内燃机燃烧原理
内燃机原理内燃机的工作循环
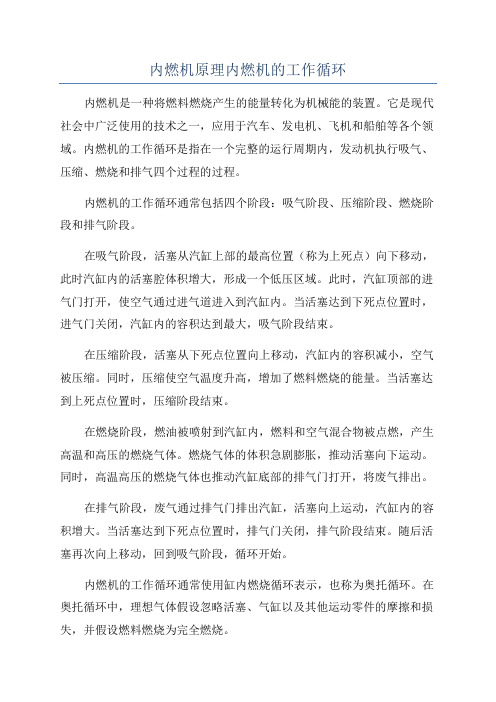
内燃机原理内燃机的工作循环内燃机是一种将燃料燃烧产生的能量转化为机械能的装置。
它是现代社会中广泛使用的技术之一,应用于汽车、发电机、飞机和船舶等各个领域。
内燃机的工作循环是指在一个完整的运行周期内,发动机执行吸气、压缩、燃烧和排气四个过程的过程。
内燃机的工作循环通常包括四个阶段:吸气阶段、压缩阶段、燃烧阶段和排气阶段。
在吸气阶段,活塞从汽缸上部的最高位置(称为上死点)向下移动,此时汽缸内的活塞腔体积增大,形成一个低压区域。
此时,汽缸顶部的进气门打开,使空气通过进气道进入到汽缸内。
当活塞达到下死点位置时,进气门关闭,汽缸内的容积达到最大,吸气阶段结束。
在压缩阶段,活塞从下死点位置向上移动,汽缸内的容积减小,空气被压缩。
同时,压缩使空气温度升高,增加了燃料燃烧的能量。
当活塞达到上死点位置时,压缩阶段结束。
在燃烧阶段,燃油被喷射到汽缸内,燃料和空气混合物被点燃,产生高温和高压的燃烧气体。
燃烧气体的体积急剧膨胀,推动活塞向下运动。
同时,高温高压的燃烧气体也推动汽缸底部的排气门打开,将废气排出。
在排气阶段,废气通过排气门排出汽缸,活塞向上运动,汽缸内的容积增大。
当活塞达到下死点位置时,排气门关闭,排气阶段结束。
随后活塞再次向上移动,回到吸气阶段,循环开始。
内燃机的工作循环通常使用缸内燃烧循环表示,也称为奥托循环。
在奥托循环中,理想气体假设忽略活塞、气缸以及其他运动零件的摩擦和损失,并假设燃料燃烧为完全燃烧。
内燃机的工作循环会受到多种因素的影响,如空气质量、燃料质量、点火时机、气门的开闭控制等。
通过调整和优化这些因素,可以提高内燃机的功率输出和燃料效率。
总结起来,内燃机的工作循环是通过吸气、压缩、燃烧和排气四个过程来完成的。
内燃机通过燃烧产生的高温高压气体推动活塞运动,将燃料的化学能转化为机械能。
内燃机的工作循环的优化和改进是实现高效能、低排放的关键。
内燃机工作原理
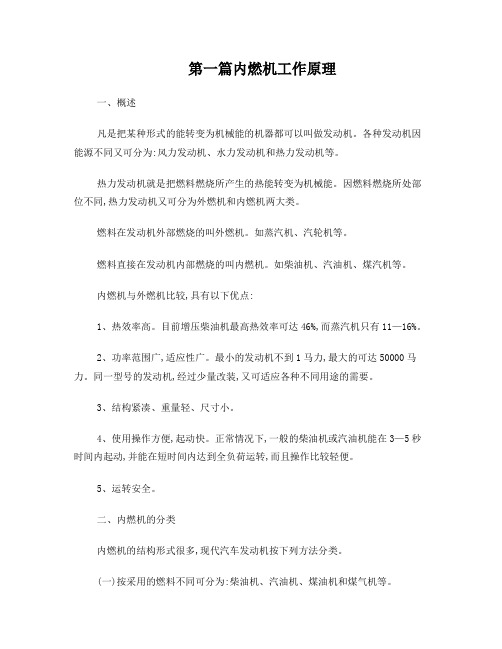
第一篇内燃机工作原理一、概述凡是把某种形式的能转变为机械能的机器都可以叫做发动机。
各种发动机因能源不同又可分为:风力发动机、水力发动机和热力发动机等。
热力发动机就是把燃料燃烧所产生的热能转变为机械能。
因燃料燃烧所处部位不同,热力发动机又可分为外燃机和内燃机两大类。
燃料在发动机外部燃烧的叫外燃机。
如蒸汽机、汽轮机等。
燃料直接在发动机内部燃烧的叫内燃机。
如柴油机、汽油机、煤汽机等。
内燃机与外燃机比较,具有以下优点:1、热效率高。
目前增压柴油机最高热效率可达46%,而蒸汽机只有11—16%。
2、功率范围广,适应性广。
最小的发动机不到1马力,最大的可达50000马力。
同一型号的发动机,经过少量改装,又可适应各种不同用途的需要。
3、结构紧凑、重量轻、尺寸小。
4、使用操作方便,起动快。
正常情况下,一般的柴油机或汽油机能在3—5秒时间内起动,并能在短时间内达到全负荷运转,而且操作比较轻便。
5、运转安全。
二、内燃机的分类内燃机的结构形式很多,现代汽车发动机按下列方法分类。
(一)按采用的燃料不同可分为:柴油机、汽油机、煤油机和煤气机等。
(二)按完成一个工作循环的冲程数可分为:四冲程发动机活塞重复四个冲程完成一个工程循环。
二冲程发动机活塞重复二个冲程完成一个工程循环。
(三)按气缸冷却方式可分为:水冷发动机和风冷发动机。
(四)按发动机气缸数可分为:单缸发动机和多缸发动机。
(五)按燃料在气缸内的着火方式可分为:压燃式发动机利用气缸内被压缩的空气所产生的高温高压使燃料着火燃烧。
柴油机就属于这种着火方式。
点燃式发动机利用外界热源(如电火花)点燃燃料,使其着火燃烧。
如汽油机、煤油机、煤气机都属于这种着火方式。
(六)按用途可分为:固定式发动机发动机用作固定作业的动力,如发电、排灌、农产品加工等作业。
移动式发动机发动机用作移动机械的动力,如汽车的发动机。
(七)按发动机转速或活塞平均速度可分为:高速发动机、中速发动机和低速发动机。
内燃机燃烧机理的研究与模拟
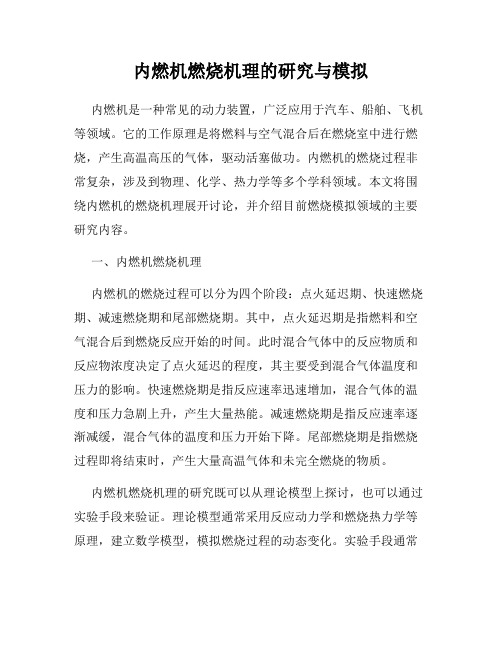
内燃机燃烧机理的研究与模拟内燃机是一种常见的动力装置,广泛应用于汽车、船舶、飞机等领域。
它的工作原理是将燃料与空气混合后在燃烧室中进行燃烧,产生高温高压的气体,驱动活塞做功。
内燃机的燃烧过程非常复杂,涉及到物理、化学、热力学等多个学科领域。
本文将围绕内燃机的燃烧机理展开讨论,并介绍目前燃烧模拟领域的主要研究内容。
一、内燃机燃烧机理内燃机的燃烧过程可以分为四个阶段:点火延迟期、快速燃烧期、减速燃烧期和尾部燃烧期。
其中,点火延迟期是指燃料和空气混合后到燃烧反应开始的时间。
此时混合气体中的反应物质和反应物浓度决定了点火延迟的程度,其主要受到混合气体温度和压力的影响。
快速燃烧期是指反应速率迅速增加,混合气体的温度和压力急剧上升,产生大量热能。
减速燃烧期是指反应速率逐渐减缓,混合气体的温度和压力开始下降。
尾部燃烧期是指燃烧过程即将结束时,产生大量高温气体和未完全燃烧的物质。
内燃机燃烧机理的研究既可以从理论模型上探讨,也可以通过实验手段来验证。
理论模型通常采用反应动力学和燃烧热力学等原理,建立数学模型,模拟燃烧过程的动态变化。
实验手段通常包括气体采样和分析技术、高速摄影技术、激光诊断技术、计算流体力学(CFD)模拟等方法。
二、内燃机燃烧模拟内燃机燃烧模拟是一种基于数学模型的燃烧过程仿真技术。
它可以帮助工程师预测内燃机的性能,优化设计参数,减少实验测试成本,提高燃烧效率和环境友好性。
目前,燃烧模拟主要关注以下几个方面:1. 燃烧过程数学模型的建立。
该模型通常基于喷雾流动、化学反应与热传递等多个过程的耦合,并采用CFD模拟技术进行求解。
数值计算能够快速获得各种燃烧工况下的温度、压力、氧化物排放等参数,并比较不同设计参数的影响。
2. 燃料喷雾和混合过程的模拟。
在内燃机中,喷雾和混合是决定燃烧效率和排放质量的关键过程。
燃烧模拟通常借助CFD模拟技术对喷雾的液滴分布、直径、速度等进行模拟,并对喷雾与气体的互相作用进行分析。
内燃机工作原理
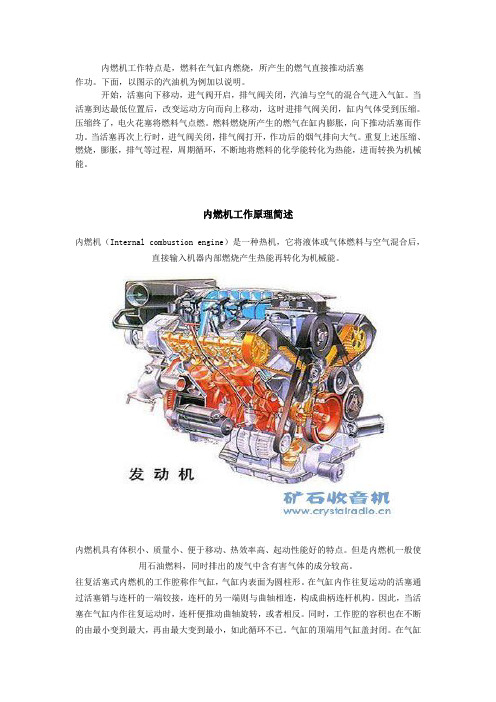
内燃机工作特点是,燃料在气缸内燃烧,所产生的燃气直接推动活塞作功。
下面,以图示的汽油机为例加以说明。
开始,活塞向下移动,进气阀开启,排气阀关闭,汽油与空气的混合气进入气缸。
当活塞到达最低位置后,改变运动方向而向上移动,这时进排气阀关闭,缸内气体受到压缩。
压缩终了,电火花塞将燃料气点燃。
燃料燃烧所产生的燃气在缸内膨胀,向下推动活塞而作功。
当活塞再次上行时,进气阀关闭,排气阀打开,作功后的烟气排向大气。
重复上述压缩、燃烧,膨胀,排气等过程,周期循环,不断地将燃料的化学能转化为热能,进而转换为机械能。
内燃机工作原理简述内燃机(Internal combustion engine)是一种热机,它将液体或气体燃料与空气混合后,直接输入机器内部燃烧产生热能再转化为机械能。
内燃机具有体积小、质量小、便于移动、热效率高、起动性能好的特点。
但是内燃机一般使用石油燃料,同时排出的废气中含有害气体的成分较高。
往复活塞式内燃机的工作腔称作气缸,气缸内表面为圆柱形。
在气缸内作往复运动的活塞通过活塞销与连杆的一端铰接,连杆的另一端则与曲轴相连,构成曲柄连杆机构。
因此,当活塞在气缸内作往复运动时,连杆便推动曲轴旋转,或者相反。
同时,工作腔的容积也在不断的由最小变到最大,再由最大变到最小,如此循环不已。
气缸的顶端用气缸盖封闭。
在气缸盖上装有进气门和排气门,进、排气门是头朝下尾朝上倒挂在气缸顶端的。
通过进、排气门的开闭实现向气缸内充气和向气缸外排气。
进、排气门的开闭由凸轮轴控制。
凸轮轴由曲轴通过齿形带或齿轮或链条驱动。
进、排气门和凸轮轴以及其他一些零件共同组成配气机构。
通常称这种结构形式的配气机构为顶置气门配气机构。
现代汽车内燃机无一例外地都采用顶置气门配气机构。
构成气缸的零件称作气缸体,支承曲轴的零件称作曲轴箱,气缸体与曲轴箱的连铸体称作机体。
甲,基本术语1. 工作循环活塞式内燃机的工作循环是由进气、压缩、作功和排气等四个工作过程组成的封闭过程。
内燃机应用的热力学原理

内燃机应用的热力学原理1. 引言内燃机是一种将燃料能转化为机械能的热机,广泛应用于汽车、飞机、船舶等交通工具中。
内燃机的工作原理基于热力学原理,通过燃烧燃料产生高温高压气体,并将其转化为机械能,从而驱动交通工具的运动。
2. 内燃机的基本原理内燃机包括燃烧室、气缸、活塞等关键部件。
其基本工作过程如下:•进气过程:活塞下行,进气门打开,气缸内充满混合气(燃料与空气的混合物)。
•压缩过程:活塞上行,进气门关闭,压缩混合气,使其达到高温高压状态。
•燃烧过程:在混合气达到高温高压状态时,喷入点火器点燃混合气,产生爆发力。
•排气过程:活塞下行,排气门打开,将燃烧产生的废气排出气缸。
3. 热力学循环内燃机的工作过程可以用热力学循环来描述。
常用的热力学循环包括奥托循环和迪塞尔循环。
3.1 奥托循环奥托循环是用于汽油发动机的热力学循环。
其基本过程如下:1.进气过程:活塞下行,进气门打开,充满混合气。
2.压缩过程:活塞上行,进气门关闭,压缩混合气。
3.燃烧过程:混合气点燃,产生爆发力,推动活塞下行。
4.排气过程:活塞下行,排气门打开,废气排出。
奥托循环有较高的热效率,适用于轻负荷长时间运行的情况。
3.2 迪塞尔循环迪塞尔循环是用于柴油发动机的热力学循环。
其基本过程如下:1.进气过程:活塞下行,进气门打开,充满空气。
2.压缩过程:活塞上行,进气门关闭,压缩空气。
3.燃烧过程:在压缩空气的同时喷入柴油,柴油自燃产生爆发力,推动活塞下行。
4.排气过程:活塞下行,排气门打开,废气排出。
迪塞尔循环具有较高的热效率和较大的爆发力,适用于高负荷短时间运行的情况。
4. 热力学参数在内燃机的分析中,热力学参数是十分重要的。
常用的热力学参数包括:•压缩比:气缸内气体压缩前后的比值,决定了发动机的效率。
•热效率:燃料能转化为机械能的比率,是内燃机的重要性能指标。
•排气温度:废气排出时的温度,反映了内燃机的工作状态。
•燃烧室温度:燃烧室内混合气点燃时的最高温度,对发动机的寿命和性能有影响。
内燃机工程中的点火与燃烧机理
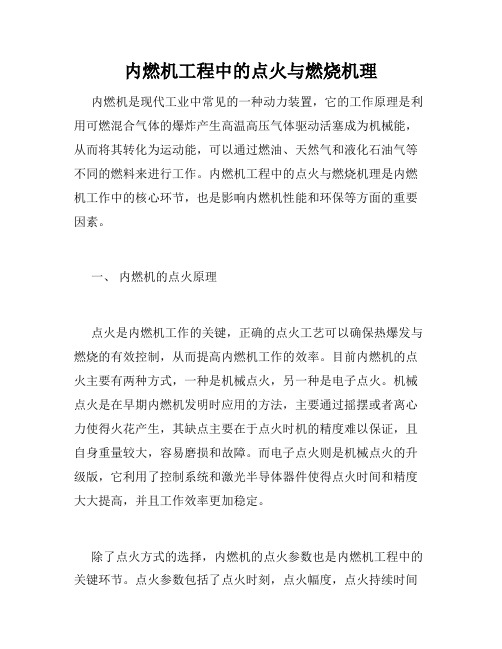
内燃机工程中的点火与燃烧机理内燃机是现代工业中常见的一种动力装置,它的工作原理是利用可燃混合气体的爆炸产生高温高压气体驱动活塞成为机械能,从而将其转化为运动能,可以通过燃油、天然气和液化石油气等不同的燃料来进行工作。
内燃机工程中的点火与燃烧机理是内燃机工作中的核心环节,也是影响内燃机性能和环保等方面的重要因素。
一、内燃机的点火原理点火是内燃机工作的关键,正确的点火工艺可以确保热爆发与燃烧的有效控制,从而提高内燃机工作的效率。
目前内燃机的点火主要有两种方式,一种是机械点火,另一种是电子点火。
机械点火是在早期内燃机发明时应用的方法,主要通过摇摆或者离心力使得火花产生,其缺点主要在于点火时机的精度难以保证,且自身重量较大,容易磨损和故障。
而电子点火则是机械点火的升级版,它利用了控制系统和激光半导体器件使得点火时间和精度大大提高,并且工作效率更加稳定。
除了点火方式的选择,内燃机的点火参数也是内燃机工程中的关键环节。
点火参数包括了点火时刻,点火幅度,点火持续时间以及点火间隔等。
其中,点火时刻是最关键的一项参数,它要求点火时刻不仅要满足燃烧的需要,同时还要保证燃油不过早燃烧,也不要过晚燃烧。
点火还需要考虑柴油机和汽油机的不同特点。
柴油机就需要保证高温高压状态下的自燃能力,而汽油机则常常采用提高燃油与空气混合比的方法。
二、内燃机的燃烧机理燃烧机理是内燃机能否正常工作的关键因素之一。
在燃烧过程中,燃气需要被均匀的分配到每个发动机缸中,产生的高温高压状态需要与气缸内壁均匀接触,从而产生高压力。
内燃机的燃烧机理主要包括燃油喷射、点火、混合气燃烧以及排气的过程。
燃油喷射是将燃料均匀的喷射到空气中的过程,这里涉及到喷油嘴的设计和燃料的盛装,喷油嘴的设计需要满足燃料均匀喷射,且需要同时满足燃料性能及引擎特点。
而燃料的盛装则需要保证制定合理的油箱容积和油位相应的设计,如果油位过高或过低,可能会导致燃油无法正常喷射,从而影响整个内燃机的工作效果。
内燃机的结构与工作原理
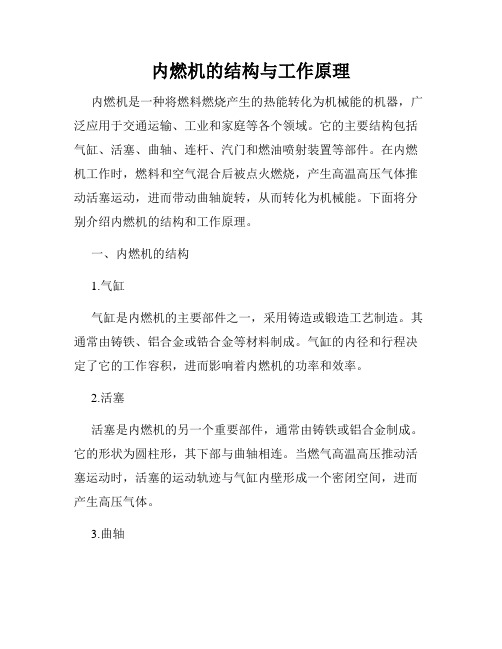
内燃机的结构与工作原理内燃机是一种将燃料燃烧产生的热能转化为机械能的机器,广泛应用于交通运输、工业和家庭等各个领域。
它的主要结构包括气缸、活塞、曲轴、连杆、汽门和燃油喷射装置等部件。
在内燃机工作时,燃料和空气混合后被点火燃烧,产生高温高压气体推动活塞运动,进而带动曲轴旋转,从而转化为机械能。
下面将分别介绍内燃机的结构和工作原理。
一、内燃机的结构1.气缸气缸是内燃机的主要部件之一,采用铸造或锻造工艺制造。
其通常由铸铁、铝合金或锆合金等材料制成。
气缸的内径和行程决定了它的工作容积,进而影响着内燃机的功率和效率。
2.活塞活塞是内燃机的另一个重要部件,通常由铸铁或铝合金制成。
它的形状为圆柱形,其下部与曲轴相连。
当燃气高温高压推动活塞运动时,活塞的运动轨迹与气缸内壁形成一个密闭空间,进而产生高压气体。
3.曲轴曲轴是内燃机的承重组件和传动组件,它将活塞的直线运动转化为曲轴的旋转运动。
曲轴通常由钢材制成,包括主轴和连杆。
主轴连接活塞和连杆,由多个主轴组成的推进旋转,进而转化为机械能。
4.连杆连杆连接活塞和曲轴,它通常由钢材制成,呈I字形或H字形。
连杆的长度和形状直接影响内燃机的工作特性和输出功率。
5.汽门汽门是控制燃气进出气缸的部件,通常由钢材制成。
它分为进气门和排气门,进气门控制燃料和空气混合物的进入,排气门控制燃气的排出。
汽门的开关由凸轮或凸轮轴控制。
6.燃油喷射装置燃油喷射装置是将燃料喷射进气缸的部件,通常由高压油泵和喷油嘴组成。
它可以更加准确地控制燃料的喷射时间和喷射量,提高内燃机的燃烧效率和功率输出。
二、内燃机的工作原理内燃机的工作原理是将燃料和空气混合后点火燃烧,产生高温高压气体推动活塞运动,转化为机械能。
内燃机的工作循环分为四个阶段:进气、压缩、燃烧和排气。
1.进气阶段在进气阶段,气缸内的活塞从上往下运动,与气缸内形成一个低压区。
此时,汽门打开,燃料和空气混合物通过汽门进入低压区,充满气缸。
2.压缩阶段在压缩阶段,气缸内的活塞向上运动,将燃料和空气混合物压缩成高压气体。
内燃机工作原理

内燃机工作原理
内燃机是一种动力系统,是由发动机构成的机械传动系统。
它将有机燃料(如汽油、
柴油等)、空气和排气气体结合起来,在发动机的内部完成能量转换。
这种能量转换能够
提供给各种内燃机类型的动力驱动和热能,从而促进机械作业。
理解内燃机工作原理可以帮助我们加深对内燃机的了解,并为内燃机的维护和保养服
务奠定基础。
一般来说,内燃机的工作原理分为四个主要阶段:压缩,燃烧,排气和喷油。
压缩阶段:压缩是内燃机能量转换过程中的第一步,在这一步中,内燃机上的活塞将
最终在缸内空气从低压吸入到高压。
此外,由于紧凑的气体会增加空气温度,因此当活塞
在缸中上下移动时,会产生更多的热量。
燃烧阶段:当空气被完全压缩后,即可开始燃烧。
通常,有机燃料(汽油、柴油等)
由喷油嘴喷射到缸中,形成一个强烈的火焰,从而使缸内的空气和燃料燃烧。
在此过程中,压缩的活塞会立即发挥作用,将热能释放到缸内气体中,从而使活塞和缸体进一步推动。
排气阶段:当有机燃料燃烧完毕后,它将排出组成排气气体的各种有毒物质,例如一
氧化碳、二氧化碳和氮氧化物,这些气体都产生了在缸中燃烧时不会改变其空气比热容。
喷油阶段:这一步的功能是将新的有机燃料(汽油、柴油等)送入缸内,以补充之前
已经燃烧的有机燃料。
在喷油嘴喷射的机器中,会主动控制有机燃料和空气量,以保证正
确的混合比例,并使缸内有机燃料火焰合理而有效地发动并迅速完成燃烧。
总体而言,内燃机的工作原理主要是指机械传动系统在发动机内部完成能量转换,并
将有机燃料混合、燃烧、释放热量以及排出排气气体,以提供动力和发动机的正常运行。
- 1、下载文档前请自行甄别文档内容的完整性,平台不提供额外的编辑、内容补充、找答案等附加服务。
- 2、"仅部分预览"的文档,不可在线预览部分如存在完整性等问题,可反馈申请退款(可完整预览的文档不适用该条件!)。
- 3、如文档侵犯您的权益,请联系客服反馈,我们会尽快为您处理(人工客服工作时间:9:00-18:30)。
Introduction to Combustion ChemistryThe gasoline-powered internal combustion engine takes air from the atmosphere and gasoline, a hydrocarbon fuel, and through the process of combustion releases the chemical energy stored in the fuel. Of the total energy released by the combustion process, about 20% is used to propel the vehicle, the remaining 80% is lost to friction, aerodynamic drag, accessory operation, or simply wasted as heat transferred to the cooling system.Modern gasoline engines are very efficient compared to predecessors of the late '60s and early '70s when emissions control and fuel economy were first becoming a major concern of automotive engineers. Generally speaking, the more efficient an engine becomes, the lower the exhaust emissions from the tailpipe. However, as clean as engines operate today, exhaust emission standards continually tighten. The technology to achieve these ever-tightening emissions targets has led to the advanced closed loop engine control systems used on today's Toyota vehicles. With these advances in technology comes the increased emphasis on maintenance, and when the engine and emission control systems fail to operate as designed, diagnosis and repair.Understanding the Combustion ProcessTo understand how to diagnose and repair the emissions control system, one must first have a working knowledge of the basic combustion chemistry which takes place within the engine. That is the purpose of this section of the program.The gasoline burned in an engine contains many chemicals, however, it is primarily made up of hydrocarbons (also referred to as HC. Hydrocarbons are chemical compounds made up of hydrogen atoms which chemically bond with carbon atoms. There are many different types of hydrocarbon compounds found in gasoline, depending on the number of hydrogen and carbon atoms present, and the way that these atoms are bonded.Inside an engine, the hydrocarbons in gasoline will not burn unless they are mixed with air. This is where the chemistry of combustion begins. Air is composed of approximately 21% oxygen (02), 78% nitrogen (N2), and minute amounts of other inert gasses.The hydrocarbons in fuel normally react only with the oxygen during the combustion process to form water vapor (H2O) and carbon dioxide (CO2), creating the desirable effect of heat and pressure within the cylinder. Unfortunately, under certain engine operating conditions, the nitrogen also reacts with the oxygen to form nitrogen oxides (NOx), a criteria air pollutant.The ratio of air to fuel plays an important role in the efficiency of the combustion process. The ideal air/fuel ratio for optimum emissions, fuel economy, and good engine performance is around 14.7 pounds of air for every one pound of fuel. This "ideal air/fuel ratio" is referred to as stoichiometry, and is the target that the feedback fuel control system constantly shoots for. At air/fuel ratios richer than stoichiometry, fuel economy and emissions will suffer. At air/fuel ratios leaner than stoichiometry, power, driveability and emissions will suffer.Under "Ideal" Combustion ConditionsIn a perfectly operating engine with ideal combustion conditions, the following chemical reaction would take place:•Hydrocarbons would react with oxygen to produce water vapor (H2O) and carbon dioxide (CO2)•Nitrogen (N2) would pass through the engine without being affected by the combustion process. In essence, only harmless elements would remain and enter the atmosphere. Although modern engines are producing much lower emission levels than their predecessors, they still inherently produce some level of harmful emission output.The Four-Stroke Combustion CycleDuring the Intake Stroke, air and fuel moves into the low pressure area created by the piston moving down inside the cylinder. The fuel injection system has calculated and delivered the precise amount of fuel to the cylinder to achieve a 14.7 to 1 ratio with the air entering the cylinder.As the piston moves upward during the Compression Strok e, a rapid pressure increase occurs inside the cylinder, causing the air/fuel mixture to superheat. During this time, the antiknock property or octane rating of the fuel is critical in preventing the fuel from igniting spontaneously (exploding). This precise superheated mixture is now prime for ignition as thepiston approaches Top Dead Center.Just before the piston reaches top dead center to start the Power Stroke, the spark plug ignites the air/fuel mixture in the combustion chamber, causing a flame-front to begin to spread through the mixture. During combustion, hydrocarbons and oxygen react, creating heat and pressure. Ideally, the maximum pressure is created as the piston is about 8 to 12 degrees past top dead center to produce the most force on the top of the piston and transmit the most power through the crankshaft. Combustion by-products will consist primarily of water vapor and carbon dioxide if the mixture and spark timing are precise.After the mixture has burned and the piston reaches bottom dead center, the Exhaust Stroke begins as the exhaust valve opens and the piston begins its return to top dead center. The water vapor, carbon dioxide, nitrogen, and a certain amount of unwanted pollutants are pushed out of the cylinder into the exhaust system.Harmful Exhaust EmissionsAs previously mentioned, even the most modern, technologically advanced automobile engines are not "perfect"; they still inherently produce some level of harmful emission output. There are several conditions in the combustion chamber which prevent perfect combustion and cause unwanted chemical reactions to occur. The following are examples of harmful exhaust emissions and their causes.Hydrocarbon (HC) EmissionHydrocarbons are, quite simply, raw unburned fuel. When combustion does not take place at all, as with a misfire, large amounts of hydrocarbons are emitted from the combustion chamber.A small amount of hydrocarbon is created by a gasoline engine due to its design. A normal process called wall quenching occurs as the combustion flame front burns to the relatively cool walls of the combustion chamber. This cooling extinguishes the flame before all of the fuel is fully burned, leaving a small amount of hydrocarbon to be pushed out the exhaust valve.Another cause of excessive hydrocarbon emissions is related to combustion chamber deposits. Because these carbon deposits are porous, hydrocarbon is forced into these pores as the air/fuel mixture is compressed. When combustion takes place, this fuel does not burn, however, as the piston begins its exhaust stroke, these hydrocarbons are released into the exhaust stream.The most common cause of excessive hydrocarbon emissions is misfire which occurs due to ignition, fuel delivery, or air induction problems. Depending on how severe the misfire, inadequate spark or a noncombustible mixture (either too rich or too lean) will cause hydrocarbons to increase to varying degrees. For example, a total misfire due to a shorted spark plug wire will cause hydrocarbons to increase dramatically. Conversely, a slight lean misfire due to a false air entering the engine, may cause hydrocarbons to increase only slightly. Excess hydrocarbon can also be influenced by the temperature of the air/ fuel mixture as it enters the combustion chamber. Excessively low intake air temperatures can cause poor mixing of fuel and air, resulting in partial misfire.Carbon Monoxide (CO) EmissionCarbon monoxide (CO) is a byproduct of incomplete combustion and is essentially partially burned fuel. If the air/fuel mixture does not have enough oxygen present during combustion, it will not bum completely. When combustion takes place in an oxygen starved environment, there is insufficient oxygen present to fully oxidize the carbon atoms into carbon dioxide (CO2). When carbon atoms bond with only one oxygen atom carbon monoxide (CO) forms.An oxygen starved combustion environment occurs as a result of air/fuel ratios which are richer than stoichiometry (14.7 to 1). There are several engine operating conditions when this occurs normally. For example, during cold operation, warm-up, and power enrichment. It is, therefore, normal for higher concentrations of carbon monoxide to be produced under these operating conditions. Causes of excessive carbon monoxide includes leaky injectors, high fuel pressure, improper closed loop control, etc.When the engine is at warm idle or cruise, very little carbon monoxide is produced because there is sufficient oxygen available during combustion to fully oxidize the carbon atoms. This results in higher levels of carbon dioxide (CO2) the principal by-product of efficient combustion. Oxides of Nitrogen (NOx) EmissionHigh cylinder temperature and pressure which occur during the combustion process can cause nitrogen to react with oxygen to form Oxides of Nitrogen (NOx). Although there are various forms of nitrogen-based emissions that comprise Oxides of Nitrogen (NOx), nitric oxide (NO) makes up the majority, about 98% of all NOx emissions produced by the engine.Generally speaking, the largest amount of NOx is produced during moderate to heavy load conditions when combustion pressures and temperatures are their highest. However, small amounts of NOx can also be produced during cruise and light load, light throttle operation. Common causes of excessive NOx include faulty EGR system operation, lean air/fuel mixture, high temperature intake air, overheated engine, excessive spark advance, etc.Air/Fuel Mixture Impact on Exhaust EmissionsAs you can see in the graph above, HC and CO levels are relatively low near the theoretically ideal 14.7 to 1 air/fuel ratio. This reinforces the need to maintain strict air/fuel mixture control. However, NOx production is very high just slightly leaner than this ideal mixture range. This inverse relationship between HC/CO production and NOx production poses a problem when controlling total emission output. Because of this relationship, you can understand thecomplexity in reducing all three emissions at the same time.。