土木工程类外文文献翻译.d
土木工程专业毕业设计外文文献及翻译
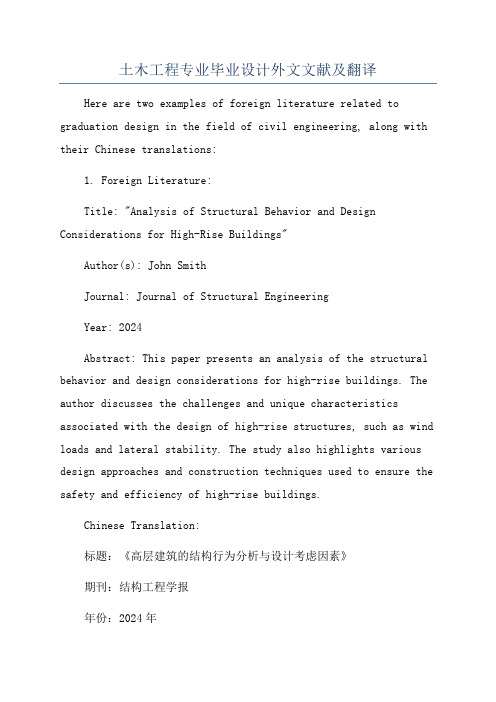
土木工程专业毕业设计外文文献及翻译Here are two examples of foreign literature related to graduation design in the field of civil engineering, along with their Chinese translations:1. Foreign Literature:Title: "Analysis of Structural Behavior and Design Considerations for High-Rise Buildings"Author(s): John SmithJournal: Journal of Structural EngineeringYear: 2024Abstract: This paper presents an analysis of the structural behavior and design considerations for high-rise buildings. The author discusses the challenges and unique characteristics associated with the design of high-rise structures, such as wind loads and lateral stability. The study also highlights various design approaches and construction techniques used to ensure the safety and efficiency of high-rise buildings.Chinese Translation:标题:《高层建筑的结构行为分析与设计考虑因素》期刊:结构工程学报年份:2024年2. Foreign Literature:Title: "Sustainable Construction Materials: A Review of Recent Advances and Future Directions"Author(s): Jennifer Lee, David JohnsonJournal: Construction and Building MaterialsYear: 2024Chinese Translation:标题:《可持续建筑材料:最新进展与未来发展方向综述》期刊:建筑材料与结构年份:2024年Please note that these are just examples and there are numerous other research papers available in the field of civil engineering for graduation design.。
土木工程英文文献及翻译-英语论文.doc
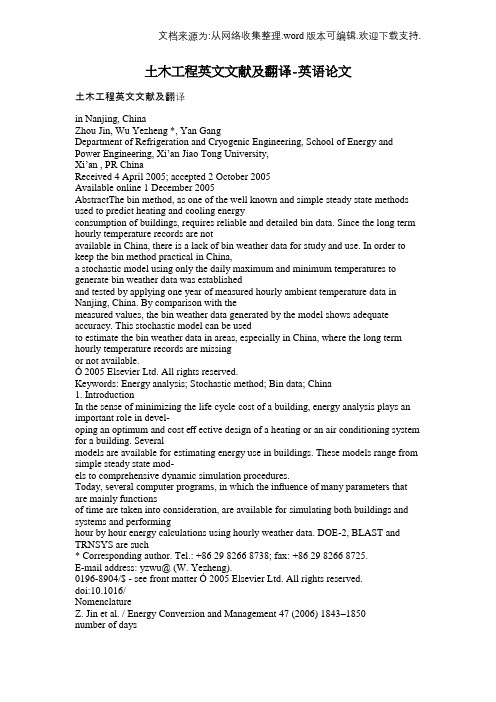
土木工程英文文献及翻译-英语论文土木工程英文文献及翻译in Nanjing, ChinaZhou Jin, Wu Yezheng *, Yan GangDepartment of Refrigeration and Cryogenic Engineering, School of Energy and Power Engineering, Xi’an Jiao Tong University,Xi’an , PR ChinaReceived 4 April 2005; accepted 2 October 2005Available online 1 December 2005AbstractThe bin method, as one of the well known and simple steady state methods used to predict heating and cooling energyconsumption of buildings, requires reliable and detailed bin data. Since the long term hourly temperature records are notavailable in China, there is a lack of bin weather data for study and use. In order to keep the bin method practical in China,a stochastic model using only the daily maximum and minimum temperatures to generate bin weather data was establishedand tested by applying one year of measured hourly ambient temperature data in Nanjing, China. By comparison with themeasured values, the bin weather data generated by the model shows adequate accuracy. This stochastic model can be usedto estimate the bin weather data in areas, especially in China, where the long term hourly temperature records are missingor not available.Ó 2005 Elsevier Ltd. All rights reserved.Keywords: Energy analysis; Stochastic method; Bin data; China1. IntroductionIn the sense of minimizing the life cycle cost of a building, energy analysis plays an important role in devel-oping an optimum and cost effective design of a heating or an air conditioning system for a building. Severalmodels are available for estimating energy use in buildings. These models range from simple steady state mod-els to comprehensive dynamic simulation procedures.Today, several computer programs, in which the influence of many parameters that are mainly functionsof time are taken into consideration, are available for simulating both buildings and systems and performinghour by hour energy calculations using hourly weather data. DOE-2, BLAST and TRNSYS are such* Corresponding author. Tel.: +86 29 8266 8738; fax: +86 29 8266 8725.E-mail address: yzwu@ (W. Yezheng).0196-8904/$ - see front matter Ó 2005 Elsevier Ltd. All rights reserved.doi:10.1016/NomenclatureZ. Jin et al. / Energy Conversion and Management 47 (2006) 1843–1850number of daysfrequency of normalized hourly ambient temperatureMAPE mean absolute percentage error (%)number of subintervals into which the interval [0, 1] was equally dividednumber of normalized temperatures that fall in subintervalprobability densityhourly ambient temperature (°C)normalized hourly ambient temperature (dimensionless)weighting factorSubscriptscalculated valuemeasured valuemax daily maximummin daily minimumprograms that have gained widespread acceptance as reliable estimation tools. Unfortunately, along withthe increased sophistication of these models, they have also become very complex and tedious touse [1].The steady state methods, which are also called single measure methods, require less data and provideadequate results for simple systems and applications. These methods are appropriate if the utilization ofthe building can be considered constant. Among these methods are the degree day and bin data methods.The degree-day methods are the best known and the simplest methods among the steady state models.Traditionally, the degree-day method is based on the assumption that on a long term average, the solarand internal gains will offset the heat loss when the mean daily outdoor temperature is 18.3 °C and thatthe energy consumption will be proportional to the difference between 18.3 °C and the mean daily tempera-ture. The degree-day method can estimate energy consumption very accurately if the building use and theefficiency of the HVAC equipment are sufficiently constant. However, for many applications, at least oneof the above parameters varies with time. For instance, the efficiency of a heat pump system and HVAC equip-ment may be affected directly or indirectly by outdoor temperature. In such cases, the bin method can yieldgood results for the annual energy consumption if different temperature intervals and time periods areevaluated separately. In the bin method, the energy consumption is calculated for several values of the outdoortemperature and multiplied by the number of hours in the temperature interval (bin) centered around thattemperature. Bin data is defined as the number of hours that the ambient temperature was in each of a setof equally sized intervals of ambient temperature.In the United States, the necessary bin weather data are available in the literature [2,3]. Some researchers[4–8] have developed bin weather data for other regions of the world. However, there is a lack of informationin the ASHRAE handbooks concerning the bin weather data required to perform energy calculations in build-ings in China. The practice of analysis of weather data for the design of HVAC systems and energy consump-tion predictions in China is quite new. For a long time, only the daily value of meteorological elements, such asdaily maximum, minimum and average temperature, was recorded and available in most meteorologicalobservations in China, but what was needed to obtain the bin weather data, such as temperature bin data,were the long term hourly values of air temperature. The study of bin weather data is very limited in China.Only a few attempts [9,10] in which bin weather data for several cities was given have been found in China.Obviously, this cannot meet the need for actual use and research. So, there is an urgent need for developing binweather data in China. The objective of this paper, therefore, is to study the hourly measured air temperaturedistribution and then to establish a model to generate bin weather data for the long term daily temperaturedata.2. Data usedZ. Jin et al. / Energy Conversion and Management 47 (2006) 1843–1850In this paper, to study the hourly ambient temperature variation and to establish and evaluate the model, aone year long hourly ambient temperature record for Nanjing in 2002 was used in the study. These data aretaken from the Climatological Center of Lukou Airport in Nanjing, which is located in the southeast of China(latitude 32.0°N, longitude 118.8°E, altitude 9 m).In addition, in order to create the bin weather data for Nanjing, typical weather year data was needed.Based on the long term meteorological data from 1961 to 1989 obtained from the China MeteorologicalAdministration, the typical weather year data for most cities in China has been studied in our former research[11] by means of the TMY (Typical Meteorological Year) method. The typical weather year for Nanjing isshown in Table 1. As only daily values of the meteorological elements were recorded and available in China,the data contained in the typical weather year data was also only daily values. In this study, the daily maxi-mum and minimum ambient temperature in the typical weather year data for Nanjing was used.3. Stochastic model to generate bin dataTraditionally, the generation of bin weather data needs long term hourly ambient temperature records.However, in the generation, the time information, namely the exact time that such a temperature occurredin a day, was omitted, and only the numerical value of the temperature was used. So, the value of each hourlyambient temperature can be treated as the independent random variable, and its distribution within the dailytemperature range can be analyzed by means of probability theory.3.1. Probability distribution of normalized hourly ambient temperatureSince the daily maximum and minimum temperatures and temperature range varied day by day, the con-cept of normalized hourly ambient temperature should be introduced to transform the hourly temperatures ineach day into a uniform scale. The new variable, normalized hourly ambient temperature is defined by^ ¼ttmintmaxtminwhere ^ may be termed the normalized hourly ambient temperature, tmaxand tminare the daily maximum andminimum temperatures, respectively, t is the hourly ambient temperature. Obviously, the normalized hourly ambient temperature ^ is a random variable that lies in the interval [0, 1].To analyze its distribution, the interval [0, 1] can be divided equally into several subintervals, and by means ofthe histogram method [12]iin each subinterval can be calculated by1137土木工程英文文献及翻译Based on the one year long hourly ambient temperature data in Nanjing, China, the probability density piwas calculated for the whole day and the 08:00–20:00 period, where the interval [0, 1] was equally divided into50 subintervals, namely n equals 50. The results are shown in Fig. 1.According to the discrete probability density data in Fig. 1, the probability density function of ^ can beobtained by a fitting method. In this study, the quadratic polynomialswere used to fit the probability density data, where a, b and c are coefficients. According to the property of theprobability density function, the following equation should be satisfiedAs shown in Fig. 1, the probability density curve obtained according to the probability density data pointsis also shown. The probability densit y functions that are fitted are described byp ¼ 2:7893^23:1228^ þ 1:6316 for the whole day periodp ¼ 2:2173^20:1827^ þ 0:3522 for the 08 : 00–20 : 00 period3.2. The generation of hourly ambient temperatureAs stated in the beginning of this paper, the objective of this study is to generate the hourly ambient tem-perature needed for bin weather data generation in the case that only the daily maximum and minimum tem-peratures are known. To do this, we can use the obtained probability density function to generate thenormalized hourly ambient temperature and then transform it to hourly temperature. This belongs to theproblem of how to simulate a random variable with a prescribed probability density function and can be doneon a computer by the method described in the literature [13]. For a given probability density function f ð^Þ, ifits distribution function F ð^Þ can be obtained and if u is a random variable with uniform distribution on [0, 1],thenF, we need only setAs stated above, the probability density function of the normalized ambient temperature was fitted using aone year long hourly temperature data. Based on the probability density function obtained, the random nor-malized hourly temperature can be generated. When the daily maximum and minimum temperature areknown, the normalized hourly temperature can be transformed to an actual temperature by the followingequationWhen the hourly temperature for a particular period of the day has been generated using the above method,the bin data can also be obtained. Because the normalized temperature generated using the model in this studyis a random variable, the bin data obtained from each generation shows somedifference, but it has much sim-ilarity. To obtain a stable result of bin data, the generation of the bin data can be performed enough times,and the bin data can be obtained by averaging the result of each generation. In this paper, 50 generations wereaveraged to generate the bin weather data.Z. Jin et al. / Energy Conversion and Management 47 (2006) 1843–18503.4. Methods of model evaluationThe performance of the model was evaluated in terms of the following statistical error test:土木工程英文文献及翻译一种产生bin气象数据的随机方法——中国南京周晋摘要:bin方法是一种众所周知且简捷的稳态的计算方法,可以用来预计建筑的冷热能耗。
土木工程 外文翻译 外文文献 英文翻译

Stress Limits in DesignHow large can we permit the stresses to be? Or conversely: How large must a part be to withstand a given set of loads what are the overall conditions or limits that will determine the size and material for a part?Design limits are based on avoiding failure of the part to perform its desired function. Because different parts must satisfy different functional requirements, the conditions which limit load-carrying ability may be quite different for different elements. As an example, compare the design limits for the floor of a house with those for the wing of an airplane.If we were to determine the size of the wooden beams in a home such that they simply did not break, we would not be very happy with them; they would be too ‘springy’. Walking across the room would be like walking out on a diving board.Obviously, we should be concerned with the maximum ‘deflection’that we, as individuals, find acceptable. This level will be rather subjective, and different people will give different answers. In fact, the same people may give different answers depending on whether they are paying for the floor or not!An airplane wing structure is clearly different. If you look out an airplane window and watch the wing during turbulentweather, you will see large deflections; in fact you may wish that they were smaller. However, you know that the important issue is that of ‘structural integrity’, not deflection.We want to be assured that the wing will remain intact. We want to be assured that no matter what the pilot and the weather do, that wing will continue to act like a good and proper wing. In fact, we really want to be assured that the wing will never fail under any conditions. Now that is a pretty tall order; who knows what the ‘worst’ conditions might be?Engineers who are responsible for the design of airplane wing structures must know, with some degree of certainty, what the ‘worst’ conditions are likely to be. It takes great patience and dedication for many years to assemble enough test data and failure analyses to be able to predict the ‘worst’case. The general procedure is to develop statistical data which allow us to say how frequently a given condition is likely to be encountered—once every 1000 hours, or once every 10000 hours, etc.As we said earlier, our object is to avoid failure. Suppose, however, that a part has failed in service, and we are asked; Why? ‘Error’ as such can come from three distinctly different sources, any or all of which can cause failure:1. Error in design: We the designers or the design analysts may have been a bit too optimistic: Maybe we ignored some loads; maybe our equations did not apply or were not properly applied; maybe we overestimated the intelligence of the user; may we slipped a decimal point.2. Error in manufacture: When a device involves heavily stressed members, the effective strength of the members can be greatly reduced through improper manufacture and assembly: May the wrong material was used; maybe the heat treatment was not as specified; maybe the surface finish was not as good as called for; may a part was ‘out of tolerance’; may be surface was damaged during machining; maybe the threads were not lubricated at assembly; or perhaps the bolts were not properly tightened.3. Error in use: As we all know, we can damage almost anything if we try hard enough, and sometimes we do so accidentally: We went too fast; we lost control; we fell asleep; we were not watching the gages; the power went off; the computer crashed; he was taking a coffee break; she forgot to turn the machine off; you failed to lubricate it, etc.Any of the above can happen: Nothing is designed perfectly; nothing is made perfectly; and nothing is used perfectly. Whenfailure does occur, and we try to determine the cause, we can usually examine the design; we can usually examine the failed parts for manufacturing deficiencies; but we cannot usually determine how the device was used (or misused). In serious cases, this can give rise to considerable differences of opinion, differences which frequently end in court.In an effort to account for all the above possibilities, we design every part with a safety factor. Simply put, the safety factor (SF) is the ratio of the load that we think the part can withstand to the load we expect it to experience. The safety factor can be applied by increasing the design loads beyond those actually expected, or by designing to stress levels below those that the material actually can withstand (frequently called ‘design stresses’).Safety factor=SF=failure load/design load=failure stress/design stress It is difficult to determine an appropriate value for the safety factor. In general, we should use larger values when:1. The possible consequences of failure are high in terms of life or cost.2. There are large uncertainties in the design analyses.Values of SF generally range from a low of about 1.5 to 5 ormore. When the incentives to reduce structural weight are great (as in aircraft and spacecraft), there is an obvious conflict. Safety dictates a large SF, while performance requires a small value. The only resolution involves reduction of uncertainty. Because of extreme care and diligence in design, test, manufacture, and use, the aircraft industry is able to maintain very enviable safety records while using safety factors as low as 1.5.We might not that the safety factor is frequently called the ‘ignorance factor’. This is not to imply that engineers are ignorant, but to help instill in them humility, caution, and care. An engineer is responsible for his or her design decisions, both ethically and legally. Try to learn from the mistakes of others rather than making your own.。
土木工程专业外文文献及翻译
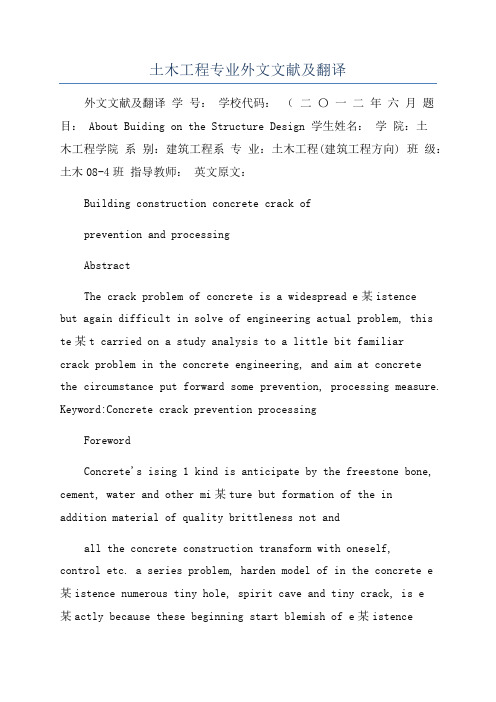
土木工程专业外文文献及翻译外文文献及翻译学号:学校代码:(二〇一二年六月题目: About Buiding on the Structure Design 学生姓名:学院:土木工程学院系别:建筑工程系专业:土木工程(建筑工程方向) 班级:土木08-4班指导教师:英文原文:Building construction concrete crack ofprevention and processingAbstractThe crack problem of concrete is a widespread e某istencebut again difficult in solve of engineering actual problem, this te某t carried on a study analysis to a little bit familiarcrack problem in the concrete engineering, and aim at concretethe circumstance put forward some prevention, processing measure. Keyword:Concrete crack prevention processingForewordConcrete's ising 1 kind is anticipate by the freestone bone, cement, water and other mi某ture but formation of the inaddition material of quality brittleness not andall the concrete construction transform with oneself,control etc. a series problem, harden model of in the concrete e某istence numerous tiny hole, spirit cave and tiny crack, is e某actly because these beginning start blemish of e某istencejust make the concrete present one some not and all the characteristic of tiny crack is a kind of harmless crack and accept concrete heavy, defend Shen and a little bit other use function not a creation to after the concrete be subjected to lotus carry, difference in temperature etc. function, tiny crack would continuously of e某pand with connect, end formation we can see without the aid of instruments of macro view the crack be also the crack that the concrete often say in the engineering.Concrete building and Gou piece usually all take sewer to make of, because of crack of e某istence and development usually make inner part of reinforcing bar etc. material creation decay, lower reinforced concrete material of loading ability, durable and anti- Shen ability, influence building of e某ternal appearance, service life, severity will threat arrive people's life and property lot of all of crash of engineerings is because of the unsteady development of the crack with the result age science research with a great deal of of the concrete engineering practice certificate, in the concrete engineering crack problem is ineluctable, also acceptable in certainly ofthe scope just need to adopt valid of measure will it endanger degree control at certain of scope reinforced concrete norm is also e某plicit provision:Some structure at place ofdissimilarity under the condition allow e某istence certain the crack of at under construction should as far as possible adopt a valid measure control crack creation, make the structure don'tappear crack possibly or as far as possible decrease crack of amount and width, particularly want to as far as possible avoid harmful crack of emergence, insure engineering quality thus.Concrete crack creation of the reason be a lot of and have already transformed to cause of crack:Such as temperature variety, constringency, inflation, the asymmetry sink to sink etc. reason cause of crack;Have outside carry the crack that the function cause;Protected environment not appropriate the crack etc. caused with chemical differentiation to treat in the actual engineering, work°out a problem according to the actual circumstance.In the concrete engineering the familiar crack and the prevention Suo crack and preventionSu constringency crack and preventionto sink crack and preventionThe creation which sink to sink crack is because of the structure foundation soil quality not and evenly, loose soft or return to fill soil dishonest or soak in water but result in the asymmetry sink to decline with the result that;Perhaps because of template just degree shortage, the template propped up to once be apart from big or prop up bottom loose move etc. to cause, especially at winter, the template prop up at jelly soil up, jelly the soil turn jelly empress creation asymmetry to sink to decline and cause concrete structure creation kind crack manyis deep enter or pierce through se某 crack, it alignment have something to do with sinking to sink a circumstance, general follow with ground perpendicular or present 30 °s-45 ° Cape direction development, bigger sink to sink crack, usually have certain of wrong, crack width usually with sink to decline quantity direct proportion width under the influence of temperature variety foundation after transform stability sink to sink crack also basic tend in stability.crack and prevention。
土木工程外文翻译-原文
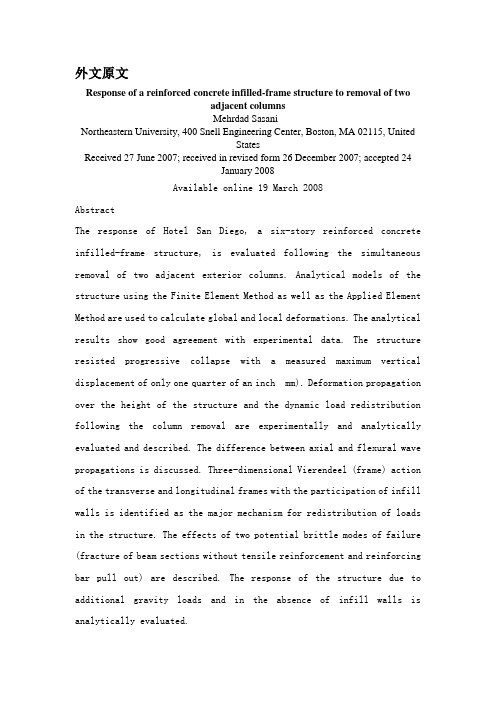
外文原文Response of a reinforced concrete infilled-frame structure to removal of twoadjacent columnsMehrdad Sasani_Northeastern University, 400 Snell Engineering Center, Boston, MA 02115, UnitedStatesReceived 27 June 2007; received in revised form 26 December 2007; accepted 24January 2008Available online 19 March 2008AbstractThe response of Hotel San Diego, a six-story reinforced concrete infilled-frame structure, is evaluated following the simultaneous removal of two adjacent exterior columns. Analytical models of the structure using the Finite Element Method as well as the Applied Element Method are used to calculate global and local deformations. The analytical results show good agreement with experimental data. The structure resisted progressive collapse with a measured maximum vertical displacement of only one quarter of an inch mm). Deformation propagation over the height of the structure and the dynamic load redistribution following the column removal are experimentally and analytically evaluated and described. The difference between axial and flexural wave propagations is discussed. Three-dimensional Vierendeel (frame) action of the transverse and longitudinal frames with the participation of infill walls is identified as the major mechanism for redistribution of loads in the structure. The effects of two potential brittle modes of failure (fracture of beam sections without tensile reinforcement and reinforcing bar pull out) are described. The response of the structure due to additional gravity loads and in the absence of infill walls is analytically evaluated.c 2008 Elsevier Ltd. All rights reserved.Keywords: Progressive collapse; Load redistribution; Load resistance; Dynamic response; Nonlinear analysis; Brittle failure1.IntroductionThe principal scope of specifications is to provide general principles and computational methods in order to verify safet y of structures. The “safety factor ”, which according t o modern trends is independent of the nature and combination of the materials used, can usually be defined as the rati o between the conditions. This ratio is also proportional to the inverse of the probability ( risk ) of failure of th e structure.Failure has to be considered not only as overall collapse o f the structure but also as unserviceability or, according t o a more precise. Common definition. As the reaching of a “limit state ”which causes the construction not to acco mplish the task it was designed for. There are two categori es of limit state :(1)Ultimate limit sate, which corresponds to the highest value of the load-bearing capacity. Examples include local buckli ng or global instability of the structure; failure of some sections and subsequent transformation of the structure intoa mechanism; failure by fatigue; elastic or plastic deformati on or creep that cause a substantial change of the geometry of the structure; and sensitivity of the structure to alte rnating loads, to fire and to explosions.(2)Service limit states, which are functions of the use and durability of the structure. Examples include excessive defo rmations and displacements without instability; early or exces sive cracks; large vibrations; and corrosion.Computational methods used to verify structures with respect to the different safety conditions can be separated into: (1)Deterministic methods, in which the main parameters are co nsidered as nonrandom parameters.(2)Probabilistic methods, in which the main parameters are co nsidered as random parameters.Alternatively, with respect to the different use of factors of safety, computational methods can be separated into:(1)Allowable stress method, in which the stresses computed un der maximum loads are compared with the strength of the mat erial reduced by given safety factors.(2)Limit states method, in which the structure may be propor tioned on the basis of its maximum strength. This strength, as determined by rational analysis, shall not be less than that required to support a factored load equal to the sum of the factored live load and dead load ( ultimate state ).The stresses corresponding to working ( service ) conditions with unfactored live and dead loads are compared with pres cribed values ( service limit state ) . From the four poss ible combinations of the first two and second two methods, we can obtain some useful computational methods. Generally, t wo combinations prevail:(1)deterministic methods, which make use of allowable stresses . (2)Probabilistic methods, which make use of limit states. The main advantage of probabilistic approaches is that, at l east in theory, it is possible to scientifically take into account all random factors of safety, which are then combine d to define the safety factor. probabilistic approaches depend upon :(1) Random distribution of strength of materials with respect to the conditions of fabrication and erection ( scatter of the values of mechanical properties through out the structu re ); (2) Uncertainty of the geometry of the cross-section sand of the structure ( faults and imperfections due to fab rication and erection of the structure );(3) Uncertainty of the predicted live loads and dead loads acting on the structure; (4)Uncertainty related to the approx imation of the computational method used ( deviation of the actual stresses from computed stresses ). Furthermore, proba bilistic theories mean that the allowable risk can be based on several factors, such as :(1) Importance of the construction and gravity of the damage by its failure; (2)Number of human lives which can be thr eatened by this failure; (3)Possibility and/or likelihood of repairing the structure; (4) Predicted life of the structure. All these factors are related to economic and social consi derations such as:(1) Initial cost of the construction;(2) Amortization funds for the duration of the construction;(3) Cost of physical and material damage due to the failure of the construction;(4) Adverse impact on society;(5) Moral and psychological views.The definition of all these parameters, for a given saf ety factor, allows construction at the optimum cost. However, the difficulty of carrying out a complete probabilistic ana lysis has to be taken into account. For such an analysis t he laws of the distribution of the live load and its induc ed stresses, of the scatter of mechanical properties of mate rials, and of the geometry of the cross-sections and the st ructure have to be known. Furthermore, it is difficult to i nterpret the interaction between the law of distribution of strength and that of stresses because both depend upon the nature of the material, on the cross-sections and upon the load acting on the structure. These practical difficulties ca n be overcome in two ways. The first is to apply different safety factors to the material and to the loads, without necessarily adopting the probabilistic criterion. The second i s an approximate probabilistic method which introduces some s implifying assumptions ( semi-probabilistic methods ) . Aspart of mitigation programs to reduce the likelihood of mass casualties following local damage in structures, the General Services Administration [1] and the Department of Defense [2] developed regulations to evaluate progressive collapse resistance of structures. ASCE/SEI 7 [3] defines progressive collapse as the spread of an initial local failure fromelement to element eventually resulting in collapse of an entire structure or a disproportionately large part of it. Following the approaches proposed by Ellinwood and Leyendecker [4], ASCE/SEI 7 [3] defines two general methods for structural design of buildings to mitigate damage due to progressive collapse: indirect and direct design methods. General building codes and standards [3,5] use indirect design by increasing overall integrity of structures. Indirect design is also used in DOD [2]. Although the indirect design method can reduce the risk of progressive collapse [6,7] estimation of post-failure performance of structures designed based on such a method is not readily possible. One approach based on direct design methods to evaluate progressive collapse of structures is to study the effects of instantaneous removal of load-bearing elements, such as columns. GSA [1] and DOD [2] regulations require removal of one load bearing element. These regulations are meant to evaluate general integrity of structures and their capacity of redistributing the loads following severe damage to only one element. While such an approach provides insight as to the extent to which the structures are susceptible to progressive collapse, in reality, the initial damage can affect more than just one column. In this study, using analytical results that are verified against experimental data, the progressive collapse resistance of the Hotel San Diego is evaluated, following the simultaneous explosion (sudden removal) of two adjacent columns, one of which was a corner column. In order to explode the columns, explosives were inserted into predrilled holes in the columns. The columns were then well wrapped with a few layers of protective materials. Therefore, neither air blast nor flying fragments affected the structure.2. Building characteristicsHotel San Diego was constructed in 1914 with a south annex added in 1924. The annex included two separate buildings. Fig. 1 shows a south view of the hotel. Note that in the picture, the first and third stories of the hotel are covered with black fabric. The six story hotel had a non-ductile reinforced concrete (RC) frame structure with hollow clay tile exterior infill walls. The infills in the annex consisted of two withes (layers) of clay tiles with a total thickness of about 8 in (203 mm). The height of the first floor was about 190–800 m). The height of other floors and that of the top floor were 100–600 m) and 160–1000 m), respectively. Fig. 2 shows the second floor of one of the annex buildings. Fig. 3 shows a typical plan of this building, whose responsefollowing the simultaneous removal (explosion) of columns A2 and A3 in the first (ground) floor is evaluated in this paper. The floor system consisted of one-way joists running in the longitudinal direction (North–South), as shown in Fig. 3. Based on compression tests of two concrete samples, the average concrete compressive strength was estimated at about 4500 psi (31 MPa) for a standard concrete cylinder. The modulus of elasticity of concrete was estimated at 3820 ksi (26 300 MPa) [5]. Also, based on tension tests of two steel samples having 1/2 in mm) square sections, the yield and ultimate tensile strengths were found to be 62 ksi (427 MPa) and 87 ksi (600 MPa), respectively. The steel ultimate tensile strain was measured at . The modulus of elasticity of steel was set equal to 29 000 ksi (200 000 MPa). The building was scheduled to be demolished by implosion. As part of the demolition process, the infill walls were removed from the first and third floors. There was no live load in the building. All nonstructural elements including partitions, plumbing, and furniture were removed prior to implosion. Only beams, columns, joist floor and infill walls on the peripheral beams were present.3. SensorsConcrete and steel strain gages were used to measure changes in strains of beams and columns. Linear potentiometers were used to measure global and local deformations. The concrete strain gages were in (90 mm) long having a maximum strain limit of ±. The steel strain gages could measure up to a strain of ±. The strain gages could operate up to a several hundred kHz sampling rate. The sampling rate used in the experiment was 1000 Hz. Potentiometers were used to capture rotation (integral of curvature over a length) of the beam end regions and global displacementin the building, as described later. The potentiometers had a resolution of about in mm) and a maximum operational speed of about 40 in/s m/s), while the maximum recorded speed in the experiment was about 14 in/sm/s).4. Finite element modelUsing the finite element method (FEM), a model of the building was developed in the SAP2000 [8] computer program. The beams and columns are modeled with Bernoulli beam elements. Beams have T or L sections with effective flange width on each side of the web equal to four times the slab thickness [5]. Plastic hinges are assigned to all possible locations where steel bar yielding can occur, including the ends of elements as well as the reinforcing bar cut-off and bend locations. The characteristics of the plastic hinges are obtained using section analysesof the beams and columns and assuming a plastic hinge length equal to half of the section depth. The current version of SAP2000 [8] is not able to track formation of cracks in the elements. In order to find the proper flexural stiffness of sections, an iterative procedure is used as follows. First, the building is analyzed assuming all elements are uncracked. Then, moment demands in the elements are compared with their cracking bending moments, Mcr . The moment of inertia of beam and slab segments are reduced by a coefficient of [5], where the demand exceeds the Mcr. The exterior beam cracking bending moments under negative and positive moments, are 516 k in kN m) and 336 k in kN m), respectively. Note that no cracks were formed in the columns. Then the building is reanalyzed and moment diagrams are re-evaluated. This procedure is repeated until all of the cracked regions are properly identified and modeled.The beams in the building did not have top reinforcing bars except at the end regions (see Fig. 4). For instance, no top reinforcement was provided beyond the bend in beam A1–A2, 12 inches away from the face of column A1 (see Figs. 4 and 5). To model the potential loss of flexural strength in those sections, localized crack hinges were assigned at the critical locations where no top rebar was present. Flexural strengths of the hinges were set equal to Mcr. Such sections were assumed to lose their flexural strength when the imposed bending moments reached Mcr.The floor system consisted of joists in the longitudinal direction (North–South). Fig. 6 shows the cross section of a typical floor. In order to account for potential nonlinear response of slabs and joists, floors are molded by beam elements. Joists are modeled with T-sections, having effective flange width on each side of the web equal to four times the slab thickness [5]. Given the large joist spacing between axes 2 and 3, two rectangular beam elements with 20-inch wide sections are used between the joist and the longitudinal beams of axes 2 and 3 to model the slab in the longitudinal direction. To model the behavior of the slab in the transverse direction, equally spaced parallel beams with 20-inch wide rectangular sections are used. There is a difference between the shear flow in the slab and that in the beam elements with rectangular sections modeling the slab. Because of this, the torsional stiffness is setequal to one-half of that of the gross sections [9].The building had infill walls on 2nd, 4th, 5th and 6th floors on the spandrel beams with some openings . windows and doors). As mentioned before and as part of the demolition procedure, the infill walls in the 1st and 3rd floors were removed before the test. The infill walls were made of hollow clay tiles, which were in good condition. The net area of the clay tiles was about 1/2 of the gross area. The in-plane action of the infill walls contributes to the building stiffness and strength and affects the building response. Ignoring the effects of the infill walls and excluding them in the model would result in underestimating the building stiffness and strength.Using the SAP2000 computer program [8], two types of modeling for the infills are considered in this study: one uses two dimensional shell elements (Model A) and the other uses compressive struts (Model B) as suggested in FEMA356 [10] guidelines.. Model A (infills modeled by shell elements)Infill walls are modeled with shell elements. However, the current version of the SAP2000 computer program includes only linear shell elements and cannot account for cracking. The tensile strength of the infill walls is set equal to 26 psi, with a modulus of elasticity of 644 ksi [10]. Because the formation ofcracks has a significant effect on the stiffness of the infill walls, the following iterative procedure is used to account for crack formation:(1) Assuming the infill walls are linear and uncracked, a nonlinear time history analysis is run. Note that plastic hinges exist in the beam elements and the segments of the beam elements where moment demand exceeds the cracking moment have a reduced moment of inertia.(2) The cracking pattern in the infill wall is determined by comparingstresses in the shells developed during the analysis with the tensile strength of infills.(3) Nodes are separated at the locations where tensile stress exceeds tensile strength. These steps are continued until the crack regions are properly modeled.. Model B (infills modeled by struts)Infill walls are replaced with compressive struts as described in FEMA 356 [10] guidelines. Orientations of the struts are determined from the deformed shape of the structure after column removal and the location of openings.. Column removalRemoval of the columns is simulated with the following procedure. (1) The structure is analyzed under the permanent loads and the internal forces are determined at the ends of the columns, which will be removed.(2) The model is modified by removing columns A2 and A3 on the first floor. Again the structure is statically analyzed under permanent loads. In this case, the internal forces at the ends of removed columns found in the first step are applied externally to the structure along with permanent loads. Note that the results of this analysis are identical to those of step 1.(3) The equal and opposite column end forces that were applied in the second step are dynamically imposed on the ends of the removed column within one millisecond [11] to simulate the removal of the columns, and dynamic analysis is conducted.. Comparison of analytical and experimental resultsThe maximum calculated vertical displacement of the building occurs at joint A3 in the second floor. Fig. 7 shows the experimental andanalytical (Model A) vertical displacements of this joint (the AEM results will be discussed in the next section). Experimental data is obtained using the recordings of three potentiometers attached to joint A3 on one of their ends, and to the ground on the other ends. The peak displacements obtained experimentally and analytically (Model A) are in mm) and in mm), respectively, which differ only by about 4%. The experimental and analytical times corresponding to peak displacement are s and s, respectively. The analytical results show a permanent displacement of about in mm), which is about 14% smaller than the corresponding experimental value of in mm).Fig. 8 compares vertical displacement histories of joint A3 in the second floor estimated analytically based on Models A and B. As can be seen, modeling infills with struts (Model B) results in a maximum vertical displacement of joint A3 equal to about in mm), which is approximately 80% larger than the value obtained from Model A. Note that the results obtained from Model A are in close agreement with experimental results (see Fig. 7), while Model B significantly overestimates the deformation of the structure. If the maximum vertical displacement were larger, the infill walls were more severely cracked and the struts were more completely formed, the difference between the results of the two models (Models A and B) would be smaller.Fig. 9 compares the experimental and analytical (Model A) displacement of joint A2 in the second floor. Again, while the first peak vertical displacement obtained experimentally and analytically are in good agreement, the analytical permanent displacement under estimates the experimental value.Analytically estimated deformed shapes of the structure at the maximumvertical displacement based on Model A are shown in Fig. 10 with a magnification factor of 200. The experimentally measured deformed shape over the end regions of beams A1–A2 and A3–B3 in the second floorare represented in the figure by solid lines. A total of 14 potentiometers were located at the top and bottom of the end regions of the second floor beams A1–A2 and A3–B3, which were the most critical elements in load redistribution. The beam top and corresponding bottom potentiometerrecordings were used to calculate rotation between the sections where the potentiometer ends were connected. This was done by first finding the difference between the recorded deformations at the top and bottom of the beam, and then dividing the value by the distance (along the height of the beam section) between the two potentiometers. The expected deformed shapes between the measured end regions of the second floor beams are shown by dashed lines. As can be seen in the figures, analytically estimated deformed shapes of the beams are in good agreement with experimentally obtained deformed shapes.Analytical results of Model A show that only two plastic hinges are formed indicating rebar yielding. Also, four sections that did not have negative (top) reinforcement, reached cracking moment capacities and therefore cracked. Fig. 10 shows the locations of all the formed plastic hinges and cracks.。
土木工程--外文文献翻译
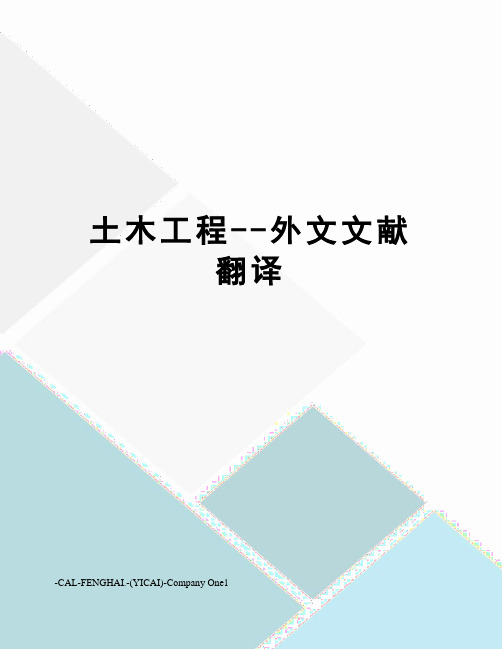
土木工程--外文文献翻译-CAL-FENGHAI.-(YICAI)-Company One1学院:专业:土木工程姓名:学号:外文出处: Structural Systems to resist (用外文写)Lateral loads附件: 1.外文资料翻译译文;2.外文原文。
附件1:外文资料翻译译文抗侧向荷载的结构体系常用的结构体系若已测出荷载量达数千万磅重,那么在高层建筑设计中就没有多少可以进行极其复杂的构思余地了。
确实,较好的高层建筑普遍具有构思简单、表现明晰的特点。
这并不是说没有进行宏观构思的余地。
实际上,正是因为有了这种宏观的构思,新奇的高层建筑体系才得以发展,可能更重要的是:几年以前才出现的一些新概念在今天的技术中已经变得平常了。
如果忽略一些与建筑材料密切相关的概念不谈,高层建筑里最为常用的结构体系便可分为如下几类:1.抗弯矩框架。
2.支撑框架,包括偏心支撑框架。
3.剪力墙,包括钢板剪力墙。
4.筒中框架。
5.筒中筒结构。
6.核心交互结构。
7. 框格体系或束筒体系。
特别是由于最近趋向于更复杂的建筑形式,同时也需要增加刚度以抵抗几力和地震力,大多数高层建筑都具有由框架、支撑构架、剪力墙和相关体系相结合而构成的体系。
而且,就较高的建筑物而言,大多数都是由交互式构件组成三维陈列。
将这些构件结合起来的方法正是高层建筑设计方法的本质。
其结合方式需要在考虑环境、功能和费用后再发展,以便提供促使建筑发展达到新高度的有效结构。
这并不是说富于想象力的结构设计就能够创造出伟大建筑。
正相反,有许多例优美的建筑仅得到结构工程师适当的支持就被创造出来了,然而,如果没有天赋甚厚的建筑师的创造力的指导,那么,得以发展的就只能是好的结构,并非是伟大的建筑。
无论如何,要想创造出高层建筑真正非凡的设计,两者都需要最好的。
虽然在文献中通常可以见到有关这七种体系的全面性讨论,但是在这里还值得进一步讨论。
设计方法的本质贯穿于整个讨论。
土木工程专业毕业设计外文文献翻译2篇
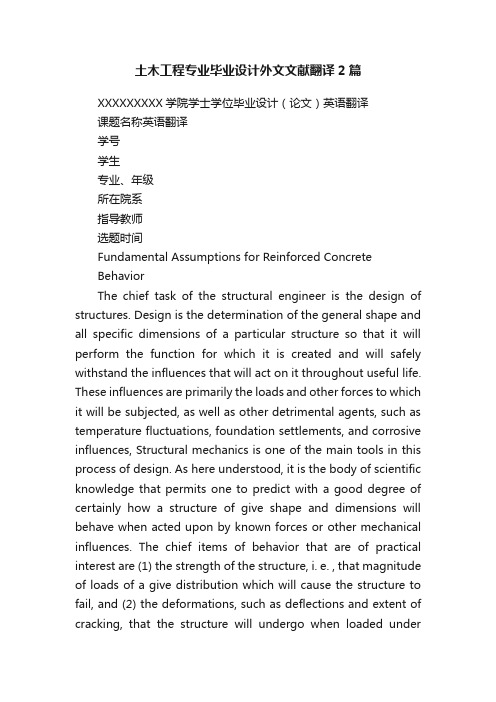
土木工程专业毕业设计外文文献翻译2篇XXXXXXXXX学院学士学位毕业设计(论文)英语翻译课题名称英语翻译学号学生专业、年级所在院系指导教师选题时间Fundamental Assumptions for Reinforced ConcreteBehaviorThe chief task of the structural engineer is the design of structures. Design is the determination of the general shape and all specific dimensions of a particular structure so that it will perform the function for which it is created and will safely withstand the influences that will act on it throughout useful life. These influences are primarily the loads and other forces to which it will be subjected, as well as other detrimental agents, such as temperature fluctuations, foundation settlements, and corrosive influences, Structural mechanics is one of the main tools in this process of design. As here understood, it is the body of scientific knowledge that permits one to predict with a good degree of certainly how a structure of give shape and dimensions will behave when acted upon by known forces or other mechanical influences. The chief items of behavior that are of practical interest are (1) the strength of the structure, i. e. , that magnitude of loads of a give distribution which will cause the structure to fail, and (2) the deformations, such as deflections and extent of cracking, that the structure will undergo when loaded underservice condition.The fundamental propositions on which the mechanics of reinforced concrete is based are as follows:1.The internal forces, such as bending moments, shear forces, and normal andshear stresses, at any section of a member are in equilibrium with the effect of the external loads at that section. This proposition is not an assumption but a fact, because any body or any portion thereof can be at rest only if all forces acting on it are in equilibrium.2.The strain in an embedded reinforcing bar is the same as that of thesurrounding concrete. Expressed differently, it is assumed that perfect bonding exists between concrete and steel at the interface, so that no slip can occur between the two materials. Hence, as the one deforms, so must the other. With modern deformed bars, a high degree of mechanical interlocking is provided in addition to the natural surface adhesion, so this assumption is very close to correct.3.Cross sections that were plane prior to loading continue to be plan in themember under load. Accurate measurements have shown that when a reinforced concrete member is loaded close to failure, this assumption is not absolutely accurate. However, the deviations are usually minor.4.In view of the fact the tensile strength of concrete is only a small fraction ofits compressive strength; the concrete in that part of a member which is in tension is usually cracked. While these cracks, in well-designed members, are generally so sorrow as to behardly visible, they evidently render the cracked concrete incapable of resisting tension stress whatever. This assumption is evidently a simplification of the actual situation because, in fact, concrete prior to cracking, as well as the concrete located between cracks, does resist tension stresses of small magnitude. Later in discussions of the resistance of reinforced concrete beams to shear, it will become apparent that under certain conditions this particular assumption is dispensed with and advantage is taken of the modest tensile strength that concrete can develop.5.The theory is based on the actual stress-strain relation ships and strengthproperties of the two constituent materials or some reasonable equivalent simplifications thereof. The fact that novelistic behavior is reflected in modern theory, that concrete is assumed to be ineffective in tension, and that the joint action of the two materials is taken into consideration results in analytical methods which are considerably more complex and also more challenging, than those that are adequate for members made of a single, substantially elastic material.These five assumptions permit one to predict by calculation the performance of reinforced concrete members only for some simple situations. Actually, the joint action of two materials as dissimilar and complicated as concrete and steel is so complex that it has not yet lent itself to purely analytical treatment. For this reason, methods of design and analysis, while using these assumptions, are very largely based on the results of extensive and continuing experimental research. They are modified and improved as additional test evidence becomes available.钢筋混凝土的基本假设作为结构工程师的主要任务是结构设计。
土木工程英文文献及翻译
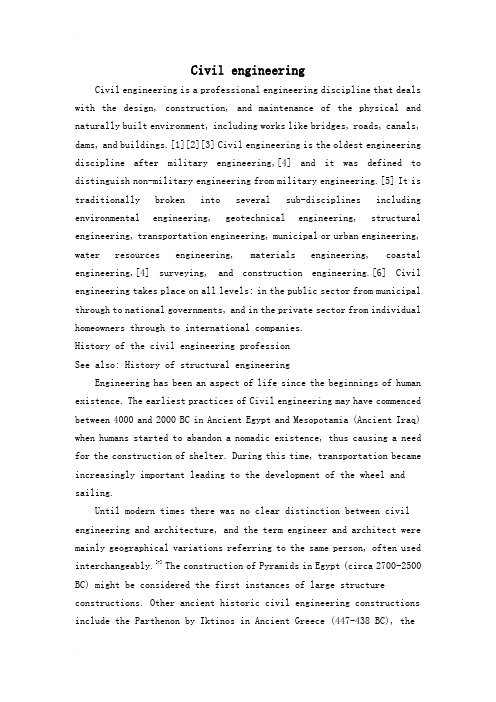
Civil engineeringCivil engineering is a professional engineering discipline that deals with the design, construction, and maintenance of the physical and naturally built environment, including works like bridges, roads, canals, dams, and buildings.[1][2][3] Civil engineering is the oldest engineering discipline after military engineering,[4] and it was defined to distinguish non-military engineering from military engineering.[5] It is traditionally broken into several sub-disciplines including environmental engineering, geotechnical engineering, structural engineering, transportation engineering, municipal or urban engineering, water resources engineering, materials engineering, coastal engineering,[4] surveying, and construction engineering.[6] Civil engineering takes place on all levels: in the public sector from municipal through to national governments, and in the private sector from individual homeowners through to international companies.History of the civil engineering professionSee also: History of structural engineeringEngineering has been an aspect of life since the beginnings of human existence. The earliest practices of Civil engineering may have commenced between 4000 and 2000 BC in Ancient Egypt and Mesopotamia (Ancient Iraq) when humans started to abandon a nomadic existence, thus causing a need for the construction of shelter. During this time, transportation became increasingly important leading to the development of the wheel and sailing.Until modern times there was no clear distinction between civil engineering and architecture, and the term engineer and architect were mainly geographical variations referring to the same person, often used interchangeably.[7]The construction of Pyramids in Egypt (circa 2700-2500 BC) might be considered the first instances of large structure constructions. Other ancient historic civil engineering constructions include the Parthenon by Iktinos in Ancient Greece (447-438 BC), theAppian Way by Roman engineers (c. 312 BC), the Great Wall of China by General Meng T'ien under orders from Ch'in Emperor Shih Huang Ti (c. 220 BC)[6] and the stupas constructed in ancient Sri Lanka like the Jetavanaramaya and the extensive irrigation works in Anuradhapura. The Romans developed civil structures throughout their empire, including especially aqueducts, insulae, harbours, bridges, dams and roads.In the 18th century, the term civil engineering was coined to incorporate all things civilian as opposed to military engineering.[5]The first self-proclaimed civil engineer was John Smeaton who constructed the Eddystone Lighthouse.[4][6]In 1771 Smeaton and some of his colleagues formed the Smeatonian Society of Civil Engineers, a group of leaders of the profession who met informally over dinner. Though there was evidence of some technical meetings, it was little more than a social society.In 1818 the Institution of Civil Engineers was founded in London, and in 1820 the eminent engineer Thomas Telford became its first president. The institution received a Royal Charter in 1828, formally recognising civil engineering as a profession. Its charter defined civil engineering as:the art of directing the great sources of power in nature for the use and convenience of man, as the means of production and of traffic in states, both for external and internal trade, as applied in the construction of roads, bridges, aqueducts, canals, river navigation and docks for internal intercourse and exchange, and in the construction of ports, harbours, moles, breakwaters and lighthouses, and in the art of navigation by artificial power for the purposes of commerce, and in the construction and application of machinery, and in the drainage of cities and towns.[8] The first private college to teach Civil Engineering in the United States was Norwich University founded in 1819 by Captain Alden Partridge.[9] The first degree in Civil Engineering in the United States was awarded by Rensselaer Polytechnic Institute in 1835.[10] The first such degree to be awarded to a woman was granted by Cornell University to Nora Stanton Blatchin 1905.History of civil engineeringCivil engineering is the application of physical and scientific principles, and its history is intricately linked to advances in understanding of physics and mathematics throughout history. Because civil engineering is a wide ranging profession, including several separate specialized sub-disciplines, its history is linked to knowledge of structures, materials science, geography, geology, soils, hydrology, environment, mechanics and other fields.Throughout ancient and medieval history most architectural design and construction was carried out by artisans, such as stone masons and carpenters, rising to the role of master builder. Knowledge was retained in guilds and seldom supplanted by advances. Structures, roads and infrastructure that existed were repetitive, and increases in scale were incremental.[12]One of the earliest examples of a scientific approach to physical and mathematical problems applicable to civil engineering is the work of Archimedes in the 3rd century BC, including Archimedes Principle, which underpins our understanding of buoyancy, and practical solutions such as Archimedes' screw. Brahmagupta, an Indian mathematician, used arithmetic in the 7th century AD, based on Hindu-Arabic numerals, for excavation (volume) computations.[13]Civil engineers typically possess an academic degree with a major in civil engineering. The length of study for such a degree is usually three to five years and the completed degree is usually designated as a Bachelor of Engineering, though some universities designate the degree as a Bachelor of Science. The degree generally includes units covering physics, mathematics, project management, design and specific topics in civil engineering. Initially such topics cover most, if not all, of thesub-disciplines of civil engineering. Students then choose to specialize in one or more sub-disciplines towards the end of the degree.[14]While anUndergraduate (BEng/BSc) Degree will normally provide successful students with industry accredited qualification, some universities offer postgraduate engineering awards (MEng/MSc) which allow students to further specialize in their particular area of interest within engineering.[15]In most countries, a Bachelor's degree in engineering represents the first step towards professional certification and the degree program itself is certified by a professional body. After completing a certified degree program the engineer must satisfy a range of requirements (including work experience and exam requirements) before being certified. Once certified, the engineer is designated the title of Professional Engineer (in the United States, Canada and South Africa), Chartered Engineer (in most Commonwealth countries), Chartered Professional Engineer (in Australia and New Zealand), or European Engineer (in much of the European Union). There are international engineering agreements between relevant professional bodies which are designed to allow engineers to practice across international borders.The advantages of certification vary depending upon location. For example, in the United States and Canada "only a licensed engineer may prepare, sign and seal, and submit engineering plans and drawings to a public authority for approval, or seal engineering work for public and private clients.".[16]This requirement is enforced by state and provincial legislation such as Quebec's Engineers Act.[17]In other countries, no such legislation exists. In Australia, state licensing of engineers is limited to the state of Queensland. Practically all certifying bodies maintain a code of ethics that they expect all members to abide by or risk expulsion.[18] In this way, these organizations play an important role in maintaining ethical standards for the profession. Even in jurisdictions where certification has little or no legal bearing on work, engineers are subject to contract law. In cases where an engineer's work fails he or she may be subject to the tort of negligence and, in extreme cases, thecharge of criminal negligence.[citation needed] An engineer's work must also comply with numerous other rules and regulations such as building codes and legislation pertaining to environmental law.CareersThere is no one typical career path for civil engineers. Most people who graduate with civil engineering degrees start with jobs that require a low level of responsibility, and as the new engineers prove their competence, they are trusted with tasks that have larger consequences and require a higher level of responsibility. However, within each branch of civil engineering career path options vary. In some fields and firms, entry-level engineers are put to work primarily monitoring construction in the field, serving as the "eyes and ears" of senior design engineers; while in other areas, entry-level engineers perform the more routine tasks of analysis or design and interpretation. Experienced engineers generally do more complex analysis or design work, or management of more complex design projects, or management of other engineers, or into specialized consulting, including forensic engineering.In general, civil engineering is concerned with the overall interface of human created fixed projects with the greater world. General civil engineers work closely with surveyors and specialized civil engineers to fit and serve fixed projects within their given site, community and terrain by designing grading, drainage, pavement, water supply, sewer service, electric and communications supply, and land divisions. General engineers spend much of their time visiting project sites, developing community consensus, and preparing construction plans. General civil engineering is also referred to as site engineering, a branch of civil engineering that primarily focuses on converting a tract of land from one usage to another. Civil engineers typically apply the principles of geotechnical engineering, structural engineering, environmental engineering, transportation engineering and construction engineering toresidential, commercial, industrial and public works projects of all sizes and levels of construction翻译:土木工程土木工程是一个专业的工程学科,包括设计,施工和维护与环境的改造,涉及了像桥梁,道路,河渠,堤坝和建筑物工程交易土木工程是最古老的军事工程后,工程学科,它被定义为区分军事工程非军事工程的学科它传统分解成若干子学科包括环境工程,岩土工程,结构工程,交通工程,市或城市工程,水资源工程,材料工程,海岸工程,勘测和施工工程等土木工程的范围涉及所有层次:从市政府到国家,从私人部门到国际公司。
- 1、下载文档前请自行甄别文档内容的完整性,平台不提供额外的编辑、内容补充、找答案等附加服务。
- 2、"仅部分预览"的文档,不可在线预览部分如存在完整性等问题,可反馈申请退款(可完整预览的文档不适用该条件!)。
- 3、如文档侵犯您的权益,请联系客服反馈,我们会尽快为您处理(人工客服工作时间:9:00-18:30)。
1 中文翻译摘要:为了研究连续型拓扑优化理论在实际工程中的应用,该文给出了一种多层钢框架支撑体系连续型拓扑优化设计方法。
基于灵敏度分析,探讨了连续体结构在多工况荷载作用下、同时受应力和多位移约束的拓扑优化删除准则。
为保证拓扑优化结果的合理性,提出了设计区域平均厚度的概念。
在该文给出的优化设计方法中,首先在不考虑位移约束的情况下对无支撑钢框架进行优化设计,然后在有位移约束的条件下采用渐进结构优化算法和删除准则对支撑体系进行连续型拓扑优化设计,并将获得的支撑最优拓扑构形转化成相应的杆件。
通过一个3跨12层钢框架支撑体系的拓扑优化设计实例验证了该文给出的钢框架支撑体系连续型拓扑优化设计方法的有效性。
关键词:钢框架;支撑体系;连续型;拓扑优化;渐进结构优化1.1钢筋混凝土素混凝土是由水泥、水、细骨料、粗骨料(碎石或;卵石)、空气,通常还有其他外加剂等经过凝固硬化而成。
将可塑的混凝土拌合物注入到模板内,并将其捣实,然后进行养护,以加速水泥与水的水化反应,最后获得硬化的混凝土。
其最终制成品具有较高的抗压强度和较低的抗拉强度。
其抗拉强度约为抗压强度的十分之一。
因此,截面的受拉区必须配置抗拉钢筋和抗剪钢筋以增加钢筋混凝土构件中较弱的受拉区的强度。
由于钢筋混凝土截面在均质性上与标准的木材或钢的截面存在着差异,因此,需要对结构设计的基本原理进行修改。
将钢筋混凝土这种非均质截面的两种组成部分按一定比例适当布置,可以最好的利用这两种材料。
这一要求是可以达到的。
因混凝土由配料搅拌成湿拌合物,经过振捣并凝固硬化,可以做成任何一种需要的形状。
如果拌制混凝土的各种材料配合比恰当,则混凝土制成品的强度较高,经久耐用,配置钢筋后,可以作为任何结构体系的主要构件。
浇筑混凝土所需要的技术取决于即将浇筑的构件类型,诸如:柱、梁、墙、板、基础,大体积混凝土水坝或者继续延长已浇筑完毕并且已经凝固的混凝土等。
对于梁、柱、墙等构件,当模板清理干净后应该在其上涂油,钢筋表面的锈及其他有害物质也应该被清除干净。
浇筑基础前,应将坑底土夯实并用水浸湿6英寸,以免土壤从新浇的混凝土中吸收水分。
一般情况下,除使用混凝土泵浇筑外,混凝土都应在水平方向分层浇筑,并使用插入式或表面式高频电动振捣器捣实。
必须记住,过分的振捣将导致骨料离析和混凝土泌浆等现象,因而是有害的。
水泥的水化作用发生在有水分存在,而且气温在50°F以上的条件下。
为了保证水泥的水化作用得以进行,必须具备上述条件。
如果干燥过快则会出现表面裂缝,这将有损与混凝土的强度,同时也会影响到水泥水化作用的充分进行。
设计钢筋混凝土构件时显然需要处理大量的参数,诸如宽度、高度等几何尺寸,配筋的面积,钢筋的应变和混凝土的应变,钢筋的应力等等。
因此,在选择混凝土截面时需要进行试算并作调整,根据施工现场条件、混凝土原材料的供应情况、业主提出的特殊要求、对建筑和净空高度的要求、所用的设计规范以及建筑物周围环境条件等最后确定截面。
钢筋混凝土通常是现场浇注的合成材料,它与在工厂中制造的标准的钢结构梁、柱等不同,因此对于上面所提到的一系列因素必须予以考虑。
对结构体系的各个部位均需选定试算截面并进行验算,以确定该截面的名义强度是否足以承受所作用的计算荷载。
由于经常需要进行多次试算,才能求出所需的截面,因此设计时第一次采用的数值将导致一系列的试算与调整工作。
选择混凝土截面时,采用试算与调整过程可以使复核与设计结合在一起。
因此,当试算截面选定后,每次设计都是对截面进行复核。
手册、图表和微型计算机以及专用程序的使用,使这种设计方法更为简捷有效,而传统的方法则是把钢筋混凝土的复核与单纯的设计分别进行处理。
1.2土方工程由于和土木工程中任何其他工种的施工方法与费用相比较,土方挖运的施工方法与费用的变化都要快得多,因此对于有事业心的人来说,土方工程是一个可以大有作为的领域。
在1935年,目前采用的利用轮胎式机械设备进行土方挖运的方法大多数还没有出现。
那是大部分土方是采用窄轨铁路运输,在这目前来说是很少采用的。
当时主要的开挖方式是使用正铲、反铲、拉铲或抓斗等挖土机,尽管这些机械目前仍然在广泛应用,但是它们只不过是目前所采用的许多方法中的一小部分。
因此,一个工程师为了使自己在土方挖运设备方面的知识跟得上时代的发展,他应当花费一些时间去研究现代的机械。
一般说来,有关挖土机、装载机和运输机械的唯一可靠而又最新的资料可以从制造厂商处获得。
土方工程或土方挖运工程指的是把地表面过高处的土壤挖去(挖方),并把它倾卸到地表面过低的其他地方(填方)。
为了降低土方工程费用,填方量应该等于挖方量,而且挖方地点应该尽可能靠近土方量相等的填方地点,以减少运输量和填方的二次搬运。
土方设计这项工作落到了从事道路设计的工程师的身上,因为土方工程的设计比其他任何工作更能决定工程造价是否低廉。
根据现有的地图和标高,道路工程师应在设计绘图室中的工作也并不是徒劳的。
它将帮助他在最短的时间内获得最好的方案。
费用最低的运土方法是用同一台机械直接挖方取土并且卸土作为填方。
这并不是经常可以做到的,但是如果能够做到则是很理想的,因为这样做既快捷又省钱。
拉铲挖土机。
推土机和正铲挖土机都能做到这点。
拉铲挖土机的工作半径最大。
推土机所推运的图的数量最多,只是运输距离很短。
拉铲挖土机的缺点是只能挖比它本身低的土,不能施加压力挖入压实的土壤内,不能在陡坡上挖土,而且挖。
卸都不准确。
正铲挖土机介于推土机和拉铲挖土机的之间,其作用半径大于推土机,但小于拉铲挖土机。
正铲挖土机能挖取竖直陡峭的工作面,这种方式对推土机司机来说是危险的,而对拉铲挖土机则是不可能的。
每种机械设备应该进行最适合它的性能的作业。
正铲挖土机不能挖比其停机平面低很多的土,而深挖坚实的土壤时,反铲挖土机最适用,但其卸料半径比起装有正铲的同一挖土机的卸料半径则要小很多。
在比较平坦的场地开挖,如果用拉铲或正铲挖土机运输距离太远时,则装有轮胎式的斗式铲运机就是比不可少的。
它能在比较平的地面上挖较深的土(但只能挖机械本身下面的土),需要时可以将土运至几百米远,然后卸土并在卸土的过程中把土大致铲平。
在挖掘硬土时,人们发现在开挖场地经常用一辆助推拖拉机(轮式或履带式),对返回挖土的铲运机进行助推这种施工方法是经济的。
一旦铲运机装满,助推拖拉机就回到开挖的地点去帮助下一台铲运机。
斗式铲运机通常是功率非常大的机械,许多厂家制造的铲运机铲斗容量为8 m³,满载时可达10 m³。
最大的自行式铲运机铲斗容量为19立方米(满载时为25 m³),由430马力的牵引发动机驱动。
翻斗机可能是使用最为普遍的轮胎式运输设备,因为它们还可以被用来送混凝土或者其他建筑材料。
翻斗车的车斗位于大橡胶轮胎车轮前轴的上方,尽管铰接式翻斗车的卸料方向有很多种,但大多数车斗是向前翻转的。
最小的翻斗车的容量大约为0.5立方米,而最大的标准型翻斗车的容量大约为4.5m³。
特殊型式的翻斗车包括容量为4 m³的自装式翻斗车,和容量约为0.5 m ³的铰接式翻斗车。
必须记住翻斗车与自卸卡车之间的区别。
翻斗车车斗向前倾翻而司机坐在后方卸载,因此有时被称为后卸卡车。
1.3结构的安全度规范的主要目的是提供一般性的设计原理和计算方法,以便验算结构的安全度。
就目前的趋势而言,安全系数与所使用的材料性质及其组织情况无关,通常把它定义为发生破坏的条件与结构可预料的最不利的工作条件之比值。
这个比值还与结构的破坏概率(危险率)成反比。
破坏不仅仅指结构的整体破坏,而且还指结构不能正常的使用,或者,用更为确切的话来说,把破坏看成是结构已经达到不能继续承担其设计荷载的“极限状态”。
通常有两种类型的极限状态,即:(1)强度极限状态,它相当于结构能够达到的最大承载能力。
其例子包括结构的局部屈曲和整体不稳定性;某此界面失效,随后结构转变为机构;疲劳破坏;引起结构几何形状显著变化的弹性变形或塑性变形或徐变;结构对交变荷载、火灾和爆炸的敏感性。
(2)使用极限状态,它对应着结构的使用功能和耐久性。
器例子包括结构失稳之前的过大变形和位移;早期开裂或过大的裂缝;较大的振动和腐蚀。
根据不同的安全度条件,可以把结构验算所采用的计算方法分成:(1)确定性的方法,在这种方法中,把主要参数看作非随机参数。
(2)概率方法,在这种方法中,主要参数被认为是随机参数。
此外,根据安全系数的不同用途,可以把结构的计算方法分为:(1)容许应力法,在这种方法中,把结构承受最大荷载时计算得到的应力与经过按规定的安全系数进行折减后的材料强度作比较。
(2)极限状态法,在这种方法中,结构的工作状态是以其最大强度为依据来衡量的。
由理论分析确定的这一最大强度应不小于结构承受计算荷载所算得的强度(极限状态)。
计算荷载等于分别乘以荷载系数的活载与恒载之和。
把对应于不乘以荷载系数的活载和恒载的工作(使用)条件的应力与规定值(使用极限状态)相比较。
根据前两种方法和后两种方法的四种可能组合,我们可以得到一些实用的计算方法。
通常采用下面两种计算方法:确定性的方法,这种方法采用容许应力。
概率方法,这种方法采用极限状态。
至少在理论上,概率法的主要优点是可以科学的考虑所有随机安全系数,然后将这些随机安全系数组合成确定的安全系数。
概率法取决于:(1)制作和安装过程中材料强度的随机分布(整个结构的力学性能数值的分散性);(2)截面和结构几何尺寸的不确定性(由结构制作和安装造成的误差和缺陷而引起的);对作用在结构上的活载和恒载的预测的不确定性;所采用的近似计算方法有关的不精确性(实际应力与计算应力的偏差)。
此外,概率理论意味着可以基于下面几个因素来确定允许的危险率,例如:建筑物的重要性和建筑物破坏造成的危害性;(2)由于建筑物破坏使生活受到威胁的人数;(3)修复建筑的可能性;(4)建筑物的预期寿命。
所有这些因素均与经济和社会条件有关,例如:(1)建筑物的初始建设费;(2)建筑物使用期限内的折旧费;(3)由于建筑物破坏而造成的物质和材料损失费;(4)在社会上造成的不良影响;(5)精神和心理上的考虑。
就给定的安全系数而论,所有这些参数的确定都是以建筑物的最佳成本为依据的。
但是,应该考虑到进行全概率分析的困难。
对于这种分析来说,应该了解活载及其所引起的盈利的分布规律、材料的力学性能的分散性和截面的结构几何尺寸的分散性。
此外,由于强度的分布规律和应力的分布规律之间的相互关系是困难的。
这些实际困难可以采用两种方法来克服。
第一种方法对材料和荷载采用不同的安全系数,而不需要采用概率准则;第二种方法是引入一些而简化假设的近似概率方法(半概率方法)。
2 外文翻译Abstract:To study the application of continuum structural topology optimization methods to real engineering structures,an optimization method for an optimal topology design of multistory steel frame bracing systems is presented.On a sensitivity analysis,an element removal criterion for continuum structures with stress and multi-displacement constraints under multiple lateral loading conditions is proposed.A concept of mean thickness of a design domain is provided to ensure the reasonableness of optimal results.In the proposed optimization method,the optimal design of an unbraced steel frame without displacement constraints is performed firstly,and then the optimal topology of a bracing system for the multistory steel frame considering displacement constraints is obtained by using evolutionary structural optimization and the given removal criterion,and finally the optima layout ofthe bracing system is interpreted as bracing members.An example of 3-bay 12-story plane steel frame shows that it is effective for the given optimization method in the optimal design of bracing systems for multistory steel frames. Key words:steel frame;bracing system;continuum;topologyoptimization;evolutionary structural optimization2.1 Reinforced ConcretePlain concrete is formed from a hardened mixture of cement ,water ,fine aggregate, coarse aggregate (crushed stone or gravel),air, and often other admixtures. The plastic mix is placed and consolidated in the formwork, then cured to facilitate the acceleration of the chemical hydration reaction lf the cement/water mix, resulting in hardened concrete. The finished product has high compressive strength, and low resistance to tension, such that its tensile strength is approximately one tenth lf its compressive strength. Consequently, tensile andshear reinforcement in the tensile regions of sections has to be provided to compensate for the weak tension regions in the reinforced concrete element.It is this deviation in the composition of a reinforces concrete section from the homogeneity of standard wood or steel sections that requires a modified approach to the basic principles of structural design. The two components of the heterogeneous reinforced concrete section are to be so arranged and proportioned that optimal use is made of the materials involved. This is possible because concrete can easily be given any desired shape by placing and compacting the wet mixture of the constituent ingredients are properly proportioned, the finished product becomes strong, durable, and, in combination with the reinforcing bars, adaptable for use as main members of any structural system.The techniques necessary for placing concrete depend on the type of member to be cast: that is, whether it is a column, a bean, a wall, a slab, afoundation. a mass columns, or an extension of previously placed and hardened concrete. For beams, columns, and walls, the forms should be well oiled after cleaning them, and the reinforcement should be cleared of rust and other harmful materials. In foundations, the earth should be compacted and thoroughly moistened to about 6 in. in depth to avoid absorption of the moisture present in the wet concrete. Concrete should always be placed in horizontal layers which are compacted by means of high frequency power-driven vibrators of either the immersion or external type, as the case requires, unless it is placed by pumping. It must be kept in mind, however, that over vibration can be harmful since it could cause segregation of the aggregate and bleeding of the concrete.Hydration of the cement takes place in the presence of moisture at temperatures above 50°F. It is necessary to maintain such a condition in order that the chemical hydration reaction can take place. If drying is too rapid, surface cracking takes place. This would result in reduction ofconcrete strength due to cracking as well as the failure to attain full chemical hydration.It is clear that a large number of parameters have to be dealt with in proportioning a reinforced concrete element, such as geometrical width, depth, area of reinforcement, steel strain, concrete strain, steel stress, and so on. Consequently, trial and adjustment is necessary in the choice of concrete sections, with assumptions based on conditions at site, availability of the constituent materials, particular demands of the owners, architectural and headroom requirements, the applicable codes, and environmental reinforced concrete is often a site-constructed composite, in contrast to the standard mill-fabricated beam and column sections in steel structures.A trial section has to be chosen for each critical location in a structural system. The trial section has to be analyzed to determine if its nominal resisting strength is adequate to carry the applied factored load. Since more than one trial is often necessary to arrive at the requiredsection, the first design input step generates into a series of trial-and-adjustment analyses.The trial-and –adjustment procedures for the choice of a concrete section lead to the convergence of analysis and design. Hence every design is an analysis once a trial section is chosen. The availability of handbooks, charts, and personal computers and programs supports this approach as a more efficient, compact, and speedy instructional method compared with the traditional approach of treating the analysis of reinforced concrete separately from pure design.2.2 EarthworkBecause earthmoving methods and costs change more quickly than those in any other branch of civil engineering, this is a field where there are real opportunities for the enthusiast. In 1935 most of the methods now in use for carrying and excavating earth with rubber-tyred equipment did not exist. Most earth was moved by narrow rail track, now relatively rare, and the main methods of excavation, with face shovel, backacter, or dragline or grab,though they are still widely used are only a few of the many current methods. To keep his knowledge of earthmoving equipment up to date an engineer must therefore spend tine studying modern machines. Generally the only reliable up-to-date information on excavators, loaders and transport is obtainable from the makers.Earthworks or earthmoving means cutting into ground where its surface is too high ( cuts ), and dumping the earth in other places where the surface is too low ( fills). Toreduce earthwork costs, the volume of the fills should be equal to the volume of the cuts and wherever possible the cuts should be placednear to fills of equal volume so as to reduce transport and double handlingof the fill. This work of earthwork design falls on the engineer who lays out the road since it is the layout of the earthwork more than anything else which decides its cheapness. From the available maps ahd levels, the engineering must try to reach as many decisions as possible in the drawing office by drawing cross sections of the earthwork. On the site when furtherinformation becomes available he can make changes in jis sections and layout,but the drawing lffice work will not have been lost. It will have helped him to reach the best solution in the shortest time.The cheapest way of moving earth is to take it directly out of the cut and drop it as fill with the same machine. This is not always possible, but when it canbe done it is ideal, being both quick and cheap. Draglines, bulldozers and face shovels an do this. The largest radius is obtained with the dragline,and the largest tonnage of earth is moved by the bulldozer, though only over short distances.The disadvantages of the dragline are that it must dig below itself, it cannot dig with force into compacted material, it cannot dig on steep slopws, and its dumping and digging are not accurate.Face shovels are between bulldozers and draglines, having a larger radius of action than bulldozers but less than draglines. They are anle to dig into a vertical cliff face in a way which would be dangerous tor a bulldozer operator andimpossible for a dragline. Each piece of equipment should be level of their tracks and for deep digs in compact material a backacter is most useful, but its dumping radius is considerably less than that of the same escavator fitted with a face shovel.Rubber-tyred bowl scrapers are indispensable for fairly level digging where the distance of transport is too much tor a dragline or face shovel. They can dig the material deeply ( but only below themselves ) to a fairly flat surface, carry it hundreds of meters if need be, then drop it and level it roughly during the dumping. For hard digging it is often found economical to keep a pusher tractor ( wheeled or tracked ) on the digging site, to push each scraper as it returns to dig. As soon as the scraper is full,the pusher tractor returns to the beginning of the dig to heop to help the nest scraper.Bowl scrapers are often extremely powerful machines;many makers build scrapers of 8 cubic meters struck capacity, which carry 10 m ³heaped.The largest self-propelled scrapers are of 19 m ³struck capacity ( 25 m ³ heaped )and they are driven by a tractor engine of 430 horse-powers.Dumpers are probably the commonest rubber-tyred transport since they can also conveniently be used for carrying concrete or other building materials. Dumpers have the earth container over the front axle on large rubber-tyred wheels, and the container tips forwards on most types, though in articulated dumpers the direction of tip can be widely varied. The smallest dumpers have a capacity of about 0.5 m ³, and the largest standard types are of about 4.5 m ³. Special types include the self-loading dumper of up to 4 m ³and the articulated type of about 0.5 m ³. The distinction between dumpers and dump trucks must be remembered .dumpers tip forwards and the driver sits behind the load. Dump trucks are heavy, strengthened tipping lorries, the driver travels in front lf the load and the load is dumped behind him, so they are sometimes called rear-dump trucks.2.3 Safety of StructuresThe principal scope of specifications is to provide general principles and computational methods in order to verify safety of structures. The “ safety factor ”, which according to modern trends is independent of the nature and combination of the materials used, can usually be defined as the ratio between the conditions. This ratio is also proportional to the inverse of the probability ( risk ) of failure of the structure.Failure has to be considered not only as overall collapse of the structure but also as unserviceability or, according to a more precise. Common definition. As the reaching of a “ limit state ” which c auses the construction not to accomplish the task it was designed for. There are two categories of limit state :(1)Ultimate limit sate, which corresponds to the highest value of the load-bearing capacity. Examples include local buckling or global instability of the structure; failure of some sections and subsequent transformation of the structure into a mechanism; failure by fatigue;elastic or plastic deformation or creep that cause a substantial change of the geometry of the structure; and sensitivity of the structure to alternating loads, to fire and to explosions.(2)Service limit states, which are functions of the use and durability of the structure. Examples include excessive deformations and displacements without instability; early or excessive cracks; large vibrations; and corrosion.Computational methods used to verify structures with respect to the different safety conditions can be separated into:(1)Deterministic methods, in which the main parameters are considered as nonrandom parameters.(2)Probabilistic methods, in which the main parameters are considered as random parameters.Alternatively, with respect to the different use of factors of safety, computational methods can be separated into:(1)Allowable stress method, in which the stresses computed under maximum loads are compared with the strength of the material reduced by givensafety factors.(2)Limit states method, in which the structure may be proportioned on the basis of its maximum strength. This strength, as determined by rational analysis, shall not be less than that required to support a factored load equal to the sum of the factored live load and dead load ( ultimate state ).The stresses corresponding to working ( service ) conditions with unfactored live and dead loads are compared with prescribed values ( service limit state ) . From the four possible combinations of the first two and second two methods, we can obtain some useful computational methods. Generally, two combinations prevail:(1)deterministic methods, which make use of allowable stresses.(2)Probabilistic methods, which make use of limit states.The main advantage of probabilistic approaches is that, at least in theory, it is possible to scientifically take into account all random factors of safety, which are then combined todefine the safety factor. probabilistic approaches depend upon :(1) Random distribution of strength of materials with respect to the conditions of fabrication and erection ( scatter of the values of mechanical properties through out the structure );(2) Uncertainty of the geometry of the cross-section sand of the structure ( faults and imperfections due to fabrication and erection of the structure );(3) Uncertainty of the predicted live loads and dead loads acting on the structure;(4)Uncertainty related to the approximation of the computational method used ( deviation of the actual stresses from computed stresses ).Furthermore, probabilistic theories mean that the allowable risk can be based on several factors, such as :(1) Importance of the construction and gravity of the damage by its failure;(2)Number of human lives which can bethreatened by this failure;(3)Possibility and/or likelihood of repairing the structure;(4) Predicted life of the structure.All these factors are related to economic and social considerations such as:(1) Initial cost of the construction;(2) Amortization funds for the duration of the construction;(3) Cost of physical and material damage due to the failure of the construction;(4) Adverse impact on society;(5) Moral and psychological views.The definition of all these parameters, for a given safety factor, allows construction at the optimum cost. However, the difficulty of carrying out a complete probabilistic analysis has to be taken into account. For such an analysis the laws of the distribution of the live load and its induced stresses, of the scatter of mechanical properties of materials, and of the geometry of the cross-sections and the structure have to be known.Furthermore, it is difficult to interpret the interaction between the law of distribution of strength and that of stresses because both depend upon the nature of the material, on the cross-sections and upon the load acting on the structure. These practical difficulties can be overcome in two ways. The first is to apply different safety factors to the material and to the loads, without necessarily adopting the probabilistic criterion. The second is an approximate probabilistic method which introduces some simplifying assumptions ( semi-probabilistic methods ) .。