerove 厌氧菌,anesthesia 无感觉,麻醉,analgesia 无痛法,痛觉消失,anamniotic 无羊膜的,anangioplasia 血管发育不全,anapepsia 胃蛋白酶缺乏,anaplasia 发育不全,anascitia 无腹水的,anastigmatic 无散光的,anacholia 胆汁缺乏。
二,ab-去,离开,除abnormal 不正常的(即背离正常的),abapical 离尖的,离心尖的(尖以外的,心尖外的)。
abarticular 关节外的,abaxial 轴外的,离轴的,abduct 外展神经,ablactation 断奶(离开奶)。
abneural 神经外的。
三.ant-,anti-,对抗,取消,抑制,解除antagonistic 对抗的,antioxin 搞毒素,antibody 抗体,antigen 抗原,antacid 制酸剂,antipyretie 解热剂,antibiotic 抗菌素,antispasmin 解痉剂,→antiamylase 抗淀粉酶,antieoagulant 抗凝的。
tumor suppressor genes
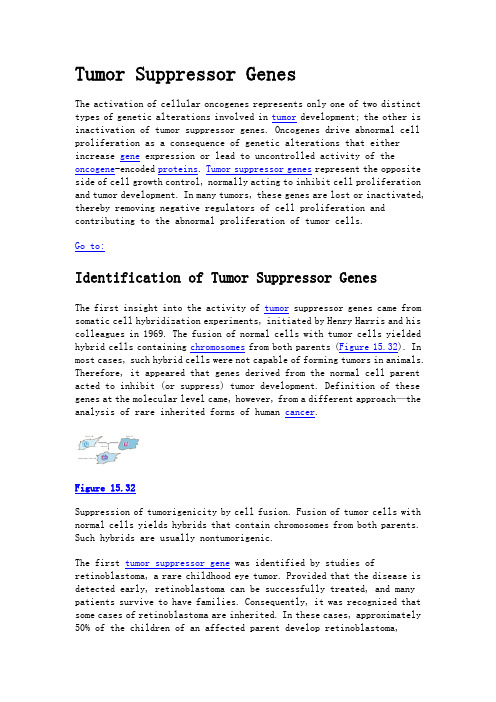
Tumor Suppressor GenesThe activation of cellular oncogenes represents only one of two distinct types of genetic alterations involved in tumor development; the other is inactivation of tumor suppressor genes. Oncogenes drive abnormal cell proliferation as a consequence of genetic alterations that either increase gene expression or lead to uncontrolled activity of the oncogene-encoded proteins. Tumor suppressor genes represent the opposite side of cell growth control, normally acting to inhibit cell proliferation and tumor development. In many tumors, these genes are lost or inactivated, thereby removing negative regulators of cell proliferation and contributing to the abnormal proliferation of tumor cells.Go to:Identification of Tumor Suppressor GenesThe first insight into the activity of tumor suppressor genes came from somatic cell hybridization experiments, initiated by Henry Harris and his colleagues in 1969. The fusion of normal cells with tumor cells yielded hybrid cells containing chromosomes from both parents (Figure 15.32). In most cases, such hybrid cells were not capable of forming tumors in animals. Therefore, it appeared that genes derived from the normal cell parent acted to inhibit (or suppress) tumor development. Definition of these genes at the molecular level came, however, from a different approach—the analysis of rare inherited forms of human cancer.Figure 15.32Suppression of tumorigenicity by cell fusion. Fusion of tumor cells with normal cells yields hybrids that contain chromosomes from both parents. Such hybrids are usually nontumorigenic.The first tumor suppressor gene was identified by studies of retinoblastoma, a rare childhood eye tumor. Provided that the disease is detected early, retinoblastoma can be successfully treated, and many patients survive to have families. Consequently, it was recognized that some cases of retinoblastoma are inherited. In these cases, approximately 50% of the children of an affected parent develop retinoblastoma,consistent with Mendelian transmission of a single dominant gene that confers susceptibility to tumor development (Figure 15.33).Figure 15.33Inheritance of retinoblastoma. Susceptibility to retinoblastoma is transmitted to approximately 50% of offspring. Affected and normal indivi- duals are indicated by purple and green symbols, respectively.Although susceptibility to retinoblastoma is transmitted as a dominant trait, inheritance of the susceptibility gene is not sufficient to transform a normal retinal cell into a tumor cell. All retinal cells in a patient inherit the susceptibility gene, but only a small fraction of these cells give rise to tumors. Thus, tumor development requires additional events beyond inheritance of tumor susceptibility. In 1971, Alfred Knudson proposed that the development of retinoblastoma requires two mutations, which are now known to correspond to the loss of both of the functional copies of the tumor susceptibility gene (the Rb tumor suppressor gene) that would be present on homologous chromosomes of a normal diploid cell (Figure 15.34). In inherited retinoblastoma, one defective copy of Rb is genetically transmitted. The loss of this single Rb copy is not by itself sufficient to trigger tumor development, but retinoblastoma almost always develops in these individuals as a result of a second somatic mutation leading to the loss of the remaining normal Rb allele. Noninherited retinoblastoma, in contrast, is rare, since its development requires two independent somatic mutations to inactivate both normal copies of Rb in the same cell.Figure 15.34Mutations of Rb during retinoblastoma development. In hereditary retinoblastoma, a defective copy of the Rb gene (Rb-) is inherited fromthe affected parent. A second somatic mutation, which inactivates the single normal Rb+ copy in a retinal cell, then (more...)The functional nature of the Rb gene as a negative regulator of tumorigenesis was initially indicated by observations of chromosome morphology. Visible deletions of chromosome 13q14 were found in some retinoblastomas, suggesting that loss (rather than activation) of the Rb gene led to tumor development (Figure 15.35). Gene-mapping studies further indicated that tumor development resulted from loss of normal Rb alleles in the tumor cells, consistent with the function of Rb as a tumor suppressor gene. Isolation of the Rb gene as a molecular clone in 1986 then firmly established that Rb is consistently lost or mutated in retinoblastomas. Gene transfer experiments also demonstrated that introduction of a normal Rb gene into retinoblastoma cells reverses their tumorigenicity, providing direct evidence for the activity of Rb as a tumor suppressor.Figure 15.35Rb deletions in retinoblastoma. Many retinoblastomas have deletions of the chromosomal locus (13q14) that contains the Rb gene.Although Rb was identified in a rare childhood cancer, it is also involved in some of the more common tumors of adults. In particular, studies of the cloned gene have established that Rb is lost or inactivated in many bladder, breast, and lung carcinomas. The significance of the Rb tumor suppressor gene thus extends beyond retinoblastoma, apparently contributing to development of a substantial fraction of more common human cancers. In addition, as noted earlier in this chapter, the Rb protein is a key target for the oncogene proteins of several DNA tumor viruses, including SV40, adenoviruses, and human papillomaviruses, which bind to Rb and inhibit its activity (Figure 15.36). Transformation by these viruses thus results, at least in part, from inactivation of Rb at the protein level rather than from mutational inactivation of the Rb gene.Figure 15.36Interaction of Rb with oncogene proteins of DNA tumor viruses. The oncogene proteins of several DNA tumor viruses (e.g., SV40 T antigen) induce transformation by binding to and inactivating Rb protein.Characterization of Rb as a tumor suppressor gene served as the prototype for the identification of additional tumor suppressor genes that contribute to the development of many different human cancers (Table 15.5). Some of these genes were identified as the causes of rare inherited cancers, playing a role similar to that of Rb in hereditary retinoblastoma. Other tumor suppressor genes have been identified as genes that are frequently deleted or mutated in common noninherited cancers of adults, such as colon carcinoma. In either case, it appears that most tumor suppressor genes are involved in the development of both inherited and noninherited forms of cancer. Indeed, mutations of some tumor suppressor genes appear to be the most common molecular alterations leading to human tumor development.Table 15.5Tumor Suppressor Genes.The second tumor suppressor gene to have been identified is p53, which is frequently inactivated in a wide variety of human cancers, including leukemias, lymphomas, sarcomas, brain tumors, and carcinomas of many tissues, including breast, colon, and lung. In total, mutations of p53 may play a role in up to 50% of all cancers, making it the most common target of genetic alterations in human malignancies. It is also of interest that inherited mutations of p53 are responsible for genetic transmission of a rare hereditary cancer syndrome, in which affectedindividuals develop any of several different types of cancer. In addition, the p53 protein (like Rb) is a target for the oncogene proteins of SV40, adenoviruses, and human papillomaviruses.Like p53, the INK4 and PTEN tumor suppressor genes are very frequently mutated in several common cancers, including lung cancer, prostate cancer, and melanoma. Two other tumor suppressor genes (APC and MADR2) are frequently deleted or mutated in colon cancers. In addition to being involved in noninherited cases of this common adult cancer, inherited mutations of the APC gene are responsible for a rare hereditary form of colon cancer, called familial adenomatous polyposis. Individuals with this condition develop hundreds of benign colon adenomas (polyps), some of which almost inevitably progress to malignancy. Inherited mutations of two other tumor suppressor genes, BRCA1and BRCA2, are responsible for hereditary cases of breast cancer, which account for 5 to 10% of the total breast cancer incidence.Additional tumor suppressor genes have been implicated in the development of brain tumors, pancreatic cancers, and basal cell skin carcinomas, as well as in several rare inherited cancer syndromes, such as Wilms' tumor. The number of identified tumor suppressor genes is rapidly expanding, and the characterization of these genes remains an active area of cancer research.Go to:Functions of Tumor Suppressor Gene ProductsIn contrast to proto-oncogene and oncogene proteins, the proteins encoded by most tumor suppressor genes inhibit cell proliferation or survival. Inactivation of tumor suppressor genes therefore leads to tumor development by eliminating negative regulatory proteins. In several cases, tumor suppressor proteins inhibit the same cell regulatory pathways that are stimulated by the products of oncogenes.The protein encoded by the PTEN tumor suppressor gene is an interesting example of antagonism between oncogene and tumor suppressor gene products (Figure 15.37). The PTEN protein is a lipid phosphatase that dephosphorylates the 3 position of phosphatidylinositides, such as). By dephosphorylating phosphatidylinositol 3,4,5-bisphosphate (PIP3PIP, PTEN antagonizes the activities of PI 3-kinase and Akt, which can 3act as oncogenes by promoting cell survival. Conversely, inactivation or loss of the PTEN tumor suppressor protein can contribute to tumordevelopment as a result of increased levels of PIP, activation of Akt,3and inhibition of programmed cell death.Figure 15.37Suppression of cell survival by PTEN. The tumor suppressor protein PTENat the 3 position of is a lipid phosphatase that dephosphorylates PIP3. PTEN thus counters the action of the oncogenes inositol, yielding PIP2PI 3-kinase and Akt, which promote cell (more...)Proteins encoded by both oncogenes and tumor suppressor genes also function in the Hedgehog signaling pathway (see Figure 13.44). The receptor Smoothened is an oncogene in basal cell carcinomas, whereas Patched (the negative regulator of Smoothened) is a tumor suppressor gene. In addition, the Gli proteins (the mammalian homologs of the Drosophila Ci transcription factor activated by Smoothened) were first identified as the products of an amplified oncogene.Several tumor suppressor genes encode transcriptional regulatory proteins. A good example is provided by the product of WT1, which is frequently inactivated in Wilms' tumors (a childhood kidney tumor). The WT1 protein is a repressor that appears to suppress transcription of a number of growth factor-inducible genes. One of the targets of WT1 is thought to be the gene that encodes insulin-like growth factor II, which is overexpressed in Wilms' tumors and may contribute to tumor development by acting as an autocrine growth factor. Inactivation of WT1 may thus lead to abnormal growth factor expression, which in turn drives tumor cell proliferation. Two other tumor suppressor genes, DPC4 and MADR2, encode SMAD family transcription factors that are activated by TGF-βsignaling and lead to inhibition of cell proliferation.The products of the Rb and INK4tumor suppressor genes regulate cell cycle progression at the same point as that affected by cyclin D1 (Figure 15.38).by repressing Rb inhibits passage through the restriction point in G1transcription of a number of genes involved in cell cycle progression and DNA synthesis (see Figure 14.20). In normal cells, passage through the restriction point is regulated by Cdk4/cyclin D complexes, which phosphorylate and inactivate Rb. Mutational inactivation of Rb in tumorsprogression. The INK4also regulates inhibits Cdk4/cyclin D activity. Inactivation of INK4therefore leads toelevated activity of Cdk4/cyclin D complexes, resulting in uncontrolled phosphorylation of Rb.Figure 15.38Inhibition of cell cycle progression by Rb and p16. Rb inhibits. Cdk4/cyclin D complexes progression past the restriction point in G1promote passage through the restriction point by phosphorylating and inactivating Rb. The activity of Cdk4/cyclin D is (more...)The p53gene product regulates both cell cycle progression and apoptosis (Figure 15.39). DNA damage leads to rapid induction of p53, which activates transcription of the Cdk inhibitor p21 (see Figure 14.21). The inhibitor p21 blocks cell cycle progression, both by acting as a general inhibitor of Cdk/cyclin complexes and by inhibiting DNA replication by binding to PCNA (proliferating cell nuclear antigen). The resulting cell cycle arrest presumably allows time for damaged DNA to be repaired before it is replicated. Loss of p53 prevents this damage-induced cell cycle arrest, leading to increased mutation frequencies and a general instability of the cell genome. Such genetic instability is a common property of cancer cells, and it may contribute to further alterations in oncogenes and tumor suppressor genes during tumor progression.Figure 15.39Action of p53. Wild-type p53 is required for both cell cycle arrest and apoptosis induced by DNA damage.In addition to mediating cell cycle arrest, p53is required for apoptosis induced by DNA damage. Unrepaired DNA damage normally induces apoptosisof mammalian cells, a response that is presumably advantageous to the organism because it eliminates cells carrying potentially deleterious mutations (e.g., cells that might develop into cancer cells). Cells lacking p53 fail to undergo apoptosis in response to agents that damage DNA, including radiation and many of the drugs used in cancer chemotherapy. This failure to undergo apoptosis in response to DNA damage contributes to the resistance of many tumors to chemotherapy. In addition, loss of p53 appears to interfere with apoptosis induced by other stimuli, such as growth factor deprivation and oxygen deprivation. These effects of p53 inactivation on cell survival are thought to account for the high frequency of p53 mutations in human tumors.Go to:Roles of Oncogenes and Tumor Suppressor Genes in Tumor DevelopmentAs discussed earlier, the development of cancer is a multistep process in which normal cells gradually progress to malignancy. The complete sequence of events required for the development of any human cancer is not yet known, but it is clear that both the activation of oncogenes and the inactivation of tumor suppressor genes are critical steps in tumor initiation and progression. Accumulated damage to multiple genes eventually results in the increased proliferation, invasiveness, and metastatic potential that are characteristic of cancer cells.The role of multiple genetic defects is best understood in the case of colon carcinomas, which have been studied extensively by Bert Vogelstein and his colleagues. These tumors frequently involve mutation of ras K oncogenes and inactivation or deletion of three distinct tumor suppressor genes—APC, MADR2,and p53. Lesions representing multiple stages of colon cancer development are regularly obtained as surgical specimens, so it has been possible to correlate these genetic alterations with discrete stages of tumor progression (Figure 15.40).Figure 15.40Genetic alterations in colon carcinomas. Inactivation of APC is an early event in tumor development, giving rise to a proliferative cell population. Mutations of ras K then frequently occur and are found in early-stage adenomas. Subsequent mutations of (more...)These studies indicate that inactivation of APC is an early event in tumor development. Genetic transmission of mutant APC genes in patients with familial adenomatous polyposis results in abnormal colon cell proliferation, leading to the outgrowth of multiple adenomas in the colons of affected patients. Mutations of APC also occur frequently in patients with noninherited colon carcinomas and are generally detected at early stages of the disease process. Mutations of ras K genes then appear to occur, and ras K oncogenes are also frequently present in small and intermediate-size adenomas. In contrast, the MADR2 and p53 tumor suppressor genes are inactivated usually at later stages of tumor progression. Mutations in these genes are only rarely found in early-stage adenomas, but they are frequently present in advanced adenomas and malignant carcinomas. The loss of these tumor suppressor genes in colon cancers thus appears to be involved in later stages of progression to malignancy, rather than in the initial stages of tumor formation.Although these genetic alterations most often occur in the order described here, this is not an obligatory sequence of events. For example, mutations in p53are sometimes detected in early adenomas. More important than the order in which mutations occur is the fact that colon cancer ultimately results from accumulated damage to multiple genes. Accumulated damage toboth oncogenes and tumor suppressor genes similarly appears to be responsible for the development of other types of cancer, including breast and lung carcinomas. The progressive loss of growth control that is characteristic of cancer cells is thus thought to be the end result of abnormalities in the products of multiple genes that normally regulate cell proliferation, differentiation, and survival.BoxMolecular Medicine: Herception: Cancer Treatment Targeted against the erbB-2 Oncogene. Breast cancer is the most common cancer among women, accounting for nearly one-third of all cancers diagnosed in women in the United States. In the year 2000, it is (more...)By agreement with the publisher, this book is accessible by the search feature, but cannot be browsed.。
2009-plant root growth, architecture and function - Hodge
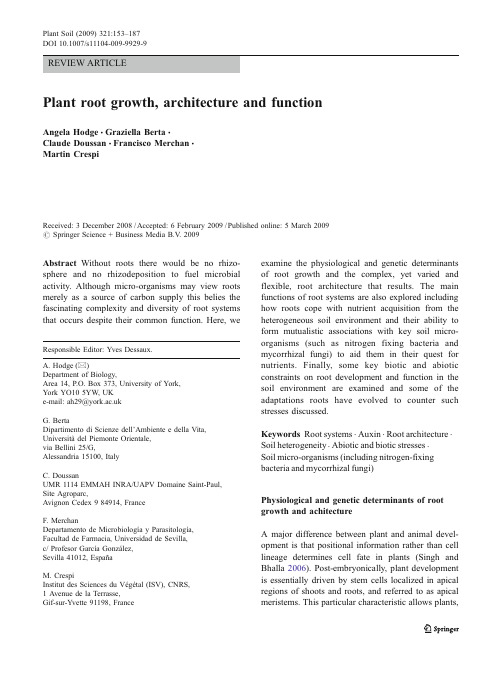
REVIEW ARTICLEPlant root growth,architecture and function Angela Hodge&Graziella Berta&Claude Doussan&Francisco Merchan&Martin CrespiReceived:3December2008/Accepted:6February2009/Published online:5March2009 #Springer Science+Business Media B.V.2009Abstract Without roots there would be no rhizo-sphere and no rhizodeposition to fuel microbial activity.Although micro-organisms may view roots merely as a source of carbon supply this belies the fascinating complexity and diversity of root systems that occurs despite their common function.Here,we examine the physiological and genetic determinants of root growth and the complex,yet varied and flexible,root architecture that results.The main functions of root systems are also explored including how roots cope with nutrient acquisition from the heterogeneous soil environment and their ability to form mutualistic associations with key soil micro-organisms(such as nitrogen fixing bacteria and mycorrhizal fungi)to aid them in their quest for nutrients.Finally,some key biotic and abiotic constraints on root development and function in the soil environment are examined and some of the adaptations roots have evolved to counter such stresses discussed.Keywords Root systems.Auxin.Root architecture. Soil heterogeneity.Abiotic and biotic stresses.Soil micro-organisms(including nitrogen-fixing bacteria and mycorrhizal fungi)Physiological and genetic determinants of root growth and achitectureA major difference between plant and animal devel-opment is that positional information rather than cell lineage determines cell fate in plants(Singh and Bhalla2006).Post-embryonically,plant development is essentially driven by stem cells localized in apical regions of shoots and roots,and referred to as apical meristems.This particular characteristic allows plants,Plant Soil(2009)321:153–187DOI10.1007/s11104-009-9929-9Responsible Editor:Yves Dessaux.A.Hodge(*)Department of Biology,Area14,P.O.Box373,University of York,York YO105YW,UKe-mail:ah29@G.BertaDipartimento di Scienze dell’Ambiente e della Vita, Universitàdel Piemonte Orientale,via Bellini25/G,Alessandria15100,ItalyC.DoussanUMR1114EMMAH INRA/UAPV Domaine Saint-Paul, Site Agroparc,Avignon Cedex984914,FranceF.MerchanDepartamento de Microbiología y Parasitología, Facultad de Farmacia,Universidad de Sevilla,c/Profesor García González,Sevilla41012,EspañaM.CrespiInstitut des Sciences du Végétal(ISV),CNRS,1Avenue de la Terrasse,Gif-sur-Yvette91198,Francewhich are sessile organisms,to adapt their morphol-ogy and organ development to the encountered environmental conditions.The spatial configuration of the root system(number and length of lateral organs),so-called root architecture,vary greatly depending on the plant species,soil composition, and particularly on water and mineral nutrients availability(Malamy2005).Plants can optimize their root architecture by initiating lateral root primordia and influencing growth of primary or lateral roots. The root system results from the coordinated control of both genetic endogenous programs(regulating growth and organogenesis)and the action of abiotic and biotic environmental stimuli(Malamy2005).The interactions between these extrinsic and intrinsic signals however complicate the dissection of specific transduction pathways.Such complex traits likely depending on multiple genes may be analyzed through quantitative genetics via the identification of quantitative trait loci(QTL)linked to root architecture (e.g.Mouchel et al.2004,Fitz Gerald et al.2006). Understanding the molecular mechanisms governing such developmental plasticity is therefore likely to be crucial for crop improvement in sustainable agricul-ture.In this section,we focus on hormonal and genetic determinants of root architecture.The embryonic root apical meristem(RAM) specification occurs very early in embryo develop-ment(Benfey and Scheres2000).The RAM con-stitutes the stem cell niche that eventually produces all below-ground organs,including lateral roots (Sabatini et al.2003).The cellular organization of Arabidopsis RAMs is very regular,with the initials of the various tissue types surrounding a quiescent center(QC).An asymmetric division of the hypoph-ysis generates a large basal daughter and a small apical daughter,called the lens-shaped cell,which is the progenitor of the QC.During root initiation,the QC appears to act like an organizing center that can direct surrounding cells to produce a set of initials (Aida et al.2004,Jenik et al.2007).Different mechanisms specify the initials above the QC that give rise to the ground tissue and the stele(proximal initials)as well as the initials below the QC that produce the root cap(distal initials).This root cap and the underlying root apical meristem form the root zone called the distal root apex,where cells divide actively.At certain distance from the RAM,the anticlinal and asymmetric divisions of a group of pericycle cells are the initial event of the formation of a lateral root.Several consequent divisions result in the formation of a dome-shape primordium that grows through outer cell layers and a meristem is estab-lished.Upon activation of this meristem,the lateral root emerges from the parental root and continue to grow in the soil(Malamy1995and references therein).Phytohormonal regulation of the root system:Auxin as a major playerThe different stages of root development are con-trolled and regulated by various phytohormones with auxin playing a major role(reviewed by Leyser 2006).In roots,auxin is involved in lateral root formation,maintenance of apical dominance and adventitious root formation.All these developmental events require correct auxin transport and signaling.During embryonic RAM formation many auxin-related mutants,such as those involved in its biosynthesis yuc1yuc4yuc10yuc11quadruple mutant (Cheng et al.2007),or mutants affected in transport or signaling(monopteros,bodenlos,auxin transport inhibitor resistant1(tir1)and related tir1/afb1–3 quadruple mutant(Dharmasiri et al.2005,Jenik et al. 2007)are unable to form the embryonic RAM.The auxin flux coming from the apical region of the embryo into the hypophysis leads to TIR1(and related redundant AFBs)pathway activation and induction of auxin-response genes such as PIN genes (coding for auxin efflux carriers),whose products will increase auxin transport and accumulation to further differentiate the hypothesis(Benkova et al.2003).Auxin also plays a major role in lateral root initiation and teral root development can be divided in different steps:primordium initia-tion and development,emergence,and meristem activation.Auxin local accumulation in Arabidopsis root pericycle cells adjacent to xylem vessels,triggers lateral root initiation by re-specifying these cells into lateral root founder cells(Dubrovsky et al.2008). Furthermore,it is also involved in the growth and organization of lateral root primordia and emergence from the parent root(Laskowski et al.2006).Indeed, mutants or transgenic lines with elevated auxin biosynthesis and endogenous levels of IAA display significant increased root branching(Seo et al.1998, King et al.1995).In addition,overexpression of theDFL1/GH3-6or the IAMT1genes,which encode enzymes modulating free IAA levels,results in a reduction in lateral root formation(Staswick et al. 2005;Qin et al.2005).Hence,normal auxin biosyn-thesis and homeostasis are necessary for lateral root initiation in Arabidopsis.However,overall auxin content is not always positively correlated with lateral root number(Ivanchenko et al.2006).Auxin transport into the regions where lateral root initiate also seems crucial for the regulation of root branching(Casimiro et al.2001).Functional analyses of mutants impaired in auxin transport,such as the aux1,axr4,and pin multiple mutants(Benkova et al. 2003;Marchant et al.2002),have demonstrated the crucial role of auxin transport in lateral root forma-tion.In the root,auxin is transported in both an acropetal(base-to-apex;Ljung et al.2005)and basipetal(apex-to-base)direction within inner and outer tissues of the root apex(Swarup and Bennett 2003).Studies using auxin transport inhibitors have shown that the polarity of auxin movement provides an important developmental signal during lateral root initiation(Casimiro et al.2001)and root gravitropism (Parry et al.2001).The strong bias in the direction of auxin transport within a tissue results from the asymmetrical cellular localization of an influx system involving the AUX1protein(Swarup and Bennett 2003)and an efflux apparatus with PIN-type efflux facilitators(reviewed by Paponov et al.2005).The auxin efflux system regulated by PIN protein family members is also crucial for lateral root development(Benkova et al.2003).Although not all pin mutations affect lateral root formation,multiple pin mutants have dramatic defects in root patterning, including lateral root development(Benkova et al. 2003;Blilou et al.2005).Cellular localisation of PIN proteins is also important as a gnom mutant allele is defective in PIN-dependent developmental processes, including lateral root primordium development,pre-sumably due to disorganized PIN1localization (Geldner et al.2004).When auxin reaches the target tissue it induces a transcriptional response.Several auxin-responsive genes and gene families involved in auxin signalling have been identified,of which the Aux/IAAs and ARFs are the best studied.Auxin induces expression of Aux/IAA proteins,which in many cases reduces the sensitivity of cells toward auxin.This induction is mediated by ARF proteins that bind to the auxin-responsive elements(AuxREs)in the promoters of auxin-responsive genes,and activate or repress transcription through interaction with specific Aux/ IAA proteins(Liscum and Reed2002).These loops represent extensive feedback as well as feed-forward regulation(for further information on Aux/IAA pro-teins,their degradation and their interaction with ARF transcription factors see the review by Benjamins and Scheres2008).Alterations in these control systems affect several auxin-dependent plant pathways includ-ing root development.For example,normal differen-tiation of a root cap from the distal initials depends on a pair of redundantly acting auxin response factors, ARF10and ARF16(Wang et al.2005).In double mutants,the root cap initials overproliferate,thereby generating a mass of undifferentiated progeny. Another example are the gain-of-function solitary root(slr)mutants which carries a stabilizing mutation in the IAA14negative regulator of auxin signaling and cannot form lateral root primordia.Detailed analyses of the slr mutant demonstrated that the slr mutation blocks early cell divisions during initiation (Fukaki et al.2002)Other hormones involved in the control of root developmentAlthough auxin plays a fundamental role in root growth and development,several other phytorho-mones modulate auxin action and consequently affect root development and architecture.In contrast to auxin,exogenous cytokinin application suppresses lateral root formation,and transgenic Arabidopsis plants with decreased cytokinin levels display in-creased root branching and enhanced primary root growth(Werner et al.2003).The notion that cytokinin negatively regulates root growth has also been verified by studies of cytokinin perception and signalling.For instance,double mutants for the redundant Arabidopsis cytokinin receptors AHK2 and AHK3display a faster growing primary root and greatly increased root branching(Riefler et al. 2006).To identify the stage of lateral root develop-ment sensitive to cytokinin,Laplaze et al.(2007) performed transactivation experiments that revealed that xylem-pole pericycle cells,but not lateral root primordial,are sensitive to cytokinin.Cytokinins perturb the expression of PIN genes in lateral root founder cells and prevent the formation of the auxingradient required to pattern the primordia.Recently cytokinin have also been shown to induce differenti-ation of cells situated between the root meristem and the elongation zone(Dello Ioio et al.2007),providing new insights on the molecular mechanisms involved in root meristem maintenance.The actively growing primary root of dicotyledon plants may exhibit apical dominance forcing their lateral roots to develop further away from the root tip (Lloret and Casero2002).Root apical dominance may be triggered by the root-cap-synthesized cytoki-nin and the balance between auxin and cytokinin regulates root gravitropism,a major determinant of root architecture(Aloni et al.2006).The control of lateral root initiation depends on the primary root vascular system which conveys spatial information as well as photosynthetic carbon to the newly formed primordial(Parizot et al.2008).Three types of vascular differentiation can be distinguished:(1) primary differentiation,which occurs in cells origi-nating from the primary vascular meristem,the procambium;(2)secondary differentiation,where the derivates originate from the vascular cambium; and(3)regenerative differentiation,in which the vascular elements re-differentiate from parenchyma cells at lateral root junctions.The vascular tissues are induced by polar auxin movement along the plant body,from the hydathodes of young leaves downward to the root tips.Cytokinin by itself does not induce vascular tissues,but in the presence of auxin,it promotes vascular differentiation and regeneration,a critical process for the establishment of a root system (Aloni et al.2006).Another hormone involved in lateral root for-mation is ethylene.Treatments that induce C2H4 production(i.e.flooding)promote adventitious and lateral root formation(Mergemann and Sauter2000). Ethylene stimulates auxin biosynthesis and basipetal auxin transport toward the elongation zone,where it activates a local auxin response leading to inhibition of cell elongation.Stepanova et al.(2005)showed that ethylene-triggered inhibition of root growth is mediated by the action of the weak ethylene insensitive2/anthranilate synthaseα1(WEI2/ASA1) and wei7/anthranilate synthaseß1(ASB1)genes that encode rate-limiting tryptophan biosynthesis enzymes In addition,ethylene modulates the tran-scription of several components of the auxin trans-port machinery and induces a local activation of the auxin signaling pathway to regulate root growth (Ruzicka et al.2007).Abscisic acid(ABA),a universal stress hor-mone,plays a significant role in stimulating elongation of the main root and emergence of lateral roots in response to drought(De Smet et al. 2006b).As with other hormones,the complexity of the plant responses induced by this hormone makes it difficult to distinguish primary from secondary effects(e.g.stomata closure affects water movement in the plant).In general,ABA has an antagonistic effect on lateral root primordial formation and emergence to auxin,which initiates lateral roots (Malamy2005).This role of ABA in the control of the activity of the lateral root meristems has a significant impact on the final size and architecture of the root system(De Smet et al.2006b).Indeed, responsiveness to certain abiotic factors is lost in certain ABA signaling mutants(Signora et al.2001; He et al.2005;Fujii et al.2007)such as the systemic inhibition of lateral root formation in high N conditions in the ABA-insensitive mutant abi4 (Signora et al.2001).Genetic and genomic approaches to analyze root growth and architectureForward and reverse genetic approaches have been undertaken to elucidate the genetic control of lateral root formation.As expected,most of the lateral root mutants(approximately40%)identified in A.thaliana are affected in a specific component of the auxin pathways.Their phenotypes can usually be rescued or mimicked through auxin application.Several mutants having lateral root phenotypes(De Smet et al.2006a) have been linked to auxin signaling(axr mutants,tir1, msg2-1,shy2),auxin transport(aux1and pin mutants),and auxin homeostasis(alf1,ydk1,dfl1). Analysis of these mutants has led to the identification of several genes that regulate lateral root formation and/or coordinate this process in response to environ-mental cues(reviewed in Osmont et al.2007).Few Arabidopsis mutants such as the previously men-tioned slr-1/AUXIAA14(Fukaki et al.2002)and alf4-1(Celenza et al.1995),are not capable of initiating any lateral roots.The encoded ALF4protein is conserved among plants and has no similarities to proteins from other kingdoms.The Arabidopsis gene is involved in lateral root initiation but not in primaryroot formation as the alf4-1mutant forms a primary root.DiDonato et al.(2004)have shown that ALF4 functions are required to maintain the pericycle in a mitosis-competent state needed for lateral root forma-tion.Other mutants are affected in vascular tissues and consequently cannot produce lateral roots(e.g. Ohashi-Ito and Bergmann2007).In contrast,ectopic expression of PLT genes, coding for AP2-type transcription factors,trigger de novo formation of roots from shoot structures.PLT1 was isolated by reverse genetics technologies based on the identification of a QC–and stem-cell–specific enhancer trap line.Indeed,PLT genes are pivotal determinants of QC and stem cell identity(Aida et al.2004).Although plt1mutants only display mild root growth defects,the establishment and mainte-nance of the QC and stem cells in the root meristem, are severely impaired in the plt1plt2double mutant. NAC1is another transcription factor implicated in lateral root development since its overexpression enhances lateral root initiation(Xie et al.2002).A genetic framework for the control of cell division and differentiation in root meristematic cells have been recently proposed(Dello Ioio et al.2008).A second strategy used to identify non-essential modulators of root development,is exploiting natural genetic variation by analysis of quantitative trait loci(QTL).QTL mapping has the advantage of identifying genomic regions containing genes with subtle effects masked in a particular genetic background.For instance,in A.thaliana and maize QTLs linked to root architecture have been identified (Mouchel et al.2004;Tuberosa et al.2002;Loudet et al.2005).Exploring the polymorphisms underlying natural variation can identify the DNA sequence changes that lead to a modified root system architec-ture in nature.In recent years,several new genomics resources and tools(e.g.genome sequences,tens of thousands of molecular markers,microarrays,and knock-out collections)have become available to assist in QTL mapping and cloning,even if these genes have subtle effects on phenotype(see Paran and Zamir2003).For example,quantitative morpholog-ical and physiological variation analysis of a cross between isogenized Arabidopsis accessions revealed that the BREVIS RADIX(BRX)transcription factor controls the extent of cell proliferation and elonga-tion in the growth zone of the root tip(Mouchel et al. 2004).Genomics have provided new tools to explore downstream and upstream genes of broad regulatory signals such as hormone-responsive factors.Tran-scriptional changes during root branching in Arabi-dopsis have been monitored to identify key regulators of root architecture(Himanen et al.2004),including several core cell cycle genes.In addition,Vanneste et al.(2005)compared the early transcriptome of lateral root formation using synchronised induction of these organs in wild type and slr mutants.This revealed negative and positive feedback mechanisms that regulate auxin homeostasis and signalling in the pericyle initial cells.The advent of genomic resources have allowed the combination of global profiling with novel biological approaches such as enhancer trap lines driving green fluorescent protein(GFP)expression in specific root cell types(Brady et al.2007).These authors used transgenic lines that expressed GFP in specific root tissues to isolate corresponding fluores-cent protoplast populations derived from them and characterise their expression patterns using micro-arrays.Thus were able to determine which genes were expressed and at what level in a particular root cell type.Recently,these transgenic plants were used to characterise the transcriptional response to high salinity of different cell layers and developmental stages of the Arabidopsis root(Dinneny et al.2008). Transcriptional responses are highly constrained by developmental parameters further revealing interac-tions between developmental fates and environmental stresses.Genomic approaches mainly rely on the expression patterns of protein-coding genes.However,the dis-covery of small RNAs(microRNAs,miRNA;and small interfering RNAs,siRNA)in the last decade altered the paradigm that protein coding genes are the only significant components of gene regulatory net-works(Chapman and Carrington2007).Small RNAs are involved in a variety of phenomena that are essential for genome stability,establishment or main-tenance of organ identity,adaptive responses to biotic and abiotic stresses.In plants,MIRNAs are genes generally encoded in intergenic regions whose matu-ration requires a particular type III RNase named DICER-LIKE1(DCL1)(Chapman and Carrington 2007).The small mature miRNA is then incorporated in a protein complex,the so-called RISC(RNA-Induced Silencing Complex)that can recognizemRNAs partially complementary to the miRNA nucleotide sequence.This recognition event mediated by the RISC-loaded miRNA leads to cleavage or translational inhibition of the target mRNA.Mutants affected in miRNA metabolism(biosynthesis,action and transport as dcl1,ago1,hen1,hyl1,hst1,se)show pleiotropic phenotypes confirming the role of miR-NAs in diverse developmental processes(Chapman and Carrington2007).Several MIRNAs have been involved in root development.For example,Arabidopsis mir164a and mir164b mutant plants produced more lateral roots than wild-type plants(Guo et al.2005).These phenotypes are similar to those obtained by NAC1 overexpression,a transcription factor targetted by miR164(Xie et al.2002).Overexpression of miRNA-resistant NAC1mRNA results in slight increases in lateral root numbers.In addition,the auxin-related transcription factors ARF10and ARF16 are also targets of three related microRNA(miRNA) genes,miR160a,miR160b and miR160c.These miR-NAs limit the expression domain of ARF10and ARF16 to the columella,and have a complementary pattern. The over-expression of a miRNA-resistant ARF16 mRNA under its own promoter results in pleiotropic developmental defects.MIR160overexpressing plants, in which the expression of ARF10and ARF16is repressed,and the arf10-2arf16-2double mutants display the same root tip defect,with uncontrolled cell division and blocked cell differentiation in the root distal region showing a tumor-like root apex and loss of gravity-sensing(Wang et al.2005).Apart from these examples,due to the large diversity of these novel regulatory RNAs,it is likely that many other miRNAs or other small RNAs participate in the regulation of root development and architecture.Root meristematic cells integrate signals from the environment to regulate specific developmental responses and cope with external constraints.This post-embryonic growth and development requires the activation of hormone homeostasis and signalling pathways,transcriptional regulation by specific tran-scription factors and post-transcriptional regulation of developmental regulators by non-coding RNAs. These regulatory mechanisms may be particularly relevant to adjust differentiation processes to the environmental conditions encountered during growth, notably on primary and lateral root developmental programs.Root system achitectureRoot systems and architecture definitionsSoil is a complex medium with high spatial and temporal environmental variability at a wide range of scales,including those relevant to plant roots.The root system,with its extensive but structured devel-opment,can be considered as an evolutionary response to such spatio-temporal variability in re-source supply and associated constraints upon growth (Harper et al.1991).As a consequence,the extension in space and time of the root system is governed by genetically driven developmental rules which are modulated by environmental conditions.As demon-strated by QTL mapping which recently revealed that root morphology is in most cases regulated by a suite of small-effect loci that interact with the environment (de Dorlodot et al.2007).The architecture of a root system is the result of developmental processes and is a dynamic notion. Root architecture addresses two important concepts: the shape of the root system and its structure.The shape defines the location of roots in space and the way the root system occupies the soil.Its quantifica-tion is generally achieved by measuring variables such as root depth,lateral root expansion and root length densities.In contrast,root structure describes the variety of the components constituting the root system(roots and root segments)and their relation-ship(e.g.topology:connexion between roots;root gradients).While,root differentiation has important impacts upon structure—function relations(Clarkson 1996).The rhizosphere(i.e.the volume of soil around living plant roots that is influenced by root activity; Hinsinger et al.2005),is often simply thought of as a cylindrical shape around the root.However,this oversimplification does not account for integration at the root system level or for the inherent complexity of root systems which arise from geometry,temporal dynamics and the heterogeneous aspects of roots. These complexities are incorporated into the concept of root architecture.Root geometry is complex because of the specific motion in space of each root, the relative locations between roots and the possible overlapping of their zones of influence.The temporal dynamic comes both from the growth of the different root axes and from physiological processes associatedwith root segments (i.e.tissue differentiation)result-ing in temporal and spatial variability of function along the root axes.The diversity among roots within the root system and soil heterogeneity further increase this variability.Root classification and elaboration of the root system It has long been recognized that the in situ morphol-ogy of a root system can be complex and may vary greatly,even within a species (Weaver 1926;Cannon 1949;Kutschera 1960),reflecting the interplay be-tween developmental processes and environmental constraints (Fig.1).Consequently,the complexity of root systems has led to a number of different classification systems.These classifications can be based on:branch structure (topology)(Fitter 1987;2002),root activity (Wahid 2000)or development (Cannon 1949).The latter is the more classical approach and is useful in understanding growth as well as obtaining a more global view of root architecture,for example,in relation to plant habitat.Thus,developmental classification has been widely used in modelling approaches to simulate architecture (cf section below).From a developmental stand point roots are classified according to their ontogenesis into three main categories:primary,nodal and lateral roots(Cannon 1949;Harper et al.1991;Klepper 1992),the formation of which has been shown to be genetically separable (Hochholdinger et al.2004).This classification also reflects differences between monocot –and dicotyledon species:dicots root system is derived from primary roots and lateral branching (primary root system),with roots that may exhibit radial growth.Depending on the extension of laterals relative to the primary axis,the morphology of the root system will vary between taprooted and diffuse or fasciculate (Fig.1).In monocots,root systems derive not only from branching of primary roots but also from emmited nodal roots (adventitious root system).Monocot roots do not undergo secondary radial growth.The primary root differentiates from the seed ’s radicle already present in the seed embryo.This generally gives rise to a single-axis root system,or taproot system,with dominant vertical growth (gravitropism).Adventitious (or nodal)roots differ-entiate from organs other than roots (e.g.rhizomes,stems etc)and are initiated at precise locations (near stem nodes for example)with a defined temporal pattern,in coordination with the development of the shoot.They are often abundant and give rise to a fasciculated root system.Adventitious roots are much less sensitive to gravitropism than primary roots (Klepper 1992).Lateral roots originate from the branching of a parent axis,often at near rightangles,Fig.1Diversity of root systems.On the top row,root systems are little branched,while more profusely branched on the bottom row.Dominance of a single main axis increases from left to right (from Kutschera 1960)。
ApproachtoRadiculopathyPennStateUniversity方法对神经根型颈椎病宾夕法尼亚州立大学

Reflexes:
• C5-C6 Biceps • C5-C6 Brachioradialis • C7 Triceps • L3-L4
Quadriceps/patellar • S1 Ankle
60s—no ankle jerks—could be normal if on both sides, but if only on one side With pertinent symptoms on that side--significant
• Inflammation is important as a pain mechanism:
– Phospholipase A and E, NO, TNF, other pro-inflammatory mediators are released by a herniated disk
– The dura surrounding the ventral and dorsal nerve root is bathed in this exudate
– Inflammation or prior injury to nerve root is necessary to cause compression to generate continued pain
dura
Radicular pain:
• Lancinating or electric quality
pain and by associated clinical symptoms and signs 2) Describe the anatomy of the ventral and dorsal nerve roots, the location of
蒙脱石层间阳离子对其结构与性质影响的密度泛函理论研究

蒙脱石层间阳离子对其结构与性质影响的密度泛函理论研究李慎敏;张晶晶;王洪磊;尹静梅【摘要】采用密度泛函理论研究层间碱金属(Li+,Na+,K+)和碱土金属(Mg2+,Ca2+,Ba2+)阳离子变化对蒙脱石晶体结构、电学性质和光学性质的影响.研究发现:这种影响不仅与层间阳离子的大小有关,还受层间阳离子价态的影响.层间阳离子的引入对蒙脱石的晶格角度影响较小,而对蒙脱石的晶格矢量c影响较大.随着层间阳离子半径增大,蒙脱石层间距随之增大;对于同价态的层间阳离子,随着层间阳离子半径增大,蒙脱石的四面体层变厚、而八面体层变薄.此外,层间阳离子对于蒙脱石电学性质和光学性质的影响主要由其价态决定,当层间阳离子价态越高时,蒙脱石的绝缘性越差,同时其对可见光越敏感.【期刊名称】《辽宁师范大学学报(自然科学版)》【年(卷),期】2018(041)002【总页数】8页(P207-214)【关键词】密度泛函理论;蒙脱石;层间阳离子;电学性质;光学性质【作者】李慎敏;张晶晶;王洪磊;尹静梅【作者单位】大连大学辽宁省生物有机化学重点实验室,辽宁大连 116622;大连大学辽宁省生物有机化学重点实验室,辽宁大连 116622;大连大学辽宁省生物有机化学重点实验室,辽宁大连 116622;大连大学辽宁省生物有机化学重点实验室,辽宁大连 116622【正文语种】中文【中图分类】O641.121膨润土在我国分布广,材料加工成本低,应用广泛.膨润土的主要成分是蒙脱石(MMT),蒙脱石属于蒙脱石-皂石族中的一员,具有四面体(Si—O)-八面体(Al—O)-四面体(Si—O)的(t-o-t)结构,也就是说,蒙脱石是由铝硅酸盐层堆积而形成的黏土[1].由于蒙脱石框架中的阳离子存在同晶置换(主要由Mg2+置换Al3+),每一层都有一定的净负电荷,因此,金属阳离子作为可交换的层间阳离子,存在于蒙脱石中,用于中和净负电荷.通常这些可交换的层间阳离子主要是碱金属和碱土金属中以及个别副族金属离子如Fe3+.不同种类的同晶置换和交换阳离子使蒙脱石具有不同的性质[2],例如,钠基蒙脱石对硝基苯等污染物有良好的过滤作用[3],钙基蒙脱石是处理废水中的钴和铬效果良好的吸附剂[4],铜基蒙脱石可以作为催化剂以减少空气中的污染物NO和CO[5].揭示蒙脱石层间阳离子变化对其结构及性质影响的原因,探索改性蒙脱石在新材料方面更广泛的应用,引起了许多科学家的兴趣.近年来,实验与理论计算方法对含有不同层间阳离子的蒙脱石进行了研究[6-12],这些研究集中于考察层间阳离子尺寸大小对于蒙脱石的晶面间距等结构性质的影响,很少考虑层间阳离子价态的变化对于蒙脱石结构的影响.Wungu等[2,13]利用密度泛函理论研究了锂基、钙基蒙脱石的导电性能;Pirillo等[14]通过色散校正的密度泛函理论研究了钠基蒙脱石的电学、磁学特性.然而,系统研究蒙脱石电学性质的比较少,特别是对于蒙脱石光学性质研究的更是稀少.邹云玲等[15]对纳米CeO2粉体发光性能的研究和蔡河山等[16]对纳米TiO2光催化剂的研究对蒙脱石光学性质的研究具有指导作用.尝试采用密度泛函理论方法研究脱水蒙脱石晶体层间阳离子与晶体层相互作用如何影响蒙脱石结构变形,以及层间阳离子尺寸与价态对晶体电学性质和光学性质的影响.1 计算模型与方法由于X射线数据库中可用的蒙脱石晶体结构数据非常少,特别是准确的原子位置难以得到,因此,我们从叶蜡石结构出发获得蒙脱石的晶胞结构模型[2,17-23].蒙脱石和叶蜡石(pyrophyllite)都属于蒙脱石-皂石族,蒙脱石除了存在同晶取代外,其结构与叶蜡石一样,具有相同的铝硅酸盐层.叶蜡石的1×1×1单胞结构是在X射线粉末衍射分析[24]确定的数据基础上,对其优化得到的.为了得到合理的优化构象,优化分为晶胞原子和晶格参数2步进行.将优化后的叶蜡石结构中的Al3+用Mg2+离子取代,再优化获得了MMT的1×1×1的晶胞.为了考察层间阳离子对结构的影响,将不同的未水合化金属阳离子M(M:Li+,Na+,K+,Mg2+,Ca2+,Ba2+)添加到晶胞层间隙中以获得电中性的晶体结构(图1).图1 含有层间阳离子(M)的蒙脱石结构图1 dT, dO,dl,d001 分别对应四面体层厚度、八面体层厚度、层间距和001面晶面距离;阳离子M:Li+,Na+,K+,Mg2+,Ca2+,Ba2+Fig.1 Montmorillonite structure with interlayer cations(M)dT,dO,dl,d001 is the thickness of tetrahedral sheet,octahedral layer,the distance of interplanar spacing and the distance of 001 plane,respectively; the cation M:Li+,Na+,K+,Mg2+,Ca2+,Ba2+本文中,所有结构优化以及电学性质和光学性质计算均是采用维也纳从头算模拟软件包VASP完成的.计算中,交换相关函数选用广义梯度近似(GGA)的Perdew-Burke-Ernzerhof(PBE)[25]泛函,布里渊区k点取样网格为5×3×3,由Monkhorst-Pack[26]方法确定;体系总能量收敛阈值设置为5×10-5eV,最大原子力为0.1 eV/nm.2 结果与讨论2.1 蒙脱石的晶体结构分析表1给出了蒙脱石和含有6种层间阳离子蒙脱石(M-MMT,M:Li+,Na+,K+,Mg2+,Ca2+,Ba2+)的晶胞参数及阳离子半径r,优化得到的晶格矢量a、b、c 与实验测量值[27-28]较好的一致,表明所选取计算方法的可靠性.从表1可以看出,层间阳离子的加入对MMT晶格矢量a、b影响较小,但对晶格矢量c(这里c 的长度为晶面间距d001)影响较大;层间阳离子的加入,导致晶格矢量c的长度不同程度地增大,对于同价态的阳离子,离子半径越大,晶格矢量c增大越明显;而对于同周期的阳离子(Na+,Mg2+和K+,Ca2+),尽管1价层间阳离子半径明显大于2价层间阳离子半径,但是晶格矢量c相差不大,这是因为1价阳离子具有范德华较大排斥作用,2价阳离子静电吸引作用较大,两者相互作用抵消的结果.层间阳离子的加入也引起了晶格角β的变化,但是β变化不大,表明MMT的层状硅铝酸盐发生了微小的侧向位移.表1 蒙脱石以及含层间阳离子的蒙脱石晶格常数Table 1 Lattice parameters of MMT and M-MMT晶体类型a/nmb/nmc/nmα/(°)β/(°)γ/(°)r/nmMMT计算值0.5260.9091.02690.00101.3990.00Li-MMT计算值0.5230.9091.02890.00102.8790.000.076Na-MMT实验值[27]0.5170.8960.97490.0096.1090.00计算值0.5250.9041.03390.00102.1690.000.102K-MMT实验值[28]0.5190.9061.01090.0099.7090.00计算值0.5230.9041.04290.00101.8090.000.138Mg-MMT实验值[28]0.5140.8981.00490.00100.9090.00计算值0.5240.9111.02790.00102.2290.000.072Ca-MMT计算值0.5210.9011.04090.00102.2090.000.100Ba-MMT实验值[28]0.5200.9021.00290.00102.3090.00计算值0.5200.9061.07090.00102.1690.000.135r:离子半径为了进一步考察层间阳离子对蒙脱石晶面间距的影响,把晶面间距变化贡献划归为3部分,即Si—O四面体层(dT)、Al—O八面体层(dO)以及蒙脱石层间距(d1)的变化贡献,见图2.图2 层间阳离子半径与MMT和M-MMT结构性质关系Fig.2 Function of the interlayer cation radius and the structural of MMT and M-MMT图2(a)~图2(c)分别为蒙脱石和含层间阳离子晶体结构中四面体层厚度、八面体层厚度及层间距,图中虚线代表了纯净蒙脱石相应值.可以看出,3种贡献中,层间距的变化对晶面间距的贡献最大,也就是说,层间阳离子所引起的晶面间距的变化主要来自于层隙宽度的变化,层间距(dl)随层间阳离子半径增大呈二次函数增大.同价态的层间阳离子的离子半径越大,四面体层越厚,八面体层越薄,这与Li等[11]的研究结果是一致的.值得注意的是,半径较小的层间阳离子的加入,导致四面体层厚度小于纯净蒙脱石的四面体层厚度,而八面体层厚度却大于纯净蒙脱石的八面体层厚度.将MMT的层间距与M-MMT(M:Li+,Na+,K+,Mg2+,Ca2+,Ba2+)的层间距进行比较,发现dl的增加远远小于离子直径,这表明层间阳离子和晶体层之间的静电吸引力将相邻层更紧密地结合在一起.此外,较小半径的层间阳离子(Li+,Mg2+)的插入甚至使得dl减小而不是增加,表明如果层间阳离子的半径足够小,层间阳离子可能会进入四面体层形成的空腔中.2.2 蒙脱石的电学性质分析图3(a)~图3(g)分别是纯净的MMT、Li-MMT、Na-MMT、K-MMT、Mg-MMT、Ca-MMT、Ba-MMT的态密度(DOS)图,费米能级设为0.图3 MMT和M-MMT的总电子密度图Fig.3 Total density of states of MMT and M-MMT图3显示MMT、Li-MMT、Na-MMT、K-MMT体系在5 eV附近没有DOS跨越,而Mg-MMT、Ca-MMT、Ba-MMT体系在-5 eV附近没有DOS跨越,表明这些体系的Femi能级均具有较大能带间隙,相应的,计算得到的MMT、Li-MMT、Na-MMT、K-MMT、Mg-MMT、Ca-MMT、Ba-MMT的HOMO-LUMO能级差,即带隙宽度,分别是5.360、4.910、4.865、4.955、3.403、4.520、4.639 eV,其中,与He等人[29]和Wungu等人[2]计算得到MMT(5.35 eV)、Li-MMT(5.29 eV)的带隙宽度是一致的.比较MMT和M-MMT(M:Li+,Na+,K+,Mg2+,Ca2+,Ba2+)的带隙宽度差值0.45、0.495、0.405、1.957、0.84、0.721 eV,可以看出,引入层间阳离子使得蒙脱石的能隙变小,绝缘性减弱,其中,含有1价层间阳离子的蒙脱石带隙宽度减小值在0.5 eV以内,说明M-MMT(Li+,Na+,K+)依然保持较好的绝缘性;但含有2价层间阳离子的蒙脱石中,Mg-MMT带隙宽度改变最大,仅为3.403 eV,表明Mg-MMT可能具有半导体性质.如图3(b)~图3(g)所示,M-MMT(M:Li+,Na+,K+,Mg2+,Ca2+,Ba2+)体系DOS图中自旋向上和自旋向下的态密度是对称的,其对称性并没有因为层间阳离子的加入而改变,这意味着M-MMT没有磁偶极子.由于MMT和M-MMT 具有较大的带隙宽度,又缺少磁偶极子,因此,MMT和M-MMT都表现为绝缘性,与Wungu等人[2]和Pirillo等[14]计算锂基蒙脱石和钠基蒙脱石所得到的结论是一致的.2.3 蒙脱石的光学性质分析蒙脱石的光学性质可以根据其介电函数的虚部分析得到.图4(a)~图4(g)分别为MMT和M-MMT(M:Li+,Na+,K+,Mg2+,Ca2+,Ba2+)体系介电函数的虚部图.图4 MMT和M-MMT的介电函数的虚部图Fig.4 The imaginary part ofdielectirc function of MMT and M-MMT图4(续)需要说明的是,图中曲线开始攀升的起点能量值对应着体系吸收光子的能量阈值.如图所示,纯净蒙脱石的介电函数虚部曲线开始攀升的初值为6.090 eV,而M-MMT(M:Li+,Na+,K+,Mg2+,Ca2+,Ba2+)体系分别在5.702、5.643、5.708、0.761、0.079、0.070 eV.也就是说,层间阳离子的加入使得体系吸收光子的能量阈值降低,导致体系中的电子更容易发生跃迁.纯净的蒙脱石开始吸收光的波长为203 nm,而Li-MMT、Na-MMT、K-MMT吸收波长初值分别是218、220、218 nm,说明Li+、Na+、K+离子的加入使体系吸收光发生红移,位于可见光吸收范围之外的紫外区,故Li-MMT、Na-MMT、K-MMT与MMT一样,在可见光的照射下不发生光的吸收.但Mg-MMT、Ca-MMT、Ba-MMT体系吸收光的能量阈值在1.63~3.11 eV间,且对光有较强的吸收,暗示Mg2+、Ca2+、Ba2+离子的加入将会使体系的可见光光敏性增强,提升光催化活性.3 结论利用密度泛函理论计算方法,讨论了6种不同的金属阳离子嵌入在蒙脱石夹层时,层间阳离子对蒙脱石结构及其电子结构和光学性质的影响.研究表明:层间阳离子的离子半径、价态变化会对蒙脱石结构产生不同的影响,对于本文所讨论的碱金属(Li+,Na+,K+)离子和碱土金属(Mg2+,Ca2+,Ba2+)离子情况,同价态层间阳离子,半径越大,蒙脱石晶面层间距则越大,同时四面体层变厚、八面体层变薄;而同周期层间阳离子,不同价态的蒙脱石晶面层间距差异不大;分析导致上述变化的原因,认为对于同价态层间离子加入情况,离子与MMT的范德华作用占主导作用,蒙脱石晶面层间距由离子半径大小决定;而对于同周期不同价态层间离子加入情况,离子与MMT的范德华作用与库伦作用共同决定了蒙脱石晶面层间距的变化.此外,碱金属蒙脱石导电性能的影响比碱土金属蒙脱石小,即碱金属蒙脱石的绝缘性大于碱土金属蒙脱石.Li+、Na+、K+离子的加入使体系对光的吸收发生红移,但是不能吸收可见光;Mg2+、Ca2+、Ba2+离子的加入使得体系对可见光比较敏感,光催化活性比较高.参考文献:[1] AKALIN E,AKYUZ S,AKYUZ T.Adsorption and interaction of 5-fluorouracil with montmorillonite and saponite by FT-IRspectroscopy[J].Journal of Molecular Structure,2007,834:477-481.[2] WUNGU T D K,ASPERA S M,DAVID MY,et al.Absorption of lithium in montmorillonite:a density functional theory(DFT) study[J].Journal of Nanoscience and Nanotechnology,2011,11(4):2793-2801.[3] GORB L,GU J,LESZCZYNSKA D,et al.The interaction of nitrobenzene with the hydrate basal surface of montmorillonite:An ab initio study[J].Physical Chemistry Chemical Physics,2002,2(1):5007-5012.[4] ADELEYE S A,RAUTIU R,WHITE D A,et al.Clay minerals as sorbents for nuclear reactor activation products[J].Journal of MaterialsScience,1995,30(3):583-586.[5] JURADOAND C B,VALDES EA.On the possible removal of nitrogen monoxide and carbon monoxide on copper ion-exchanged montmorillonite:A DFT study[J].International Journal of Quantum Chemistry,2010,108(10):1802-1809.[6] MULEWA W,TAGIR M,AMIN N A S.MMT-supported Ni/TiO2 nano-composite for low temperature ethanol steam reforming toward hydrogen production[J].Chemical Engineering Journal,2017,326(1):956-969.[7] XU C,GU F L,WUH.BiOCl-montmorillonite as a photo catalyst for highly efficient removal of rhodamine B and orange G:Importance of the acidity and dissolved oxygen[J].Applied Clay Science,2017,147(1):28-35.[8] GOURNIS D,LAPPAS A,KARAKASSIDES M A,et al.A neutron diffraction study of alkali cation migration in montmorillonites[J].Physics & Chemistry of Minerals,2008,35(1):49-58.[9] BERGHOUT A,TUNEGA D,ZAOUIA.Density functional theory(DFT) study of the hydration steps of Na+/Mg2+/Ca2+/Sr2+/Ba2+ exchanged montmorillonites[J].Clays & Clay Minerals,2010,58(2):174-187.[10] VOORA V K,AL-SAIDI W A,JORDAN K D.Density functional theory study of pyrophyllite and M-montmorillonites(M = Li,Na,K,Mg,and Ca):Role of dispersion interactions[J].Journal of Physical ChemistryA,2011,115(34):9695-9703.[11] LI H,KANG T,ZHANGB,et al.Influence of interlayer cations on structural properties of montmorillonites:A dispersion-corrected density functional theory study[J].Computational Materials Science,2016,117:33-39.[12] 庞艳华,丁永生,孙冰,等.十六烷基三甲基铵改性膨润土的制备与物化性能研究[J].辽宁师范大学学报(自然科学版),2008,31(3):322-325.[13] TRIATI D K W,FAUZAN M R A,WIDAYANI,et al.A density functional theory study of a calcium-montmorillonite:a first investigation for medicine application[J].Journal of Physics:ConferenceSeries,2016,739(1):1-5.[14] PIRILLO S,LUNA C R,LPEZCORRAL I,et al.Geometrical and electronic properties of hydrated sodium montmorillonite and tetracyclinemontmorillonite from DFT calculations[J].Journal of Physical ChemistryC,2015,119(28):16082-16088.[15] 邹云玲,李酽,郭英,等.纳米CeO2粉体发光性能的研究[J].辽宁师范大学学报(自然科学版),2009,32(2):212-214.[16] 蔡河山,刘国光,熊心美.纳米TiO2光催化剂的制备、表征及其光催化活性的研究进展[J].辽宁师范大学学报(自然科学版),2005,28(4):449-453.[17] WUNGU T D K,AGUSTA M K,SAPUTRO A G,et al.First principles calculation on the adsorption of water on lithium-montmorillonite(Li-MMT)[J].Journal of Physics Condensed Matter:An Institute of Physics Journal,2012,24(47):475-506.[18] MIGONO P,SODUPE M.Theoretical study of the adsorption of DNA bases on the acidic external surface of montmorillonite[J].Physical Chemistry Chemical Physics,2012,14(2):945- 954.[19] CHATTERJEE A,IWASAKI T,EBINA T,et al.Quantum chemical calculation on clay-water interface[J].Applied Surface Science,1997,121/122(2):167-170.[20] HACKETT E,MANIAS E,GIANNELIS puter simulation studies of PEO/layer silicate nano-composites[J].Chemistry ofMaterials,2000,12(8):2161-2167.[21] ICHIKAWA Y,KAWAMURA K,NAKANO M,et al.Seepage and consolidation of bentonite saturated with pure- or salt-water by the method of unified molecular dynamics and homogenizationanalysis[J].Engineering Geology,2001,60(1/2/3/4):127-138.[22] STACKHOUSE S,COVENEY P V.Study of thermally treated lithium montmorillonite by ab initio methods[J].Journal of Physical ChemistryB,2002,106(48):12470-12477.[23] YAN K F,LI X S,XU C G,et al.Molecular dynamics simulation of the intercalation behaviors of methane hydrate in montmorillonite[J].Journal of Molecular Modeling,2014,20(6):1-11.[24] WARDLE R,BRINDLEY G.The crystal structures of pyrophyllite,1Tc,and of its dehydroxylate[J].American Mineralogist,1972,57(5/6):732-750. [25] PERDEW J P,BURKE K,ERNZERHOF M.Generalized gradient approximation made simple[J].Physical Review Letters,1996,77(18):3865-3868.[23] HENDRIK J,MONKHORST,PACK J D.Special points for Brillouin-zone integrations[J].Physical Review B,1976,13(12):5188-5192.[27] GOURNIS D,LAPPAS A,KARAKASSIDES M,et al.A neutron diffraction study of alkali cation migration in montmorillonites[J].Physics & Chemistry of Minerals,2008,35(1):49-58.[28] BEERMANN T,BROCKAMP O.Structure analysis of montmorillonite crystallites by convergent-beam electron diffraction[J].ClayMinerals,2005,40(1):1-13.[29] HE M C,FANG Z J,ZHANG P.Atomic and electronic structures of montmorillonite in soft rock[J].Chinese Physics B,2009,18(7):2933-2937.。
- 1、下载文档前请自行甄别文档内容的完整性,平台不提供额外的编辑、内容补充、找答案等附加服务。
- 2、"仅部分预览"的文档,不可在线预览部分如存在完整性等问题,可反馈申请退款(可完整预览的文档不适用该条件!)。
- 3、如文档侵犯您的权益,请联系客服反馈,我们会尽快为您处理(人工客服工作时间:9:00-18:30)。
IntroductionMagnesium alloys are among the lightest metallic materials for structural applications.Their high-specific strength and rigidness makes them attractive for a broad range of technical applications,particularly in automotive and air-craft industries as well as in electronic devices[1].How-ever,Mg alloys have poor formability and limited ductility slip direction is still no way to accommodate strains along the c-axis because of the a-axis slip direction without a c-axis slip component.Therefore,the f1022gh1123i sec-ond-order pyramidal slip system is activated as the next easiest slip system in all hcp metals and has a c-slip component,which is a very important slip system in the deformation of hcp polycrystalline materials.It can provide five independent deformation modes[1,2].Therefore,any hcp crystal exhibiting pyramidal h c?a i slip fulfills the von Mises criterion for a general homogeneous plastic deformation,regardless of which other systems are present. The role of h c?a i slip in plastic deformation of hcp metals is important not only for fundamental understanding of dislocation mechanisms involved in a generalized plas-ticity of polycrystals,but also for alloy/process design strategies in the development of lightweight structural alloys.So it is important to investigate the activation conditions of h c?a i non-basal slip system.For the pur-pose,in this article the studies of the activation conditions of h c?a i non-basal slip system are reviewed and the influence of h c?a i non-basal slip system on the plasticity of Mg alloys.The effect of hydrostatic pressure on the activation of non-basal slip is emphasized.F.Kang(&)ÁP.ChengÁH.Y.WuDesign Department of Technology Center,Jinxi Axle Company Limited,Taiyuan030027,People’s Republic of Chinae-mail:fengkang@Z.LiÁJ.T.WangDepartment of Materials Science and Engineering,Nanjing University of Science and Technology,Nanjing210094, People’s Republic of ChinaThe activation conditions of h c1a i non-basal slip systemLithium element additions to MgNumerous reports have been made regarding the benefit of Li to improving the ductility of Mg[3–10].With above 5wt%Li,a duplex alloy containing the body centered cubic b-phase is obtained,and above11wt%Li the alloy is entirely b-phase[11].The b-phase is soft and ductile; hence,the high-Li alloys are ductile.The focus of the article is only on the hcp a-phase.In the early work of Hauser et al.[3]in1956,the alloys are completely ductility of Mg–Li solid solution a-phase alloys was connected with the general activation of a non-basal slip mode(namely prism h a i).Hauser et al.[3]cited a decrease axial ratio (c/a)of Mg as a function of Li content(from1.624to1.607 at the solid-solubility limit).This change is primarily associated with a decrease in the(0002)lattice spacing, rather than an increase in the{1010}planar spacing. Hence,it was argued that the Peierls stress for basal slip would increase relative to prism slip.The different view-points were occurred[10–13].Ando and Tonda[10]in 2000performed two surface-slip-trace analyses on single crystals of pure Mg and Mg–Li alloys strained in tension along a h1210i direction at room temperature and below.In this orientation,the Schmid factors for h c?a i slip on second-order pyramidal planes and for h a i slip on prismatic planes are similar(0.446and0.433,respectively)[10]. Hence,the fact that the slip-trace analyses indicated h c?a i slip,rather than prismatic h a i slip,is significant.In an hcp material with easy basal slip,if the critical resolved shear stress(CRSS)for h c?a i slip is lower than that of prismatic h a i slip,there is essentially no stress condition for which the latter slip system will be favored over the former.In addition,the work of Agnew et al.[13]showed the subtle differences in the mechanical behavior and the uniaxial compression textures of Mg and a-phase solid solution alloys containing Li.Texture simulation was used to interpret the distinctions in textures to be attributed to the increased activity of the non-basal h c?a i slip mode. Later,Agnew et al.[13]used transmission electron microscopy to investigating the dislocation structures in these alloys,their results further corroborated that Li additions to Mg enhance the activity of the pyramidal h c?a i slip mode.Theoretically,the most appealing configuration for understanding glissile h c?a i disloca-tions is a collinear dissociation into two h c?a i partial dislocations with an intervening stacking fault on the f11"2"2g glide plane.Li additions may lower this non-basal stacking fault energy and,thereby,increase the stability of this glissile configuration[13].TemperatureThe deformation behavior of Mg polycrystals is very sen-sitive to temperature.The work hardening with increasing temperature decreased strongly above about0.3T m(T m is the absolute melting point).Simultaneously,the ductility is increasing[14].The activity of the second-order pyramidal slip system also depends strongly on temperature.The critical resolved shear stress(CRSS)necessary for slip in this system at room temperature is about100times larger than that for basal slip[15].This value is decreasing with increasing temperature and above200°C the activation of dislocation motion in the pyramidal system is energetically more favorable than twinning.Ma´this et al.[14]estab-lished the fractions of the three Burgers vector types,h a i, h c i,and h c?a i,as a function of the deformation tem-perature through high resolution X-ray diffraction peak profile measurements and numerical procedures.For more details see equation in[16–18].It is clearly from Fig.1 given by Ma´this et al.[14]that at room temperature and at 100°C the dominant dislocation type is h a i or mainly basal in Mg.At higher temperatures the fraction of a decreases, whereas the fraction of h c?a i increases.The fraction of h c i remains practically unchanged.It can be seen that the average dislocation density increases considerably upon deformation as compared to the value in the as-cast state. However,with increasing deformation temperature the increment of dislocation density decreases strongly in accordance with dynamic recovery[14].Similarly,Tonda and Ando[2]found that the yield stress due to the second-order pyramidal slip system increased with increasing temperature in hcpmetals.Fig.1The h i fractions of the three Burgers vector types,h a i,h c i, and h c?a i,as a function of the deformation temperature.The error bars in thefigure are for the ranges of the Burgers vector types in the numerical procedure described in the literature.q is the average dislocation density[14]Grain refinementSeveral investigations show that poor ductility of Mg alloys at room temperature can be improved by grain refinement[19–24].Furui et al.[21]reported the tensile elongation of a two-phase Mg-8%Li alloy was*1,780% through grain refinement in the Cast?Extrude?ECAP condition,grain size reduces to*1–7l m after ECAP. Such large elongation values cannot be expected if the basal slip system is the sole mechanism of plastic defor-mation.Moreover,twinning becomes increasingly difficult with decreasing grain size[25].Thus,additional disloca-tion mechanisms are necessary.Micro-analyses show that the critical factor in the improvement of ductility is the activation of non-basal slip systems infine-grained Mg alloys-dependent compatibility stress at grain boundaries [26–28].The effects of compatibility stress have been investigated in cubic metals[29–32]and in hcp metals[26, 33].In cubic metals,elastic heterogeneity is very strong, and the second-order slip systems are activated by elastic compatibility stress[29,30].In contrast,elastic heteroge-neity is weak,but plastic heterogeneity is very obvious in hcp metals and may give rise to a large plastic compati-bility stress[26,33].The work of Koike et al.in Mg alloys [26]showed the improvement of ductility due to the activity of non-basal slip in terms of grain-size-dependent plastic compatibility stress at grain boundaries.And the schematicfigure of the activation of non-basal glide sys-tems was given by Koike et al.(see Fig.2)[26].To satisfy the compatibility effects among the grain boundaries,non-basal slip systems become active by additional shear stress (plastic compatibility stress)during deformation,as illu-minate by arrows in Fig.2c.In addition,Koike et al.[27, 28]studied the microscopic mechanisms of plastic defor-mation in polycrystalline Mg alloys.They considered that when the grain size\*100l m,the range of non-basal slipping is about10l m from grain boundaries.Hence, non-basal slip systems will run through the entire grain,if grain size reduces to*10l m,as illuminate in Fig.3[28]. It is clear that non-basal slip systems become active much easier in thefine grain boundaries.Hydrostatic pressureSeveral researches have shown that the plasticity and strength of materials could be improved under high-hydrostatic pressure[34–37].Moreover,it has been pro-posed that hydrostatic pressure might help to activate dif-ferent slip systems[38–40].Our recent work[41,42]is designed to present experimental evidence for probing if non-basal slip system in Mg alloy is activated under hydrostatic pressure.Applying backpressure during ECAP is used to produce hydrostatic pressure.Fig.2Schematic drawing of stressed bicrystals:a before deforma-tion,b deformed condition without bonding along a grain boundary, and c possible stress state in order to maintain contact between two grains along a grain boundary[26]Fig.3Schematic drawing of non-basal slip systems infine-grained and coarse-grained Mg alloys:in both of the two grains,coarse lines represent basal slip systems andfine lines represent non-basal slip systems[28]An extruded rod(/30mm)of commercial AZ31Mg alloy is cut into rods with a dimension of209209 100mm3along the extrusion direction for ECAP with and without backpressure(BP).The average grain size of the as-received AZ31alloys is estimated to be*28l m. ECAP,using a90°die with no outer curvature at the intersection of the two channels,is conducted at*200°C at a speed of0.2mm s-1,and a backpressure of125MPa is applied according to investigation schedule.Molybde-num disulphide(MoS2)is used as lubricant.For compari-son,some samples were processed under the same conditions without applying back pressure.Tensile speci-mens with a gauge section of2(thickness)93(width)9 10mm(length)were cut along the longitudinal direction of the ECAP processed bar.Tensile tests were conducted at room temperature with an initial strain rate of 5910-2s-1on a SANS-CMT5105testing machine using a10kN load cell.Six samples were tested for each dif-ferent processing condition.Optical microscopy was con-ducted using an Axiover40MAT microscope on specimens ground and polished mechanically before being etched for5s in a solution of5ml acetic acid,5g picric acid,10ml H2O,and100ml ethanol.Polefigures from longitudinal section of the samples are measured using X-ray diffractometer(model Philip X’pert PRD)with Co K a radiation operating at35kV and40mA.The TEM specimens cut from Y planes[41]arefinal thinned to perforation with a twin-jet in a solution of1%perchloric acid and99%ethanol,using a voltage75V and a current of10mA at-30°C.A JEOL-2100electron microscope operating at200kV is used to examine the foils.The results[41,42]show that the application of hydrostatic pressure in a single pass of ECAP leads to significant improvement in both strength and ductility in initially extruded AZ31bars with a strong base planefiber texture(see Fig.4);results in much enhanced grain refinement(see Fig.5).Moreover,dominant texture com-ponent in(0002)polefigure is split from one strong peak with an intensity of*15at*50°to sample axis in ECAP without backpressure,to two peaks with a much lower intensity of*5in ECAP with backpressure(see Fig.6). This experimental result corresponds well to the existing texture simulation results[43]supposing the contribution of h c?a i non-basal slip.TEM observations shows the prevailing of h c?a i dislocations in the sample ECAP processed with backpressure,and confirms the significanceof h c?a i non-basal slip(see Fig.7).This demonstrates that different deformation mechanism is activated by the application of hydrostatic pressure,which will help to open new window to understand the basic physics of plastic deformation under hydrostatic pressure.At the same time, another new question is brought forward,‘‘how did hydrostatic pressure activate h c?a i non-basal slip?’’A possibility is that hydrostatic pressure leads to the increasing of grain-boundary compatibility stresses,then the increased stresses compensate for the requirement stresses in activating h c?a i non-basal slip,and the yield anisotropy(the CRSS ratio of non-basal slip to basal slip) sharp decreases,so non-basal slip systems become active much easier under hydrostatic pressure.Moreover,theXZ50µmY(b)(a)Fig.5Microstructures in AZ31alloy after a single pass of a ECAP and b BP-ECAP[42]+Simulation20°7°25°25°(0002)and(1010)polefigures for BP-ECAP?Simulation.simulation results from[43]are rotated90°clockwise from the fit the present coordinate frame[41]thermal energy comes from sever plastic deformation mainly leads to the increasing temperature in local region,which is also help to make it much easier.These will be focuses of future work.ConclusionsThe activation of h c ?a i non-basal slip system can help to improving the mechanical properties of Mg alloys.The activation conditions of h c ?a i non-basal slip system in Mg alloys are reviewed,such as the addition of Li elements leads to decreases in the lattice axial ratio (c /a )of the alloy,or/and lower the (1122)stacking fault energy;increasing temperatures and regions of stress concentration to decreases in the critical resolved shear stresses of h c ?a i non-basal slip.It is more important that h c ?a i non-basal slip systems become active much easier under hydrostatic pressure,which will help to open new window to explore the basic physics of the activation of non-basal slip.References1.Stalmann A,Sebastian W,Friedrich H,Schumann S,Dro¨der K (2001)Adv Eng Mater 3:9692.Tonda H,Ando S (2002)Metall Trans A 33:8353.Hauser FE,Landon PR,Dorn JE (1956)Trans ASM 48:9864.Raynor GV (1959)The physical metallurgy of Magnesium and its alloys.Pergamon,New York,NY5.Roberts CS (1960)Magnesium and its alloys.Wiley,New York,NY6.Emley EF (1966)Principles of Magnesium metallurgy.Perg-amon,New York,NY7.Metenier P,Gonzalez-Doncel G,Ruano OA,Wolfenstine J,Sherby OD (1990)Mater Sci Eng A125:1958.Takuda H,Kikuchi S,Kubota K (2000)Magnesium alloys and their applications.Wiley-VCH,Frankfurt,p 2859.Saito N,Mabuchi M,Nakanishi M,Kubota K,Higashi K (1997)Scr Mater 36:55110.Ando S,Tonda H (2000)Mater Trans JIM 41:118811.Massalski TB (1986)Binary alloy phase diagrams.ASM,MetalsPark,OH,p 148712.Agnew SR,Yoo MH,Tome CN (2001)Acta Mater 49:427713.Agnew SR,Horton JA,Yoo MH (2002)Met Mater Trans A33:85114.Ma ´this K,Nyilas K,Axt A,Dragomir-Cernatescu I,Unga ´r T,Luka ´c ˇP (2004)Acta Mater 52:288915.Yoo MH (1981)Metall Trans A 12:40916.Dragomir IC,Ungar TJ (2002)Appl Cryst 35:55617.Gubicza J,Weber F (1999)Mat Sci Eng A 263:10118.Szepvolgyi J,Mohai I,Gubicza J (2001)J Mater Chem 11:85919.Del Valle JA,Carreno F,Ruano OA (2006)Acta Mater 54:424720.Kim WJ,An CW,Kim YS,Hong SI (2002)Scr Mater 47:3921.Furui M,Kitamura H,Anada H,Langdon TG (2007)Acta Mater55:108322.Kim WJ,Sa YK (2006)Scr Mater 54:139123.Kim WJ,Hong SI,Kim YS,Min SH,Jeong HT,Lee JD (2003)Acta Mater 51:329324.Kim HK,Kim WJ (2004)Mater Sci Eng A 385:30025.Meyers MA,Vohringer O,Lubarda VA (2001)Acta Mater49:402526.Koike J,Kobayashi T,Mukai T,Watanabe H,Suzuki M,Mar-uyama K,Higashi K (2003)Acta Mater 51:205527.Koike J (2003)Mater Sci Forum 189:41928.Koike J,Miyamura T (2004)J Jpn Inst Light Met 11:46029.Takasugi T,Izumi O (1978)Fat-Halla N.J Mater Sci 13:2013.doi:10.1007/BF0055290930.Sittner P,Paidar V (1989)Acta Metall 37:171731.Hook RE,Hirth JP (1967)Acta Metall 15:53532.Meyers MA,Ashworth E (1982)Phil Mag A 46:73733.Maruyama K,Akabori M,Karashima S (1981)Trans JIM 22:72334.Karman T (1911)Festigkeitsversuche unter allseitigem.Z Ve-reins Deutscher Ingenieure 55:174935.Bridgman PW (1923)Proc Am Acad Arts Sci 58:16336.Bridgman PW (1943)J Appl Phys 14:27337.Spitzig WA (1979)Acta Metall 27:52338.Kolpashnikov AI,Vyalov VA,Federov AA,Petrov AP (eds)(1977)Thermal hydraulic compression of metals,Mashinost-roenie,MoscowFig.7TEM bright field image from BP-ECAP sample,taken under two beam condition using diffraction vector g =0002(a ),g =1010(b ),and g =1011(c )[41]39.Wenk HR,Matthies S,Hemley RJ,Mao HK,Shu J(2000)Nature405:104440.Thurel E,Cordier P(2003)Phys Chem Minerals30:25641.Kang F,Liu JQ,Wang JT,Zhao X(2009)Scr Mater61:84442.Kang F,Liu JQ,Wang JT,Zhao X,Wu X,Xia K(2009)Int J MatRes100:168643.Agnew SR,Mehrotra P,Lillo TM,Stoica GM,Liaw PK(2005)Acta Mater53:3135。