Atomistic Simulations of Nanotube Fracture
Web of Science检索规则

Web of Science检索规则目录大写字母 (2)检索运算符 (2)检索运算符 (2)布尔运算符 (3)AND (3)OR (3)NOT (3)来源出版物名称中的布尔运算符 (3)组织名称中的布尔运算符 (4)位置限定运算符 (4)NEAR/x (4)SAME (5)检索运算符优先顺序 (6)使用括号 (7)通配符 (9)通配符 (9)有关通配符的一般规则 (9)通配符、连字号和撇号 (11)有用窍门 (11)短语检索 (13)括号 (14)撇号 (14)连字号 (15)您知道吗 (15)大写字母不区分大小写:可以使用大写、小写或混合大小写。
例如,AIDS、Aids 以及aids 可查找相同的结果。
检索运算符在各个检索字段中,检索运算符(AND、OR、NOT、NEAR 和SAME)的使用会有所变化。
例如:●在“主题”字段中可以使用AND,但在“出版物名称”或“来源出版物”字段中却不能使用。
●您可以在多数字段中使用NEAR,但不要在“出版年”字段中使用。
●在“地址”字段中可以使用SAME,但不能在其他字段中使用。
请记住,使用检索运算符时不区分大小写。
例如,OR、Or 和or 返回的结果相同。
我们在示例中都使用大写字母只是为了样式美观起见。
注:Korean Journal Database 没有将SAME 包括为检索运算符。
检索运算符逻辑运算符AND、OR、NOT、NEAR 和SAME 可用于组配检索词,从而扩大或缩小检索范围。
请记住,使用检索运算符时不区分大小写。
例如,OR、Or 和or 返回的结果相同。
我们在示例中都使用大写字母只是为了样式美观起见。
注:Korean Journal Database 没有将SAME 包括为检索运算符。
此帮助页面包含以下主题信息。
●布尔运算符●位置限定运算符●检索运算符优先顺序●括号的使用布尔运算符AND使用AND 可查找包含被该运算符分开的所有检索词的记录。
Web of science-new(文献检索)

通配符
• 所有可以使用单词和短语的检索字段均可 以使用通配符。 它们可在检索式中表示未 知字符。 • 星号 (*) 表示任何字符组,包括空字符。 • 问号 (?) 表示任意一个字符。 • 美元符号 ($) 表示零或一个字符。
有关通配符的一般规则
• • • • • • • • • • • 在 Web of Science 的所有产品数据库中,可以在如下检索字段中使用左截词符: “主题”、“标题”、“入藏号”和“识别代码”。 在“主题”和“标题”检索中,如果使用左截词符,那么必须在通配符后至 少输入 3 个字符。 例如:*bio 在“入藏号”和“识别代码”检索中,如果使用左截词符,那么必须在通配 符后至少输入 1 个字符。 例如:*2307 在“主题”和“标题”检索中,如果使用右截词符,那么必须在通配符前至 少输入 3 个字符。 例如:bio* 在“作者”检索中,检索姓氏时必须在通配符前输入至少 2 个字符,例如: sm* 通配符可位于检索词的中间。 例如,odo$r 可查找到 odor 和 odour。 您不能在以下字符后面使用通配符:特殊字符 (/ @ #) 和标点符号 (. , : ; !)。 您不能在出版年检索中使用通配符。 例如,可以使用 2007,但不能使用 200*。 如果通配符出现在单词或名称中,您就不能对其进行检索。 例如,检索式 TS=E*Trade OR TS="E*Trade" 无法返回有关此公司的记录。 不能在引起的检索内使用美元符号 ($)。 在截词后匹配范围很广的情况下,请尽量避免使用通配符。 例如,检索式 UT=*2 或 UT=*2* 或 UT=*22 或 UT=*22* 返回的结果可能并不完全(或者没有 结果),这是因为产品数据库中与之匹配的记录太多。
Web of Science
Interactive Molecular Dynamics for Nanomechanical

Interactive Molecular Dynamics for Nanomechanical and Nanochemical ExperimentsAxel KohlmeyerMolecular Dynamics●Simulate motion of atoms and molecules according to physical models: classical (empirical) or quantum (electrons and/or core)●Microscopic look into the atomic scale;simulated experiment with perfect control.●Connection to macroscopic world throughstatistical mechanics or thermodynamics:=> molecular level interpretation of thermodynamic quantities●Beyond two particles chaotic => coupled differential equations, solved numerically => model and CPU determine time scalesTypical Molecular Dynamics Work Flow●Setup: construct initial geometry, idealized or assembled pieces ●Equilibration: relax and propagate until the desiredthermodynamical state is reached (or close enough)●Production: propagate atoms and record statisticallyrelevant data of system evolution while equilibrium is maintained ●Analysis & Visualization: done “off-line” (i.e. after production)●Statistical analysis to derive structural or thermodynamicalproperties to confirm, guide or predict experiment(s).●Visual inspection of structural changes or “special” eventsStudying 'Rare Events' in Molecular Dynamics●Time scales in MD simulations are limited by the fastest motion => total time that can be studied is restricted.●Size of system is finite => large (local) energy fluctuations rare => events that have (free) energy barriers are often 'impossible'●Various 'biasing methods' exist to make those events possible=> biasing needs to be programmed, cannot “just play around”●Programmed biasing or steering works best for simple moves: 'collective variables' => well defined for statistical analysis●Difficult to study “What would happen if?”-scenarios on the flyEvolution of Interactive MD (IMD)●Origin in steered molecular dynamics (SMD) by adding run-time visualization to monitor the progress of steering●Next step: Interactive determination of steering forces through a pointer device (2d: mouse, 3d: 3d-joystick, 3d-mouse, WiiMote)●Then: better visual feedback with stereo displayeven better with 'immersive visualization', e.g. CAVE●Full IMD framework with support for haptic devicesadding force feedback to 3d tracking => VMD●Limited adoption due to cost and disruptive nature (VR facility)Interactive MD Applications Examples●Education and Outreach:Unique experience through immersive visualizationand force feedback allows students to “grasp” MD simulations●Simulation Monitoring:Visualization can be connected to ongoing production run●Simulation Preparation:Components of an MD simulation system can be interactivelyrearranged (“sculpting”) as needed while close to equilibrium●Nano-mechanical or -chemical experimentsThe IMD Infrastructure in VMDMD Engine:NAMD,Gromacs,HOOMD-blue,LAMMPS, ...Visualization& VR Client:VMDCoords FeedbackTrackerForcesVR Server:VRPN(-ICMS),Haptic deviceSocketSocketTime Scale Issues with IMDSimulated System:●Atom velocity: ~100 m/s●MD Time step: ~10-15 sTime and length scales of simulation & visualization are coupled Typical parameters for a smooth IMD configuration:Tangible System:●Compute time: ~1 ms / step●Atom movement: ~10-10 m / s Newton's second law: F = m a=> faster running MD=> less IMD force needed=> objects appear to be lighterTo move large object:=> run MD faster (parallel,GPU)=> or scale applied IMD force=> or change particle massMore Time Scale Issues with IMD●How realistic should an IMD simulation/visualization be?If too large IMD force, too small particle mass => unphysicalmay be tolerated for educational use, unacceptable for research●Local movements (solvent) limit length of time step●Compute capability limits speed of MD code●Visualization update rate limits position update frequency=> At higher MD speed, less frequent IMD position updates => position data becomes more “noisy”, need denoising filter●What if I want to look at “slow” processes? Move large objects?Recorded IMD Demos with “Falcon” Controller●“Stick the buckyballs intothe nanotube demo●Virtual vacuum AFM demowith 3 types of LJ particlesRevived Interest in Interactive MD●A smooth IMD visualization needs about 20-30 frames/s●Significant compute power for fast MD on all but the smallest systems●A powerful graphics workstation with stereo capability is required=> a dedicated and expensive facility was needed that few locations could afford and that would require to schedule access ahead of time ●3d screens affordable (3d-TVs, Scanline polarized LCD)●High performance graphics with 3d capability available (games)●Multi-core CPUs and GPUs turn workstations into clusters●Affordable controllers (Falcon (gaming), smartphones (6DOF))VRPN and VMD Enhancements●Support in VRPN for Novint Falcon as haptic 3DOF device●Implement Tracker and Button classes as sending devices●Implement ForceDevice class as receiving device●Use libnifalcon ( ) to access Falcon●Implement damping scheme for smooth force constraint updates(force update in device at 1000Hz, update from VMD less frequent)●Enhancements in VMD●Support for enforced TCP only communication with VRPN server forusing remote visualization facility via VirtualGL (LRZ Munich)●Support for whole residue mode with “tug” toolLAMMPS Enhancements●OpenMP (LAMMPS-ICMS) and GPU (GPULAMMPS) acceleration for non-bonded interactions=> faster MD for smaller (OpenMP) or larger (GPU) systems●Improvements in IMD module (fix imd):●Listening for IMD force input in separate thread.No more need to “drain” all incoming IMD communication data●Sending of coordinate data in separate threadNo more need to wait when large IMD data is sent over slow link●Addition of Savitzky-Golay filtering of coordinate dataDenoises coordinate updates with large Δt with minimal distortionIMD Appliance Concept●Combines:●Multi-core/CPU/GPU compute●Stereo capable visualization●3d display●Haptic device●Software●No special facility needed●Commodity components●Kiosk mode for educationPerspectives●Advances in GPU acceleration will expand applicability●GPU acceleration more effective in compute intense models=> nano-mechanics (Tersoff, Stillinger-Weber, AIREBO)=> nano-chemistry (Reaxx)●More approximate models for large changes(temporary coarse graining)●More experiments with example applications or demos needed ●IMD protocol expansions and optimizations●VRPN-ICMS improvements (multi-Falcon support, alternate grip)References●VMD: /Research/vmd●LAMMPS: /LAMMPS-ICMS (code gets merged to LAMMPS when stable): /site/akohlmey/software/lammps-icms●VRPN: /Research/vrpn/VRPN-ICMS (code will be merged into VRPN when stable): /site/akohlmey/software/vrpn-icms●GPULAMMPS (GPU acceleration with CUDA for LAMMPS):/p/gpulammps/●Michael L. Klein (freedom and funding, NSF CHE 09-46358)●John Stone, Klaus Schulten (VMD and much more)●Steve J. Plimpton, Paul S. Crozier and many others (LAMMPS)●Russ Taylor (VRPN), Kyle Machulis (libnifalcon)●Tom Anderson, Brandon Williams, Novint, (free Falcons) ...and...●Greg & Gary Scantlen, Creative Consultants,(contacts, encouragement, perseverance, 3D-buckyballs) Make sure you try out the “Nano Dome”, Booth 29/30.Acknowledgements。
《氮化物半导体量子点中的束缚极化子、激子及应变效应》范文

《氮化物半导体量子点中的束缚极化子、激子及应变效应》篇一一、引言随着科技的快速发展,半导体材料及其在电子和光电子器件中的应用已经成为当今科技进步的关键领域。
其中,氮化物半导体因其独特的光电性能在光电子器件、纳米电子器件等领域具有广泛的应用前景。
近年来,氮化物半导体量子点因其尺寸小、量子效应显著等特点,在光电器件中展现出独特的性能。
本文将重点探讨氮化物半导体量子点中的束缚极化子、激子以及应变效应的特性和影响。
二、氮化物半导体量子点中的束缚极化子束缚极化子是氮化物半导体量子点中一种重要的电子激发态。
在量子点中,由于尺寸效应和量子限域效应,电子和空穴的波函数发生明显的变化,形成束缚态。
这种束缚态的电子和空穴由于库仑相互作用而形成极化子。
束缚极化子的存在对氮化物半导体量子点的光学性质和电学性质具有重要影响。
首先,束缚极化子能够影响氮化物半导体量子点的能级结构。
由于电子和空穴的相互作用,量子点的能级发生分裂,形成一系列分立的能级。
这些能级的存在使得量子点具有独特的光学吸收和发射特性。
其次,束缚极化子还对氮化物半导体量子点的光电转换效率产生影响。
由于极化子的存在,量子点中的光生载流子更容易被分离和传输,从而提高光电转换效率。
三、氮化物半导体量子点中的激子激子是氮化物半导体量子点中另一种重要的电子激发态。
当光子能量大于半导体材料的带隙时,光子被吸收并激发出电子和空穴对,形成激子。
激子的存在对氮化物半导体量子点的发光性能具有重要影响。
首先,激子能够影响氮化物半导体量子点的发光颜色。
由于激子的能级与量子点的能级相匹配,激子的复合发光可以产生特定颜色的光。
通过调节激子的能级和数量,可以实现对氮化物半导体量子点发光颜色的调控。
其次,激子还对氮化物半导体量子点的发光效率产生影响。
激子的复合发光是发光效率的主要来源,因此激子的数量和寿命直接影响到量子点的发光效率。
通过优化激子的产生和复合过程,可以提高氮化物半导体量子点的发光效率。
WebofScience检索规则
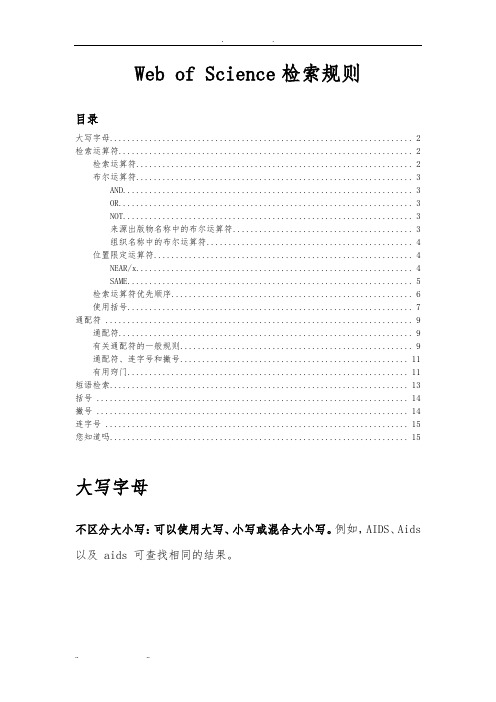
Web of Science检索规则目录大写字母 (2)检索运算符 (2)检索运算符 (2)布尔运算符 (3)AND (3)OR (3)NOT (3)来源出版物名称中的布尔运算符 (3)组织名称中的布尔运算符 (4)位置限定运算符 (4)NEAR/x (4)SAME (5)检索运算符优先顺序 (6)使用括号 (7)通配符 (9)通配符 (9)有关通配符的一般规则 (9)通配符、连字号和撇号 (11)有用窍门 (11)短语检索 (13)括号 (14)撇号 (14)连字号 (15)您知道吗... .. (15)大写字母不区分大小写:可以使用大写、小写或混合大小写。
例如,AIDS、Aids 以及 aids 可查找相同的结果。
检索运算符在各个检索字段中,检索运算符(AND、OR、NOT、NEAR 和 SAME)的使用会有所变化。
例如:●在“主题”字段中可以使用 AND,但在“出版物名称”或“来源出版物”字段中却不能使用。
●您可以在多数字段中使用 NEAR,但不要在“出版年”字段中使用。
●在“地址”字段中可以使用 SAME,但不能在其他字段中使用。
请记住,使用检索运算符时不区分大小写。
例如,OR、Or 和 or 返回的结果相同。
我们在示例中都使用大写字母只是为了样式美观起见。
注:Korean Journal Database 没有将 SAME 包括为检索运算符。
检索运算符逻辑运算符 AND、OR、NOT、NEAR 和 SAME 可用于组配检索词,从而扩大或缩小检索围。
请记住,使用检索运算符时不区分大小写。
例如,OR、Or 和 or 返回的结果相同。
我们在示例中都使用大写字母只是为了样式美观起见。
注:Korean Journal Database 没有将 SAME 包括为检索运算符。
此帮助页面包含以下主题信息。
●布尔运算符●位置限定运算符●检索运算符优先顺序●括号的使用布尔运算符AND使用 AND 可查找包含被该运算符分开的所有检索词的记录。
晶体自范性和自生成模板法结合生长组装多级纳米结构_谢毅

570
中国科学技术大学学报
第 38 卷
acted as the suppo rt fo r the co nst ruction of rutile TiO 2 3D hollow nanostructures . F urthermo re , VOOH hollo w “ dandelions” w ere sy nthesized owi ng t o the planar sheet nature of the bui lding blo cks in the newphased VOOH and the in-si tu produced N 2 gas bubbles as t he templates t hat acted as the sheet-like nanouni t ssupport er . Also , tit anat e 3D t ubular hierarchit ect ures w ere successfully prepared undergoing t he self-produced template methodolog y coupled w it h precurso r tem plat ing approach based on the similar st rategy . T he appearance of tit anat e nanof lakes i s actually the o ut w ard em bodiment o f the internal crystal st ructure , w hile the sacri ficed t em plat ing ef fect of the int ermediate precurso r of T iO x Cl2 -2x ( EN ) y is w ell underst ood . Key words : crystal g ro w th ; self-limi tatio n prope rty ;self-produced t emplate st rategy ; t hree-dimensi onal hie ra rchi tectures ; cry st al st ruct ure
Material Studio简介

结晶学、晶粉衍射以及材料特性等。
主要模块:
建模模块 计算和分析模块
Amorphous Cell
Blends
GULP MesoDyn Morphology
CASTEP
Visualizer
Conformers DMol3
Onetep
Polymorph QMERA Reflex Synthia
DPD
Discover
实例4. Understanding the Properties (structural, mechanical, vibrational, and electronic) of Carbon and Boron-nitride Nanotubes
Phys. Rev. B, 2003, 67, 245404
Reflex Plus 模块: 在Reflex标准功能的基础上加入已被广泛验证的Powder Solve 技术,提供了一套可以从高质量的粉末衍射数据确定晶体结构 的完整工具。包括粉末指标化、Pawley精修、解结构以及 Rietveld精修。
Reflex QPA 模块: 利用粉末衍射数据及Rietveld方法进行定量相分析的强大工具, 可以通过多相样品的粉末衍射图判定不同组成成分相对比例的。 用于化学品或医药工业中有机或无机材料组成成分的确定。
Equilibria
Forcite
VAMP
Gaussian
Visualizer:图形化计算模型的构建模块 晶胞,分子,晶体表面,纳米结构,聚合物 构建计算的模型
TiO2(111)
锐钛矿TiO2
Pt(110)-CO(2x1)
碳纳米管
TiO2纳米棒
Amorphous Cell:用于对无定形材料的性质研究
ViDESmanual

3
4
CONTENTS
License term
Copyright c 2004-2008, G. Fiori, G. Iannaccone, University of Pisa All rights reserved.
Redistribution and use in source and binary forms, with or without modification, are permitted provided that the following conditions are met: • Redistributions of source code must retain the above copyright notice, this list of conditions and the following disclaimer. • Redistributions in binary form must reproduce the above copyright notice, this list of conditions and the following disclaimer in the documentation and/or other materials provided with the distribution. • All advertising materials mentioning features or use of this software must display the following acknowledgement: ” This product includes software developed by G.Fiori and G.Iannaccone at University of Pisa” • Neither the name of the University of Pisa nor the names of its contributors may be used to endorse or promote products derived from this software without specific prior written permission. THIS SOFTWARE IS PROVIDED BY G.FIORI AND G.IANNACCONE ”AS IS” AND ANY EXPRESS OR IMPLIED WARRANTIES, INCLUDING, BUT NOT LIMITED TO, THE IMPLIED WARRANTIES OF MERCHANTABILITY AND FITNESS FOR A PARTICULAR PURPOSE ARE DISCLAIMED. IN NO EVENT SHALL G.FIORI AND G.IANNACCONE BE LIABLE FOR ANY DIRECT, INDIRECT, INCIDENTAL, SPECIAL, EXEMPLARY, OR CONSEQUENTIAL DAMAGES (INCLUDING, BUT NOT LIMITED TO, PROCUREMENT OF SUBSTITUTE GOODS OR SERVICES; LOSS OF USE, DATA, OR PROFITS; OR BUSINESS INTERRUPTION) HOWEVER CAUSED AND ON ANY THEORY OF LIABILITY, WHETHER IN CONTRACT, STRICT LIABILITY, OR TORT (INCLUDING NEGLIGENCE OR OTHERWISE) ARISING IN ANY WAY OUT OF THE USE OF THIS SOFTWARE, EVEN IF ADVISED OF THE POSSIBILITY OF SUCH DAMAGE.
- 1、下载文档前请自行甄别文档内容的完整性,平台不提供额外的编辑、内容补充、找答案等附加服务。
- 2、"仅部分预览"的文档,不可在线预览部分如存在完整性等问题,可反馈申请退款(可完整预览的文档不适用该条件!)。
- 3、如文档侵犯您的权益,请联系客服反馈,我们会尽快为您处理(人工客服工作时间:9:00-18:30)。
T. Belytschko 1 , S.P. Xiao2 , G.C. Schatz3 and R. Ruoff4 Department of Mechanical Engineering 2145 N Sheridan Rd Northwestern University Evanston, IL 60208
1. 2. 4.
Walter P Murphy Professor and Chair of Mechanical Engineering, tedbelytshko@ Research Assistant Professor of Chemistry Professor of Mechanical Engineering
ABSTRACT The fracture of carbon nanotubes is studied by atomistic simulations. The fracture behavior is found to be almost independent of the separation energy and to depend primarily on the inflection point in the interatomic potential. The range of fracture strains compares well with experimental results, but the predicted range of fracture stresses is markedly higher than observed. Various plausible small-scale defects do not suffice to bring the failure stresses into agreement with available experimental results. As in the experiments, the fracture of carbon nanotubes is predicted to be brittle. The results show moderate dependence of fracture strength on chirality. 1. INTRODUCTION We report here molecular mechanics and molecular dynamics studies of the failure of nanotubes. Nanotubes, since their discovery in 1991[1], have attracted much interest because of their ability to sustain large deformations and their great stiffness and possible high strength. The ability of carbon nanotubes to sustain large bending deformations has been observed experimentally and verified by molecular dynamics studies[2][3][4] . Molecular dynamics studies have also replicated the ability of nanotubes to sustain very distorted configurations with only elastic deformations and no creation of atomic defects[2][3][5][6]. Previous molecular mechanics simulations predict that ideal single-walled carbon nanotubes should possess extremely high tensile strengths. The strain at tensile failure has been predicted to be as high as ~30% for SWCNTs[7] by molecular mechanics. However, in the only experiments[8] reported so far, the tensile failure strain is usually between ~10% to ~13% and as low as 2%. A recent study[9] of SWCNTs with the tight binding approximation describes a mechanism of defect nucleation in which two hexagon pairs are converted, through a Stone-Wales bond rotation, into 5/7/7/5 (pairs of pentagon-heptagon) defects. It was hypothesized that at high temperatures, plastic response may occur due to separation and glide of these 5/7/7/5 defects whereas at lower temperature this may result in fracture[9][10][11]. Molecular simulations of fracture are now quite commonplace. The earliest atomistic simulations of fracture were reported in the early 1970's[12][13][14] and many materials have been
1
investigated. Works closely related to this are Shenderova et al[15] who examined the fracture of polycrystalline diamond, and Omeltchenko et al[16] who studied notched graphene. In this paper, the fracture of carbon nanotubes is studied by molecular mechanics and dynamics. We show that the experimental results of Yu et al[8] for the fracture strain and stress of carbon nanotubes can be reproduced reasonably well by molecular mechanics. Furthermore, we show that some of the variability of failure strains reported by Yu et al[8] can be partially explained by the presence of defects and variations due to chirality. The simulations always exhibit brittle fracture, which also agrees with these experiments. Nanotubes are particularly attractive for studying fracture by molecular mechanics because they are single molecules with all atoms joined by identical bonds. Thus the degree of heterogeneity and variety of length scales found in fracture of most materials is absent in nanotubes. The major defects which have been postulated are the previously mentioned StoneWales defects that are of the order of several bond lengths. In experiments performed in the TEM environment, it is possible for single atoms to be ejected by impact, see Smith[17] and Barnhart[18] , but there are also small-scale defects. Of course, the interpretation of fracture simulations by atomistic models must be treated with care, since the reconfiguration of bonds is not treated. However, we show that for small initial defects, the fracture strength depends primarily on the inflection point of the interatomic energy and is almost independent of the dissociation energy. Since the inflection strain occurs substantially before the strain associated with of bond breaking, where the formation of other bonds is expected, these results may give the correct picture of fracture at moderate temperatures (0 ~ 500 K ). 2. COMPUTATIONAL METHODS We used standard molecular mechanics and molecular dynamics methods. By molecular mechanics, we refer to methods where the equilibrium configuration of the model system is sought by minimizing the energy, which consists of the sum of the interatomic potentials minus any work by external forces. Such methods imply a temperature of 0 K and cannot account for the effects of temperature. In molecular dynamics methods, the momentum equations are integrated in time for the system of atoms with interatomic forces given by the interatomic potential. Thus the effects of nonzero temperatures are included, although there are always limitations of such predictions due to the statistical nature of molecular motion for systems of bonded atoms. The interatomic bonds in nanotubes are hybridized sp 2 bonds. In most of our calculations, the interatomic potential used is a modified Morse potential function in which a bond anglebending is added E = Estretch + E angle (1)