Electrochemistry of conductive polymers XXXV
导电高分子材料
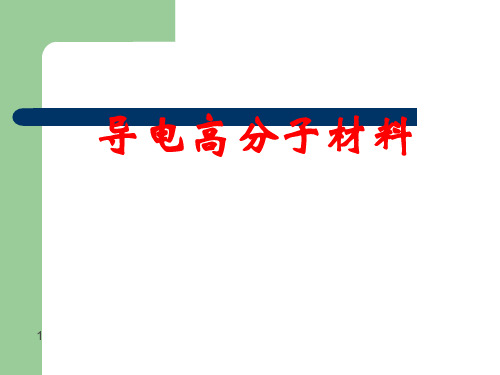
电子导电聚合物的性质与应用
其他性质与应用:
① 化学催化性质:p型掺杂、n型掺杂的电子导电 高分子材料具有氧化还原催化功能 应用:分析化学、催化和化学敏感器的制作 ② 开关性能:掺杂电子导电高分子材料的电导率 可通过电极控制 应用:有机分子开关器件 还可在超级电容器和腐蚀防护方面应用
13
场作用下发生可逆改变,即当施加电场时材料的 光吸收波长发生变化;去掉电场时又能够完全恢 复的性质。在外观性能上则表现为颜色的变化。 应用:制备无视角限制的显示器件
11
电子导电聚合物的性质与应用
电致发光性能及其应用:
电致发光:也称为电致荧光现象,指材料在电场作 用下可以发出可见光的性质。在电场作用下注入的 电子和空穴在电致发光材料内部复合成高能态的激 子,而处在高能态的激子回到低能态时又能够将获 得的能量以光能形式发出。 应用:多层电致发光器件
电化学聚合法:采用电极电位作为聚合反应的引发 和反应驱动力,在电极表面进行聚合反应并直接生 成导电聚合物膜。 反应完成后,生成的导电聚合物膜已经被反应时采 用的电极电位所氧化,即同时完成了掺杂过程;这 里所指的掺杂过程只是使导电聚合物的荷电情况发 生了变化,改变了分子轨道的占有情况,而并没有 加入第二种物质。
9
电子导电聚合物的性质与应用
电子导电聚合物的性能与应用
导电能力
缺点
非掺杂 半导体 掺杂后 可超过碳黑
电导率低 化学稳定性差
其次,导电聚合物溶解性差,有一定的加工难度
应用:抗静电材料、屏蔽材料、二次电池电极 材 料 材料、微波材料等
10
电子导电聚合物的性质与应用
电致变色性能及其应用 电致变色现象:材料的光吸收特性在施加的电
5
电子导电聚合物的性质
导电高分子
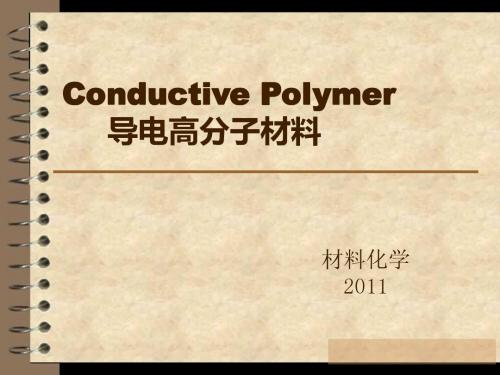
子在整个分子骨架内运动。离域π键的形成,增大了π
电子活动范围,使体 系能级降低、能级间 隔变小,增加物质的 导电性能。
导电高分子材料的导电机理
导电高分子材料的共同特征-交替的单键、双键共轭结构
聚乙炔由长链的碳分子以sp2键链接而成,每一个
碳原子有一个价电子未配对,且在垂直于sp2面上形成
未配对键。其电子云互相接触,会使得未配对电子很 容易沿着长链移动,实现导电能力。
导电高分子材料的掺杂途径
氧化掺杂 (p-doping): [CH]n + 3x/2 I2 ——> [CH]nx+ + x I3还原掺杂 (n-doping): [CH]n + x Na ——> [CH]nx- + x Na+
添补后的聚合物形成盐类,产生电流的原因并不是 碘离子或钠离子而是共轭双键上的电子移动。
2004,13英寸
导 电 高 分 子 应 用
半导体特性的应用-太阳能电池
电高分子可制成太阳电池,结 构与发光二极管相近,但机制却相
反,它是将光能转换成电能。优势
在于廉价的制备成本,简单的制备 工艺,具有塑料的拉伸性、弹性和
柔韧性。
导 电 高 分 子 应 用
导体特性的应用-防静电、电磁屏蔽、防腐蚀
聚乙炔的掺杂反应
1975年,G. MacDiarmid 、 J.Heeger与
H.Shirakawa合作进行研究,他们发现当聚乙炔曝露于 碘蒸气中进行掺杂氧化反应(doping)后,其电导率令人 吃惊地达到3000S/m。
导电高分子材料的导电机理 有机化合物中的σ 键和π键
在有机共轭分子中,σ键是定域键,构成分子骨架; 而垂直于分子平面的p轨道组合成离域π键,所有π电
Synthesis and Properties of Conductive Polymers

Synthesis and Properties of ConductivePolymers合成和性质的导电聚合物导电聚合物是一种新型的材料,在电子器件和能源存储装置中担任着重要的角色。
这些聚合物是和传统聚合物有很大的区别,他们具有优异的导电性能和机械性能。
导电聚合物的发现历程导电聚合物的发现可以追溯到上个世纪六十年代初期,那个时候科学家意识到聚拢物中的共轭结构能够提高聚合物的电导率。
可是,由于当时合成方法和控制技术上面的限制,导电聚合物没有被广泛研究和应用。
直到1980年代,人们才开始广泛的研究导电聚合物,这些研究主要集中在聚噻吩和聚苯胺材料上。
在过去的三十年间,研究人员们指导了大量的时间和精力,进一步提高了导电聚合物的性能和稳定性。
导电聚合物的分类目前,导电聚合物可以分成五类。
第一类是聚噻吩材料,这些聚合物能够形成常见的针状或纤维状结构,具有高导电性能和相对较好的稳定性。
第二类是聚苯胺材料,相比于聚噻吩材料,聚苯胺材料的分子结构更为复杂,能够形成不同的电导态。
第三类是聚氨酯材料,这种材料的导电性能并不强,但是由于它们的光学性能和机械稳定性,成为了一些特殊应用的理想选择。
第四类是聚茜素材料,这些材料能够形成大分子链状结构,密度较高,导电性能也好。
第五类是聚吲哚材料,这些材料相较其他的导电聚合物,具有宽带隙,因而表现出独特的光电性能。
导电聚合物的合成方法导电聚合物的合成方法包括化学氧化法、电化学合成法、固相合成法等几种。
化学氧化法是最为传统的一种合成方法,主要通过化学氧化剂的加入,使得单体发生氧化反应,完成实验。
电化学合成法则是利用电流作为促进聚合物反应的驱动力,通过在合适的电极上进行反应,来实现导电聚合物的合成。
固相合成法则是通过聚合物的反应活性官能团在固体表面的缓慢和稳定折叠以实现聚合物的合成。
导电聚合物的性质导电聚合物的性质决定了它们在电子器件和能源存储装置中的应用前景。
其中最重要的两个性能就是导电性以及光电性能。
电子材料作业 导电聚吡咯的相关研究
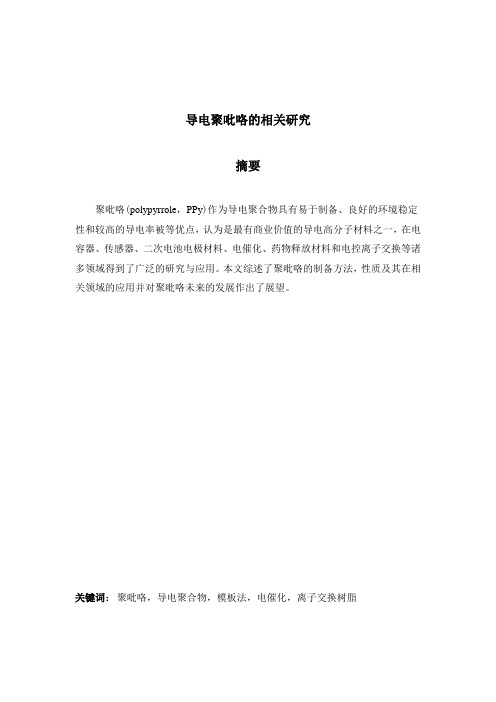
导电聚吡咯的相关研究摘要聚吡咯(polypyrrole,PPy)作为导电聚合物具有易于制备、良好的环境稳定性和较高的导电率被等优点,认为是最有商业价值的导电高分子材料之一,在电容器、传感器、二次电池电极材料、电催化、药物释放材料和电控离子交换等诸多领域得到了广泛的研究与应用。
本文综述了聚吡咯的制备方法,性质及其在相关领域的应用并对聚吡咯未来的发展作出了展望。
关键词:聚吡咯,导电聚合物,模板法,电催化,离子交换树脂THE RELATED RESEARCH OF CONDUCTIVE POLYPYRROLEAbstractAlypyrrole are considered one of the most commercially valuable cons a c -onductive polymer,polypyrrole are readily prepared, having a good environment al stability and high electrical conductivity, etc.. Therefore,poductive polymer m -aterials.Polypyrrole materials have been widely studied and applied in electroca -talysis,sensors,secondary battery electrode materials,drug delivery materials and electronic control ion exchange etc..This article overviewed the Preparation me -thod.Electrochemical properties and related areas of application werecontained,a -nd future development of polypyrrole are pointed out.Key words:polypyrrole;conducting polymer; doping; ion exchange;electrocatal -ysis.目录一、前言 (4)二、聚吡咯的结构及性质 (4)2.1 导电性 (5)2.2 氧化还原特性 (5)2.3 稳定性 (5)三、聚吡咯的制备 (6)3.1 电化学合成法 (6)3.2 化学氧化法 (6)3.3 模板法 (7)3.3.1 硬模板法 (7)3.3.2 软模板法 (7)3.3.3 无模板法 (8)四、聚吡咯的应用 (9)4.1 聚吡咯在电容器方面的应用 (9)4.2 聚吡咯光电性能方面的应用 (9)4.3 聚吡咯在传感器方面的应用 (9)4.4二次电池的电极材料 (10)4.5 电催化 (10)4.6 离子交换树脂 (11)4.7 电控药物释放 (11)四、结束语 (11)参考文献 (12)导电聚吡咯的相关研究一、前言在过去很长一段时间里,有机高分子一直被视为结构绝缘材料。
Electronic conductivity of conducting polymers

Electronic conductivity of conductingpolymers导言聚合物材料是一类具有良好可塑性、可连续加工性和广泛应用前景的材料。
其中,导电性高的导电聚合物成为现代电子学领域的重要材料之一。
导电聚合物的导电性能决定了其在电子学设备、传感器和能源存储等领域的应用效果。
近年来,科学家们对导电聚合物的研究愈发深入,其中最为重要的一项研究内容就是导电聚合物的电子传输机制和电导率规律。
本文将从电子传输机制、电导率规律和导电聚合物的应用三个方面,深入探究导电聚合物的电子导电特性。
电子传输机制聚合物材料是一种高度结晶形态的有机材料,其分子结构中常含有许多共轭体系。
由于共轭体系的存在,聚合物分子内部会出现大量的π-π堆积现象,从而形成一些能隙特征较弱的价带和导带。
电子在导电聚合物的导电过程中,就是通过这些能隙传输到导带实现电导的。
与金属、半导体不同,导电聚合物具有非常复杂的电子能级结构,因此其电子传输机制也有着一定的特殊之处。
常见的、被广泛研究的导电聚合物材料包括了聚苯乙烯、聚噻吩、聚丙烯腈等。
在这些材料中,大部分电子传输都是通过共轭体系实现的。
然而由于共轭体系对于电子的传输存在着严格的限制,因此电子传输通常还是受到晶体结构、材料形貌和材料制备工艺等因素的影响。
电导率规律导电聚合物的电导率通常是其使用效能的一个关键指标。
在导电聚合物中,电子传输受限于材料内大量的π-π堆积现象和电阻性附加物的存在,因此其导电行为的规律性相对复杂。
一般来说,导电聚合物的电导率与以下因素密切相关:1. 聚合物的共轭度:共轭单元越多,材料的导电性越高。
在大部分情况下,共轭越强,封口内部的电子结构就越稳定,导电性就越高。
因此,聚噻吩和聚苯乙烯等极端共轭聚合物,其导电性都相对较好。
2. 材料的晶体结构:导电聚合物的晶体结构对于电子传输有着非常重要的影响。
由于共轭体系对于电子能级的限制,因此导电性与材料的晶体结构紧密相关。
导电聚合物的研究_3

导电聚合物的研究论文导读:导电聚合物大多都有一个较长的π共轭主链,因此又称为共轭聚合物。
聚吡咯具有质量轻,电导率高,易于制备与掺杂,空气稳定性好,合成方便,电化学可逆性强等优点,但价格及工艺流程比较昂贵,因此还没有大规模推广。
聚苯胺以其良好的热稳定性,化学稳定性,电化学可逆性,优良的电磁微波吸收性能,潜在的溶液和熔融加工性能,原料易得,合成方法简便,还有独特的掺杂现象等特性,成为现在研究进展最快的导电高分子材料之一;聚噻吩作为高分子材料的一种,具有极其小的尺寸、丰富潜在的功能,导电能力,可以在酸性体系中聚合,生成粉末状不溶物或者液态的聚合物但是由于导电高分子聚吡咯聚噻吩聚苯胺链的强刚性和链间的强的相互作用使得它们的溶解性极差,相应的可加工性也差,限制了它们在技术上的广泛应用。
聚苯胺可看作是苯二胺与醌二亚胺的共聚物,y值用于表征聚苯胺的氧化还原程度,(1-y)值代表了聚苯胺的氧化状态。
关键词:导电聚合物,聚噻吩,聚苯胺,聚吡咯,对比1、引言1977白川英树等人发现了碘掺杂的聚乙炔具有很高的导电性,比一般的有机高分子材料高约13个数量级。
这一惊人发现,彻底改变了人们以往的观念-—有机高分子是绝缘体。
导电聚合物大多都有一个较长的π共轭主链,因此又称为共轭聚合物。
论文大全。
共轭分子中,σ键是定域键,构成分子骨架;而垂直于分子平面的p轨道组合成离域π键,所有π电子在整个分子骨架内运动。
离域π键的形成,增大了π电子活动范围,使体系能级降低、能级间隔变小,增加物质的导电性能。
交替的单键、双键共轭结构是导电高分子材料的共同特征,若进行掺杂可使其电导率增加若干数量级,接近于金属电导率,这为导电高分子进入市场提供了强劲的力量。
2.三种导电高分子的对比本文导电高分子材料研究主要是聚噻吩,聚苯胺,聚吡咯这三种聚合物,其中只有聚苯胺初步形成了工业化规模,由此可见他们之间存在一定程度的差异,接下来将从以下四个方面对三种物质的性质进行对比:2.1优缺点比较:聚吡咯具有质量轻,电导率高,易于制备与掺杂,空气稳定性好,合成方便,电化学可逆性强等优点,但价格及工艺流程比较昂贵,因此还没有大规模推广;聚苯胺以其良好的热稳定性,化学稳定性,电化学可逆性,优良的电磁微波吸收性能,潜在的溶液和熔融加工性能,原料易得,合成方法简便,还有独特的掺杂现象等特性,成为现在研究进展最快的导电高分子材料之一;聚噻吩作为高分子材料的一种,具有极其小的尺寸、丰富潜在的功能, 导电能力,可以在酸性体系中聚合,生成粉末状不溶物或者液态的聚合物但是由于导电高分子聚吡咯聚噻吩聚苯胺链的强刚性和链间的强的相互作用使得它们的溶解性极差,相应的可加工性也差,限制了它们在技术上的广泛应用。
离子液体应用于电合成导电聚合物

2. 2 Polyaniline and its derivatives 2. 3 Polythiophene and its derivatives 2. 4 Poly(3 ,42ethylenedioxythiophene) and its deriva2
tives 2. 5 Poly( p2phenylene)
离子液体具有一系列突出优点 : (1) 几乎无蒸 气压 ,在使用 、贮藏中不会蒸发散失 ,可以循环使用 而不污染环境 ; (2) 很宽的液态温度范围 ,甚至超过 300 ℃,相比之下水只有 100 ℃; (3) 较宽的电化学窗 口 ,达 3 —5V ; (4) 导电性 、导热性和热力学稳定性 好 ; (5) 无可燃性 、无着火点 ; (6) 可以通过对阴阳离 子的设计来调节其对无机物 、水 、有机物及聚合物的 溶解性 。正是基于这些独特的性质 ,离子液体的研 究在世界范围内迅速开展 。
1914 年 ,Walden[2] 报道了第一个离子液体 ——— 硝酸乙基铵 [ EtNH3 ] [ NO3 ] 。1951 年 , Hurley 等[3] 合 成了乙基吡啶溴化物2三氯化铝 [ EPyBr ]2[ AlCl3 ] ,标 志着 AlCl3 型离子液体诞生 。1992 年 ,Wilkes 等[4] 合 成了 12乙基232甲基咪唑四氟硼酸盐 [ EMIm ] [ BF4 ] , 这种非 AlCl3 型离子液体熔点低 、抗水解 、稳定性 强 ,推动了离子液体研究的迅速发展 。近年来 ,离子 液体已被成功引入到清洁能源 、生命科学 、功能材料 制备 、催化 、电化学等领域 ,成为自然科学和应用研 究领域中的热点 ,国际国内也出现了不少介绍离子 液体及其应用的综述性文章[5 —20] 。
media 6 Conclusion
导电聚合物电化学.
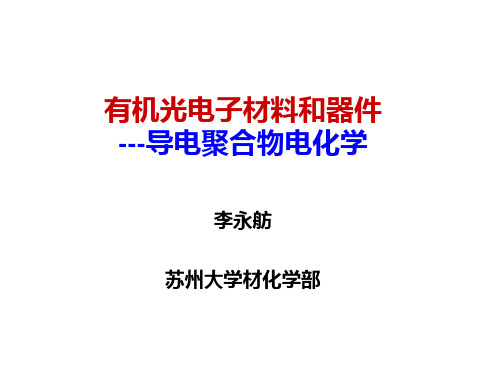
聚合反应间歇进行
导电聚吡咯的电化学制备
表 1. 支持电解质阴离子对制备的聚吡咯膜的结构和性能的影响 阴离子 BF4PF6AsF6ClO4HSO4CF3SO3CH3C6H4SO3(TsO-) CF3COO0.25 1.45 12 掺杂度 0.25-0.32 0.25-0.32 0.25-0.32 0.30 0.30 0.31 0.32 密度(g/cm3) 1.48 1.48 1.48 1.51 1.58 1.48 1.37 电导率(S/cm) 30-100 30-100 30-100 60-200 0.3 0.3-1 20-100
43.8 11.9 1.6 5.7 2.3
0.5
54.2
1.0 73.8 17.7 2.6 9.1
2.0 56.9 18.0 4.4 12.3
3.0 17.1 5.5
4.0 14.3 2.2 14.3
Y.F. Li, J. Yang, J. Appl. Polym. Sci., 65(1997) 2739.
* 在含 1%水的乙腈电解液中电化学聚合.
水溶液中支持电解质阴离子对制备的聚吡咯膜电导和密度的影响
聚吡咯膜的性质 电导率 / Scm-1 20~30 - 12 12 60~200 60~200 4~30 10 真实密度/ gcm-3 1.575 1.558 1.540 1.549 1.368 1.364 1.516 1.532 表观密度/gcm-3 0.52 0.36 0.38 0.46 1.24 1.25 1.25 1.26
[Y.F. Li, G.F. He, Synth. Met., 94(1998) 127]
小结
吡咯电化学聚合条件对制备的导电聚吡咯性质的影响
- 1、下载文档前请自行甄别文档内容的完整性,平台不提供额外的编辑、内容补充、找答案等附加服务。
- 2、"仅部分预览"的文档,不可在线预览部分如存在完整性等问题,可反馈申请退款(可完整预览的文档不适用该条件!)。
- 3、如文档侵犯您的权益,请联系客服反馈,我们会尽快为您处理(人工客服工作时间:9:00-18:30)。
Electrochimica Acta50(2005)3085–3092Electrochemistry of conductive polymers XXXV:Electrical and morphological characteristics of polypyrrolefilms prepared in aqueous media studied by current sensing atomic force microscopyDong-Hun Han,Hyo Joong Lee,Su-Moon Park∗Department of Chemistry and Center for Integrated Molecular Systems,Pohang University of Scienceand Technology,Pohang,Gyeongbuk790-784,KoreaReceived24August2004;received in revised form19October2004;accepted22October2004Available online11April2005AbstractElectrical and morphological characteristics of polypyrrole(PPy)films electro-deposited in four different aqueous electrolyte solutions including p-toluenesulfonate,dodecylsulfate,poly(styrenesulfonate),and perchlorate have been investigated using current-sensing atomic force microscopy.Experimental parameters including the electrolyte,applied current density,and deposition time were shown to affect the morphologies and current images of PPyfilms thus prepared.The PPyfilms were galvanostatically prepared under the identical conditions except for different electrolytes;the globular-shaped topographic structures with the unusually large sizes were obtained in dodecylsulfate. The p-toluenesulfonate doped PPyfilm showed the highest average conductivity whereas the perchlorate doped one showed the lowest of all thefilms prepared.©2005Elsevier Ltd.All rights reserved.Keywords:Polypyrrole;Current sensing AFM;Electrolyte effects;Conductivity1.IntroductionSince polyacetylene was shown to have high electrical conductivities when properly doped[1],-conjugated poly-mers have been studied extensively from both fundamental and practical points of view because these classes of poly-mers showed possibilities of a wide range of applications [2].Recently,they are regaining a great deal of attention owing to growing interests in“organic electronics”includ-ingfield effect transistors[3],light-emitting diodes[4],solar cells[5],electrochromic devices[6],electronic circuits[7], sensors[8],and other devices[9]because of their favorable processibility,reasonable stability,low cost,and possibilities of tailoring the structures on the molecular scale.Most of the-conjugated(conducting)polymers are straightforwardly prepared by chemical and/or electrochem-∗Corresponding author.Tel.:+82542792102;fax:+82542793399.E-mail address:smpark@(S.-M.Park).ical methods and their electronic properties can be reversibly changed between insulating and conducting states by chemi-cal and/or electrochemical doping reactions[2].PPy is one of the most studied conducting polymers because of its rather straightforward preparation methods and reasonable stabil-ities in air and aqueous media.However,it is well known from a number of experimental studies that various param-eters such as the solvent,electrolyte,electrode,deposition time,temperature,current density,and applied voltage all af-fect both its structures and electroactivities[2].Thus far,the conductivity data obtained from macroscopic experiments along with spectroscopic data have been used to describe its various electrical states depending on its preparation meth-ods and doping levels[2].Thus,primarily the bulk electrical properties were measured and averaged out over many inho-mogeneous regions.However,in order to ultimately control the electrical properties of conducting polymerfilms and to use those in organic electronics on nanometer scales,it is necessary to make the electrical measurements on nanome-0013-4686/$–see front matter©2005Elsevier Ltd.All rights reserved. doi:10.1016/j.electacta.2004.10.0853086 D.-H.Han et al./Electrochimica Acta50(2005)3085–3092ter scales.Also,as the dimension of devices becomes smaller, the change in electrical properties and its measurements on nanometer scales would be increasingly important.Atomic force microscopy(AFM)is a widely used sur-face characterization tool with nanometer scale resolution, some modifications of which can give a variety of otherwise impossible valuable information[10].The current-sensing AFM(CS-AFM)with its conducting tip allows not only the topographical and current images to be obtained simultane-ously but also current–voltage traces to be recorded on se-lected spots of the image,where one wants to study elec-trical characteristics on a nanometer scale[11].This tech-nique has been applied to various nanostructures such as single molecules[12],biomolecules[13],carbon nanotubes [14],self-assembled monolayers[15],quantum dots[16],and other nanostructures[17]because it allows easy and repro-ducible contacts to be made with various substances without having to use the difficult lithography processes.Thus,the CS-AFM has a number of advantages in studies of electri-cal properties of conducting polymerfilms.Recently,we and other investigators applied this technique successfully to the studies of conducting polymerfilms of various doping lev-els,proving its usefulness in the study of doping distributions by obtaining two-dimensional current images and nanoscale electrical properties by measuring the current–voltage char-acteristics[18,19].In the present study,we demonstrate that the electrical properties of PPyfilms are visualized by obtaining the two-dimensional current images under various preparation con-ditions and the two-dimensional current images offer useful data in following and understanding the changes of electri-cal properties of conducting polymerfilms depending on the preparation conditions and doping levels.2.Experimentalp-Toluenesulfonic acid(p-TS:Aldrich,98.5%),sodium dodecylsulfate(SDS:Fluka,99%),poly(styrene sulfonic acid)(PSS:Alfar Aesar,M w:70,000),and lithium perchlo-rate(LiClO4:Aldrich,99.99%)were used as received.Pyr-role(Aldrich,98%)was used after distillation over zinc powder and stored in the dark under the nitrogen atmo-sphere.PPyfilms were electrochemically prepared on gold-on-silicon electrodes(with chromium adhesive layers,LGA Films),after the solutions were de-aerated with the nitro-gen gas(99.0%,BOC Gases).The gold-on-silicon electrodes were annealed for5min with a hydrogenflame prior to their use as a working electrode after they had been rinsed thor-oughly with methanol and deionized water.A platinum foil was used as a counter electrode and an Ag|AgCl(in sat-urated KCl)electrode as a reference electrode.PPyfilms were grown galvanostatically with an EG&G model273 potentiostat–galvanostat after the aqueous solution contain-ing0.10M pyrrole and0.10M electrolyte was purged with nitrogen for1h through an aqueous solution.The solution containing SDS was not purged due to the bubbles gener-ated during the purging process and,thus,the dodecylsulfate (DS−)doped PPyfilm was grown immediately after prepar-ing the solution.After electrochemical synthesis,thefilms were rinsed thoroughly with deionized water to remove ex-cess monomer,oligomer,and electrolyte molecules,and dried under vacuum at room temperature.A Molecular Imaging’s PicoSPM AFM with a current-sensing tip,CS-AFM,was used in a contact mode to simultaneously obtain topographical and current im-ages.The platinum/iridium-coated cantilevers(spring con-stant,0.31–0.41N/m)were purchased from nanosensors (/).The load force was main-tained below6nN to avoid damages of the tip and thefilms. During current–voltage measurements at given spots of the film,a load force was always maintained at6nN.A bias voltage between the substrate(gold electrode)and the con-ducting cantilever(which is grounded)was50mV during all the imaging experiments.Before imaging the PPyfilm cov-ered surfaces,they were purged with the high purity nitrogen gas(99.999%,BOC Gases)to minimize the moisture and water,and all the AFM experiments were carried out under the controlled nitrogen atmospheric environment.3.Results and discussionFig.1shows the topographic(top)and current(bot-tom)images obtained simultaneously for an identical area of1m×1m on a PPyfilm surface,which was galvano-statically grown by applying an anodic current of0.10mA for100s(=0.238mA/cm2or23.8mC/cm2)in aqueous so-lution containing0.10M p-TS(a),SDS(b),PSS(c),and LiClO4(d).The globular-shaped surface morphologies of the PPyfilms grown in aqueous and non-aqueous solutions are typical[20],although their sizes and shapes are slightly different from each other depending on growth conditions [18a,21].The sizes of DS−-doped PPy globules were signif-icantly larger than those on the other PPyfilm surfaces as can be seen from Fig.1b.Naoi et al.[22]reported columnar structures with globular surfaces when the PPyfilms were grown while the concentrations of the surfactants and anodic charges were varied.Thus,the large globules shown in Fig.1b were formed due to the interaction between DS−micelles and pyrrole monomers[22].The critical micelle concentration for SDS is8mM and large amounts of the micelles must have been formed in the SDS concentration used here[22].The current images shown in Fig.1,which were obtained at a50mV bias voltage,showed bright(high current-flowing) and dark(low current-flowing)regions;so the doping pro-cess of PPyfilms during their preparation in aqueous media is not as homogeneous as in acetonitrile solutions[18a].Sur-face potential images for polybithiophene and PPy recorded by the Kelvin probe method[23]and the current images of doped polyaniline[18b]recorded by CS-AFM also showed inhomogeneity of conducting polymers,but our recent re-D.-H.Han et al./Electrochimica Acta50(2005)3085–30923087Fig.1.Concurrently obtained topographic(top)and current(bottom)images with their cross-sectional analyses for the PPyfilms galvanostatically grown by applying0.1mA for100s(=23.8mC/cm2)in aqueous solution containing0.1M p-TS(a),SDS(b),PSS(c),and LiClO4(d).The scan area was1m×1m.sults have shown that relatively homogeneous surfaces were obtained for PPy when prepared in acetonitrile[18a].The PPyfilms shown in Fig.1are all inhomogenous and it ap-pears very difficult to obtain homogeneousfilms in aqueous media.In general,the conductivities of PPyfilms prepared in aqueous solutions are lower than those obtained in organic solvents[18a,24].p-TS-doped PPy,however,exhibited sig-nificantly high conductivities even when prepared in aqueous electrolyte solutions[24].The average conductance values obtained from the cross-sectional analyses of the current im-ages in Fig.1are4.76×10−6,1.46×10−6,1.41×10−6,and 4.10×10−9S for p-TS,SDS,PSS,and LiClO4,respectively, when calculated using Ohm’s law from the average currents read and the bias voltage of50mV.The average currents were calculated from those read across the cross sectional current profiles given under the current images,which were drawn using256data points across a1m distance.Thus each of these currents was obtained at every about4nm.In order to obtain the vertical conductivity of each PPy film,the contact area of the probe with thefilm was calculated using the Hertz theory[18b,25].The contact area between the tip and the sample was then calculated to be37.4nm2 under our experimental conditions,assuming the Poisson’s ratio of PPy as0.38just as for platinum and Young’s mod-ulus of all PPyfilms as the value(1.1GPa)of p-TS-doped PPy[26].Thefilm thickness was measured from the cross-sectional view of the SEM image,and the average thicknesses are101.7nm(±11.6)for p-TS,239.9nm(±59.7)for SDS,114.0nm(±26.8)for PSS,and53.1nm(±8.9)for LiClO4 doped PPy.The conductivities of the PPyfilms were calcu-lated from these thicknesses and the contact area to be129.4, 102.1,43.0,and5.8×10−2S/cm for p-TS-,SDS-,PSS-,and LiClO4-doped PPyfilms,respectively.These conductivities were calculated with an assumption that the currentflows vertically in one dimension from the substrate to the tip. While the path for the currentflow may not be exactly one-dimension,we believe this assumption is reasonable as the currentflows through the least resistive path of the shortest distance.In calculating the conductivity,the contact resis-tance between the tip and thefilm was not taken into account as not much is known about it.For this reason,the conductivi-ties we present here would be a bit smaller than the true values obtained with a four-probe method.Unfortunately,however, it is not possible to obtain conductivities in the vertical di-rection with the four-probe method.The bulk conductivities of PPy prepared in the aqueous solutions have been reported by Warren and Anderson[24a]to increase in the order of p-TS>SDS>PSS>LiClO4,which is in excellent agreement with our results.One more point to make here is that the thickness of the polymer prepared in these electrolyte so-lutions is in the order of SDS>PSS>p-TS>ClO4−for the identical amount of anodic charges(23.8mC/cm2)used for the preparation.This indicates how important the electrolyte molecules are in making up the polymer matrix.It is thus expected that the amount of the dopant material(counter an-ions in this case)included in the polymer matrix would be3088 D.-H.Han et al./Electrochimica Acta50(2005)3085–3092Fig.2.Current–voltage curves obtained at the highest current-flowing re-gions in the each current image of the PPyfilms in Fig.1.in the same order of the thickness as listed above unless the current efficiencies for polymer synthesis are affected by the electrolyte,which is highly unlikely.Fig.2shows the current–voltage(I–V)curves obtained at the highest current-flowing regions in each current image. The slopes of the I–V curves were5.56×103,5.25×103, 4.63×103,and7.84×102nA/V for p-TS-,SDS-,PSS-,and LiClO4-doped PPyfilms,respectively.The slope of the I–V curve for the ClO4−-doped PPy is much smaller than the others as can be seen in Fig.2.This agrees with the report that the PPyfilms prepared in a solution containing small dopant anions have a low conductivity with a tendency to lose their conductivities easily by exposure to the laboratory atmosphere[24a].A similar observation has been reported on a polyanilinefilm prepared in the perchloric acid electrolyte [18b].The effects of growth time,i.e.,the amount of anodic charges,on topographic and electrical properties have been studied when the PPyfilms were galvanostatically grown in0.10M p-TS at a constant applied current of0.10mA. Fig.3shows topographic(top)and current(bottom)images of PPyfilms galvanostatically grown for different growth pe-riods or different amounts of charges.As shown in Fig.3a, the surface of the gold-on-silicon electrode is almost fully covered with insulating thin layers at the veryfirst stage (at4.76mC/cm2)of the PPyfilm formation,although the current image shows some conductive areas.So,the elec-trode is probably covered with un-doped PPy or pyrrole oligomers at this stage,which is usually observed during PPy synthesis[27],with some conductive PPy islands.In efforts to verify this,we doped thefilm further at0.80and 0.90V for20s and recorded the topographical and current im-ages.The result was that the current increased significantly when doped at0.80V without noticeable changes in topo-graphical image.However,the current was decreased when doped at0.90V suggesting the PPyfilm was over-doped. This indicates that thefilm is covered with poorly doped PPy and relatively larger oligomer molecules rather than small oligomers,which would dissolve away when oxidized in so-lution.After40s(=9.52mC/cm2),a PPyfilm with more conduc-tive islands is obtained as shown in rge aggregates of grains whose areas and heights are broader(a few hundred nm)and higher(as high as about20nm)are seen to form at this stage.Interestingly,the aggregates of grains sticking up are shown to be less conductive than the lower valley re-gions.The grains might have grown on the more conductive spots of the PPyfilm in thefirst stage but the newly formed grains may not be doped with the limited amount of charges, as most of the anodic charges must have been used up for the growth of the grains.As the charge is further increased to16.7mC/cm2(electrolysis time,70s),the large aggregates of grains shown in Fig.3b start to disappear and much of the anodic charges are now used to dope the rather evenly grown film,resulting in larger average conductive areas across the film,as can be seen in Fig.3c.Note that the amount of charges used for thefilm shown in Fig.1a,which is23.8mC/cm2,is between those for Figs.3c and d under the otherwise the same experimental conditions.As more charges are spent(Fig.3d, 47.6mC/cm2),the conductive regions increase with an in-crease in the amount of anodic charges.The line average currents obtained from cross sectional profiles in the current images of Figs.1a and3are14.1,95.2,120.2,283.4,and 651.51nA for an applied bias voltage of50mV at20,40,70, 100,and200s,respectively.This indicates that the amount of doped PPy increases,as the deposition time is increased. Our results shown in Fig.3describe the PPy growth process as:(1)the electrode surface is covered with a thin insulat-ing layer of PPy during the very early stage of the growth with occasional conductive PPy islands(Fig.3a);(2)the PPy then grows laterally at these islands resulting in broadening of the insulating grains of neutral PPy to cover a large areas of the surface with a rather insulating PPyfilm(Fig.3b)and the grain boundaries eventually disappear(Fig.3c);and(3)finally the charges are used to dope the PPyfilm to make it more conductive(Figs.1a and3d).Thus,an appropriate time longer than200s is needed for the preparation of an evenly conductive PPyfilm.Depending on the applied current,a different overpoten-tial is applied to the electrode during the polymerization re-action and the doping level of PPy is changed accordingly. The PPyfilms shown in Figs.1d and4were prepared us-ing an identical charge density(=23.8mC/cm2)by applying0.10mA for100s(Fig.1d),0.5mA for20s(Fig.4a),and1.0mA for10s(Fig.4b).By applying these currents during the polymerization reaction,steady-state potentials of0.65, 0.80,and1.0V were measured,respectively,at the electrode. The cross-sectional line average currents were0.21,5.1,and 34.7nA for thefilms shown in Figs.1d,4a and b,respectively. These results indicate that a more conductivefilm is obtained when a higher current is used even though exactly the same amount of anodic charges is used.Boxall et al.reported that the PPyfilms required an increment in an applied voltage of 72±8mV to induce a tenfold increase in conductivity[28].D.-H.Han et al./Electrochimica Acta 50(2005)3085–30923089Fig.3.Topographic (top)and current (bottom)images (1m ×1m)with their cross-sectional analyses for the PPy films galvanostatically grown by applying 0.1mA for 20s (=4.76mC/cm 2)(a),for 40s (=9.52mC/cm 2)(b),for 70s (=16.66mC/cm 2)(c),and for 200s (=47.6mC/cm 2)(d)in aqueous solution containing 0.1M p -TS and 0.1M pyrrole.Our PPy films were prepared under different experimental conditions from those used by Boxall et al.and the increase in conductivity for the potential change was not the same as those expected from their relation,but our results indi-cate that a high oxidation overpotential is necessary to obtain a highly doped PPy film.The doping current and potential should also be optimized to obtain better conductive PPy films.Large globules are formed when a surfactant,SDS,is used as an electrolyte as shown in Figs.1b and 5.It is interesting to note from the current images shown in Figs.1b and 5a that the topologically lower borders of these globules arebetterFig.4.Current images (1m ×1m)with their cross-sectional analyses for the PPy films galvanostatically grown by applying 0.5mA for 20s (=23.8mC/cm 2)(a)and 1.0mA for 10s (=23.8mC/cm 2)(b)in aqueous solution containing 0.1M LiClO 4and 0.1M pyrrole.3090 D.-H.Han et al./Electrochimica Acta 50(2005)3085–3092Fig.5.PPy films galvanostatically grown by applying 0.1mA for 100s in the aqueous solution containing 0.1M SDS (Fig.1b and then de-doped at −0.6V for 5min (a)and for 15min (b)in the 0.1M SDS solution.Topographic (top)and current (bottom)images (1m ×1m)simultaneously obtained and their cross-sectional analyses.doped than their tops.When the PPy film was reduced at −0.60V for 5min,the current image shown in Fig.5a is very similar to that in Fig.1b,indicating that the borders were still in the doped state.The amount of current flowing,however,is significantly reduced compared with that of the as prepared PPy film shown in Fig.1b.The DS −-doped PPy is almost fully de-doped when reduced at −0.60V for 15min and almost no current flows over the entire area except for a few spots as can be seen in Fig.5b.In DS −-doped poly-mers,the cations,not anions,are known to be inserted/de-inserted during the doping/de-doping process [29].Hallik et al.reported that DS −ions could be easily removed from PPy in alcohol and acetonitrile,but the electrochemical re-duction in aqueous solution was not as effective in reducing the doping level [30].When compared with PPy prepared in acetonitrile [18a],the de-doping process of DS-doped PPy shown in Fig.5is very slow.The topographic images shown in Figs.1b and 5display that the sizes of PPy globulesare significantly reduced as the polymer is thoroughly de-doped (Fig.5b).This suggests that probably the large DS anions leave the PPy matrix upon slowly de-doping the poly-mer as in the case of non-aqueous media [30],which might have led to the collapse of the PPy structure resulting in changes in both the morphology and the electrical conductiv-ity;however,the counter cations of dodecylsulfate are known to be inserted during the de-doping in aqueous solutions [29].4.ConclusionsOur study demonstrates that morphological and electrical properties of PPy films electrochemically prepared in aque-ous media are affected by the solvent,electrolyte,current density,and deposition time (amount of charge spent).Of PPy films thus prepared,the p -TS-doped PPy film showedD.-H.Han et al./Electrochimica Acta50(2005)3085–30923091the highest conductivity while the ClO4−doped one the low-est.The PPyfilms prepared in aqueous electrolyte solutions were in general inhomogeneous in their electrical properties showing much poorer conductivities than those prepared in acetonitrile[18a].The electrolyte plays a very important role in determining the morphological and electrical properties of the PPyfilms.For example,SDS,which forms large micelles in aqueous media,helps form large globules with smaller do-mains not well connected with each other.On the other hand, ClO4−is homogeneously dispersed in the polymer matrix due to its lowest basicity,perhaps providing a high density polymerfilm.The p-TS-doped PPyfilm has the highest con-ductive state due most likely to its relatively high basicity in comparison with that of ClO4−and the high polarizability of the p-TS group.The relatively high basicity would allow the radical cations,i.e.,polarons,of PPy to associate with the dopants rather well,stabilizing the conductive states.These explain the extremes in morphological and electrical proper-ties of this series of PPyfilms we have seen in this study.The growth time dependence boils down to the indirect potential dependence as we have seen from Fig.4.The morphological and electrical characteristics of PPyfilms can be as diverse as the experimental conditions,and our study on the conductiv-ity changes depending on the experimental conditions offers the basis forfinding an optimal condition to prepare better PPyfilms.AcknowledgementsThis work was supported by the grant from the Korea Sci-ence and Engineering Foundation through the Center for Inte-grated Molecular Systems located at Postech and the graduate stipends were provided by the BK21program of the Korea Research Foundation.References[1](a)H.Shirakawa,E.J.Louis,A.G.MacDiarmid,C.K.Chiang,A.J.Heeger,mun.(1977)578;(b)H.Shirakawa,Angew.Chem.Int.Ed.40(2001)2574.[2](a)T.A.Skotheim,R.L.Elsenbaumer,J.R.Reynolds,Handbook ofConducting Polymers,vols.1/2,Marcel Dekker,New York,1997;(b)H.S.Nalwa,Handbook of Organic Conductive Molecules andPolymers,vols.1–4,Wiley,Chichester,UK,1997;(c)S.-M.Park,Handbook of Organic Conductive Molecules andPolymers,vol.3,Wiley,Chichester,UK,1997.[3](a)C.D.Dimitrakopoulos,P.R.L.Malenfant,Adv.Mater.14(2002)999;(b)G.Horowitz,Adv.Mater.10(1998)365;(c)H.E.A.Huitema,G.H.Gelinck,J.B.P.H.van der Putten,K.E.Kuijk,C.M.Hart,E.Cantatore,P.T.Herwig,A.J.J.M.van Breemen,D.M.de Leeuw,Nature414(2001)599.[4]A.Kraft,A.C.Grimsdale,A.B.Holmes,Angew.Chem.Int.Ed.37(1998)402.[5](a)W.U.Huynh,J.J.Dittmer,A.P.Alivisatos,Science295(2002)2425;(b)B.Sun,E.Marx,N.C.Greenham,Nano Lett.3(2003)961;(c)G.Yu,J.Gao,J.C.Hummelen,F.Wudl,A.J.Heeger,Science270(1995)1789.[6](a)A.A.Argun,A.Cirpan,J.R.Reynolds,Adv.Mater.15(2003)1338;(b)D.DeLongchamp,P.T.Hammond,Adv.Mater.13(2001)1455.[7](a)B.Crone,A.Dodabalapur,Y.-Y.Lin,R.W.Filas,Z.Bao,A.LaDuca,R.Sarpeshkar,H.E.Katz,W.Li,Nature403(2000)521;(b)C.J.Drury,C.M.J.Mutsaers,C.M.Hart,M.Matters,D.M.deLeeuw,Appl.Phys.Lett.73(1998)108.[8](a)C.Hagleitner,A.Hierlemann,nge,A.Kummer,N.Kerness,O.Brand,H.Baltes,Nature414(2001)293;(b)G.Chen,Z.Guan,C.T.Chen,L.Fu,V.Sundaresan,F.H.Arnold,Nat.Biotechnol.15(1997)354.[9](a)A.Cravino,N.C.Sariciftci,Nat.Mater.2(2003)360;(b)J.R.Bredas,S.Marder,Adv.Funct.Mater.12(2002)555.[10]Visit,for example,/(application notes).[11](a)T.W.Kelley,E.L.Granstrom,C.D.Frisbie,Adv.Mater.11(1999)261;(b)C.E.Gardner,J.V.Macpherson,Anal.Chem.74(2002)576A;(c)A.Salomon,D.Cahen,S.Lindsay,J.Tomfohr,V.B.Engelkes,C.D.Frisbie,Adv.Mater.15(2003)1881.[12](a)X.D.Cui,A.Primak,X.Zarate,J.Tomfohr,O.F.Sankey,A.L.Moore,T.A.Moore,D.Gust,S.Lindsay,Science294(2001)571;(b)G.Leatherman, E.N.Durantini, D.Gust,T.A.Moore, A.L.Moore,S.Stone,Z.Zhou,P.Rez,Y.Z.Liu,S.Lindsay,J.Phys.Chem.B103(1999)4006.[13](a)J.Zhao,J.J.Davis,M.S.P.Sansom,A.Hung,J.Am.Chem.Soc.126(2004)5601;(b)L.Cai,H.Tabata,T.Kawai,Nanotechnology12(2001)211.[14](a)H.Dai,E.W.Wong,C.M.Lieber,Science272(1996)523;(b)P.J.de Pablo,C.Gomez-Navarro,M.T.Martinez,A.M.Benito,W.K.Maser,J.Colchero,J.Gomez-Herrero,A.M.Baro,Appl.Phys.Lett.80(2002)1462;(c)J.Li,R.Stevens,L.Delzeit,H.Tee Ng,A.Cassell,J.Han,M.Meyyappan,Appl.Phys.Lett.81(2002)910.[15](a)D.J.Wold,C.D.Frisbie,J.Am.Chem.Soc.123(2001)5549;(b)D.J.Wold,C.D.Frisbie,J.Am.Chem.Soc.122(2000)2970;(c)D.J.Wold,R.Haag,M.A.Rampi,C.D.Frisbie,J.Phys.Chem.B106(2002)2813;(d)J.M.Beebe,V.B.Engelkes,ler,C.D.Frisbie,J.Am.Chem.Soc.124(2002)11268.[16](a)B.Alperson,S.Cohen,I.Rubinstein,G.Hodes,Phys.Rev.B52(1995)R17017;(b)B.Alperson,I.Rubinstein,G.Hodes,Phys.Rev.B63(2001)081303.[17](a)M.J.Loiacono,E.L.Granstrom,C.D.Frisbie,J.Phys.Chem.B102(1998)1679;(b)W.I.Park,G.-C.Yi,J.-W.Kim,S.-M.Park,Appl.Phys.Lett.82(2003)4358;(c) A.V.Tivanski,J.E.Bemis, B.B.Akhremitchev,H.Liu,G.C.Walker,Langmuir19(2003)1929.[18](a)H.J.Lee,S.-M.Park,J.Phys.Chem.B108(2004)1590;(b)D.-H.Han,S.-M.Park,J.Phys.Chem.B108(2004)13921;(c)H.J.Lee,S.-M.Park,J.Phys.Chem.B108(2004)16365.[19](a)J.Planes,F.Houze,P.Chretien,O.Schneegans,Appl.Phys.Lett.79(2001)2993;(b)S.K.Saha,Y.K.Su,C.L.Lin,D.W.Jaw,Nanotechnology15(2004)66;(c)J.G.Park,S.H.Lee,B.Kim,Y.W.Park,Appl.Phys.Lett.81(2002)4625;(d)C.Ionescu-Zanetti,A.Mechler,S.A.Carter,l,Adv.Mater.16(2004)385.[20](a)J.M.Ko,H.W.Rhee,S.-M.Park,C.Y.Kim,J.Electrochem.Soc.92(1990)5274;(b)S.-J.Choi,S.-M.Park,J.Electrochem.Soc.149(2002)E26.[21](a)M.F.Su´a rez,pton,J.Electroanal.Chem.426(1999)211;3092 D.-H.Han et al./Electrochimica Acta50(2005)3085–3092(b)B.J.Hwang,R.Santhanam,Y.-L.Lin,J.Electrochem.Soc.147(2000)2252.[22]K.Naoi,Y.Oura,M.Maeda,S.Nakamura,J.Electrochem.Soc.142(1995)417.[23](a)O.A.Semenikhin,L.Jiang,T.Iyoda,K.Hashimoto, A.Fu-jishima,J.Phys.Chem.100(1996)18603;(b)O.A.Semenikhin,L.Jiang,T.Iyoda,K.Hashimoto,A.Fu-jishima,Electrochim.Acta42(1997)3321;(c)J.N.Barisci,R.Stella,G.M.Spinks,G.G.Wallace,Electrochim.Acta46(2000)519.[24](a)L.F.Warren,D.P.Anderson,J.Electrochem.Soc.134(1987)101;(b)K.J.Wynne,G.B.Street,Macromolecules18(1985)2361.[25](a)J.Isrraelachvili,Intermolecular and Surface Forces,AcademicPress,London,1992;(b)E.Riedo,H.Brune,Appl.Phys.Lett.83(2003)1986.[26]L.Bay,N.Mogensen,S.Skaarup,P.Sommer-Larsen,M.Jørgensen,K.West,Macromolecules35(2002)9345.[27](a)G.Zotti,S.Martina,G.Wegner,A.-D.Schl¨u ter,Adv.Mater.4(1992)798;(b)R.John,G.G.Wallace,J.Electroanal.Chem.306(1991)157.[28]D.Boxall,R.Osteryoung,J.Electrochem.Soc.151(2004)E41.[29]J.M.Pernaut,R.C.D.Peres,V.F.Juliano,M.A.De Paoli,J.Elec-troanal.Chem.274(1989)225.[30]J.Hallik,A.Alumaa,V.Sammelselg,J.Tamm,J.Solid State Elec-trochem.5(2001)265.。