有机化学英文文献翻译
有机合成英文文献翻译2
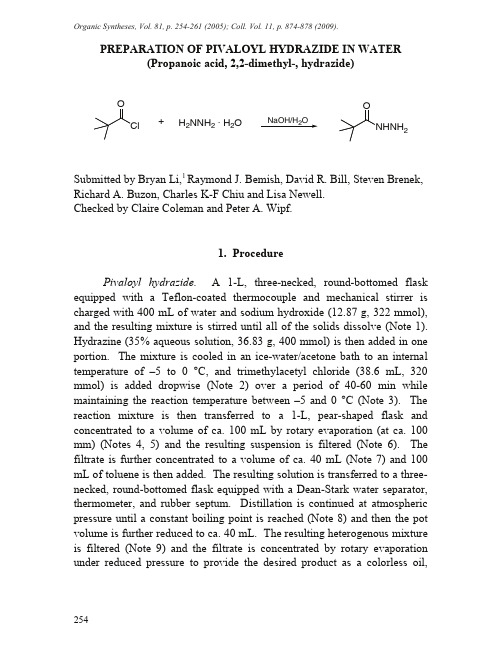
Organic Syntheses, Vol. 81, p. 254-261 (2005); Coll. Vol. 11, p. 874-878 (2009).254 PREPARATION OF PIVALOYL HYDRAZIDE IN WATER (Propanoic acid, 2,2-dimethyl-, hydrazide)2 +NaOH/H2OH2NNH2 · H2OSubmitted by Bryan Li,1Raymond J. Bemish, David R. Bill, Steven Brenek, Richard A. Buzon, Charles K-F Chiu and Lisa Newell.Checked by Claire Coleman and Peter A. Wipf.1. ProcedurePivaloyl hydrazide. A 1-L, three-necked, round-bottomed flask equipped with a T eflon-coated thermocouple and mechanical stirrer is charged with 400 mL of water and sodium hydroxide (12.87 g, 322 mmol), and the resulting mixture is stirred until all of the solids dissolve (Note 1). Hydrazine (35% aqueous solution, 36.83 g, 400 mmol) is then added in one portion. T he mixture is cooled in an ice-water/acetone bath to an internal temperature of –5 to 0 °C, and trimethylacetyl chloride (38.6 mL, 320 mmol) is added dropwise (Note 2) over a period of 40-60 min while maintaining the reaction temperature between –5 and 0 °C (Note 3). T he reaction mixture is then transferred to a 1-L, pear-shaped flask and concentrated to a volume of ca. 100 mL by rotary evaporation (at ca. 100 mm) (Notes 4, 5) and the resulting suspension is filtered (Note 6). T he filtrate is further concentrated to a volume of ca. 40 mL (Note 7) and 100 mL of toluene is then added. The resulting solution is transferred to a three-necked, round-bottomed flask equipped with a Dean-Stark water separator, thermometer, and rubber septum. Distillation is continued at atmospheric pressure until a constant boiling point is reached (Note 8) and then the pot volume is further reduced to ca. 40 mL. The resulting heterogenous mixture is filtered (Note 9) and the filtrate is concentrated by rotary evaporation under reduced pressure to provide the desired product as a colorless oil,255which on sta nding solidifies to a white semi-solid. This ma teria l is recrysta llized from 100 mL of isopropyl ether to a fford 18.6-20.4 g (50-55%) of pivaloyl hydrazide (Notes 10, 11).2. Notes1. All rea gents were purcha sed from Aldrich Chemica l Compa ny (except for trimethyla cetyl chloride, which the checkers obta ined from Acros) and were used without further purification. The checkers used a low temperature alcohol thermometer in place of a Teflon-coated themocouple. The third neck of the flask was left open to the atmosphere.2. A syringe pump was used for the addition of acid chloride in order to achieve a steady flow rate. The tip of the syringe needle (gauge 20) was submerged in the rea ction mixture. Dropwise a ddition of trimethyla cetyl chloride at 0-5 °C resulted in the immediate formation of a precipitate.3. The rea ction wa s complete a t the end of the piva loyl chloride addition. On 5-L or larger scale, the reaction was conducted at temperatures of 10-15 °C without loss of selectivity.4. A small a mount of hydrazine hydrate was present in the reaction mixture a t this point, but a sa fety eva lua tion indica ted the fina l rea ction mixture ha d a very low therma l potentia l (DH=15.3 J/g). This poses a minimum thermal hazard for vacuum distillation.5. The submitters concentra ted the rea ction mixture by va cuum distilla tion (100 mm, ba th tempera ture 70 °C, va por tempera ture 51 °C). The weight a fter concentra tion wa s ca. 120 g. The checkers used rota ry evaporation with a bath temperature of 65 to 70 °C without any problems, and employed an explosion shield as a safety precaution.6. The bis-a cyl a tion byproduct (Me 3CCONHNHCOCMe 3) w a s removed by filtration; 20 mL of water was used for washing the filter cake.7. The submitters removed solvent by vacuum distillation (100 mm, bath temperature 70 °C, vapor temperature 51 °C).8. Azeotropic remova l of wa ter wa s complete when the va por temperature reached 111 °C.9. Sodium chloride was removed by filtration.10.T he submitters obtained the product in 72% yield without recrystallization and determined the product to be >97% pure by HPLC (by area; conditions: 250 mm Kromasil C4 column using acetonitrile (A)/water(B) and 0.1% TFA in water (C), 0:90:10 A:B:C ramp to 90:0:10 A:B:C over15 min and hold for 5 min.Waste Disposal InformationAll toxic materials were disposed of in accordance with “Prudent Practice in the Laboratory”; National Academy Press; Washington, DC, 1995.3. DiscussionHydrazides (RCON HN H2) are highly useful starting materials and intermediates in the synthesis of heterocyclic molecules.2 They can be synthesized by hydrazinolysis of amides, esters and thioesters.3 The reaction of hydrazine with acyl chlorides or anhydrides is also well known,4 but it is complicated by the formation of 1,2-diacylhydrazines, and often requires the use of anhydrous hydrazine which presents a high thermal hazard. Diacylation products predominate when hydrazine reacts with low molecular weight aliphatic acyl chlorides, which makes the reaction impractical for preparatory purposes.5Recently we needed to prepare large amounts of pivaloyl hydrazide (1). A literature survey indicated several approaches: (1) heating pivalic acid with hydrazine hydrate with a Lewis acid catalyst such as activated alumina6 or titanium oxide;7 (2) heating hydrazine hydrate at high temperature (140 °C) with ethyl pivalate;8 (3) condensing phthaloyl hydrazine with pivaloyl chloride, followed by deprotection of the phthaloyl group;9 and (4) reaction of ethyl thiopivalate with hydrazine hydrate. Reaction safety evaluations revealed that hydrazine monohydrate has an onset temperature of ca. 125 °C in a Differential Scanning Calorimetry (DSC) experiment, and possesses a very high thermal potential ( H = 2500 J/g),10,11 which prompted us to develop a method for the synthesis of 1 that did not require heating. After some experimentation we determined that the reaction of pivaloyl chloride with hydrazine proceeds most efficiently in water to give a 4:1 ratio12 of 1 to Me3CCONHNHCOCMe3(2). The use of organic solvents (MeOH, THF, 2-propanol) with water13 invariably led to formation of biphasic mixtures and predominant formation of 2.14 Reaction workup is also simplified using256water as solvent. Upon partial concentration the bis-acylhydrazide byproduct 2precipitated out of the reaction mixture and is convenientlyremoved by filtration. Removal of the remainder of the water bydisplacement with toluene leads to precipitation of NaCl, which is alsoeasily removed by filtration. The filtrate is then further concentrated toprovide 1 in >97% purity, typically in 55–75% yield. This procedure hasbeen employed to prepare 10 Kg batches of 1 with no difficulty.译文:在水相中制备特戊酰氯 (丙酸,2,2-二甲基酰肼)论文由Bryan Li, Raymond J 、Bemish, David R 、Bill, Steven Brenek,Richard A 、Buzon, Charles K-F Chiu 和 Lisa Newell 等发表,经Claire Coleman 与Peter A. Wipf 审核。
ref2-joc有机化学英文文献
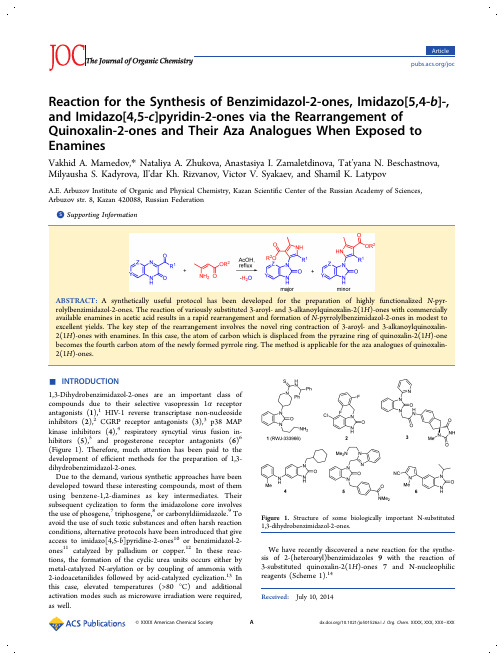
Reaction for the Synthesis of Benzimidazol-2-ones,Imidazo[5,4‑b]‑, and Imidazo[4,5‑c]pyridin-2-ones via the Rearrangement of Quinoxalin-2-ones and Their Aza Analogues When Exposed to EnaminesVakhid A.Mamedov,*Nataliya A.Zhukova,Anastasiya I.Zamaletdinova,Tat’yana N.Beschastnova, Milyausha S.Kadyrova,Il’dar Kh.Rizvanov,Victor V.Syakaev,and Shamil typovA.E.Arbuzov Institute of Organic and Physical Chemistry,Kazan Scientific Center of the Russian Academy of Sciences, Arbuzov str.8,Kazan420088,Russian Federation*Supporting Informationsynthetically useful protocol has been developed for the preparation of highly rolylbenzimidazol-2-ones.The reaction of variously substituted3-aroyl-and3-alkanoylquinoxalin-2(1H)-onesacetic acid results in a rapid rearrangement and formation of N-pyrrolylbenzimidazol-2-oneskey step of the rearrangement involves the novel ring contraction of3-aroyl-andenamines.In this case,the atom of carbon which is displaced from the pyrazine ring ofcarbon atom of the newly formed pyrrole ring.The method is applicable for the aza analoguesINTRODUCTION1,3-Dihydrobenzimidazol-2-ones are an important class ofcompounds due to their selective vasopressin1αreceptorantagonists(1),1HIV-1reverse transcriptase non-nucleosideinhibitors(2),2CGRP receptor antagonists(3),3p38MAPkinase inhibitors(4),4respiratory syncytial virus fusion in-hibitors(5),5and progesterone receptor antagonists(6)6(Figure1).Therefore,much attention has been paid to thedevelopment of efficient methods for the preparation of1,3-dihydrobenzimidazol-2-ones.Due to the demand,various synthetic approaches have beendeveloped toward these interesting compounds,most of themusing benzene-1,2-diamines as key intermediates.Theirsubsequent cyclization to form the imidazolone core involvesthe use of phosgene,7triphosgene,8or carbonyldiimidazole.9Toavoid the use of such toxic substances and often harsh reactionconditions,alternative protocols have been introduced that giveaccess to imidazo[4,5-b]pyridine-2-ones10or benzimidazol-2-ones11catalyzed by palladium or copper.12In these reac-tions,the formation of the cyclic urea units occurs either by metal-catalyzed N-arylation or by coupling of ammonia with 2-iodoacetanilides followed by acid-catalyzed cyclization.13In this case,elevated temperatures(>80°C)and additional activation modes such as microwave irradiation were required, as well.We have recently discovered a new reaction for the synthe-sis of2-(heteroaryl)benzimidazoles9with the reaction of 3-substituted quinoxalin-2(1H)-ones7and N-nucleophilic reagents(Scheme1).14Received:July10,2014Figure 1.Structure of some biologically important N-substituted 1,3-dihydrobenzimidazol-2-ones.The key step of the reaction (Scheme 1)involved a novel acid-catalyzed rearrangement of intermediate spiro-quinoxalin-2(1H )-one derivatives 814b −g with a contraction of the pyrazine ring of the quinoxalin-2-one system.It was also shown that the necessary condition for rearrangement is the presence of at least one mobile hydrogen atom in the spiro-forming fragment,which is responsible for the elimination of water.As can be seen from Scheme 1,the formation of water takes place with the involvement of the oxygen atom of the carbonyl group.We assumed that if the spiro-quinoxalinone derivative with no mobile hydrogen atom in the spiro fragment was subjected to rearrangement,there might probably be two options.The first one is that the rearrangement would not occur at all;the second one is that another rearrangement would take place without any water elimination and with the preservation of the carbonyl oxygen atom,probably quinox-alinone benzimidazolone.To con firm this assumption,it was necessary to synthesize the spiro derivatives of the quinox-alinone without any mobile hydrogen atoms.To this end,after analyzing all of the possible nucleophilic reagents,we have chosen the enamines as CN-nucleophiles.Here,the results of our study on a novel rearrangement of 3-aroyl-and alkanoylquinoxalin-2(1H )-ones when exposed to the commer-cially available enamines (methyl-and ethyl 3-aminocroto-nates)as CN-nucleophiles under acid catalysis condition are presented.■RESULTS AND DISCUSSIONOptimization of Reaction Conditions.To optimize the process,we initially carried out the reaction of 3-benzoylqui-noxalin-2(1H )-one 7a with methyl 3-aminocrotonate 10a in boiling acetic acid with various ratios of reagents (1:1,1:5,and 1:77a /10a )and at di fferent reaction times (Table 1).When the reaction is carried out with the equimolar ratio of the reagents regardless of the reaction time (1,3,6,8,or 12h),no reaction takes place (Table 1,entries 1−5).The use of a 5-fold excess of methyl 3-aminocrotonate 10a leads to the formation of two unexpected regioisomeric products of the rearrangement (11a and 12a )in high overall yield.In these cases,a small amount (1−3%)of unimpaired 3-benzoylquinoxalin-2(1H )-one 7a is reverted regardless of the reaction time (1,3,6,or 8h)(Table 1,entries 6−9).Methyl 3-aminocrotonate 10a apparently undergoes polymerization.The optimal condition for carrying out the investigated reaction appears to be the use of reagents in a ratio of (1:77a /10a )in boiling acetic acid for 6h (Table 1,entry 12).At such reagent ratios,the reaction proceeds successfully for 1h;however,the desired products of the rearrangement are allocated easily if the reaction has been carried out for 6h.This is apparently due to the complete decomposition or polymerization of excess enamine.Synthesis of N -Pyrrolylbenzimidazol-2-ones.Having the optimized reaction conditions at our disposal,we pro-ceeded to explore the scope and limitations of the reaction.The procedure was extended to 3-aroylquinoxalin-2(1H )-ones 7a −ihaving various substituents and methyl-(10a )and ethyl-(10b )3-aminocrotonates.As shown in Table 2,the reaction pro-ceeded very e fficiently and led to the formation of the corresponding N -(pyrrol-3-yl)benzimidazol-2-ones (11)as major and N -(pyrrol-2-yl)benzimidazol-2-ones (12)as minor products with overall yields of 89−99%.The reaction pro-ceeded so fast that we were unable to allocate the expected spiro compound.Under the reaction conditions,they im-mediately rearranged to N -pyrrolylbenzimidazol-2-ones in high yields.The structure of regioisomers 11and 12can be unequivo-cally established by a variety of 1D/2D NMR correlation methods.15,16First,benzimidazole (BI)fragments are revealed from 1H −15N/1H −13C HSQC/HMBC connectivities starting from the NH (BI)protons (see Supporting Information).After that,the structures of pyrrole moieties of 11and 12are discerned from the NMR heteronuclear correlations.Finally,the regioisomeric structure of these compounds has been proven by the NOE data,which makes it possible to correlate the mutual spatial position of pyrrole ly,there are key NOEs between the Ar and NH pyrrole (P)protons in 11and between the Ar and OR (R =Me or Et)protons in 12(e.g.,for 11l and 12l ,see Figure 2).There are also nontrivial NOEs between the OR and H7(BI)protons in 11which are absent in 12.These assignments are also strongly supported by the 1H chemical shift (CS)data;namely,in 11,the OR protons occurred in the shielding zone of the BI aromatic system and as a result resonated at a higher field than in 12.It is interesting to note that pyrrole nitrogen ’s CSs also depend on the regioisomeric structure.While in 11the CSs are ca.162ppm,in 12,its resonances are observed at lower fields (ca.167ppm).Mechanism of the Reaction.On the basis of the known chemistry of imines,17ketones,18enamines,19quinoxalinones,20and the previous reports,14a −f a plausible reaction mechanism for the formation of N -pyrrolylbenzimidazol-2-ones 11andScheme 1.Schematic Presentation of the Rearrangement for the Synthesis of2-(Heteroaryl)benzimidazolesTable 1.Optimization of ReactionConditionsentry ratio of 7a /10atime (h)11a/12a a overall yield 11a +12a (%)11:11NR 21:13NR 31:16NR 41:18NR 51:112NR 61:51 3.4:185b 71:53 3.3:185b 81:56 3.4:187b 91:58 3.3:186b 101:71 3.7:195111:73 3.4:195121:763.5:197aThe ratio was determined by 1H NMR of the crude product.b Traces of 7a were present in the crude product.12has been proposed (Scheme 2).The formation of N -pyrrolylbenzimidazol-2-ones occurs in two di fferent ways (pathway I and pathway II),di ffering in the initial stage of the process.In the case of the formation of N -(pyrrol-3-yl)-benzimidazol-2-ones 11,the reaction starts (Scheme 2,pathway I)with the acid-catalyzed activation of the imine group (of 7a )and subsequent Michael-type reaction 21between 7a and 10a involving a nucleophilic attack by the enamino double bond (of 10a )on the electron-de ficient double bond (of 7a ),which leads to the formation of A .The intra-molecular cyclization of A involving the attack by the imine nitrogen on the nearby −C(O)Ph moiety a ffords the spiro-quinoxaline derivative B .The rearrangement of the spiro-quinoxalinone B is then assumed to occur according to Scheme 2,which proceeds by cascade reactions involving (a)the intramolecular nucleophilic attack by the amino group on the carbonyl group with the intermediate formation of the hydroxy derivative C ,(b)the ring opening with cleavage of the C2−C3bond in the hydroxy derivative C with theelimination of water leading to the formation of the final product 11a .In the case of the formation of N -(pyrrol-2-yl)benzimidazol-2-ones 12a at its initial stage,there occurs a nucleophilic attack by the enamino double bond (of 10a )on the electron-de ficient benzoyl carbonyl group (of 7a )which leads to the formation of D (Scheme 2,pathway II).This brings about the rearrange-ment product via intermediates E and F .Synthesis of the Aza Analogues of N -Pyrrolylbenzi-midazol-2-ones.With this result at our disposal,we went on to study the scope of the methodology with respect to the 5-and 7-aza-quinoxalin-2(1H )-ones,namely,3-benzoylpyrido-[3,2-b ]pyrazin-2(1H )-one 13and 3-benzoylpyrido[3,4-b ]-pyrazin-2(1H )-one 14(Scheme 3).As can be seen,this chem-istry is not limited to the quinoxalin-2(1H )-ones,and the com-pounds composed of two heterocyclic fragments are acceptable substrates,as well.The reactions proceed perfectly well with both 13and 14pyrazin-2(1H )-one derivatives,with the formation of easily separable regioisomeric products 15/16and 17/18with overall quantitative yields.In comparison with the existing methods,the present ap-proach o ffers the following advantages:(i)it proceeds faster and a ffords good to excellent yields under mild conditions with no additional activation modes such as microwave irradiation;(ii)it is very cost-e ffective and uses inexpensive,easy,14a,22and commercially available reagents;and (iii)it is applicable to a broader range of substrates,including 3-aroyl(alkanoyl)-quinoxalin-2(1H )-ones,3-benzoylpyrido[3,2-b ]pyrazin-2(1H )-one,and 3-benzoylpyrido[3,4-b ]pyrazin-2(1H )-one and various enamines.Table 2.Synthesis of N -Pyrrolylbenzimidazol-2-ones 11and12entry 7R 1R 2R 310R 4products (yield)a,boverall yield (11/12)c 17a H H Ph 10a Me 11a (45%)+12a (n/i)97%,78:2227b H H C 6H 4F-410a Me 11b (42%)+12b (n/i)94%,74:2637c H H C 6H 4Cl-410a Me 11c (52%)+12c (n/i)97%,72:2847d H H C 6H 4Br-410a Me 11d (51%)+12d (n/i)97%,70:3057e H H C 6H 4I-410a Me 11e (53%)+12e (n/i)98%,75:2567f H H n -Pr 10a Me 11f (39%)+12f (n/i)97%,91:977g Me Me Ph 10a Me 11g (62%)+12g (n/i)97%,83:1787h C(O)Ph H Ph 10a Me 11h (43%)+12h (n/i)97%,79:2197a H H Ph 10b Et 11i (58%,24%b )+12i (4%)b99%,78:22107b H H C 6H 4F-410b Et 11j (59%)+12j (n/i)96%,77:23117c H H C 6H 4Cl-410b Et 11k (61%,40%b )+12k (5%)b 97%,67:33127d H H C 6H 4Br-410b Et 11l (56%,38%b )+12l (4%)b 99%,67:33137e H H C 6H 4I-410b Et 11m (56%)+12m (n/i)98%,60:40147g Me Me Ph 10b Et 11n (65%)+12n (n/i)99%,84:16157h C(O)Ph H Ph 10b Et 11o (41%)+12o (n/i)98%,72:28167iC(O)OHHPh10bEt11p +12p 89%,75:25(77%)(inseparable mixture)a,bYields refer to isolated products.a Method A.b Method B.See Experimental Section with regard to isolated yields.c Ratio determined by the 1H NMR of the crudeproducts.Figure 2.Optimized (B3LYP/6-31G(d))structures of 11l and 12l with key NOEs (blue arrays).■CONCLUSIONIn conclusion,we have developed an e ffective synthetic strategy via the novel quinoxalin-2(1H )-one/benzimidazol-2-one rear-rangement that permits a rapid access to the N -pyrrolylbenzi-midazol-2-ones from the readily available 3-aroylquinoxalin-2(1H )-ones having various substituents and commercially available enamines (methyl and ethyl 3-aminocrotonates).The methodology is found to be general,and a wide variety of N -pyrrolylbenzimidazol-2-one derivatives are prepared in good yields.Due to the availability of the starting materials and the potential applications of products,this method is highly prospective in organic synthesis and medicinal chemistry.This protocol also represents an extremely simple,e fficient,and metal-free environmentally friendly way to construct substituted pyrroles and benzimidazol-2-ones in overall high yields.Aza analogues of benzimidazol-2-ones can be ob-tained using aza analogues of quinoxalin-2(1H )-one.Thus,itcomplements the method for rapid formation of multifunc-tional heterocycles.■EXPERIMENTAL SECTIONGeneral Information.All NMR experiments were performed with 600,500,and 400MHz (600and 500MHz for 1H NMR;376MHz for 19F NMR;100MHz for 13C NMR;60MHz for 15N NMR,respectively)spectrometers equipped with a 5mm diameter gradient inverse broad band probehead and a pulsed gradient unit capable of producing magnetic field pulse gradients in the z -direction of 53.5G ·cm −1.NMR experiments were carried out at 303K.DPFGROE 23and TOCSY spectra were obtained using a Hermite-shaped pulse for selective excitation.Chemical shifts (δin ppm)are referenced to the solvent DMSO-d 6(δ=2.49ppm for 1H and 39.5ppm for 13C NMR),to external CD 3NO 2(380.2ppm)for 15N NMR spectra (conversion factor to NH 3,−380.2ppm),and to external C 6F 6(−164.9ppm)for 19F NMR spectra.The quantum chemical calculations were performed using a Gaussian 98w software package.24Full geometry optimizations have been carried out within the framework of the DFT (B3LYP)method using 6-31G(d)basis sets.Melting points were determined on a hot-stage apparatus.The IR spectra were recorded on a FT-IR spectrometer.The electron ionization mass spectra (EI-MS)were obtained on gas chromatography mode using “GC MS QP2010”with the following conditions:capillary column =15m;0.25mm;0.25μm “Rxi-1ms ”;carrier gas is helium with constant flow of 3mL/min used;constant temperature of oven =280°C,and injection temperature =280°C.The masses of ions had more than 10%relative intensity on a mass range from 70to 500Da.MALDI experiments (MALDI-MS)were performed with a mass spectrometer equipped with an Nd:YAG laser.The mass spectra were measured in the positive ion linear mode.The data were processed using the software FlexAnalysis 3.0fromScheme 2.Proposed Mechanisms for the Formation of 11a (Pathway I via an Initial Attack on the C3Atom of Quinoxalin-2(1H )-one)and 12a (Pathway II via an Initial Attack on the C Atom of the BenzoylGroup)Scheme 3.Synthesis of N -Pyrrolyl-1H -imidazo[5,4-b ]-(15/16)and N -Pyrrolyl-1H -imidazo[4,5-c ]pyridin-2(3H )-ones (17/18)Bruker Daltonics.The p -nitroaniline was used as a matrix.The dried-droplet spotting technique (matrix,analyte)was applied.For each sample,0.5μL of the analyte solution in DMSO was coated onto a target plate with the matrix solution of 10mg/mL in acetonitrile MTP AnchorChip.General Procedure for the Synthesis of N -Pyrrolylbenzimi-dazol-2-one Derivatives 11a −p/12a −p.A mixture of quinoxalin-2(1H )-one 7(1.0equiv)and enamine 10(7.0equiv)in glacial acetic acid (10mL)was re fluxed for 6h.After the solvent was cooled,it was removed under vacuum and the residue was treated with a 5%NaHCO 3solution (15mL);the precipitate was filtered o ff,washed with water (5×5mL),and dried in air.A mixture of isomers 11a −p /12a −p wasobtained.The individual compounds were isolated according to the following methods.Method A.The mixture of isomers 11/12(with the exception of the mixtures of benzoyl derivatives of methyl and ethyl esters 11h /12h and 11o /12o )was treated with cooled chloroform (2mL);the insoluble residue was fil-tered o ff,washed with chloroform (2×2mL),and dried in air to give the predominant isomers 11a −g and 11i −n in indi-vidual form as analytically pure samples.The same procedure for the isomers 11p /12p results in the isolation of isomers 11p and 12p as an analytically pure mixture in the ratio of 4:1.In the cases of 11h and 11o ,the predominant isomers were allocated by column chromatography on silica gel (0.060−0.200mm,40Å)with a mixture of ethyl acetate/hexane (10:90→70:30)as eluents.Method B.The mixture of isomers 11i /12i ,11k /12k ,and 11l /12l was treated with ether (2×5mL);the precipitate was filtered o ff,dried in air,and re fluxed in toluene (25mL).The insoluble portion was filtered o ffto give the predominant isomers 11i ,11k ,and 11l .The precipitate was filtered o fffrom the filtrate to give the minor isomers 12k and 12l .In the case of processing the mixture of isomers 11i /12i ,the toluene filtrate was evaporated to dryness and the residue was puri-fied by column chromatography on silica gel (0.060−0.200mm,40Å)with a mixture of i -PrOH/hexane (5:95)as eluents.As a result,an analytical pure minor isomer 12i was obtained.1-[(3-Methoxycarbonyl-2-methyl-5-phenyl)pyrrol-4-yl]benzimi-dazol-2(3H)-one (11a )and 1-[(3-Methoxycarbonyl-2-methyl-4-phenyl)pyrrol-5-yl]benzimidazol-2(3H)-one (12a ).Method A:Yield 311mg (45%),11a ,beige solid,mp 310−312°C;1H NMR (600MHz,DMSO-d 6)δ2.53(s,3H,CH 3),3.41(s,3H,OCH 3),6.46(d,J =7.6Hz,1H,H7),6.84(dd,J =7.6,7.6Hz,1H,H6),6.94(dd,J =7.6,7.6Hz,1H,H5),7.00(d,J =7.6Hz,1H,H4),7.20(dd,J =7.6,7.1Hz,1H,H4-Ar),7.30(dd,J =7.6,7.6Hz,2H,H3-Ar,H5-Ar),7.37(d,J =7.6Hz,2H,H2-Ar,H6-Ar),10.83(s,1H,NH-BI),11.96(s,1H,NH-P);13C{1H}NMR (100MHz,DMSO-d 6)δ163.5(C(O)OCH 3),154.6(C2),135.8(C2-P),132.2(C7a),130.2(C1-Ar),128.8(C3a),128.7(C3-Ar,C5-Ar),127.6(C5-P),127.1(C4-Ar),125.3(C2-Ar,C6-Ar),120.9(C5),120.5(C6),113.9(C4-P),108.6(C4),108.6(C3-P),108.0(C7),50.3(OCH 3),13.3(CH 3);15N NMR (60MHz,DMSO-d 6)δ161.6(N1-P),121.2(N1),118.4(N3);IR (KBr)ν3392,3129,3063,1704,1675,1615,1480,1451,1394,1370,1347,1295,1262,1245,1193,1104,763,733,702cm −1;MALDI-MS MH +348.Anal.Calcd for C 20H 17N 3O 3:C,69.15;H,4.93;N,12.10.Found:C,69.01;H,4.85;N,11.90%.1-[(5-(4-Fluorophenyl)-3-methoxycarbonyl-2-methyl)pyrrol-4-yl]-benzimidazol-2(3H)-one (11b )and 1-[(4-(4-Fluorophenyl)-3-methoxycarbonyl-2-methyl)pyrrol-5-yl]benzimidazol-2(3H)-one (12b ).Method A:Yield 286mg (42%),11b ,beige solid,mp 307−309°C;1H NMR (600MHz,DMSO-d 6)δ2.53(s,3H,CH 3),3.41(s,3H,OCH 3),6.46(d,J =7.6Hz,1H,H7),6.84(dd,J =7.6,7.6Hz,1H,H6),6.95(dd,J =7.6,7.1Hz,1H,H5),6.99(d,J =7.6Hz,1H,H4),7.17(dd,J =9.0,9.0Hz,2H,H3-Ar,H5-Ar),7.39(dd,J =9.0,7.4Hz,H2-Ar,H6-Ar),10.85(s,1H,NH-BI),11.96(s,1H,NH-P);13C{1H}NMR (100MHz,DMSO-d 6)δ163.5(C(O)OCH 3),161.2(d,1J CF =244.2Hz,C4-Ar),154.6(C2),135.7(C2-P),132.2(C7a),128.8(C3a),127.4(d,3J CF =8.1Hz,C2-Ar,C6-Ar),126.8(C5-P),126.7(C1-Ar),120.97(C5),120.56(C6),115.7(2J CF =21.6Hz,C3-Ar,C5-Ar),113.8(C4-P),108.7(C4),108.5(C3-P),108.0(C7),50.3(OCH 3),13.3(CH 3);15N NMR (60MHz,DMSO-d 6)δ161.5(N1-P),121.5(N1),118.5(N3);19F{1H}NMR (376MHz,DMSO-d 6)δ−114.4;IR (KBr)ν3406,3117,3061,1702,1678,1618,1516,1481,1455,1370,1262,1239,1191,1100,833,727,700cm −1;EI-MS (m /z (I rel %))366(20),365(77)M +,334(28),333(100),332(29),306(10),305(28),304(36),167(23).Anal.Calcd for C 20H 16FN 3O 3:C,65.75;H,4.41;N,11.50.Found:C,65.96;H,4.52;N,11.33%.1-[(5-(4-Chlorophenyl)-3-methoxycarbonyl-2-methyl)pyrrol-4-yl]-benzimidazol-2(3H)-one (11c )and 1-[(4-(4-Chlorophenyl)-3-methoxycarbonyl-2-methyl)pyrrol-5-yl]benzimidazol-2(3H)-one (12c ).Method A:Yield 349mg (52%),11c ,beige solid,mp 338−341°C;1H NMR (600MHz,DMSO-d 6)δ2.52(s,3H,CH 3),3.41(s,3H,OCH 3),6.44(d,J =7.6Hz,1H,H7),6.84(dd,J =7.6,7.6Hz,1H,H6),6.95(dd,J =7.6,7.6Hz,1H,H5),7.05(d,J =7.6Hz,1H,H4),7.35(d,J =9.0Hz,2H,H2-Ar,H6-Ar),7.36(d,J =9.0Hz,H3-Ar,H5-Ar),10.89(s,1H,NH-BI),12.04(s,1H,NH-P);13C{1H}NMR (100MHz,DMSO-d 6)δ163.5(C(O)OCH 3),154.6(C2),136.2(C2-P),131.9(C7a),131.7(C4-Ar),128.96(C1-Ar),128.77(C3-Ar,C5-Ar,C3a),126.9(C2-Ar,C6-Ar),126.5(C5-P),121.1(C5),120.7(C6),114.4(C4-P),108.8(C4),108.6(C3-P),108.0(C7),50.3(OCH 3),13.3(CH 3);15N NMR (60MHz,DMSO-d 6)δ161.6(N1-P),120.9(N1),118.5(N3);IR (KBr)ν3391,3117,3060,1700,1676,1614,1502,1480,1453,1410,1369,1344,1295,1265,1245,1190,1096,826,724,697cm −1;EI-MS (m /z (I rel %))383(26),382(17),381(74)M +,351(35),350(35),349(100),348(23),323(20),322(16),321(22),320(25),286(10),175(16),166(9),157(11).Anal.Calcd for C 20H 16ClN 3O 3:C,62.91;H,4.22;N,11.01.Found:C,62.97;H,4.29;N,11.10%.1-[(5-(4-Bromophenyl)-3-methoxycarbonyl-2-methyl)pyrrol-4-yl]-benzimidazol-2(3H)-one (11d )and 1-[(4-(4-Bromophenyl)-3-methoxycarbonyl-2-methyl)pyrrol-5-yl]benzimidazol-2(3H)-one (12d ).Method A:Yield 330mg (51%),11d ,beige solid,mp 345−347°C;1H NMR (600MHz,DMSO-d 6)δ2.53(s,3H,CH 3),3.42(s,3H,OCH 3),6.45(d,J =7.6Hz,1H,H7),6.84(dd,J =7.6,7.6Hz,1H,H6),6.95(dd,J =7.6,7.6Hz,1H,H5),7.01(d,J =7.6Hz,1H,H4),7.30(d,J =8.6Hz,2H,H2-Ar,H6-Ar),7.39(d,J =8.6Hz,H3-Ar,H5-Ar),10.87(s,1H,NH-BI),12.03(s,1H,NH-P);13C{1H}NMR (100MHz,DMSO-d 6)δ163.4(C(O)OCH 3),154.5(C2),136.2(C2-P),131.97(C7a),131.67(C3-Ar,C5-Ar),129.4(C1-Ar),128.8(C3a),127.2(C2-Ar,C6-Ar),126.5(C5-P),121.0(C5),120.6(C6),120.1(C4-Ar),114.5(C4-P),108.7(C4,C3-P),108.0(C7),50.3(OCH 3),13.3(CH 3);15N NMR (60MHz,DMSO-d 6)δ161.4(N1-P),120.9(N1),118.4(N3);IR (KBr)ν3387,3117,3059,1700,1676,1613,1500,1479,1453,1406,1369,1344,1295,1264,1190,1098,823,725,704cm −1;EI-MS (m /z (I rel %))441(17),439(16),428(18),427(78)M +,426(17),425(78),409(16),407(118),396(25),395(100),394(41),393(98),392(19),369(23),368(12),367(39),366(28),365(20),364(22),314(10),286(19),184(21),157(52),156(23),135(40),129(17),128(11),123(12),122(15),115(13).Anal.Calcd for C 20H 16BrN 3O 3:C,56.35;H,3.78;N,9.86.Found:C,56.28;H,3.70;N,9.91%.1-[(5-(4-Iodophenyl)-3-methoxycarbonyl-2-methyl)pyrrol-4-yl]-benzimidazol-2(3H)-one (11e )and 1-[(4-(4-Iodophenyl)-3-methoxycarbonyl-2-methyl)pyrrol-5-yl]benzimidazol-2(3H)-one (12e ).Method A:Yield 334mg (53%),11e ,beige solid,mp >350°C;1H NMR (600MHz,DMSO-d 6)δ2.53(s,3H,CH 3),3.42(s,3H,OCH 3),6.45(d,J =7.6Hz,1H,H 7),6.84(dd,J =7.6,7.6Hz,1H,H6),6.95(dd,J =7.6,7.6Hz,1H,H5),7.01(d,J =7.6Hz,1H,H4),7.16(d,J =8.6Hz,2H,H2-Ar,H6-Ar),7.67(d,J =8.6Hz,2H,H3-Ar,H5-Ar),10.87(s,1H,NH-BI),12.00(s,1H,NH-P);13C{1H}NMR (100MHz,DMSO-d 6)δ163.4(C(O)OCH 3),154.5(C2),137.5(C3-Ar,C5-Ar),136.2(C2-P),132.0(C7a),129.7(C1-Ar),128.8(C3a),127.2(C2-Ar,C6-Ar),126.6(C5-P),121.0(C5),120.6(C6),114.5(C4-P),108.7(C3-P),108.7(C4),108.0(C7),92.9(C4-Ar),50.3(OCH 3),13.3(CH 3);15N NMR (60MHz,DMSO-d 6)δ161.4(N1-P),120.9(N1),118.6(N3);IR (KBr)ν3383,3119,3057,1699,1675,1612,1479,1449,1402,1366,1343,1293,1265,1189,1098,819,731,702cm −1;EI-MS (m /z (I rel %))487(37),474(22),473(100)M +,455(31),442(24),441(94),440(12),429(13),415(36),413(15),412(19),315(10),314(14),286(17),157(36),144(10),135(25),129(12),123(12),122(14),115(11).Anal.Calcd for C 20H 16IN 3O 3:C,50.76;H,3.41;N,8.88.Found:C,50.59;H,3.28;N,9.04%.1-[(5-Propyl-3-methoxycarbonyl-2-methyl)pyrrol-4-yl]benzimi-dazol-2(3H)-one (11f )and 1-[(4-Propyl-3-methoxycarbonyl-2-methyl)pyrrol-5-yl]benzimidazol-2(3H)-one (12f ).Method A:Yield 281mg (39%),11f ,beige solid,mp 249−251°C;1H NMR (600MHz,DMSO-d 6)δ0.76(dd,J =7.6,7.1Hz,3H,CH 2CH 2CH 3),1.40−1.48(m,2H,CH 2CH 2CH 3),2.19−2.23(m,2H,CH 2CH 2CH 3),2.42(s,3H,CH 3),3.37(s,3H,OCH 3),6.50(d,J =7.6Hz,1H,H7),6.88(dd,J =7.6,7.1Hz,1H,H6),6.94(dd,J =7.6,7.1Hz,H5),6.98(d,J =7.6Hz,1H,H4),10.73(s,1H,NH-BI),11.37(s,1H,NH-P);13C{1H}NMR (100MHz,DMSO-d 6)δ163.8(C(O)OCH 3),154.4(C2),133.6(C2-P),132.6(C7a),129.1(C5-P),128.5(C3a),120.6(C5),120.4(C6),113.2(C4-P),108.5(C4),107.8(C7),106.7(C3-P),50.0(OCH 3),26.2(CH 2CH 2CH 3),21.8(CH 2CH 2CH 3),13.5(CH 2CH 2CH 3),13.3(CH 3);15N NMR (60MHz,DMSO-d 6)δ164.1(N1-P),121.1(N1),118.0(N3);IR (KBr)ν3355,3174,3133,2957,1702,1674,1617,1482,1467,1397,1269,1248,1192,1166,1095,734cm −1;EI-MS (m /z (I rel %))314(11),313(60)M +,282(11),281(36),280(57),266(33),252(34),224(23),181(13),180(100),148(11),126(30),125(10),120(16).Anal.Calcd for C 17H 19N 3O 3:C,65.16;H,6.11;N,13.41.Found:C,65.05;H,6.02;N,13.26%.5,6-Dimethyl-1-[(3-methoxycarbonyl-2-methyl-5-phenyl)pyrrol-4-yl]benzimidazol-2(3H)-one (11g )and 5,6-Dimethyl-1-[(3-methoxycarbonyl-2-methyl-4-phenyl)pyrrol-5-yl]benzimidazol-2(3H)-one (12g ).Method A:Yield 415mg (62%),11g ,o ff-white solid,mp 256−258°C;1H NMR (600MHz,DMSO-d 6)δ2.06(s,3H,CH 36-BI),2.18(s,3H,CH 35-BI),2.53(s,3H,CH 3),3.43(s,3H,OCH 3),6.27(s,1H,H7),6.79(s,1H,H4),7.19(dd,J =7.6,7.1Hz,1H,H4-Ar),7.30(dd,J =8.1,7.6Hz,2H,H3-Ar,H5-Ar),7.37(d,J =8.1Hz,2H,H2-Ar,H6-Ar),10.61(s,1H,NH-BI),11.91(s,1H,NH-P);13C{1H}NMR (100MHz,DMSO-d 6)δ163.5(C(O)OCH 3),154.7(C2),135.7(C2-P),130.4(C7a),130.2(C1-Ar),128.7(C3-Ar,C5-Ar),128.4(C5),127.9(C6),127.5(C5-P),126.97(C4-Ar),126.85(C3a),125.2(C2-Ar,C6-Ar),114.3(C4-P),109.8(C4),108.97(C7),108.65(C3-P),50.3(OCH 3),19.4(CH 35-BI),19.3(CH 36-BI),13.4(CH 3);15N NMR (60MHz,DMSO-d 6)δ161.3(N1-P),119.8(N1),116.9(N3);IR (KBr)ν3407,3236,3024,1702,1681,1612,1481,1448,1387,1371,1346,1295,1263,1194,1099,755,714cm −1;EI-MS (m /z (I rel %))376(25),375(100)M +,344(13),343(34),342(16),329(22),328(88),314(11),301(10),172(16),150(14).Anal.Calcd for C 22H 21N 3O 3:C,70.38;H,5.64;N,11.19.Found:C,70.28;H,5.71;N,11.13%.5-Benzoyl-1-[(3-methoxycarbonyl-2-methyl-5-phenyl)pyrrol-4-yl]benzimidazol-2(3H)-one (11h )and 5-Benzoyl-1-[(3-methoxy-carbonyl-2-methyl-4-phenyl)pyrrol-5-yl]benzimidazol-2(3H)-one (12h ).Method A:Yield 274mg (43%),11h ,o ff-white solid,mp 261−263°C;1H NMR (600MHz,DMSO-d 6)δ2.53(s,3H,CH 3),3.45(s,3H,OCH 3),6.62(d,J =8.1Hz,1H,H7),7.22(dd,J =7.6,7.1Hz,1H,H4-Ar),7.31(dd,J =7.6,7.6Hz,2H,H3-Ar,H5-Ar),7.34−7.37(m,3H,H2-Ar,H6-Ar,H6),7.44(d,J =1.0Hz,1H,H4),7.52(dd,J =7.6,7.6Hz,2H,H3-Ar ′,H5-Ar ′),7.62(dd,J =7.7,7.6Hz,1H,H4-Ar ′),7.66(d,J =7.1Hz,2H,H2-Ar ′,H6-Ar ′),11.23(s,1H,NH-BI),12.06(s,1H,NH-P);13C{1H}NMR (100MHz,DMSO-d 6)δ195.1(C(O)Ar ′),163.5(C(O)OCH 3),154.8(C2),138.0(C1-Ar ′),136.2(C7a),136.1(C2-P),131.9(C4-Ar ′),130.1(C5),129.9(C1-Ar),129.1(C2-Ar ′,C6-Ar ′),128.8(C3-Ar,C5-Ar),128.7(C3a),128.4(C3-Ar ′,C5-Ar ′),127.9(C5-P),127.4(C4-Ar),125.4(C2-Ar,C6-Ar),124.7(C6),113.1(C4-P),110.0(C4),108.4(C3-P),107.6(C7),50.4(OCH 3),13.3(CH 3);15N NMR (60MHz,DMSO-d 6)δ162.3(N1-P),125.2(N1),119.1(N3);IR (KBr)ν3424,3064,1702,1649,1620,1472,1446,1305,1265,1189,1092,1026,701cm −1;MALDI-MS MH +452.Anal.Calcd for C 27H 21N 3O 4:C,71.83;H,4.69;N,9.31.Found:C,72.04;H,4.77;N,9.15%.1-[(3-Ethoxycarbonyl-2-methyl-5-phenyl)pyrrol-4-yl]benzimi-dazol-2(3H)-one (11i )and 1-[(3-Ethoxycarbonyl-2-methyl-4-phenyl)pyrrol-5-yl]benzimidazol-2(3H)-one (12i ).Method A:Yield 418mg (58%),11i ,o ff-white solid.Method B :Yield 173mg (24%),11i ,o ff-white solid and 29mg (4%),12i ,white solid.Data for 11i :mp 305−307°C;1H NMR (600MHz,DMSO-d 6)δ0.75(t,J =7.1Hz,3H,OCH 2CH 3), 2.54(s,3H,CH 3), 3.82(q,J =7.1Hz,2H,OCH 2CH 3),6.49(d,J =7.6Hz,1H,H7),6.85(ddd,J =7.6,7.6,1.0Hz,1H,H6),6.95(ddd,J =7.6,7.6,1.0Hz,1H,H5),7.00(d,J =7.6Hz,1H,H4),7.20(dd,J =7.6,7.1Hz,1H,H4-Ar),7.30(dd,J =7.6,7.1Hz,2H,H3-Ar,H5-Ar),7.39(d,J =7.1Hz,2H,H2-Ar,H6-Ar),10.85(s,1H,NH-BI),11.94(s,1H,NH-P);13C{1H}NMR (100MHz,DMSO-d 6)δ163.3(C(O)OCH 2CH 3),154.7(C2),136.2(C2-P),132.5(C7a),130.3(C1-Ar),128.9(C3a),128.7(C3-Ar,C5-Ar),127.6(C5-P),127.1(C4-Ar),125.4(C2-Ar,C6-Ar),120.9(C5),120.5(C6),113.6(C4-P),108.9(C3-P),108.6(C4),108.1(C7),58.5(OCH 2CH 3),13.4(OCH 2CH 3),13.1(CH 3);15N NMR (60MHz,DMSO-d 6)δ161.5(N1-P),121.7(N1),118.4(N3);IR (KBr)ν3394,3173,3060,1706,1673,1612,1481,1448,1397,1376,1340,1294,1263,1244,1189,1105,762,733,698cm −1;EI-MS (m /z (I rel %))362(15),361(66)M +,316(30),315(100),314(22),288(11),287(22),286(29),158(10).Anal.Calcd for C 21H 19N 3O 3:C,69.79;H,5.30;N,11.63.Found:C,70.10;H,5.19;N,11.46%.Data for 12i :mp 205−208°C;1H NMR (600MHz,DMSO-d 6)δ1.05(t,J =6.7Hz,3H,OCH 2CH 3), 2.47(s,3H,CH 3), 4.03(q,J = 6.7Hz,2H,OCH 2CH 3),6.55(d,J =7.6Hz,1H,H7),6.83−6.88(m,1H,H6),6.94−6.96(m,2H,H4,H5),7.09(dddd,J =7.1,6.7,1.9,1.9Hz,1H,H4-Ar),7.12−7.19(m,4H,H-Ar),10.97(s,1H,NH-BI),11.90(s,1H,NH-P);13C{1H}NMR (100MHz,DMSO-d 6)δ164.3(C(O)OCH 2CH 3),154.3(C2),134.1(C2-P),133.7(C1-Ar),131.4(C7a),129.3(C2-Ar,C6-Ar),128.1(C3a),127.1(C3-Ar,C5-Ar),126.1(C4-Ar),122.5(C4-P),121.7(C5),120.9(C6),116.7(C5-P),109.4(C3-P),108.9(C4),108.1(C7),58.6(OCH 2CH 3),13.9(OCH 2CH 3),13.2(CH 3);15N NMR (60MHz,DMSO-d 6)δ166.7(N1-P),121.4(N1),118.2(N3);IR (KBr)ν3426,3255,1703,1621,1479,1447,1192,1096,700cm −1;EI-MS (m /z (I rel %))362(24),361(100)M +,333(14),332(39),316(10),315(10),289(14),288(10),286(11),228(12).Anal.Calcd for C 21H 19N 3O 3:C,69.79;H,5.30;N,11.63.Found:C,70.04;H,5.39;N,11.76%.1-[(5-(4-Fluorophenyl)-3-ethoxycarbonyl-2-methyl)pyrrol-4-yl]-benzimidazol-2(3H)-one (11j )and 1-[(4-(4-Fluorophenyl)-3-ethoxy-carbonyl-2-methyl)pyrrol-5-yl]benzimidazol-2(3H)-one (12j ).Meth-od A:Yield 417mg (59%),11j ,beige solid,mp 315−318°C;1H NMR (600MHz,DMSO-d 6)δ0.74(t,J =7.1Hz,3H,OCH 2CH 3),2.54(s,3H,CH 3),3.81(q,J =7.1Hz,2H,OCH 2CH 3),6.49(d,J =8.1Hz,1H,H7),6.85(dd,J =8.1,7.6Hz,1H,H6),6.95(dd,J =7.6,7.6Hz,1H,H5),7.00(d,J =7.6Hz,1H,H4),7.18(dd,J =9.1,8.6Hz,2H,H3-Ar,H5-Ar),7.42(dd,J =9.0,7.4Hz,2H,H2-Ar,H6-Ar),10.86(s,1H,NH-BI),11.95(s,1H,NH-P);13C{1H}NMR (100MHz,DMSO-d 6)δ163.2(C(O)OCH 2CH 3),161.1(d,1J CF =244.6Hz,C4-Ar),154.7(C2),136.1(C2-P),132.4(C7a),128.9(C3a),127.5(d,3J CF =8.4,C2-Ar,C6-Ar),126.8(d,4J CF =3.1,C1-Ar),126.8(C5-P),120.9(C5),120.5(C6),115.7(d,2J CF =21.8,C3-Ar,C5-Ar),113.5(C4-P),108.8(C3-P),108.6(C4),108.0(C7),58.4(OCH 2CH 3),13.3(OCH 2CH 3),13.1(CH 3);15N NMR (DMSO-d 6,60MHz)δ161.7(N1-P),121.5(N1),118.6(N3);19F{1H}NMR (376MHz,DMSO-d 6)δ−114.5;IR (KBr)ν3396,3116,3058,2982,1703,1671,1617,1516,1482,1452,1414,1380,1340,1295,1262,1240,1185,1099,833,727,699cm −1;EI-MS (m /z (I rel %))380(17),379(70)M +,361(27),334(30),333(100),332(23),316(13),315(42),306(13),305(26),304(28),287(10),286(13),167(13).Anal.Calcd for C 21H 18FN 3O 3:C,66.48;H,4.78;N,11.08.Found:C,66.39;H,4.74;N,11.04%.1-[(5-(4-Chlorophenyl)-3-ethoxycarbonyl-2-methyl)pyrrol-4-yl]-benzimidazol-2(3H)-one (11k )and 1-[(4-(4-Chlorophenyl)-3-ethoxycarbonyl-2-methyl)pyrrol-5-yl]benzimidazol-2(3H)-one (12k ).Method A:Yield 424mg (61%),11k ,beige solid.Method B :Yield 278mg (40%),11k ,beige solid and 35mg (5%),12k ,white。
有机合成英语论文原文与翻译

Organic Syntheses, Vol. 82, p. 18-21 (2005); Coll. Vol. 11, p. 778-781 (2009).2,2-DIETHOXY-1-ISOCYANOETHANE(2,2-Diethoxyethyl isocyanide, Isocyanoacetaldehyde diethyl acetal)Submitted by Francesco Amato and Stefano Marcaccini.Checked by Raghuram S. Tangirala and Dennis P. Curran.1. ProcedureCAUTION: All the operations must be conducted in an efficient hood because the isocyanide has an obnoxious odor.A. N-(2,2-Diethoxy)ethyl formamide. A 100-mL round-bottomed flask equipped with a magnetic stir bar and fitted with a reflux condenser is charged with aminoacetaldehyde diethyl acetal (28.34 g, 213 mmol, Note 1) and propyl formate (22.48 g, 255 mmol, Note 2). The resulting clear solution is heated at reflux in an oil bath for 3 h (Note 3). After cooling, the reaction mixture is transferred to a 250-mL round-bottomed flask and freed from the 1-propanol and the unreacted propyl formate by rotary evaporation. The residue is transferred to a distillation apparatus equipped with a 10 cm Vigreux column and a two-necked receiver, and distilled under reduced pressure. After a short forerun, the fraction boiling at 110-111 °C (0.5 mmHg) is collected to give 29.3–29.5 g (86% yield) of N-(2,2-diethoxy)ethyl formamide (Notes 4, 5).B. 2,2-Diethoxy-1-isocyanoethane. A 500-mL one-necked flask equipped with a reflux condenser bearing a CaCl2 trap at the upper end is charged with N-(2,2-diethoxyethyl formamide (24.20 g, 150 mmol), tetrachloromethane (24.61 g, 160 mmol, Note 6), triphenyl phosphine (44.59 g,170 mmol, Note 7), triethylamine (17.20 g, 150 mmol, Note 8), and 150 mL dichloromethane (Note 9). The clear mixture is heated at reflux in an oil bath, and a precipitate (triphenyl phosphine oxide) begins to appear after 20- 30 min. After 3.5 h at reflux, the suspension is cooled to 5 °C and filtered through a Büchner funnel under vacuum from a water aspirator. The collected solid is washed with 50 mL diethyl ether. The filtrate and the washings are combined and evaporated to dryness, and the residue is stirred with a mixture of 100 mL ethyl ether and 100 mL pentanes (Note 10). The resulting suspension is allowed to stand overnight in the freezer (Note 11), and then filtered through a fritted funnel under vacuum with chilling of thecollected filtrate in an ice-sodium chloride bath. The solid residue is washed with 60 mL of pentanes. The filtrate is concentrated on a rotary evaporatorin a fume hood and the residue is transferred to a flask equipped with a short path distillation head and a two-necked receiver. Distillation under reduced pressure gives 13.5–13.8 g (63-64%) of 2,2-diethoxy-1-isocyanoethane (Note 12), bp 60-61 °C at 1 mm Hg, as a colorless, vile-smelling liquid (Notes 13, 14).2. Notes1. Aminoacetaldehyde diethyl acetal was purchased from Aldrich and used as supplied.2. Propyl formate (40 mL, purchased from Aldrich) is stirred with 20 mL aq. 5% NaHCO3 for 2 min. The layers are separated, and the propyl formate layer is washed with 3 x 20 mL of distilled water then dried over magnesium sulfate. After filtration, the filtrate is distilled at atmospheric pressure and the fraction boiling at 80-81 °C is collected for use in Step A.3. The submitters used an electric shell for heating.4. The distillation tends to bump, but the Vigreux column prevents overflow into the receiver.5. IR spectrum (neat) 3304, 1666 cm-1; 1H NMR (300 MHz, CDCl3), the formamide is an 6/1 ratio of amide rotamers in this solvent, major rotamer resonances: 8.21 (s, 1 H, NCHO), 5.81 (broad s, 1 H, NH), 4.52 (t, J = 5.1 Hz, 1 H, O-CH-O), 3.50-3.77 (m, 4 H, CH2CH3), 3.45 (t, J = 5.4 Hz, 2 H, CH2N), 1.29 (t, J = 7.1 Hz, 3 H, CH2CH3), minor rotamer resonances: 8.05 (d, J = 13.5 Hz, 1 H, NCHO), 4.45 (t, J = 6 Hz, 1 H, O-CH-O), 3.31 (t, J = 6 Hz, 2 H, CH2N); 13C NMR (75 MHz, CDCl3) major rotamer resonances: 161.3, 100.2, 62.4, 40.1, 14.9, minor rotamer resonances: 165.0, 101.3, 63.0, 44.3; LRMS (EI) m/z 117 (M – CH3NO, 10%), 103 (100%), 91 (17%), 84 (55%), 75 (77%); HRMS (EI) m/z calcd for C7H16NO2 (M + H) 162.1130, found, 162.1126.6. Tetrachloromethane was purchased from Baker or Fisher and dried over molecular sieves before use.7. Triphenyl phosphine (99%) was purchased from Aldrich or Acros and used as supplied.8. Reagent grade triethylamine (Fluka) was dried over calcium hydride pellets and distilled. The fraction boiling at 89 °C was employed.9. Dichloromethane was stored overnight on 4 Å molecular sieves (submitters) or distilled from calcium hydride (checkers) prior to use.10. The lumps that formed were carefully broken with a spherical- ended glass rod.11. The freezer temperature is about –15 °C. If this operation is omitted, then additional solid that precipitates during the distillation makes this process difficult.12. IR (neat) 2156 cm-1; 1H NMR (300 MHz, CDCl3) 4.64 (t, J = 5.4 Hz, 1 H, O-CH-O), 3.48-3.71 (m, 2 H, CH2CH3), 3.43 (d, J = 5.4 Hz, 2 H, CH2NC), 1.17 (t, J = 7.0 Hz, 3 H, CH2CH3); 13C NMR (75 MHz, CDCl3) 157.8, 99.1, 62.8, 44.3, 14.8.13. The submitters obtained 71-75% yields. The submitters report that 2,2-diethoxy-1-isocyanoethane can be stored for at least two years at –30 °C under nitrogen without appreciable decomposition. The checkers stored a sample at –20 °C for three months, and the resulting liquid was still clear and exhibited a 1H NMR spectrum identical to that recorded on the starting sample.14. The checkers had the impression that this isonitrile smells fouler than phenyl isonitrile and related aryl isonitriles. Glassware can be freed from the isonitrile odor by rinsing with a 1:10 mixture of 37% hydrochloric acid/ethanol.Waste Disposal InformationAll hazardous materials should be handled and disposed of in accordance with "Prudent Practices in the Laboratory"; National Academy Press; Washington, DC, 1995.3. DiscusssionThis synthesis of 2,2-diethoxy-1-isocyanoethane is based on the dehydration of N-substituted formamides, which is the most important route to isocyanides.2 The combination of triphenylphosphine, carbon tetrachloride and triethylamine allows a smooth dehydration and a facile workup. The formylation of aminoacetaldehyde diethyl acetal employs propyl formate, because of the instability of acetals towards acidic reagents such as formic acid and formic-acetic anhydride that are usually employed in N-formylations.Hartke3 reported a synthesis of 2,2-diethoxy-1-isocyanoethane in which amino acetaldehyde acetal was transformed initially into the corresponding thioformamide. The thioformamide was then converted into the isocyanide by treatment with diphenylacetyl chloride/diisopropyl carbodiimide/triethylamine. The present method appears to be more convenient, because the experimental procedures are simpler, the yields are higher (62-68% overall) and the reagents are easily available and cheap.This isocyanide has been employed as C–C–N–C unit in the synthesis of imidazoles, imidazo-imidazoles and aminoisoxazoles.4 2,2-Dimethoxy-1- isocyanoethane can be prepared starting from aminoacetaldehyde dimethyl acetal following this procedure. The yields are similar and the product shows the same reactivity of its ethyl analogues. A procedure for thesynthesis of 2,2-dimethoxy-1-isocyanoethane based on the dehydration ofthe corresponding formamide with POCl3/NEt3 has recently been reported.5 The dehydration with PPh3/CCl4/NEt3 appears to be a superior method,giving better yields without employing a tedious aqueous work-up. 2,2- Dimethoxy-1-isocyanoethane has been used for the synthesis of 4,5- dihydrothiazoles and thiazoles.译文:2,2-二乙氧基-1-异氰基乙烷的合成(2,2-二乙氧基乙基异腈,异氰基乙醛缩二乙醇)论文由Francesco Amato 和Stefano Marcaccini发表,经Raghuram S. Tangirala 和Dennis P. Curran审核。
双语有机化学Carboylic Acids and Their Drivatives..
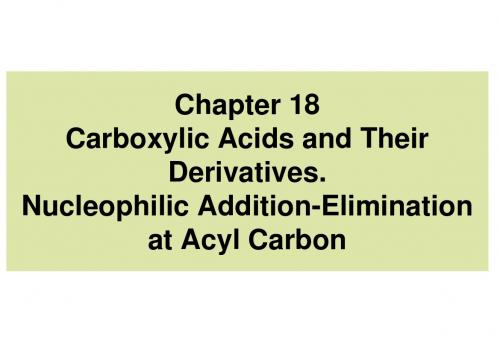
Some straight chain acids
Water Solubility (g 100mL-1 H2O) Structure
HCO2H CH3CO2H CH3CH2CO2H CH3(CH2)2CO2H CH3(CH2)3CO2H CH3(CH2)4CO2H CH3(CH2)6CO2H CH3(CH2)8CO2H CH3(CH2)10CO2H CH3(CH2)12CO2H CH3(CH2)14CO2H CH3(CH2)16CO2H
pKa
3.7 4.7 4.8 4.8 4.8 4.8 4.8 4.8 5.3 6.4 6.4
• Positions of substituents on the chain are labeled with Greek letters. • Carbon adjacent to the carbonyl carbon is α-carbon, the carbon adjacent to α-carbon is βcarbon, and so on.
O
6 5 4 3 2
6
CH3CH2CHCH2CH2COH CH3
4-Methylhexanoic acid
5
4
3
2
O
CH3CH
CHCH2CH2COH
4-Hexenoic acid (or hex-4-enoic acid)
•
The common names for many carboxylic acids remain in use
Structure
• Carbon is sp2 hybridized. • Bond angles are close to 120. • O-H eclipsed with C=O, to get overlap of orbital with orbital of lone pair on oxygen.
英文介绍有机化学
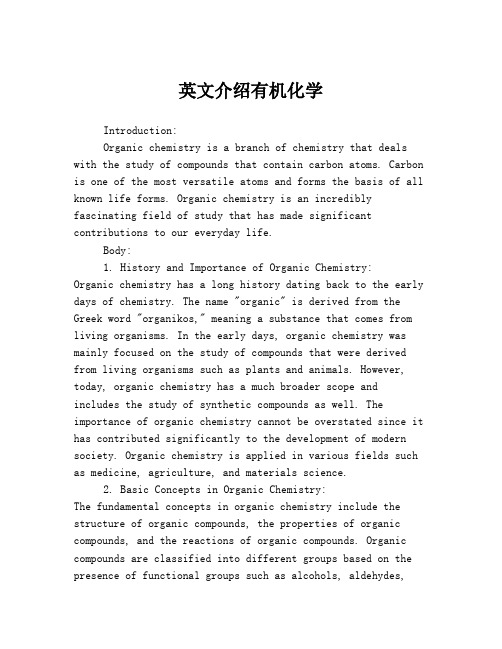
英文介绍有机化学Introduction:Organic chemistry is a branch of chemistry that deals with the study of compounds that contain carbon atoms. Carbon is one of the most versatile atoms and forms the basis of all known life forms. Organic chemistry is an incredibly fascinating field of study that has made significant contributions to our everyday life.Body:1. History and Importance of Organic Chemistry:Organic chemistry has a long history dating back to the early days of chemistry. The name "organic" is derived from the Greek word "organikos," meaning a substance that comes from living organisms. In the early days, organic chemistry was mainly focused on the study of compounds that were derived from living organisms such as plants and animals. However, today, organic chemistry has a much broader scope and includes the study of synthetic compounds as well. The importance of organic chemistry cannot be overstated since it has contributed significantly to the development of modern society. Organic chemistry is applied in various fields such as medicine, agriculture, and materials science.2. Basic Concepts in Organic Chemistry:The fundamental concepts in organic chemistry include the structure of organic compounds, the properties of organic compounds, and the reactions of organic compounds. Organic compounds are classified into different groups based on the presence of functional groups such as alcohols, aldehydes,ketones, esters, and ethers. The properties of organic compounds such as solubility, boiling point, and meltingpoint depend on the type of functional group present in the molecule. Organic reactions are classified into different categories such as substitution, addition, and elimination reactions.3. Organic Chemistry and its Applications:Organic chemistry has numerous applications in different fields, some of which include:- Medicine: Organic chemistry is used in the development of drugs and medicines. Most drugs are organic compounds that are synthesized in the laboratory.- Agriculture: Organic chemistry is used in the development of pesticides and fertilizers. Organic compounds such as insecticides and herbicides are used to control pests and weeds in agriculture.- Materials Science: Organic compounds are used in the production of different materials such as plastics, fibers, and adhesives.- Petroleum Industry: Organic chemistry is used in the refining of crude oil to produce fuels such as gasoline and diesel.4. Future Developments in Organic Chemistry:The field of organic chemistry is constantly evolving, and there are numerous ongoing developments in the field. Some of the future developments in organic chemistry include: - Green Chemistry: Green chemistry is the development of sustainable processes that minimize the use of toxic substances and reduce waste.- Nanotechnology: Organic compounds are used in the development of nanotechnology devices such as sensors andelectronics.- Biofuels: Organic chemistry is used in the production of biofuels such as ethanol and biodiesel.Conclusion:In conclusion, organic chemistry is a fascinating field of study that has significant applications in different areas of our everyday lives. The field is constantly evolving with new developments, and its importance to society cannot be overstated. As we move forward, organic chemistry will continue to play a vital role in the development of new materials, drugs, and technologies.。
化学专业英语文献翻译
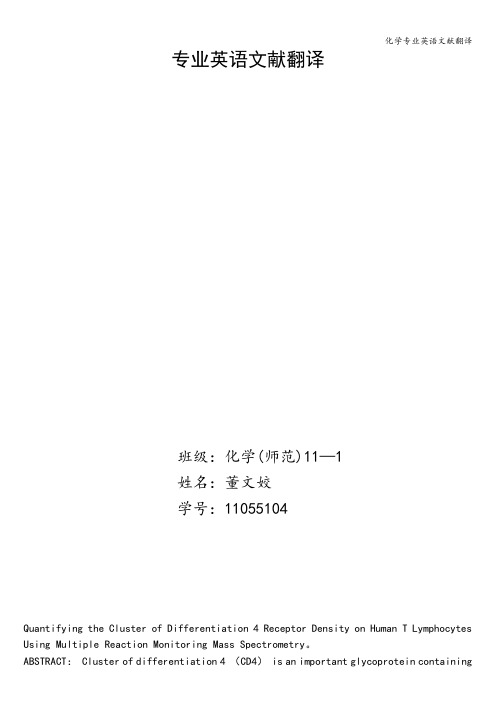
专业英语文献翻译Quantifying the Cluster of Differentiation 4 Receptor Density on Human T Lymphocytes Using Multiple Reaction Monitoring Mass Spectrometry。
ABSTRACT: Cluster of differentiation 4 (CD4) is an important glycoprotein containingfour extracellular domains, a transmembrane portion and a short intracellular tail. It locates on the surface of various types of immune cells and performs a critical role in multiple cellular functions such as signal amplification and activation of T cells。
It is well-known as a clinical cell surface protein marker for study of HIV progression and for defining the T helper cell population in immunological applications。
Moreover,CD4 protein has been used as a biological calibrator for quantification of other surface and intracellular proteins. However,flow cytometry, the conventional method of quantification of the CD4 density on the T cell surface depends on antibodies and has suffered from variables such as antibody clones, the ommatophore and conjugation chemistries, the fixation conditions, and the flow cytometric quantification methods used. In this study, we report the development of a highly reproducible na no liquid chromatography−multiple reaction monitoring mass spectrometry-based quantitative method to quantify the CD4 receptor density in units of copy number per cell on human CD4+ T cells. The method utilizes stable isotope—labeled full—length standard CD4 as an internal standard to measureendogenous CD4 directly, without the use of antibodies. The development of the mass spectrometry-based approach of CD4 protein quantification is important as a complementary strategy to validate the analysis from the cytometry-based conventional method。
有机化学 chinese journal of organic chemistry
有机化学chinese journal of organic chemistry 《有机化学》(Chinese Journal of Organic Chemistry)是中国化学会主办的一本有机化学领域的学术期刊。
该期刊于1981年创刊,目前以英文版发行。
其主要目标是促进和推动有机化学领域的研究,包括有机物的合成方法、反应机理、结构与活性关系等方面。
该期刊涵盖了广泛的研究领域,包括有机合成化学、新材料合成、催化剂设计、药物化学、生物有机化学等。
它为国内外的研究人员提供了一个交流和分享最新研究成果的平台。
《有机化学》期刊以严谨的学术质量和高水平的论文发表而闻名,经过同行评审的严格审稿流程确保了所发表的文章的科学性和可靠性。
它在国际学术界具有很高的声誉和影响力,并获得了许多国内外学术奖项和荣誉。
作为有机化学领域的重要期刊,《有机化学》鼓励学者和科研工作者积极投稿,并提供了一个展示研究成果的平台。
它不仅为学术界提供了一个交流和合作的机会,也为广大读者提供了最新的研究进展和前沿知识。
《有机化学》是中国有机化学领域的重要学术期刊,对推动和促进该领域的研究具有重要意义。
化学毕业论文英文献及翻译
化学毕业论文英文献及翻译负载水杨醛1,3丙二酸二异丙酯二亚胺(BSPDI)的活性炭分离富集食物样品中某些重金属——火焰原子吸收光谱法测定摘要:在已有的报导中有一种灵敏而又简单的方法,能同时富集实际样品3+3+2+2+2+2+中的Cr、Fe、Cu、Ni、Co和Zn。
在该方法的基础上,将BSPDI 负载-1-1到活性炭上,再用8ml 2mol.L的硝酸的丙酮溶液或10ml 4mol.L的硝酸溶液对改性的活性炭洗提后吸附金属。
经调查分析,包括采样体积和PH值都是影响结果的分析参数。
检测分析物的残留物上的基质离子的影响,通常分析物的回收率是能测定的。
该方法已成功地应用于对一些食物样品中某些金属的内容评价。
1、介绍在包括自然水域的环境样品中,对微量金属的测定是为了表明生态污染程度和有关健康问题。
通常先确定所分离的元素是样品的主要组成部分,而后才涉及到时对这些微量组成的分离富集。
对微量金属的分析存在于各种样品中,像自然界的废水,泥沙和一些分析技术能直接测定的组织。
比如由于其他离子的基质影响、样品中金属离子的浓度转低和选择性低灵敏性低的分析技术而使原子吸收方法就不能用来真接测定。
事实上,固相萃取是一个强大的工具,能分离富集各种无机有机分析物。
在技术上它有风几个优点:固相稳定性好,可重复性好,能达到较高的富集效果,分离富集的动力条件温和,无需特殊的有机溶剂,试剂消耗低产生费用小。
几个选择性的用物理负载或化学绑定的鳌合剂等不同载体的固相萃取物已经准备好,例如:硅胶,活性炭,涂SDS氧化铝,改性硅藻土载体,标记离子聚合物,XAD-2000安伯来树脂。
活性炭是一种广泛应用在水、高纯度物质、蔬菜样品等分析物的多元微量富集的收集物。
一般使用活性炭对金属富集的方法是通过简单调整水溶液的PH到适当的值后与金属进行螯合,此过程的缺点是它需要一系列的络合步骤,因此,最近提出使用螯合活性炭。
金属螯合物可以提供高选择性和高富集效果,寻找这样一种分离富集技术。
有机化学专业英语中英文对照
有机化学专业英语中英文对照第一章无机化学英文命名1.1元素的英文命名S-block ElementIAH Hydrogen Li Lithium Na Sodium K PotassiumRb Rubidium Cs Cesium Fr FranciumIIABe Beryllium Mg Magnesium Ca Calcium Sr Strontium Ba Barium Ra RadiumP-block ElementIIIA IV A V AB BoronC Carbon N NitrogenAl Aluminium Si Silicon P PhosphorusGa Gallium Ge Germanium As ArsenicIn Indium Sn Tin Sb AntimonyTl Thallium Pb Lead Bi BismuthVIA VIIA0He Helium O Oxygen F Fluorine Ne NeonS Sulfur Cl Chlorine Ar ArgonSe Selenium Br Bromine Kr KryptonTe Tellurium I Iodine Xe XenonPo Polonium At Astatine Rn RadonCommon Transition ElememtFe:iron Mn:manganese Cu:copper Zn:zincHg:mercury Ag:silver Au:gold1.2离子、氧化物、酸、碱、盐及其他化合物的英文命名Na+Sodium Al3+AluminumK+Potassium Ca2+CalciumFe2+Iron(II)or FerrousFe3+Iron(III)or FerricCr2+Chromium(II)Cr3+Chromium(III)Mn4+Manganese(IV)Mn2+Manganese(II)FeO:iron(II)oxide或ferrous oxideFe2O3:iron(III)oxide或ferric oxideCu2O:copper(I)oxide或cuprous oxideCuO:copper(II)oxide或cupric oxideCl-Chloride O=OxideBr-Bromide OH-HydroxideI-Iodide CN-CyanideS=Sulfide H-Hydride HF hydrogen fluoride HCl hydrogen chloride HBr hydrogen bromide HI hydrogen iodideH2S hydrogen sulfide H2Se hydrogen selenideH2Te hydrogen telluridePH3:phosphine或phosphane AsH3:arsine或arsaneSbH3:stibine或stibane BiH3:bismuthaneCH4:methane SiH4:silaneB2H6:diboraneHCl:hydrochloric acidH2S:hydrosulfuric acidAl(OH)3Aluminum hydroxideNaOH Sodium hydroxideCa(OH)2Calcium hydroxideBa(OH)2Barium hydroxideCo(OH)2Cobalt(II)hydroxideHgSO4Mercury(II)sulfateHg2SO4Mercury(I)sulfateKNO3Potassium nitrateNa2CO3Sodium carbonateNaClO Sodium hypochloriteFeSO4iron(II)sulfateKMnO4potassium permanganateNaHSO4Sodium hydrogen sulfateNa2HPO4Disodium hydrogen phosphateNaH2PO4Sodium dihydrogen phosphateCa(HSO4)2Calcium bisulfateNaHCO3Sodium hydrogencarbonate或Sodium bicarbonateAlCl3·6H2O:aluminum chloride6-water或aluminum chloride hexahydrateAlK(SO4)2·12H2O:aluminium potassium sulfate12-waterH2CO3Carbonic acidH2SO4Sulfuric acidH3PO4Phosphoric acidHNO3Nitric acidHClO4Perchloric acidHCl Hydrochloric acidH2SO3Sulfurous acidH3PO3Phosphorous acidHNO2Nitrous acidHClO Hypochlorous acidHClO2Chlorous acidNaming coordination complexLigandsCN-Cyano NO2-NitroF-Fluoro NO3-NitratoCl-Chloro CO3=CarbonatoBr-Bromo CH3COO-AcetatoO=Oxo H-HydridoOH-Hydroxo-O2CCO2-Oxalato1.3配位化学中常见配体的名称1.4常用无机化学专业英语词汇相对原子质量relative atomic mass消去反应elimination reaction硝化反应nitratlon reaction硝酸钡barium nitrate硝酸银silver nitrate溴的四氯化碳溶液solution of bromine in carbon tetrachloride溴化钠sodium bromide溴水bromine water溴水bromine water盐类的水解hydrolysis of salts盐析salting-out焰色反应flame test氧化剂oxidizing agent氧化铝aluminium oxide氧化铁iron(III)oxide 乙醇ethanol乙醛ethana1乙炔ethyne乙酸ethanoic acid乙酸乙酯ethyl acetate乙烯ethene银镜反应silver mirror reaction硬脂酸stearic acid油脂oils and fats有机化合物organic compound元素周期表periodic table of elements 元素周期律periodic law of elements 原电池primary battery原子序数atomic number皂化反应saponification粘合剂adhesive蔗糖sucrose指示剂Indicator酯ester酯化反应esterification周期period族group(主族:main group)Bunsen burner本生灯product化学反应产物flask烧瓶apparatus设备PH indicator PH值指示剂,氢离子(浓度的)负指数指示剂matrass卵形瓶litmus石蕊litmus paper石蕊试纸graduate,graduated flask量筒,量杯reagent试剂test tube试管burette滴定管retort曲颈甑still蒸馏釜cupel烤钵crucible pot,melting pot坩埚pipette吸液管filter滤管stirring rod搅拌棒element元素body物体compound化合物atom原子gram atom克原子atomic weight原子量atomic number原子数atomic mass原子质量molecule分子electrolyte电解质ion离子anion阴离子cation阳离子electron电子isotope同位素isomer同分异物现象polymer聚合物symbol复合radical基structural formula分子式valence,valency价monovalent单价bivalent二价halogen成盐元素bond原子的聚合mixture混合combination合成作用compound合成物alloy合金organic chemistry有机化学inorganic chemistry无机化学derivative衍生物series系列acid酸hydrochloric acid盐酸sulphuric acid硫酸nitric acid硝酸aqua fortis王水fatty acid脂肪酸organic acid有机酸hydrosulphuric acid 氢硫酸hydrogen sulfide氢化硫alkali碱,强碱ammonia氨base碱hydrate水合物hydroxide氢氧化物,羟化物hydracid氢酸hydrocarbon碳氢化合物,羟anhydride酐alkaloid生物碱aldehyde醛oxide氧化物phosphate磷酸盐acetate醋酸盐methane甲烷,沼气butane丁烷salt盐potassium carbonate碳酸钾soda苏打sodium carbonate碳酸钠caustic potash苛性钾caustic soda苛性钠ester酯gel凝胶体analysis分解fractionation分馏endothermic reaction吸热反应exothermic reaction放热反应precipitation沉淀to precipitate沉淀to distil,to distill蒸馏distillation蒸馏to calcine煅烧to oxidize氧化alkalinization碱化to oxygenate,to oxidize脱氧,氧化to neutralize中和to hydrogenate氢化to hydrate水合,水化to dehydrate脱水fermentation发酵solution溶解combustion燃烧fusion,melting熔解alkalinity碱性isomerism,isomery同分异物现象hydrolysis水解electrolysis电解electrode电极anode阳极,正极cathode阴极,负极catalyst催化剂catalysis催化作用oxidization,oxidation氧化reducer还原剂dissolution分解synthesis合成reversible可逆的摩尔mole摩尔质量molar mass品红magenta或fuchsine葡萄糖glucose气体摩尔体积molar volume of gas 铅蓄电池lead storage battery强电解质strong electrolyte氢氟酸hydrogen chloride氢氧化铝aluminium hydroxide取代反应substitution reaction醛aldehyde炔烃alkyne燃料电池fuel cell弱电解质weak electrolyte石油Petroleum水解反应hydrolysis reaction四氯化碳carbon tetrachloride塑料plastic塑料的降解plastic degradation塑料的老化plastic ageing酸碱中和滴定acid-base neutralization titration酸雨acid rain羧酸carboxylic acid碳酸钠sodium carbonate碳酸氢铵ammonium bicarbonate碳酸氢钠sodium bicarbonate糖类carbohydrate烃hydrocarbon烃的衍生物derivative of hydrocarbon烃基hydrocarbonyl同分异构体isomer同素异形体allotrope同位素isotope同系物homo1og涂料coating烷烃alkane物质的量amount of substance物质的量浓度amount-of-substance concentration of B烯烃alkene洗涤剂detergent纤维素cellulose相对分子质量relative molecular mass 极性键polar bond加成反应addition reaction加聚反应addition polymerization甲烷methane碱金属alkali metal碱石灰soda lime结构式structural formula聚合反应po1ymerization可逆反应reversible reaction空气污染指数air pollution index勒夏特列原理Le Chatelier's principle离子反应ionic reaction离子方程式ionic equation离子键ionic bond锂电池lithium cell两性氢氧化物amphoteric hydroxide两性氧化物amphoteric oxide裂化cracking裂解pyrolysis硫氰化钾potassium thiocyanate硫酸钠sodium sulphide氯化铵ammonium chloride氯化钡barium chloride氯化钾potassium chloride氯化铝aluminium chloride氯化镁magnesium chloride氯化氢hydrogen chloride氯化铁iron(III)chloride氯水chlorine water麦芽糖maltose煤coal酶enzyme二氧化氮nitrogen dioxide二氧化硅silicon dioxide二氧化硫sulphur dioxide二氧化锰manganese dioxide芳香烃arene放热反应exothermic reaction非极性分子non-polar molecule非极性键non-polar bond肥皂soap分馏fractional distillation酚phenol复合材料composite干电池dry cell干馏dry distillation甘油glycerol高分子化合物polymer共价键covalent bond官能团functional group光化学烟雾photochemical fog过氧化氢hydrogen peroxide合成材料synthetic material合成纤维synthetic fiber合成橡胶synthetic rubber核电荷数nuclear charge number核素nuclide化学电源chemical power source化学反应速率chemical reaction rate氨ammonia氨基酸amino acid铵盐ammonium salt饱和链烃saturated aliphatic hydrocarbon苯benzene变性denaturation不饱和烃unsaturated hydrocarbon超导材料superconductive material臭氧ozone醇alcohol次氯酸钾potassium hypochlorite醋酸钠sodium acetate蛋白质protein氮族元素nitrogen group element碘化钾potassium iodide碘化钠sodium iodide电化学腐蚀electrochemical corrosion电解质electrolyte电离平衡ionization equilibrium电子云electron cloud淀粉starch淀粉碘化钾试纸starch potassium iodide paper化学键chemical bond化学平衡chemical equilibrium 还原剂reducing agent磺化反应sulfonation reaction霍尔槽Hull Cell极性分子polar molecule 2.5常用无机元素英文Actinium(Ac)锕Aluminium(Al)铝Americium(Am)镅Antimony(Sb)锑Argon(Ar)氩Arsenic(As)砷Astatine(At)砹Barium(Ba)钡Berkelium(Bk)锫Beryllium(Be)铍Bismuth(Bi)铋Boron(B)硼Bromine(Br)溴Cadmium(Cd)镉Caesium(Cs)铯Calcium(Ca)钙Californium(Cf)锎Carbon(C)碳Cerium(Ce)铈Chlorine(Cl)氯Chromium(Cr)铬Cobalt(Co)钴Copper(Cu)铜Curium(Cm)锔Dysprosium(Dy)镝Einsteinium(Es)锿Erbium(Er)铒Europium(Eu)铕Fermium(Fm)镄Fluorine(F)氟Francium(Fr)钫Gadolinium(Gd)钆Gallium(Ga)镓Germanium(Ge)锗Gold(Au)金Hafnium(Hf)铪Helium(He)氦Holmium(Ho)钬Hydrogen(H)氢Indium(In)铟Iodine(I)碘Iridium(Ir)铱Iron(Fe)铁Krypton(Kr)氪Lanthanum(La)镧Lawrencium(Lr)铹Lead(Pb)铅Lithium(Li)锂Lutetium(Lu)镥Magnesium(Mg)镁Manganese(Mn)锰Mendelevium(Md)钔Mercury(Hg)汞Molybdenum(Mo)钼Neodymium(Nd)钕Neon(Ne)氖Neptunium(Np)镎Nickel(Ni)镍Niobium(Nb)铌Nitrogen(N)氮Nobelium(No)锘Osmium(Os)锇Oxygen(O)氧Palladium(Pd)钯Phosphorus(P)磷Platinum(Pt)铂Plutonium(Pu)钚Polonium(Po)钋Potassium(K)钾Praseodymium(Pr)镨Promethium(Pm)钷Protactinium(Pa)镤Radium(Ra)镭Radon(Rn)氡Rhenium(Re)铼Rhodium(Rh)铑Rubidium(Rb)铷Ruthenium(Ru)钌Samarium(Sm)钐Scandium(Sc)钪Selenium(Se)硒Silicon(Si)硅Silver(Ag)银Sodium(Na)钠Strontium(Sr)锶Sulphur(S)锍Tantalum(Ta)钽Technetium(Tc)锝Tellurium(Te)碲Terbium(Tb)铽Thallium(Tl)铊Thorium(Th)钍Tin(Sn)锡Thulium(Tm)铥Titanium(Ti)钛Tungsten(W)钨Uranium(U)铀Vanadium(V)钒Xenon(Xe)氙Ytterbium(Yb)镱Yttrium(Y)钇Zinc(Zn)锌Zirconium(Zr)锆第二章有机化学英文命名2.1有机化学系统命名中主要官能团的优先顺序1.鎓盐(onium and similar cations)2.酸(acids):按COOH、(C)OOH次序,然后是它们的S和Se的衍生物,再次为磺酸、亚磺酸等。
有机化学中英文对照表
有机化学中英文对照表有机化学可以直接下载WORD版本英文名汉文名Angular methyl group 角甲基Alkylidene group 亚烷基Allyl group 烯丙基Allylic 烯丙型[的]Phenyl group 苯基Aryl group 芳基Benzyl group 苄基Benzylic 苄型[的]Activating group 活化基团Chromophore 生色团Auxochrome 助色团Magnetically anisotropic group 磁各向异性基团Smally ring 小环Common ring 普通环Medium rimg 中环Large ring 大环Bridged-ring system 桥环体系Spiro compound 螺环化合物Helical molecule 螺旋型分子Octahedral compound 八面体化合物Conjugation 共轭Conjugated-system 共轭体系Acyl cation 酰[基]正离子Benzylic cation 苄[基]正离子Arenirm ion 芳[基]正离子Ketyl radical 羰自由基Radical ion 自由基离子Radical cation 自由基正离子Radical anion 自由基负离子Isomerism 异构[现象]Aci form 酸式Fluxional structure 循变结构Stereochemistry 立体化学Optical activity 光学活性Dextro isomer 右旋异构体Laevo isomer 左旋异构体Tetrahedral configuration 四面体构型Stereoisomerism 立体异构[现象] Asymmetric atom 不对称原子Asymmetric carbon 不对称碳Pseudoasymmetric carbon 假不对称碳Phantom atom 虚拟原子Homotopic 等位[的]Heterotopic 异位[的]Enantiotopic 对映异位[的] Diastereotopic 非对映异位[的] Configuration 构型Absolute configuration 绝对构型Chirality 手性Chiral 手性[的]Chiral center 手性中心Chiral molecule 手性分子Achiral 非手性[的]Fischer projection 费歇尔投影式Neoman projection 纽曼投影式D-L system of nomenclature D-L命名体系R-S syytem of nomenclature R-S命名体系Cahn-Ingold-Prelon sequence 顺序规则Symmetry factor 对称因素Plane of symmetry 对称面Mirror symmetry 镜面对称Enantiomer 对映[异构]体Diastereomer 非对映[异构]体Epimer 差向异构体Anomer 端基[差向]异构体Erythro configuration 赤型构型Erythro isomer 赤型异构体Threo configuration 苏型构型Threo isomer 苏型异构体Trigonal carbon 三角型碳Cis-trans isomerism 顺反异构E isomer E异构体Z isomer Z异构体Endo isomer 内型异构体Exo isomer 外型异构体Prochirality 前手性Pro-R group 前R基团Pro-S proup 前S基团Re face Re面Si face Si面Racemic mixture 外消旋混合物Racemic compound 外消旋化合物Racemic solid solution 外消旋固体溶液Meso compound 内消旋化合物Quasi recemate 准外消旋体Conformation 构象Conformational 构象分析Torsion angle 扭转角Rotamer 旋转异构体Anti conformation 反式构象Bisecting conformation 等分构象Anti periplanar conformation 反叠构象Synperiplanar conformation 顺叠构象Synclinal conformation 反错构象Synclinal conformation 顺错构象Eclipsed conformation 重叠构象Gauche conformation, skew con-formation 邻位交叉构象Staggered conformation 对位交叉构象Steric effect 空间效应Steric hindrance 位阻Atropismer 阻转异构体Puckered ring 折叠环Conformational inversion 构象反转Chair conformation 椅型构象Boat conformation 船型构象Twist conformation 扭型构象Skew boat conformation 扭船型构象Half-chair conformation 半椅型构象Pseudorotation 假旋转Envelope conformation 信封[型]构象Axial bond 直[立]键Equatorial bond 平[伏]键Cisoid conformation 顺向构象Transoid conformation 反向构象Retention of configuration 构型保持Regioselectivity 区域选择性Regiospecificity 区域专一性Stereocelectivity 立体选择性Stereospecificty 立体专一性Conformer 构象异构体Conformational effect 构象效应Cram’s rube 克拉姆规则Prelog’rule普雷洛格规则Stereochemical orientation 立体[化学]取向Conformational transmission 构象传递Homolog 同系物Ipso position 本位Ortho position 邻位Meta position 间位Para position 对位Amphi position 远位Peri position 近位Trigonal hybridization 三角杂化Molecular orbiral method 分子轨道法Valence bond method 价键法Delocalezed bond 离域键Cross conjugation 交叉共轭Vinylog 插烯物Mesomeric effect 中介效应Resonance 共振Resonance effect 共振效应Hyperconjugation 超共轭Isovalent hyperconjugation 等价超共轭No-bond resonance 无键共振Aromaticity 芳香性Aromatic sexter 芳香六隅Huckel’rule休克尔规则Paramagnetic ring current 顺磁环电流Diamagnetic ring cruuent 抗磁环电流Homoaromaticity 同芳香性Antiaromaticity 反芳香性Alternant hydrocarbon 交替烃Non-alternant hydrocarbon 非交替烷Pericyclic reaction 周环反应Electrocyclic rearrangement 电环[化]重排Conrotatory 顺旋Disroatatory 对旋Cycloaddition 环加成Symmetry forbidden-reaction 对称禁阻反应Synfacial reaction 同面反应Antarafacial reaction 异面反应Mobius system 默比乌斯体系Leois structure 路易斯结构Coordinate-covalent bond 配位共价键Banana bond 香蕉键Pauling electronegativity scale 鲍林电负性标度Polarizability 可极化性Inductive effect 诱导效应Field effect 场效应Electrical effect 电场效应tautomerism 互变异构Tautomerization 互变异构化Keto-enol tautomerism 酮-烯醇互变异构Phenol-keto tautomerism 酚-酮互变异构Imine-enamine atutomerism 亚胺-烯胺互变异构Ring-chain tautomerism 环-链互变异构Valence tautomerism 价互变异构Ambident 两可[的]Solvent effect 溶剂效应Acid-base catalyxed reaction 酸性溶剂Basic solvent 碱性溶剂Dielectric constant 介电常数Solvated electron 溶剂化电子Acid-base catalyzed reaction 酸碱催化反应Conjugate base 共轭酸Conjugate base 共轭碱Therm odynamic acidity 热力学酸度Kinetic acidity 动力学酸度Electron donof-acceptor complex,EDAcomplex 电子给[体]受体络合物Host 主体Guest 客体Primary isotope effect 一级同位素效应Secondary isotope effect 二级同位数效应Inverse isotope effect 逆同位素效应Kinetic control 动力学控制Thermodynamic control 热力学控制Substrate 底物Intermediate 中间体Reactive intermediate 活泼中间体Microscopic reversibility 微观可逆性Hammond postulate 哈蒙德假说Linear free energy 线性自由能Non-bonded interaction 非键相互作用Torsional effect 扭转效应Pitzer strain 皮策张力Restricted rotation 阻碍旋转Eclipsing effect 重叠效应Eclipsing strain 重叠张力Small-angle strain 小角张力Large angle strain 大角张力Transannular interaction 跨环相互作用Transannular strain 跨环张力I strain 内张力F strain 前张力B strain 后张力Anomeric effect 端基异构效应Walden inversion 瓦尔登反转Racemization 外消旋化Isoinversion 等反转Isoracemization 等消旋Homochiral 纯手性[的]Mechanism 机理Unimolecular nucleophilic 单分子亲核取代Bimolecular nucleophilic sub-stitution 双分子亲核取代Bimolecular nucleophilic substi-tution(with allylic rearrange-ment) 双分子亲核取代(含烯丙型重排)Internal nucleophilic substiru-tion 分子内亲核取代Aromatic nucleophilic substitu-tion 芳香亲核取代Unimolecular electrophilic sub-stitution 单分子亲电取代Bimolecular electrophilic substi-tution 双分子亲电取代Nucleophile-assisted unimolecu-lar electrophilic substitution 亲核体协助单分子亲电取代Unimolecular elimination 单分子消除Bimolecular elimination 双分子消除Unimolecular elimination through the conjugate base 单分子共轭碱消除Bimolecular elimination through the conjugate base 双分子共轭碱消除Bimolecular elimination with for-mation of a carbonyl group 双分子羰基形成消除Unimolecular acid-catalyzed acyl-oxygen cleavage 单分子酸催化酰氧断裂Bimolecular base-catalyzed acyl-oxygen cleavage 双分子碱催化酰氧断裂Unimolecular acid-catalyzed alkyl-oxygen cleavage 单分子酸催化烷氧断裂Bimllecular base-catalyzed al- kyl-oxygen cleavage 双分子碱催化烷氧断裂π-allyl complex mechanism π烯丙型络合机理Borderline mechanism 边理机理Homolysis 均裂Heterolysis 异裂Heterolytic michanism 异裂机理Counrer[gegen]ion 反荷离子Ion pair 离子对Carbocation 碳正离子Nonclassical carbocation 非经典碳正离子Carbanion 碳负离子Masked carbanion 掩蔽碳负离子Carbenoid 卡宾体Carbene 卡宾Nitrene 氮宾Carbine 碳炔Electrophilic addition 亲电加成Electrophile 亲电体Diaxial addition 双直键加成Markovnikov’s rube 马尔科夫尼科规则Anti-Markovnikov addition 反马氏加成Michael addition 迈克尔加成Substitution 取代Electrophilic substitution 亲电取代Addition-elimination mechanism 加成消除机理Electrophilic aromatic substitu-tion 亲电芳香取代Electron transfer 电子转移Electron-donating group 给电子基团Electron-Withdrawing group 吸电子基团Deactivating group 钝化基团Orinentation 取向Ortho-para directing group 邻对位定位基Meta directing group 间位定位基Ortho effect 邻位效应Partial rate factor 分速度系数Nucleophilic reaction 亲核反应Internal return 内返Nucleophilicity 亲核体Nucleophilicity 亲核性α-effect α-效应Backside attack 背面进攻Inversion 反转Umbrella effect 伞效应Push-pull effect 推拉效应Leaving group 离去基团Electrofuge 离电体Nucleofuge 离核体Phase-transfer catalysis 相转移催化Neighboring group participation 邻基基参与Neighboring proup assistance,anchimeric assistance 邻助作用Neighboring group effect 邻基效应Apofacial reaction 反面反应Briddgehead displacement 桥头取代Aryl action 芳正离子Benzyne 苯炔Zaitsev rule 札依采夫规则Anti-Zaitsev orientation 反札依采夫定向Hofmann’s反应Alkylation 烷基化C- alkylation C-烷基化O- alkylation O-烷基化N-alkylation N-烷基化Silylation 硅烷[基]化Exhaustive methylation 彻底甲基化Seco alkylation 断裂烷基化Demethylation 脱甲基化Ethylation 乙基化Arylation 芳基化Acylation 酰化Formylation 甲酰化Carbalkoxylation 烷氧羰基化Carboamidation 氨羰基化Carboxylation 羧基化Amination 氨基化Bisamination 双氨基化Cine substitution 移位取代Transamination 氨基交换Hydroxylation 羟基化acyloxyation 酰氧基化Decarboxylative nitration 脱羧卤化Allylic halogenation 烯丙型卤化Dehalogenation 脱卤Nitration 硝化Decarboxylative nitration 脱羧硝化Nitrosation 亚硝化Sulfonation 磺化Chlorosulfonation 氯磺酰化Desulfonation 脱磺酸基Sulfenylation 亚磺酰化Sulfonylation 磺酰化Chlorosulfenation 氯亚磺酰化Chlorocarbonylation 氯羰基化Diazotization 重氮化Diazo transfer 重氮基转移Coupling reaction 偶联反应Diazonium coupling 重氮偶联Cross-coupling reaction 交叉偶联反应1,4-addition 1,4-加成Conjugate addition 共轭加成Dimerization 二聚Trimefization 三聚Additive dimerization 加成二聚sulfurization 硫化Selenylation 硒化Hydroboration 硼氢化Oxyamination 羟氨基化Insertion 插入carbonylation 羧基化Hydroformylation 加氢甲酰基化Oxo process 羰基合成Decarbonylation 脱羰Hydrocarboxylation 氢羧基化Homologization 同系化Cyanoethylation 氰乙基化Decyanoethylation 脱氰乙基Ring clsure 环合Diene synthesis 双烯合成Dienophile 亲双烯体Endo addition 内型加成Exo addition 外型加成Diels-Alder reaction 第尔斯-尔德反应Retro Diels-Alder reaction 逆第尔斯-阿尔德反应Ene synthesis 单烯合成Anionic cycloaddition 负离子环加成Dipolar addition 偶极加成- elimination -消除- elimination -消除- elimination -消除-elimination -消除Dehydrohalogenation 脱卤化氢Deamination 脱氨基Pyrolytic elimination 热解消除Elimination-addition 消除-加成Decarboxylation 脱羧Decarboxamidation 脱酰胺Decyanation 脱氰基Alkylolysis,alkyl cleavage 烷基裂解Acylolysis,acyl cleavage 酰基裂解Flash pyrolysis 闪热裂Fragmentation 碎裂Chiletropic reaction 螯键反应Chelation 螯环化Esterification 酯化Transesterification 酯交换Saponification 皂化Alcoholysis 醇解Ethanolysis 乙醇解Cyanomethylation 氰甲基化Aminomethylation 氨甲基化Hydroxymethylation 羟甲基化Hydroxyalkylation 羟烷基化Cholromethylation 氯甲基化Transacetalation 缩醛交换Enolization 烯醇化Haloform reaction 卤仿反应Condensation 缩合Aldol condensation 羟醛缩合Cross aldol condensation 交叉羟醛缩合Retrograde aldol condensation 逆羟醛缩合Acyloin condensation 偶姻缩合Cyclization 环化Annulation,annelation 增环反应Spiroannulation 螺增环Autoxidation 自氧化Allylic hydroperoxylation 烯丙型氢过氧化Epoxidation 环氧化Oxonolysis 臭氧解Electrochemical oxidation 电化学氧化Oxidative decarboxylation 氧化脱羧Aromatization 芳构化Catalytic hydrogenation 催化氢化Heterogeneous hydrogenation 多相氢化Homogeneous hydrogenation 均相氢化Catalytic dehydrogenation 催化脱氢Transfer hydrogenation 转移氢化Hydrogenolysis 氢解Dissolving metal reduction 溶解金属还原Single electron transfer 单电子转移Bimolecular reduction 双分子还原Electrochemical reduction 电化学还原Reductive alkylation 还原烷基化Reductive acylation 还原酰化Reductive dimerization 还原二聚Deoxygenation 脱氧Desulfurization 脱硫Deselenization 脱硒Mitallation 金属化Lithiation 锂化Hydrometallation 氢金属化Mercuration 汞化Oxymercuration 羟汞化Aminomercuration 氨汞化Abstraction 夺取[反应]Internal abstraction 内夺取[反应] Rearrangement 重排Prototropic rearrangement 质了转移重排Double bond migration 双键移位Allylic migration 烯丙型重排Allylic migration 烯丙型迁移Ring contraction 环缩小[反应]Ring expansion,ring enlargement 扩环[反应] -ketol rearrangement -酮醇重排Pinacol rearrangement 频哪醇重排Retropinacol rearrangement 逆频哪醇重排Semipinacol rearrangement 半频哪醇重排Benzilic rearrangement 二苯乙醇酸重排Acyl rearrangement 酰基重排Migratory aptitude 迁移倾向Transannular insertion 跨环插入Transannular rearrangement 跨环重排Migration 迁移Prototropy 质子转移Cationotropic rearrangement 正离子转移重排Anionotropy 负离子转移Anionotropic rearrangement 负离子转移重排Sigmatropic rearrangement -迁移重排Homosigmatropic rearrangement 同迁移重排Electrophilic rearrangement 亲电重排Photosensitization 光敏化Forbidden transition 禁阻跃迁photooxidation 光氧化Photoisomerization 光异构化Photochemical rearrangement 光化学重排2.4 有机化合物类名Aliphatic compound 脂肪族化合物Hpdrocarbon 碳氢化合物Alkane 烷Wax 蜡Paraffin wax 石蜡Alkene 烯Alkyen 炔Acetylide 炔化物Active hydrogen compounds 活泼氢化合物Carbon acid 碳氢酸Super acid 超酸Diene 双烯Triene 三烯Allene 丙二烯Ccumulene 累积多烯Enyne 烯炔Diyne 二炔Alkyl halide 卤代烷Alcohol 醇Homoallylic alcohol 高烯丙醇Ether 醚Epoxide 环氧化物Cellosolve 溶纤剂Crown ether 冠醚Netro compound 硝基化合物Amine 胺Quaternaryammonium com-pound 季铵化合物Amine oxide 氧化胺Diazoalkane 重氮烷Mercaptan 硫醇Sulfonic acid 磺酸Sulfoxide 亚砜Sulfone 砜Aldehyde 醛Detone 酮Aldehyde hydrate 醛水合物Ketone hydrate 酮水合物Hemiacetal 半缩醛Acetal 缩醛Ketal 缩酮Dithiane 二噻烷Aminal 缩醛胺imine 亚胺Aldimine 醛亚胺Oxime 肟Aldimine 醛肟Oxime 亚硝基化合物aldoxime 硝酮Hydrazone 腙Azine 嗪Semicarbazone 缩氯基脲Cyanohydrin 羟腈Pinacol 频哪醇Enol 烯醇Enol ether 烯醇醚Enol ester 烯醇酯Enamine 烯胺Ynamine 炔胺Mannich base 曼尼希碱Carboxylic acid 羧酸Ester 酯orthoester 原酸酯Acyl halide 酰卤Acyl fluoride 酰氟Acyl chloride 酰氯Acyl rtomide 酰溴Acyl iodide 酰碘Carbobenzoxy chloride 苄氧甲酰氯Acyl tosylate 酰基对甲苯磺酸酐Ketene 乙烯酮Peracid 过酸Perester 过酸酯Acyl peroxide 酰基过氧化物Nitrile 腈Nitrile oxide 氧化腈Isonitrile 异腈Amide 酰胺Imide 二酰亚胺N-bromo compound N-溴化物Hydrazide 酰肼Acyl azide 酰叠氮Amidine 脒Keto ester 酮酸酯Acyl cyanide 酰腈Carbon suboxide 二氧化三碳Glycidic acid 环氧丙酸Carbammic acid 氨基甲酸Carbamate 氨基甲酸酯Urea 脲Cyanamide 氨腈Carbodiimide 碳二亚胺Allophanate 脲基甲酸酯Thioester 硫代酸酯Thiol acid 硫羰酸Lactone 内酯Lactol 内半缩醛Macrolide 大环内酯Amino acid 氨基酸Zwitterions 两性离子Inner salt 内盐Betaine 甜菜碱Lactam 内酰胺Hydantion 乙内酰脲Peptide 肽Glycol 二醇Aldol 羟醛Acyloin 偶姻Carbohydrate 碳水化合物Aldose 醛糖Ketose 酮糖Furanose 呋喃糖Pyranose 吡喃糖Glycoside 糖苷Glucoside 葡[萄]糖苷Aglycon 苷元Saccharide 糖类Oligosaccharide 寡糖Polysaccharide 多糖Alditol 糖醇Osazone 脎Alicyclic compound 脂环化合物Cycloalkene 环烷Spirane 环烯Cage compound 螺烷Propellane 笼型化合物Rotazane 螺桨烷Catenane 轮烷Rused ring 索烃。
- 1、下载文档前请自行甄别文档内容的完整性,平台不提供额外的编辑、内容补充、找答案等附加服务。
- 2、"仅部分预览"的文档,不可在线预览部分如存在完整性等问题,可反馈申请退款(可完整预览的文档不适用该条件!)。
- 3、如文档侵犯您的权益,请联系客服反馈,我们会尽快为您处理(人工客服工作时间:9:00-18:30)。
对称八溴代酞菁的合成及其特征K.R. Venugopala Reddy a,*, J. Keshavayya ba Department of Studies in Industrial Chemistry, Kuvempu University,Jnanasahyadri, Shankaraghatta - 577 451Shimoga District,Karnataka, Indiab Department of Studies in Chemistry, Kuvempu University,Jnanasahyadri, Shankaraghatta - 577 451Shimoga District,Karnataka, IndiaReceived 5 November 2001; received in revised form 14 December 2001;accepted 18 January 2002* Corresponding author. Tel.: +91-08282-56225; fax: +91-08282-37255.E-mail address: universitysancharnet.in (K.R. Venugopala Reddy).摘要现在已经提出一条既方便又简单合成对称1,3,8,10,15,17,22,24-溴代金属—Cu,Co,Ni,Zn酞菁颜料的路线。
金属酞菁是由相应的八氨基取代酞菁合成的。
合成的化合物经过元素分析、电子光谱、红外光谱、磁性测试、粉末X射线衍射实验和热重研究来评估其热稳性、结晶度、结构完整性和纯度。
经过讨论和分析发现取代基对于电子光谱的影响及轨道对磁矩的贡献远远超过了电子场强的影响。
关键词:酞菁八取代合成热度电子的颜料和染料1.引言酞菁在近几年引起广泛的关注不仅是因为酞菁的结构同一些能够维持生命的重要分子如叶绿素、血红素相似,而且它具有显著的上色功能。
这些颜料是被稳定在一些通过在酞菁环外围引入一些合适的取代基的多晶转变体上。
卤素取代的金属酞菁颜料在商业上用于颜料及滤色片[1]等的制造。
由于酞菁普遍具有共轭芳香基团的发色基,所以在蓝绿色上有着非常高的吸收系数。
总之,所有的酞菁都有四苯并四氮杂卟啉结构作为发色基。
由于金属酞菁的着色能力和其华丽的色彩使其在颜料及染料[2]制造业中占有重要的地位。
他们对光、热、酸及碱有着显著地稳定性。
以上一系列化合物不溶于水和大部分有机溶剂,并被广泛用于制造印刷用油墨、颜料、涂料和塑料。
酞菁被用来做彩色打印机和激光打印机[3]的碳粉。
酞菁颜料也被用作液晶显示器的滤光镜。
一篇文献研究表明合成氯代十六烷、溴代十六烷、碘代十六烷和混合卤代金属酞菁的方法和那些颜料主要用于商业目的[4]。
大多数的合成流程都是有文献专利的因为不能通用。
关于对称四取代2,9,16,23和1,8,15,22,-溴代金属酞菁的合成和结构的研究报道是可用的[5,6]。
但是,关于对称八取代1,3,8,10,15,17,22,24-溴代金属酞菁的合成和结构的研究报道是没有可通用的。
基于以上事实,我们开始从事对标题化合物的合成及结构的研究。
在过去的一些文献中我们讨论了关于对称取代1,3,8,10,15,17,22,24-八溴代金属酞菁的合成和结构的研究。
文中所提到的工艺经过了适当的改良并应用到合成标题化合物中[5]。
1,3,8,10,15,17,22,24位置上的酞菁的主要部分的π电子体系的溴代取代效应和随之引起的电子光谱、热性能、红外光谱和结晶度的数据都在讨论中。
2.实验在其他地方报道过制备1,3,8,10,15,17,22,24-八氨基取代酞菁的工艺流程[7]。
氨基化合物通过改良后的桑德麦尔反应转化为相应的溴代衍生物[8]。
合成1,3,8,10,15,17,22,24-八溴代酞菁的路线如图一所示。
所有化学试剂皆为分析纯。
2.1 1,3,8,10,15,17,22,24-八溴代酞菁铜(Ⅱ)颜料的制备1,3,8,10,15,17,22,24-八氨基酞菁铜(Ⅱ)(5g)与HCl中偶合得到酞菁铜的重氮盐CuPON2+Cl-。
并将混合物保存在碎冰中使温度在5℃以下。
将五水硫酸铜(19.6g)和二水溴化钠(11.3g)溶解于50ml的水中,将亚硫酸氢钠(5.12g 溶于30ml水中)缓慢加入上述溶液中并不断搅拌,直至室温。
用少量亚硫酸洗涤三次所得沉淀物。
将溴化亚铜溶解于20ml48%的HBr中。
再将1,3,8,10,15,17,22,24-八溴代酞菁铜(Ⅱ)的冷藏溶液缓慢倒入溴化钠中,并搅拌。
由于副产物重氮盐和溴化亚铜的分离,混合物开始变浑浊(CuPON2+Cl_,CuBr)。
加成物要不断振荡直至室温。
然后水浴加热到60℃使其完成重盐的裂解并且一直加热到没有氮气生成。
倾析得到澄清溶液,并用水冲洗残留物。
将绿色产物离心分离并分别用1M NaOH和1MHCl洗涤四次,最后用蒸馏水清洗。
固体1,3,8,10,15,17,22,24-八溴代酞菁铜(Ⅱ)在50℃下干燥一1h。
颜料粉末是通过酸附着过程得到的,即一份粉末溶于6-10份的浓硫酸中。
将混合物静止1-2h,然后放进冰块中并搅拌彻底。
得到颜料并用热水冲洗。
最后重复用纯酒精洗涤并放在真空干燥器中干燥2天[9]。
这种方法是用于Cu(Ⅱ),Co(Ⅱ)和Ni(Ⅱ),但是Zn(Ⅱ)要经历金属移除反应。
Ni(Ⅱ)、Co(Ⅱ)和Zn(Ⅱ)的八溴代酞菁衍生物是分别通过各自的金属氨基酞菁衍生物用上述方法制备的。
在印度昌迪加尔的旁遮普大学的精细仪器中心对C、H、N做了元素分析。
对磁性的研究是在室温下通过Gouy磁天平法即包括NP-53型电磁体和半微量天平进行研究的。
Pacal 常数是用来计算抗磁修正的。
Hg [Co (SCN)4] 化合物作为岛津紫外可见分光光度计的校准物[10],UV-160A使用来研究电子吸收光谱。
红外光谱是通过Nicolet MX-FT红外分光仪测量的。
PW1710X-ray 衍射仪是用来研究化合物衍射部分的。
光谱是在40Kv,20mA,7mm宽的孔道且图表速度为10mm/min进行记录的。
TGA/SDTA热分析仪是用来研究热分解的。
3.结果与讨论由于金属原子半径非常接近于1.35A所以形成的金属酞菁化合物具有热稳定性和抗酸腐蚀性。
八溴代酞菁Cu(Ⅱ)、Zn(Ⅱ)和Co(Ⅱ)衍生物制备完毕,且都为深绿色,但是只有Zn带有些许棕色。
它们浓硫酸中为澄清溶液也完全溶于DMSO、DMF和吡啶中,但是不溶于水及大部分有机溶液如乙醇、乙醚、四氯化碳和苯中。
对C、H、N及金属的分析结果如表一所示。
4.红外光谱红外光谱红外数据记录总结在表2中,光谱为图2。
所有化合物在3394-3410cm-1上有宽峰吸收,即显示出酞菁高分子中的氮氢键[11]。
在1533-1613cm-1处有尖锐的峰行,即表示出有碳碳双键存在。
在1341-1352cm-1处有吸收峰是因为碳氮键的存在。
在752-757cm-1处有吸收峰是因为碳溴键的伸缩频率。
剩下的吸收峰是由于酞菁骨架振动引起的[12]。
5.磁性磁性和磁矩都是在化合物为固态情况下测量的,数据显示CuPOBr和CoPOBr为顺磁性的,ZnPOBr为逆磁性的。
CuPOBr和CoPOBr的磁矩比未成对电子的要高。
高出来的大概是由于简并轨道的贡献。
这种轨道作用会随电子磁场的增强而增加。
只有在高电子磁场下化合物的磁矩与自旋对应。
CuPOBr和CoPOBr在u eff上的较高的数据可能是因为在π电子云下分子间的磁性各向异性[12.13]。
人们可能注意从Xm和u eff的变化趋势注意到CoPOBr中相邻金属原子的自旋偶合和在CuPOBr的酞菁分子中氮原子的超交换[13.14]。
由于酞菁环上[15.16]的金属轨道和π轨道的相互作用,在高电子场中发现了预期中的CuPOBr和CoPOBr的数值比未取代的酞菁要高。
但是得到的数据并不与原来的未取代的金属酞菁相符合[17]。
这个可能是占主导地位的阻碍叠加超过预期中的功能团的霍尔迁移。
6.电子光谱溴代酞菁是在18M的硫酸中进行电子光谱测量,且浓度围为5.1-5.2×10-4M。
数据记录总结在表2中。
所有化合物的峰值都在611-647nm的围,此围为酞菁特征峰值。
在224-231nm 上的波峰显示有碳键。
所有的化合物在471-482nm上的波峰为a2u-e g的贡献。
7.X射线衍射分析Cu(Ⅱ)、Co(Ⅱ)、Ni(Ⅱ)、Zn(Ⅱ)的酞菁粉末衍射是在2θ,6-70°下显示出与弱结晶性相符的显著的特点。
人们能在图3明显的观察到在未取代的金属酞菁的峰值面积会扩大。
这显示出标题化合物比未取代的金属酞菁的结晶度要小。
这可能是因为体积较为庞大的溴原子在堆积金属酞菁的衍生物中占了主导地位。
粉末X射线衍射结果只能定性的解释结晶度。
溴取代的效果可能在第一个d数据中显示出来。
8.热重分析在静态下的对溴代金属酞菁的热重分析显示出这些化合物是一步降解。
CuPOBr、CoPOBr、NiPOBr、ZnPOBr在220-440℃下重量减少,即分子外围的溴基团损失。
最主要的重量损失是在440-650℃下发生的分子降解。
而最后未被分解的产物为金属氧化物。
热稳性顺序为ZnPOBr >CoPOBr >CuPOBr >NiPOBr。
可能的碎片模式总结在表3中。
9.结论对溴代金属酞菁磁性的研究显示CuPOBr、CoPOBr是顺磁性的而NiPOBr、ZnPOBr是逆磁性的。
磁矩由于轨道贡献会比未取代的高,在电子场中的磁矩变化是由于分子间的磁性影响。
对于热重的研究显现出热稳性的顺序为:ZnPOBr >CoPOBr >CuPOBr >NiPOBr。
致感化工中心所提供的实验仪器。
感印度UGC大学所授予的国际药学联合会研究会会员称号。
References[1] Moser FH, Thomas AL. Phthalocyanine compounds.New York: Reinhold, 1963.[2] Jagadeesh MB. Studies on phthalocyanine complexs. PhD thesis, Mysore University, Mysore, India 1993.[3] Gregory P. Industrial applications of phthalocyanines. JPorphyrines and Phthalocyanines 2000;4:432–7.[4] Leznoff CC, Lever ABP. Phthalocyanines properties and applications, vol. 1. New York: VCH Publishers Inc, 1989.[5] Somashekarappa MP. Studies on phthalocyanine complexes.PhD thesis, Kuvempu University, Shankaraghatta,India, 2000.[6] Keshavayya J. Studies on metal phthalocyanine complexes.PhD thesis, Mysore University, Mysore.[7] Keshavayya J, Venugopala Reddy KR. Synthesis, magnetic,spectral and thermal studies on metal (II)-1,3,8,10,15,17,22,24-octaaminophthalocyanine. Manuscript communicated.[8] Vogel’s textbook of practical organic chemistry. Vth : Longman Group UK Ltd, 1989.[9] Lemi T, Levent O. The synthesis of some phthalocyanine intermediates and pigments. Dyes and Pigments 1990;13:81–92.[10] Selwood P. Magnetochemistry. New York: Interscience,1956.[11] Somashekarappa MP, Keshavayya J. Synthesis, magnetic,spectral and thermalstudies on metal (II) 1,8,15,22-tetranitrophthalocyanines.Synth React Inorganic Met -Org Chem 1999;29(5):767–83.[12] Somashekarappa MP, Keshavayya J. Synthesis and structural studies of metal (II) 1,8,15,22-phthalocyanine tetraamines.J Saudi Chem Soc 1999;3(2):113–9.[13] Achar BN, Bhandari JM. Magnetis properties and ESR studies on copper phthalocyanines. Synth React Inorg Met—Org Chem 1993;23(1):133–48.[14] Kettle SFA. Physical inorganic chemistry–coordination chemistry approach spektrum: 1996: p. 206–8.[15] Harrison SE, Assour JM. Relationship of electron spin resonance and semi conduction phthalocyanines. J Chem Phy 1964;40(2):365–70.[16] Heilmeier GH, Harrison SE. Charge transport in copper phthalocyanines. Physical Review 1963;132(5):2010–6.[17] Gouterman, M. The phorphyrins, vol. III. Academic Press Inc., 1978.。