轮机专业毕业论文英文翻译
发动机类毕业设计外文翻译
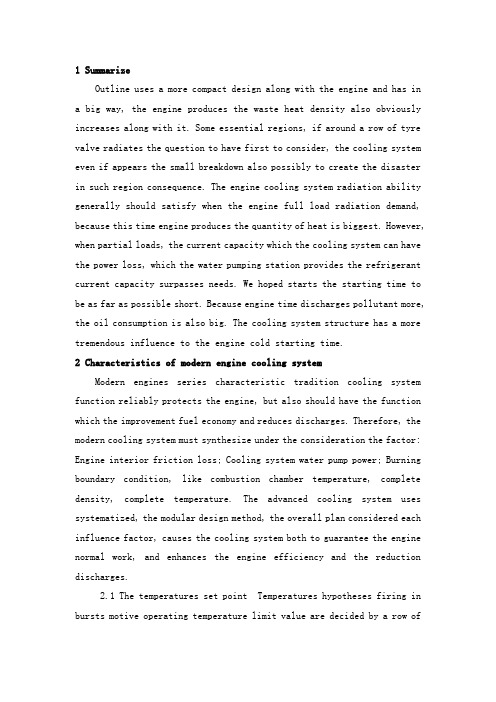
1 SummarizeOutline uses a more compact design along with the engine and has in a big way, the engine produces the waste heat density also obviously increases along with it. Some essential regions, if around a row of tyre valve radiates the question to have first to consider, the cooling system even if appears the small breakdown also possibly to create the disaster in such region consequence. The engine cooling system radiation ability generally should satisfy when the engine full load radiation demand, because this time engine produces the quantity of heat is biggest. However, when partial loads, the current capacity which the cooling system can have the power loss, which the water pumping station provides the refrigerant current capacity surpasses needs. We hoped starts the starting time to be as far as possible short. Because engine time discharges pollutant more, the oil consumption is also big. The cooling system structure has a more tremendous influence to the engine cold starting time.2 Characteristics of modern engine cooling systemModern engines series characteristic tradition cooling system function reliably protects the engine, but also should have the function which the improvement fuel economy and reduces discharges. Therefore, the modern cooling system must synthesize under the consideration the factor: Engine interior friction loss; Cooling system water pump power; Burning boundary condition, like combustion chamber temperature, complete density, complete temperature. The advanced cooling system uses systematized, the modular design method, the overall plan considered each influence factor, causes the cooling system both to guarantee the engine normal work, and enhances the engine efficiency and the reduction discharges.2.1 The temperatures set point Temperatures hypotheses firing in bursts motive operating temperature limit value are decided by a row oftire valve the peripheral region maximum temperature. The most ideal situation is according to the metal temperature but is not the refrigerant temperature control cooling system, like this can protect the engine well. Because the cooling system hypothesis cooling temperature is by the full load time most is big is the foundation, therefore, engine and cooling system in partial loads time is at not too the perfect condition, when urban district travel and low speed travel, can have the high oil consumption and discharge. Supposes the fixed point through the change refrigerant temperature to be possible to improve the engine and the cooling system in partial loads time performance. According to a row of tyre valve the peripheral region temperature limit value, may elevate either reduce the refrigerant or the metal temperature supposes the fixed point. Elevates or reduced temperature all respectively has the characteristic, this is decided the goal which achieved to the hope.2.2 Enhances the temperature Enhances the temperature to suppose the fixed-point enhancement operating temperature to suppose the fixed point is one kind of comparison the method which welcome. Enhances the temperature to have many merits, it directly affects the engine loss and the cooling system effect as well as the engine discharging formation. Will enhance the operating temperature to enhance the engine Mac reduce the engine to rub wears, reduces the engine fuel oil consumption. The research indicated that, the engine operating temperature to rubs the loss to have the very tremendous influence. Discharges the temperature the refrigerant to enhance to 150 ℃, causes the cylinder temperature to elevate to 195 ℃, the oil consumption drops 4%-6%. Maintains the refrigerant temperature in 90-115 ℃ scope, causes the engine machine oil the maximum temperature is 140 ℃, then oil consumption in partial loads time drops 10%. Enhances the operating temperature also obvious influence cooling system the potency. Enhances the refrigerant or the metaltemperature can improve the engine and disperse the steam heat transfer transmission the effect, reduces the refrigerant the speed of flow, reduces the water pump the rated power, thus reduces the engine the power dissipation. In addition, may select the different method, further reduces the refrigerant the speed of flow.2.3 Reduce the temperatures set point Reduced temperatures suppose the fixed point to reduce the cooling system the operating temperature to be possible to enhance the engine charge efficiency, reduces the inlet temperature. This to the combustion process, the fuel oil efficiency and discharges advantageously. The reduced temperature supposes the fixed point to be allowed to save the engine movement cost, enhances the part service life. The research indicated, if the cylinder head temperature reduces to 50 ℃, the ignition angle of advance may 3 ℃ A but not have the engine knock ahead of time, the charge efficiency enhances 2%, the engine operational factor improvement, is helpful to the optimized compression ratio and the parameter choice, obtains the better fuel oil efficiency and discharges the performance.2.4 Precise cooling system Precise cooling systems precise cooling system mainly manifests in the cooling jacket structural design and in the refrigerant speed of flow design. In precise cooling system, hot essential area, if around a row of tyre valve, the refrigerant has an greater speed of flow, the heat transfer efficiency is high, the refrigerant gradient of temperature changes slightly. Such effect comes from to reduce these place refrigerant channels the lateral section, enhances the speed of flow, reduces the current capacity. The precise cooling system design key lies in the determination cooling jacket the size, the choice match cooling water pump, guaranteed the system the radiation ability can satisfy when the low speed big load essential region operating temperature demand. The engine refrigerant speed of flow rangeof variation is quite big, from time 1 m/s to maximum work rate time 5 m/s. Therefore should considered the cooling jacket and the cooling system whole that, mutually supplemented, display biggest potential. The research indicated that, uses the precise cooling system, in the engine entire work rotational speed scope, the refrigerant current capacity may drop 40%. Covers the cooling jacket to the air cylinder the precise design, may make the ordinary speed of flow to enhance from 1.4m/s to 4 m/s, greatly enhances the cylinder cover or cap thermal conductivity, cylinder cover or cap metal temperature drop to 60 ℃.2.5 Divergences types cooling system Divergences types cooling system divergence type cooling system for other one kind of cooling system. In this kind of cooling system, the hine oil temperature, will cylinder cover or cap friendly cylinder body cools by respective return route, the cylinder cover or cap friendly cylinder body has the different temperature. The divergence -like cooling system has the unique superiority, may cause engine each part to suppose the fixed-point work at the most superior temperature. The cooling system overall efficiency achieves in a big way. Each cooling return route will suppose under the fixed point or the speed of flow in the different cooling temperature works, will create the ideal engine temperature distribution. The ideal engine hot active status is the cylinder head temperature lower but the air cylinder body temperature relative is higher. The cylinder head temperature is lower may enhance the charge efficiency, increases. The temperature is low also greatly may promote completely to burn, reduces CO, HC and the NOx formation, also enhances the output. The higher air cylinder body temperature can reduce the friction to lose, directly improves the fuel oil efficiency, indirectly reduces in the cylinder the peak value pressure and the temperature. The divergence type cooling system may cause the cylinder cover and the cylinder body temperaturediffers 100 ℃. The cylinder temperature may reach as high as 150 ℃, but the cylinder cover temperature may reduce 50 ℃, reduces the cylinder body to rub loses, reduces the oil consumption. The higher cylinder body temperature causes the oil consumption to reduce 4%-6%, when partial loads HC reduces 20%-35%. When the damper all opens, the cylinder cover and the cylinder body temperature supposes the definite value to be possible to move to 50 ℃ and 90 ℃, improves the fuel oil consumption, the power output from the whole and discharges.2.6 Controllable cooling system Controllable engine cooling system tradition engine cooling system belongs to the passive form, the structure simple or the cost is low. The controllable cooling system may make up at present cooling system the insufficiency. Now the cooling system design standard solves time the full load radiation problem, thus partially shoulders time the oversized radiation ability will cause the engine power waste. This to the light vehicle said especially obvious, these vehicles majority time all under the partial loads go in the urban district, only uses the partial engine power, causes a cooling system higher loss. In order to solve the engine to get down the hot question in the peculiar circumstance, the present cooling system volume was bigger, causes the evaporation efficiency to reduce, has increased the cooling system power demand, lengthened the engine during warm machine-hour. The controllable engine cooling system generally includes the sensor, the execution and the electrically controlled module. The controllable cooling system can act according to the engine working condition adjustment cooling quantity, reduces the engine power loss. In the controllable cooling system, the execution for the cooling water pump and the thermostat, generally and the control valve is composed by the electrically operated water pump, may act according to requests to adjust the cooling quantity. Temperature sensor for a system part, but rapidly bequeaths the engine hot conditionthe controller. Controllable installment, if the electrically operated water pump, may suppose the temperature the fixed point from 90 ℃ to enhance to 110 ℃, saves 2%-5% fuel oil, CO reduces 20%, HC reduces 10%. When steady state, the metal temperature ratio tradition cooling system is high 10 ℃, the controllable cooling system has the quicker response ability, may cool the temperature to maintain is supposing the fixed point ±2 ℃ the scope. From 110 ℃ drops to 100 ℃ only needs 2 s. The engine during warm machine-hour reduces 200s, the cooling system operating region draws close to the work limit region, can reduce the engine cooling temperature and the metal temperature undulation scope, reduces circulates the fatigue of metal which the hot load creates, lengthens the component life.3 ConclusionIn front of 3 conclusions introduced several kind of advanced cooling systems have the improvement cooling system performance the potential, can enhance the fuel oil efficiency and discharge the performance. The cooling system can control the nature is improves the cooling system the key, can the controlling expression to the engine structure protection essential parameter, like the metal temperature, the refrigerant temperature and the machine oil temperature and so on can control, guarantees the engine to work in the safety margin scope. The cooling system can make the rapid reaction to the different operating mode, the most earth saves the fuel, reduces discharges, but does not affect the engine overall performance. Looked from the design and the operational performance angle that, divergence type cooling and precise cooling unifies has the very good prospects for development, both can provide the ideal engine protection, and can enhance the fuel oil efficiency and discharge the nature. This kind of structure is advantageous to forming the engine ideal temperature distribution. Directly to a cylinder coveror cap row of tyre valve around the supplies refrigerant, reduced the cylinder head temperature change, causes the cylinder cover temperature distribution to be evener, also can maintains the machine oil and the cylinder body temperature at the design operating region, has a lower friction to damage the pollution withdrawal. method as follows: 1st, the cooling system function, is part of quantity of heats which absorbs the engine part carries off, guaranteed the diesel engine various components maintain in the normal temperature range. cooling system function and maintenance maintenance2nd, the cooling water should be does not contain dissolves the Xie salt the soft water, like clean river water, rain water and so on. Do not use hard water and so on the well water, water seepage or sea water, guards against produces, causes the engine to radiate not good, question occurrence and so on air cylinder heat.3rd, with the funnel when joins the cooling water the water tank, must prevent the water splashes to on the engine and the radiator, prevented on the radiator fin and the organism accumulates the dust, smears, affects the cooling effect.4th, if when the engine lacks the water causes the hyperpyrexia, cannot immediately add water, should cause the engine idling speed to revolve 10 □15 minutes, after the uniform temperature slightly reduces, slowly does not join the cooling water in the engine situation.5th, the winter, the water tank planted agent adds the hot water. After the start should surpass 40 degree-hour the slow revolution to the water temperature to be able to work. After the work had ended, must put the completely cooling water.6th, must regularly eliminate in the water tank, must frequently scour the sludge to the forced-air cooling engine radiator fin, dirty is filthy. The radiator fin cannot damage, after if damages must promptlyreplace, in order to avoid influence radiation effect.4 LathesLathes are machine tools designed primarily to do turning, facing and boring, Very little turning is done on other types of machine tools, and none can do it with equal facility. Because lathes also can do drilling and reaming, their versatility permits several operations to be done with a single setup of the work piece. Consequently, more lathes of various types are used in manufacturing than any other machine tool.The essential components of a lathe are the bed, headstock assembly, tailstock assembly, and the leads crew and feed rod.The bed is the backbone of a lathe. It usually is made of well normalized or aged gray or nodular cast iron and provides s heavy, rigid frame on which all the other basic components are mounted. Two sets of parallel, longitudinal ways, inner and outer, are contained on the bed, usually on the upper side. Some makers use an inverted V-shape for all four ways, whereas others utilize one inverted V and one flat way in one or both sets, They are precision-machined to assure accuracy of alignment. On most modern lathes the way are surface-hardened to resist wear and abrasion, but precaution should be taken in operating a lathe to assure that the ways are not damaged. Any inaccuracy in them usually means that the accuracy of the entire lathe is destroyed.The headstock is mounted in a foxed position on the inner ways, usually at the left end of the bed. It provides a powered means of rotating the word at various speeds . Essentially, it consists of a hollow spindle, mounted in accurate bearings, and a set of transmission gears-similar to a truck transmission—through which the spindle can be rotated at a number of speeds. Most lathes provide from 8 to 18 speeds, usually in a geometric ratio, and on modern lathes all the speeds can be obtained merely by moving from two to four levers. An increasing trend is to provide a continuouslyvariable speed range through electrical or mechanical drives.Because the accuracy of a lathe is greatly dependent on the spindle, it is of heavy construction and mounted in heavy bearings, usually preloaded tapered roller or ball types. The spindle has a hole extending through its length, through which long bar stock can be fed. The size of maximum size of bar stock that can be machined when the material must be fed through spindle.The tailsticd assembly consists, essentially, of three parts. A lower casting fits on the inner ways of the bed and can slide longitudinally thereon, with a means for clamping the entire assembly in any desired location, An upper casting fits on the lower one and can be moved transversely upon it, on some type of keyed ways, to permit aligning the assembly is the tailstock quill. This is a hollow steel cylinder, usually about 51 to 76mm(2to 3 inches) in diameter, that can be moved several inches longitudinally in and out of the upper casting by means of a hand wheel and screw.The size of a lathe is designated by two dimensions. The first is known as the swing. This is the maximum diameter of work that can be rotated on a lathe. It is approximately twice the distance between the line connecting the lathe centers and the nearest point on the ways, The second size dimension is the maximum distance between centers. The swing thus indicates the maximum work piece diameter that can be turned in the lathe, while the distance between centers indicates the maximum length of work piece that can be mounted between centers.Engine lathes are the type most frequently used in manufacturing. They are heavy-duty machine tools with all the components described previously and have power drive for all tool movements except on the compound rest. They commonly range in size from 305 to 610 mm(12 to 24 inches)swing and from 610 to 1219 mm(24 to 48 inches) center distances,but swings up to 1270 mm(50 inches) and center distances up to 3658mm(12 feet) are not uncommon. Most have chip pans and a built-in coolant circulating system. Smaller engine lathes-with swings usually not over 330 mm (13 inches ) –also are available in bench type, designed for the bed to be mounted on a bench on a bench or cabinet.Although engine lathes are versatile and very useful, because of the time required for changing and setting tools and for making measurements on the work piece, thy are not suitable for quantity production. Often the actual chip-production tine is less than 30% of the total cycle time. In addition, a skilled machinist is required for all the operations, and such persons are costly and often in short supply. However, much of the operator’s time is consumed by simple, repetitious adjustments and in watching chips being made. Consequently, to reduce or eliminate the amount of skilled labor that is required, turret lathes, screw machines, and other types of semiautomatic and automatic lathes have been highly developed and are widely used in manufacturing.5 Limits and TolerancesMachine parts are manufactured so they are interchangeable. In other words, each part of a machine or mechanism is made to a certain size and shape so will fit into any other machine or mechanism of the same type. To make the part interchangeable, each individual part must be made to a size that will fit the mating part in the correct way. It is not only impossible, but also impractical to make many parts to an exact size. This is because machines are not perfect, and the tools become worn. A slight variation from the exact size is always allowed. The amount of this variation depends on the kind of part being manufactured. For examples part might be made 6 in. long with a variation allowed of 0.003 (three-thousandths) in. above and below this size. Therefore, the part could be 5.997 to 6.003 in. and still be the correct size. These are knownas the limits. The difference between upper and lower limits is called the tolerance.1 概述随着发动机采用更加紧凑的设计和具有更大的比功率,发动机产生的废热密度也随之明显增大。
轮机英语补充材料翻译
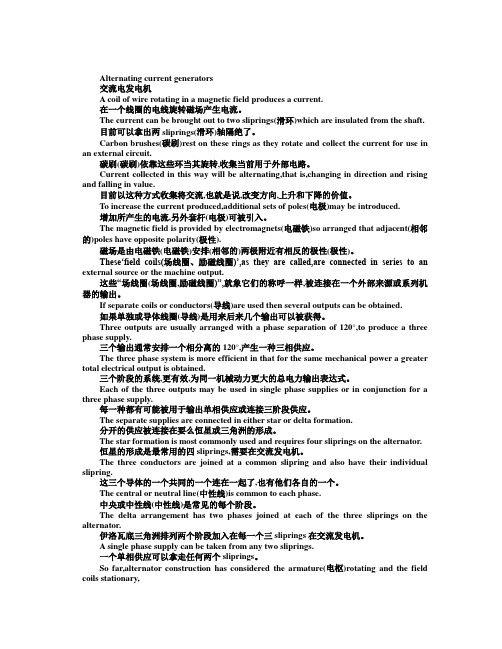
Alternating current generators交流电发电机A coil of wire rotating in a magnetic field produces a current.在一个线圈的电线旋转磁场产生电流。
The current can be brought out to two sliprings(滑环)which are insulated from the shaft.目前可以拿出两sliprings(滑环)轴隔绝了。
Carbon brushes(碳刷)rest on these rings as they rotate and collect the current for use in an external circuit.碳刷(碳刷)依靠这些环当其旋转,收集当前用于外部电路。
Current collected in this way will be alternating,that is,changing in direction and rising and falling in value.目前以这种方式收集将交流,也就是说,改变方向,上升和下降的价值。
T o increase the current produced,additional sets of poles(电极)may be introduced.增加所产生的电流,另外套杆(电极)可被引入。
The magnetic field is provided by electromagnets(电磁铁)so arranged that adjacent(相邻的)poles have opposite polarity(极性).磁场是由电磁铁(电磁铁)安排(相邻的)两极附近有相反的极性(极性)。
These…field coils(场线圈、励磁线圈)‟,as they are called,are connected in series to an external source or the machine output.这些“场线圈(场线圈,励磁线圈)”,就象它们的称呼一样,被连接在一个外部来源或系列机器的输出。
轮机英语作文模板
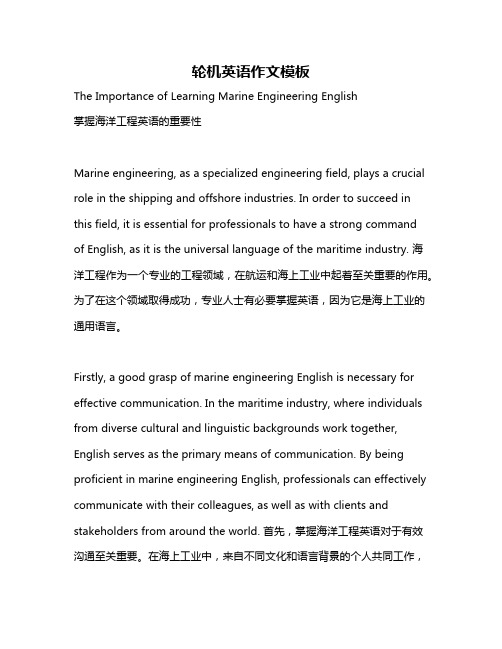
轮机英语作文模板The Importance of Learning Marine Engineering English掌握海洋工程英语的重要性Marine engineering, as a specialized engineering field, plays a crucial role in the shipping and offshore industries. In order to succeed in this field, it is essential for professionals to have a strong commandof English, as it is the universal language of the maritime industry. 海洋工程作为一个专业的工程领域,在航运和海上工业中起着至关重要的作用。
为了在这个领域取得成功,专业人士有必要掌握英语,因为它是海上工业的通用语言。
Firstly, a good grasp of marine engineering English is necessary for effective communication. In the maritime industry, where individuals from diverse cultural and linguistic backgrounds work together, English serves as the primary means of communication. By being proficient in marine engineering English, professionals can effectively communicate with their colleagues, as well as with clients and stakeholders from around the world. 首先,掌握海洋工程英语对于有效沟通至关重要。
轮机工程专业英语资料

轮机工程专业英语资料Shipboard Engineering ResourcesThe field of shipboard engineering encompasses a vast array of specialized knowledge and skills required to operate and maintain the complex systems found on modern vessels. From the propulsion machinery that drives the ship through the water to the intricate electrical and control systems that power onboard equipment, the role of the shipboard engineer is essential to the safe and efficient operation of any seagoing vessel.At the heart of this critical function are the technical resources and reference materials that enable engineers to stay informed and up-to-date on the latest advancements in marine engineering. These resources come in many forms, from technical manuals and repair guides to online databases and professional journals, all of which play a vital role in equipping shipboard engineers with the knowledge and tools they need to perform their duties.One of the most fundamental resources for the shipboard engineeris the equipment manufacturer's technical manuals. These comprehensive guides provide detailed information on the operation, maintenance, and repair of specific machinery and systems found on the vessel. From the main propulsion engines to the auxiliary generators, these manuals outline the recommended procedures for everything from routine inspections to major overhauls, ensuring that engineers have the necessary information to keep the ship's vital systems running smoothly.In addition to the manufacturer's manuals, shipboard engineers also rely heavily on a variety of reference materials and technical publications. These include industry-specific journals, such as the Journal of Marine Engineering & Technology, which feature peer-reviewed articles on the latest research and developments in the field of marine engineering. By staying informed on these cutting-edge advancements, engineers can better anticipate and address the challenges they may face in the course of their work.Another invaluable resource for shipboard engineers is the growing body of online databases and information repositories. These digital platforms provide instant access to a wealth of technical data, repair guides, and troubleshooting information, allowing engineers to quickly find the answers they need, even in the midst of an emergency situation. Some of the most widely used online resources in the maritime industry include the International Association ofClassification Societies (IACS) Recommendations and the International Maritime Organization (IMO) regulations, both of which provide essential guidance on the design, construction, and operation of marine vessels.Beyond these technical resources, shipboard engineers also rely on a range of hands-on training and professional development opportunities to hone their skills and stay current with industry best practices. This can include participation in specialized training programs, such as those offered by marine engineering schools and professional associations, as well as on-the-job mentorship and shadowing opportunities with experienced engineers.One such example is the Chief Engineer Training program, which provides comprehensive instruction on the management and leadership responsibilities of the senior-most engineer on board a vessel. By developing strong technical and interpersonal skills, participants in this program are better equipped to effectively oversee the operation and maintenance of the ship's engineering systems, as well as to mentor and guide the junior members of the engineering team.In addition to formal training programs, shipboard engineers also benefit from the wealth of knowledge and expertise that can be found within their own professional networks. Through participationin industry associations, such as the Institute of Marine Engineering, Science, and Technology (IMarEST) or the Society of Naval Architects and Marine Engineers (SNAME), engineers can connect with their peers, share best practices, and stay informed on the latest trends and developments in the field.These professional networks not only provide valuable opportunities for continued learning and professional development but also serve as a critical support system for shipboard engineers, who often work in remote and isolated environments. By tapping into these networks, engineers can access a wealth of institutional knowledge and practical guidance, helping them to navigate the unique challenges they may face in the course of their work.Ultimately, the success of any shipboard engineering operation is dependent on the availability and effective utilization of a comprehensive suite of technical resources and professional development opportunities. From the manufacturer's manuals to the online databases and industry publications, these tools and resources are essential to equipping shipboard engineers with the knowledge and skills they need to ensure the safe and efficient operation of the vessel.By staying informed, engaged, and committed to continuous learning, shipboard engineers can not only fulfill their critical role onboard but also contribute to the ongoing advancement of the maritime industry as a whole. Whether through the application of the latest technological innovations or the sharing of best practices and lessons learned, the shipboard engineer plays a vital role in shaping the future of marine engineering and ensuring the continued success of the vessels they serve.。
轮机英语介绍船员作文

轮机英语介绍船员作文Title: Introduction to Marine Engineering in English。
Marine engineering is a crucial aspect of seafaring, encompassing the design, construction, operation, and maintenance of a ship's propulsion and power generation systems. It is a field that requires both theoretical knowledge and practical skills to ensure the efficient and safe operation of vessels at sea. In this article, we will delve into the responsibilities and roles of marine engineers, as well as the significance of theircontribution to the maritime industry.First and foremost, marine engineers play a pivotalrole in ensuring the proper functioning of a ship's propulsion system. This involves the operation and maintenance of engines, turbines, propellers, and auxiliary machinery such as pumps, compressors, and electrical generators. Marine engineers are trained to troubleshoot and rectify any mechanical or electrical issues that mayarise during the voyage, thereby ensuring the uninterrupted operation of the vessel.Moreover, marine engineers are responsible for overseeing the safety and environmental sustainability of the ship's operations. They must adhere to strict regulatory standards and guidelines to minimize the risk of accidents and pollution at sea. This includes ensuring compliance with international conventions such as the International Maritime Organization (IMO) regulations on pollution prevention and safety of life at sea.In addition to their technical responsibilities, marine engineers also play a crucial role in managing the ship's energy consumption and efficiency. They are constantly striving to optimize fuel efficiency and reduce emissions through the implementation of advanced propulsion technologies and operational practices. This not only benefits the environment but also contributes to cost savings for shipowners and operators in the long run.Furthermore, marine engineers work closely with othermembers of the ship's crew, including deck officers, ratings, and shore-based personnel, to ensure seamless coordination and communication during operations. They participate in safety drills and emergency response exercises to prepare for any unforeseen events that may occur at sea. Their expertise and quick thinking are invaluable in mitigating risks and ensuring the safety of everyone on board.In conclusion, marine engineering is a dynamic and multidisciplinary field that plays a vital role in the maritime industry. From ensuring the smooth operation of propulsion systems to maintaining safety standards and optimizing energy efficiency, marine engineers are at the forefront of keeping ships afloat and navigating theworld's oceans. Their dedication and expertise are essential for the sustainable development of the shipping industry and the protection of the marine environment.Aspiring marine engineers should be prepared for a challenging yet rewarding career that offers opportunities for continuous learning and professional growth. With therapid advancement of technology and the growing emphasis on environmental sustainability, the role of marine engineers will only become more critical in the years to come.。
轮机英文文献
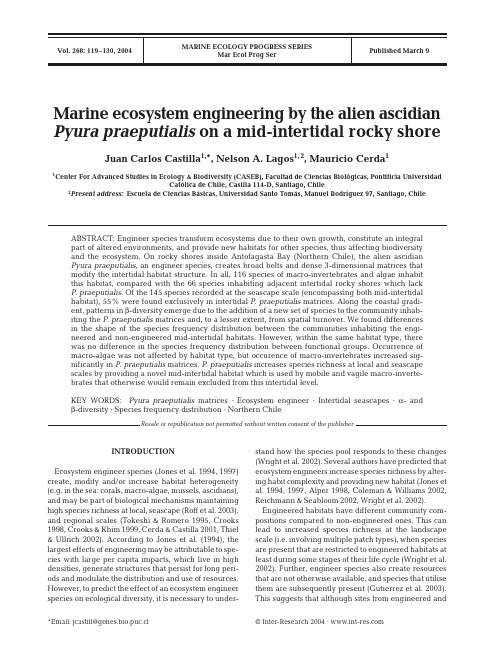
MARINE ECOLOGY PROGRESS SERIESMar Ecol Prog SerVol. 268: 119–130, 2004Published March 9INTRODUCTIONEcosystem engineer species (Jones et al. 1994, 1997)create, modify and/or increase habitat heterogeneity (e.g. in the sea: corals, macro-algae, mussels, ascidians),and may be part of biological mechanisms maintaining high species richness at local, seascape (Roff et al. 2003),and regional scales (Tokeshi & Romero 1995, Crooks 1998, Crooks & Khim 1999, Cerda & Castilla 2001, Thiel & Ullrich 2002). According to Jones et al. (1994), the largest effects of engineering may be attributable to spe-cies with large per capita impacts, which live in high densities, generate structures that persist for long peri-ods and modulate the distribution and use of resources.However, to predict the effect of an ecosystem engineer species on ecological diversity, it is necessary to under-stand how the species pool responds to these changes (Wright et al. 2002). Several authors have predicted that ecosystem engineers increase species richness by alter-ing habit complexity and providing new habitat (Jones et al. 1994, 1997, Alper 1998, Coleman & Williams 2002,Reichmann & Seabloom 2002, Wright et al. 2002).Engineered habitats have different community com-positions compared to non-engineered ones. This can lead to increased species richness at the landscape scale (i.e. involving multiple patch types), when species are present that are restricted to engineered habitats at least during some stages of their life cycle (Wright et al.2002). Further, engineer species also create resources that are not otherwise available, and species that utilise them are subsequently present (Gutierrez et al. 2003).This suggests that although sites from engineered and© Inter-Research 2004 · *Email: jcastill@genes.bio.puc.clMarine ecosystem engineering by the alien ascidian Pyura praeputialis on a mid-intertidal rocky shoreJuan Carlos Castilla 1,*, Nelson A. Lagos 1,2, Mauricio Cerda 11Center For Advanced Studies in Ecology & Biodiversity (CASEB), Facultad de Ciencias Biológicas, Pontificia UniversidadCatólica de Chile, Casilla 114-D, Santiago, Chile2Present address: Escuela de Ciencias Básicas, Universidad Santo Tomás, Manuel Rodríguez 97, Santiago, ChileABSTRACT: Engineer species transform ecosystems due to their own growth, constitute an integral part of altered environments, and provide new habitats for other species, thus affecting biodiversity and the ecosystem. On rocky shores inside Antofagasta Bay (Northern Chile), the alien ascidian Pyura praeputialis , an engineer species, creates broad belts and dense 3-dimensional matrices that modify the intertidal habitat structure. In all, 116 species of macro-invertebrates and algae inhabit this habitat, compared with the 66 species inhabiting adjacent intertidal rocky shores which lack P. praeputialis . Of the 145 species recorded at the seascape scale (encompassing both mid-intertidal habitat), 55% were found exclusively in intertidal P. praeputialis matrices. Along the coastal gradi-ent, patterns in β-diversity emerge due to the addition of a new set of species to the community inhab-iting the P. praeputialis matrices and, to a lesser extent, from spatial turnover. We found differences in the shape of the species frequency distribution between the communities inhabiting the engi-neered and non-engineered mid-intertidal habitats. However, within the same habitat type, there was no difference in the species frequency distribution between functional groups. Occurrence of macro-algae was not affected by habitat type, but occurence of macro-invertebrates increased sig-nificantly in P.praeputialis matrices. P. praeputialis increases species richness at local and seascape scales by providing a novel mid-intertidal habitat which is used by mobile and vagile macro-inverte-brates that otherwise would remain excluded from this intertidal level.KEY WORDS: Pyura praeputialis matrices · Ecosystem engineer · Intertidal seascapes ·α- and β-diversity · Species frequency distribution · Northern ChileResale or republication not permitted without written consent of the publisherMar Ecol Prog Ser 268: 119–130, 2004non-engineered habitats may have similar local or α-di-versity patterns, β-diversity, along a geographical gra-dient (i.e. the spatial turnover in species composition), may show high values when sites from distinctive habitats are compared. However, spatial patterns in β-diversity may also arise from spatial trends in α-diversity (Harrison et al. 1992). Therefore, to under-stand the increase in species diversity promoted by ecosystem engineers, it is necessary to distinguish be-tween real spatial turnover in species composition be-tween engineered and non-engineered habitats and spatial differentiation between communities which are generated by the addition or loss of species from the regional pool. So far there are no studies addressing changes in marine diversity along geographical gradi-ents with regards to engineering processes. If the con-sequences of engineering are observed at the land-scape (or seascape) scale, with some species present exclusively in the modified habitat and others solely in the non-modified habitat (Wright et al. 2002), then the differences must be also reflected in changes in the species frequency distribution between habitats. Meta-population models have addressed regional frequency distributions with few common and many rare species (Brown 1984), or a bimodal shape (Hanski 1982). For in-stance, Collins & Glenn (1997) demonstrated that such patterns depend on the aerial extent of the study (i.e. distance scaling; see van Rensburg et al. 2000 for changes in species frequency distribution between patch types). This may also apply to patterns in species frequency distribution when comparing engineered and non-engineered habitats (hereafter referred to as habitat scaling). Since there are species that inhabit only non-engineered or engineered habitats (Wright et al. 2002), it is expected that at the landscape scale the species frequency distribution does not present com-mon species (i.e. species occurring in all plots across the landscape). The engineering effect on biodiversity would not necessarily be identical for all species in the landscape pool, where some species would benefit from the new habitat availability, while others would be excluded (Wright et al. 2002). Therefore, spatial pat-terns in species diversity and frequency distribution may change when applied to different taxa (i.e. organ-ismal scaling, Collins & Glenn 1997). In the context of ecosystem engineering, the segregation of species into functional groups (e.g. for rocky intertidal habitats: macro-invertebrates, sessile, mobile, macro-algae) may reveal which species set is most affected, in terms of spatial distribution, by the availability of the engineered habitat, and may also provide information regarding the mechanisms by which a engineer species generates an increase in species richness at the landscape scale. The barrel-shaped ascidian Pyura praeputialis gen-erates extensive aggregations of individuals in the mid-intertidal zone of rocky shores. In Chile, P. praeputialis is considered a recent invader (Castilla et al. 2002) and is exclusively present along approximately 60 to 70 km of coastline inside Antofagasta Bay. It shows a notable disjoint geographical distribution (Castilla & Guiñez 2000), with the nearest neighbours present along the southern shores of Australia (Kott 1985, Fairweather 1991, Dalby 1997, Castilla et al. 2002, Monteiro et al. 2002). P. praeputialis aggregations attain 3-dimensional (3D) pseudo-colonality (Paine & Suchanek 1983, Guiñez & Castilla 2001) that show wide, almost continuous in-tertidal belts and high percentage cover (ca. 1800 ind. m–2, Castilla et al. 2000). Cerda & Castilla (2001) re-ported that the macro-invertebrate diversity of species (96 taxa) inhabiting the P. praeputialis matrices is one of the highest values reported for the mid-intertidal zone in this geographical area. Nevertheless, the study did not incorporate mid-intertidal macro-algae, which are known to be important components of species diversity in northern Chile (Camus & Lagos 1996).The objectives of this paper are to: (1) report on the diversity of macro-algae and macro-invertebrates in-habiting Pyura praeputialis matrices, (2) test whether the novel mid-intertidal habitat provided by this alien ascidian promotes an increase in the species richness at the seascape scale (i.e. encompassing both habitat types: rocky shores with and without P. praeputialis matrices), (3) explore α-and β-diversity patterns to evaluate whether the increase in species richness is due to the addition or spatial turnover of species, (4)test whether species frequency distribution differs between the 2contrasting mid-intertidal habitats and between functional groups. Thus, our main underlying hypothe-sis is that matrices of P. praeputialis create new mid-intertidal habitats, otherwise unavailable in the region, which significantly increase species richness at the seascape scale and thereby modify biodiversity and the structure of intertidal communities in northern Chile.MATERIALS AND METHODSThe study was performed on 7 sites along approxi-mately 200 km of coastline (Fig. 1). All study sites were mid-intertidal rocky platforms that received direct wave exposure, had slopes <20°, and similar substrate heterogeneity (as described in Castilla 1981). Inside Antofagasta Bay we sampled mid-intertidal Pyura praeputialis matrices (i.e. belts of P. praeputialis matrix habitats:hereafter Pp MH) at 3 sites: Curva Lenguado, El Way and El Eden. North and south of the bay we sampled the mid-intertidal rocky habitat at 4 sites where P. praeputialis was absent (i.e. P. praeputialis-less substrate habitat: hereafter Pp-LSH): Caleta El Cobre and Jorguillo Point, both to the south of Antofa-120Castilla et al.: Intertidal ecosystem engineering by Pyura praeputialis gasta Bay, and Lagarto Point and La Lobería Point,both to the north of the bay (Fig. 1).Sampling of Pp MH diversity.The optimum plot sizefor sampling macro-invertebrates in the Pyura praepu-tialis matrices was estimated as a 35 ×35 cm (0.1225m2)quadrant, with 4 replicates per site (Cerda & Castilla2001). To sample the P. praeputialis matrices we used thefollowing procedure: (1) a 35 ×35 ×35 cm iron cube wasrandomly placed on top of the Pyura matrix, at the heightof the mid-intertidal fringe (100% cover in all cases)approximately 1 m above the lower intertidal limit ofP.praeputialis belt (Castilla 1998, Cerda & Castilla2001),(2) the cube was hammered into the Pyura matrixuntil reaching the underlying rock, (3) individual P.praeputialis were carefully removed using iron chisels,(4) all macro-invertebrates and macro-algae (larger than5 mm) found inside the P. praeputialis clumps werecollected, (5) the removed P. praeputialis clumps werestored in plastic bags, transported to the laboratoryand kept at –18°C, (6)each clump was separated into P.praeputialis individuals,and invertebrates and algaefound in or on the tunicate where collected, (7) residualmaterial (i.e. sand, gravel, and broken shells) generatedduring the sorting was separated out using 500 µm plas-tic sieves, and remaining invertebrates were collected.All individuals collected were identified to the lowesttaxonomic level (Cerda & Castilla 2001).Sampling of Pp-LSH diversity. We sampled mid-intertidal communities on rocky platforms, where thecondition of rocky shore lack Pyura praeputialis andthe mussel Perumytilus purpuratus(which is the domi-nant intertidal species of central Chile, Broitman et al.2001). In general, the mid-intertidal zone of northernChile is dominated by 3 sessile species: the barnacles Jehlius cirratus and Notochthamalus scabrosus and the macro-alga Ulva sp. (Camus & Lagos 1996). At each study site, we used 6 randomly placed 50 ×50 cm (0.25m2) sample quadrants following this sampling scheme: (1) plots were randomly placed at the mid-intertidal fringe, approximately 1 m above the upper limit of the Lessonia nigrescens kelp belt, (2) macro-invertebrates and macro-algae were removed by hand, using knives and iron chisels, and stored, analysed and taxonomically identified as indicated above.Data analysis. We constructed a database combining our data for macro-algae and macro-invertebrates, for both mid-intertidal habitat types, with data from Cerda & Castilla (2001) for macro-invertebrates inhabiting the Pp MH. Data were pooled from the 2study programs, presenting differences in the number and size of quadrats used; therefore, standardisation by area was carried out (Gotelli & Colwell 2001).To estimate the de-gree of overlap in species composition between the 2 habitat types we used non-metric multidimensional scaling ordination (NMS, McCune & Meffort 1999)based on species presence–absence data at the plot level. We also explored differences in levels of similar-ity in species composition within and between habitat types, calculating the Morisita-Horn similarity index for all pair-wise comparisons of plots1.Testing for differ-ences in species composition was done using 1-way ANOVA with 3 levels of comparison: Pp-LSH vs Pp-LSH, Pp MH vs Pp MH, and Pp MH vs Pp-LSH. The pro-portional data of similarity indices were square-root transformed to meet ANOVA assumptions (SAS Insti-tute 1996). Species richness in Pp MH and Pp-LSH was estimated by pooling all plots from each habitat type (12 and 24 plots respectively). At the seascape scale, species richness was estimated by pooling data from all plots (n = 36). Estimates of species richness were rar-efied to correct for differences in area and sample size, as suggested by Gotelli & Colwell (2001), by using 100 runs of the Coleman rarefaction estimate1.In all cases,121 Fig. 1. Study region on the northern Chilean coast. Pyura praeputialis only inhabit the rocky intertidal and shallow subtidal areas (bold line) along the coast of Antofagasta Bay (d: study sites). Adjacent sites are located in areas with ex-tended rocky shores (s: Pyura praeputialis-less substratehabitat[Pp-LSH] study sites)1Colwell RK (1997) EstimateS: statistical estimation of species richness and shared species from samples, Version 5. Avail-able at /estimatesMar Ecol Prog Ser 268: 119–130, 2004the estimated species richness reached an asymptotic value indicating an adequate sample size. The size of the effect of Pp MH on species richness (∆S)in the mid-intertidal zone was assessed by estimating the differ-ence in species richness between both habitat types.This was accomplished by computing the 2 observed distributions for species richness (S), with mean S 2 for Pp -LSH and S 1for Pp MH. This was calculated as: ∆S =S 1– S 2. To avoid non-independence of pair-wise differ-ences between S 1 and S 2 we approximated the distribu-tion of ∆S through simulations in a Bayesian framework (see Albert 1996). Using a uniform non-informative prior distribution, we simulated 5000 random values from each normal distribution using the Coleman esti-mated species richness and its standard deviation (Col-well 1997). The difference between S 2 and S 1was com-puted for each pair of simulated values, and the 95%Student’s t -test confidence interval for the posterior simulated distribution of ∆S was estimated. These analyses provided us with an error measure in the esti-mation (e.g. Wright et al. 2002). We computed ∆S using the Coleman estimated species richness at the asymp-totic value and the estimate corrected for the sampled area (Gotelli & Colwell 2001, Wright et al. 2002). Given that the Pp MH plots were smaller than the Pp -LSH plots, we selected a corrected area of 1.4 m 2to compare the estimated species richness between the contrasting habitats (11 plots from Pp MH and 6 plots from Pp -LSH).To explore how the presence of mid-intertidal Pyura praeputialis matrices modifies the spatial geographic di-versity pattern along the studied rocky shores, we grouped the species into 4 functional groups: macro-algae, sessile macro-invertebrates, mobile macro-invertebrates, and vagile macro-invertebrates. For each functional group, and for the entire set of taxa, we cal-culated the α-and β-diversity along the geographical gradient. To examine the spatial turnover in species composition between adjacent sites, we used Whittaker’s (1972) measure of β-diversity, β-1 = (S /m ) – 1; where S is the combined number of species in the paired, adjacent sites, and m is the average richness for the 2 sites. This measurement ranges from zero (complete similarity) to 1(complete dissimilarity). However, to distinguish between the true spatial turnover from the spatial trends in the α-diversity patterns induced by simple losses or additions of new species, we used the β-2-diversity index = (S /αmax ) – 1/(n – 1); where αmax refers to the maximum value of α-diversity of all n sites (Harrison et al. (1992).The spatial patterns of α-, β-1- and β-2-diversity, calcu-lated for the entire set of taxa, were correlated with the corresponding diversity index, but calculated separately for each functional group. The analysis provided an approximation for which functional groups were more important in the generation of the geographical pattern of differentiation in species composition.To assess whether the frequency distributions of spe-cies inhabiting Pp MH was different from those in Pp -LSH, we calculated the proportion of plots (P)occupied by each species i , as P i = p/n;where p is the number of plots occupied by the species and n is the total number of plots. The analysis was done at the scale of plots since there was evidence of changes in species diver-sity at the level of individual Pyura praeputialis (Mon-teiro et al. 2002) and not at the scale of the sites (Cerda & Castilla 2001). We explored the effect of habitat scal-ing (see Collins & Glenn 1997) by plotting the species frequency distribution of the mid-intertidal community for each habitat type and at the seascape scale. We also explored the effect of organismal scaling (Collins &Glenn 1997) by plotting the species frequency distribu-tion for 2 functional groups (macro-invertebrates and macro-algae) within the 3habitat categories. To under-stand whether Pp MH, in addition to causing changes in the shape of the species frequency distribution, also promoted an increase or decrease in species occur-rence, we estimate the effect of Pp MH as the differ-ence, ∆P i , = P i Pp MH – P i Pp -LSH . We plotted the frequency distribution of ∆P to obtain the shape and direction of the effect, using the entire taxa data set, and segre-gated the data into macro-invertebrate and macro-al-gae functional groups. Difference in organismal, habi-tat scaling and in the shape of ∆P between functional groups was tested using a Kolmogorov-Smirnov 2-sam-ple test, whereas the direction of the effect of ∆P was tested using a 1-sample Wilcoxon signed-rank test (Sokal & Rohlf 1991).RESULTSEffects on mid-intertidal species richness Community compositions of the mid-intertidal Pp MH and the corresponding rocky mid-intertidal habitat (Pp -LSH) were different (Table 1). Of the122Functional groupPp MHPp -LSH CommonAlgae Sessile 71213Invertebrates Mobile 56917Sessile 977Vagile 710Total 792937Table 1. Species richness for macro-algae and macro-inverte-brates (separated by functional groups) inhabiting the mid-in-tertidal of the Pyura praeputialis matrix habitats (Pp MH),Pyura -less substrate habitat (Pp -LSH) and species common to both mid-intertidal habitats (last column) in the rocky shore ofthe study zoneCastilla et al.: Intertidal ecosystem engineering by Pyura praeputialis79species recorded exclusively in the Pp MH, 91% cor-responded to macro-invertebrates and 9% to macro-algae species. In the Pp-LSH there was a total of 29intertidal species, of which 59% were macro-inver-tebrates and 41% macro-algae species. Thirty-seven species were common to both mid-intertidal habitats (Table 1). The difference in community composition was evident using NMS, which showed a strong segre-gation between Pp MH and Pp-LSH plots (Fig. 2A). Furthermore, there was a significant difference in the similarity of plots within and between habitat types (ANOVA, F2,969= 72.76, p = 0.0001) (Fig. 2B). A com-parison of the community composition within Pp MH was highly similar, in contrast to the comparison within Pp-LSH plots. The between-habitat comparison, Pp MH vs Pp-LSH, showed a lower level of similarity (Fig. 2B).Differences in species composition translated into differences in species richness (Fig. 3A). Asymptotic Coleman species richness estimates (mean ±1 SD) for each habitat type were: 65.5 ±0.68 for Pp-LSH, and 116.4 ±1.2 for Pp MH (Fig. 3A). At the seascape scale, the species richness estimated by random sampling of plots from both habitat types was higher than that esti-mated for each habitat individually (S= 144.5 ±0.69). At the asymptote of the Coleman estimate, the effect size of the Pp MH on species richness with respect to richness in the Pp-LSH, was positive and significant, with ∆S = 50.91 species ±0.042 SD (Student’s t-test, p < 0.0001) (Fig. 3B). Nevertheless, the Coleman estimates corrected for area showed that the difference in spe-cies richness in both habitat types rose to ∆S = 62.8 spe-123 Fig. 2. (A) Ordination of community composition betweenintertidal habitats. Ordination of plots based on speciespresence using non-metric multi-dimensional scaling. d:Pyura praeputialis matrix habitats(Pp MH); s: rocky platformsor P.praeputialis-less substrate habitat (Pp-LSH). (B) Similar-ity (mean ±1 SE of Morisita-Horn similarity index for pairwisecomparison) of community composition within (Pp MH orPp-LSH) and between (Pp MH and Pp-LSH) mid-intertidalhabitat typesFig. 3. Pyura praeputialis.(A) Asymptotic estimated speciesrichness (S; ±1 SD) of hypothetical intertidal seascapes com-posed only by rock substrata (P.praeputialis-less substratehabitat, Pp-LSH), only by P. praeputialis matrix habitats(Pp MH), and the combination of both habitats types (seascapescale). Black bars represent the Coleman rarefaction estimateof species richness in an equal sized area of 1.4 m2in bothhabitat types. (B) Histogram for simulated values of ∆S, theaveraged effect size of P. praeputialis on mid-intertidal spe-cies richness. ∆S was calculated as the difference betweenboth habitat types in the Coleman estimate of species richnessat the asymptotic value (white bars) and corrected for anequal sized area (black bars)Mar Ecol Prog Ser 268: 119–130, 2004cies ±0.092 SD (Student’s t-test, p < 0.0001) (Fig. 3B). These patterns caused increased richness at the seascape scale, which exhibited 28.06species ±0.04 SD more than the richness estimated in the Pp MH. Of the 145 species recorded at the seascape scale, 55% were found exclusively in Pp MH plots, whereas 25% of the species shared both habitat types, and only 20% were found exclusively in Pp-LSH (Table 1).Effects on spatial turnover in species composition The geographical patterns along the studied rocky shores showed that for the entire taxa set, α-diversity increased in the sites with Pp MH and decreased in Pp-LSH sites (Fig. 4A). In addition, high differences in community composition, or β-1-diversity, were found in the pair-wise comparison between different habitat types (Fig. 4A). These spatial patterns, for total mid-intertidal species, corresponded well with those of α- and β-1-diversity for total macro-inverte-brate species (Fig.4B), but less so with the spatial pattern for total macro-algae (Fig. 4C, see Table 2 for r-Pearson correlation and p-values). In general, the relationship of α- and β-1-diversity patterns among the entire taxa set and the functional groups was positive and significant for all invertebrates and mobile inver-tebrates (Fig.4A,D, Table 2). However, the β-2 esti-mate did not translate into high values of community differentiation for the pair-wise comparison between different habitat types (×in Fig. 4). In addition, the relationship of β-2-diversity patterns among the entire taxa set and the functional groups was non-significant in all cases (Table 2). Along-shore spatial patterns in β-1 support that differences in community composition between habitat is influenced by invertebrates (in general) and mobile invertebrates (in particular), after removing the spatial trends in α-diversity (i.e. the increase in richness in Pp MH sites); the β-2 estimates do not show a clear spatial turnover. Thus, spatial differentiation among habitat types emerge from the addition of species, mainly mobile invertebrates and,to a lesser extent, to spatial turnover in species composition.124Fig. 4. α-, β-1-andβ-2-diversity patterns for (A) the all taxa set and (B–F) functional groups across the geographical gradient. β-measures were calculated between pairwise adjacent sites. Sites are aligned from south to north (see Fig. 1). Abreviations: CC = Caleta El Cobre, Jp = Jorgillo Point, CL = Curva Lenguado, EW = El Way, EE = El Eden, LP = Lagarto Point, LLP = La Loberia PointCastilla et al.: Intertidal ecosystem engineering by Pyura praeputialis Effects on species frequency distribution At the seascape scale, the species frequency distrib-ution showed an inverse relationship between the pro-portion of plots occupied and the number of species,with a mode (48 species) at a the lower proportion and,as predicted, a total absence of species at the highest proportion of plot occupied (zero species with a pro-portion of plot occupied of 1, Fig. 5A). When species frequency distributions were separated by habitat type (habitat scaling), the distribution in Pp MH appeared tobe multi-modal, with 3 modes, at low, mid- and highoccurrence (18, 15 and 20 spp. respectively) (Fig. 5B).For species inhabiting Pp -LSH, the species frequencydistribution also showed an inverse relationship, withmodes at low and intermediate proportions of plotoccupied (Fig. 5C). Significant statistical differences in species frequency distribution (p < 0.05) emerged when comparisons were done among habitat types (i.e.habitat scaling), but non-significant differences were founded in frequency distribution among functionalgroups within the same habitat type (i.e. organismalscaling, p > 0.05) (see Fig. 5).In the Pp MH a total of109 species exhibited an increasing, and 36 a decreas-ing, trend in the plot occurrence ∆P (Fig. 6A). This dis-125Functional group αβ-1β-2r-Pearson r -Pearson r -Pearson Invertebrates 0.99***0.98***0.06 nsAlgae 0.35 ns 0.77 ns 0.59 nsAll taxa with:mobile invertebrates 0.98***0.98***0.34 nssessile invertebrates 0.84 ns 0.90 ns 0.06 nsvagile invertebrates 0.89**0.60 ns 0.37 ns Table 2. Correlation in α-, β-1- and β-2-diversity among all taxa (see Fig. 4A) with the corresponding measure for each functional group (Fig. 4B–F). Correlation based on n = 7 sites for α-diversity and n = 6 pairwise comparison of adjacent sites for β-indices. Significance was evaluated using a Bonferroni correction: ***p < 0.001; **p < 0.01;ns: correlation was notsignificantFig. 5.Effects of habitat type (Pp MH =Pyura praeputialis matrix habitats; Pp -LSH = P.praeputialis -less substrate habitat, or rocky platforms; seascape scale = pooled habitats) and functional group (algae, invertebrates and all taxa set) on species frequency distribution. Numbers above each bar indicate the number of species. Habitat scaling corresponds to comparison of species frequency distribution the vertical axis (Kolmogorov-Smirnov test, p < 0.05 in all cases). Organismal scaling corresponds tocomparison on the horizontal axis (Kolmogorov-Smirnov test, p > 0.05 in all cases)。
轮机英语新版教材译文(党坤)
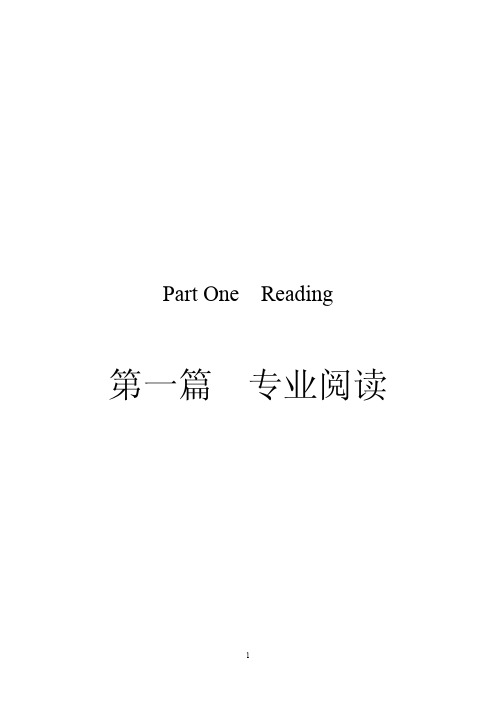
干货
单元货物(杂货):
√集装箱船 √滚装船 √重货船 √冷藏船
散货: √散货船 √矿砂船
√牲畜船
表 1 船舶类型 (1) 液货
旅客
√原油船 √成品油船 √化学品船 √液化石油气/液化天然气船
√客船 √客、货轮渡 √游船
海军渔业(Fra bibliotek) 多功能船挖泥等
工程船
2
√航母 √巡洋舰 √驱逐舰 √护卫舰 √潜艇 √扫雷舰
√拖网渔船 √其他捕鱼船
√耙吸式挖泥船 √绞吸式挖泥船 √废石倾倒船
√起重船 √布缆船 √浮筒带缆船 √溢油回收船 √人字起重架
辅助小船 √远洋拖船 √港内拖船 √破冰船 √引航船 √巡逻船 √科考船
轮机英语1800字
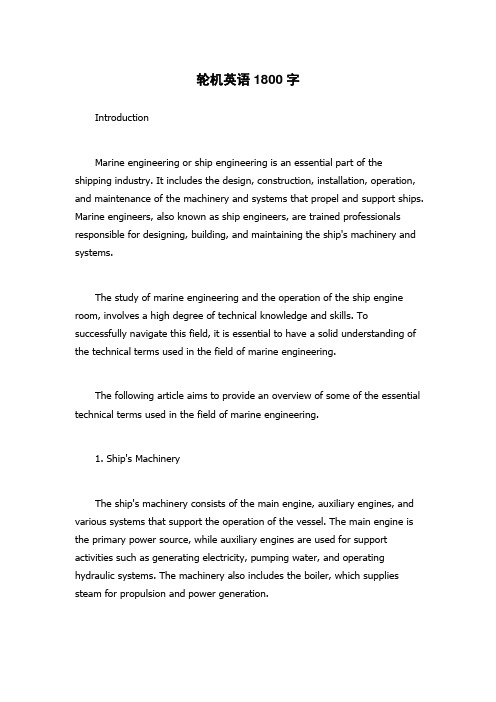
轮机英语1800字IntroductionMarine engineering or ship engineering is an essential part of the shipping industry. It includes the design, construction, installation, operation, and maintenance of the machinery and systems that propel and support ships. Marine engineers, also known as ship engineers, are trained professionals responsible for designing, building, and maintaining the ship's machinery and systems.The study of marine engineering and the operation of the ship engine room, involves a high degree of technical knowledge and skills. To successfully navigate this field, it is essential to have a solid understanding of the technical terms used in the field of marine engineering.The following article aims to provide an overview of some of the essential technical terms used in the field of marine engineering.1. Ship's MachineryThe ship's machinery consists of the main engine, auxiliary engines, and various systems that support the operation of the vessel. The main engine is the primary power source, while auxiliary engines are used for support activities such as generating electricity, pumping water, and operating hydraulic systems. The machinery also includes the boiler, which supplies steam for propulsion and power generation.2. Propulsion MachineryPropulsion machinery refers to the systems that generate and transmit power to drive the ship through the water. The propulsion system can be either mechanical or electrical. The mechanical propulsion system typically comprises of the main engine, reduction gear, and propeller.The electrical propulsion system, on the other hand, uses electric motors to drive the propeller. These motors are powered by the ship's generators, which convert the mechanical energy from the main engine into electrical energy.3. Engine RoomThe engine room is the space on the ship where the main and auxiliary engines, as well as other machinery, are located. This space is typically located below the main deck and is insulated to prevent heat transfer and minimize noise.To ensure the proper functioning of the machinery, the engine room is equipped with various systems that control the engine and maintain the optimal operating conditions. These systems include the lubrication system, cooling system, fuel system, and ventilation system.4. Lubrication SystemThe lubrication system is responsible for the smooth operation of the engine's moving parts. It supplies oil to the engine's bearings, cylinders, and other critical components to reduce friction and prevent wear and tear. Thesystem operates at high pressures and temperatures, making it essential to maintain the optimal oil level and pressure.5. Cooling SystemThe cooling system is responsible for maintaining the optimal operating temperature of the engine. It regulates the temperature by circulating water or coolant around the engine and radiating heat away from the system. The cooling system typically comprises the sea-water cooling system and freshwater cooling system.6. Fuel SystemThe fuel system supplies fuel to the engine and auxiliary engines. It includes the fuel storage tanks, fuel filters, fuel pumps, and fuel injection system. The system must be designed to prevent the ingress of water into the fuel, which can cause engine failure.7. Ventilation SystemThe ventilation system is responsible for circulating fresh air to all areas of the engine room. It ensures that the crew working in the engine room is not exposed to any hazardous gases or fumes that can cause respiratory problems.8. BoilerThe boiler is responsible for producing steam that drives the ship's turbine and generators. It works by heating water to a high temperature and pressure, converting it into steam. The steam is used to move the ship or generate electrical power.ConclusionMarine engineering and the operation of the ship engine room are complex and technical fields that require in-depth knowledge and expertise. Understanding the technical terms used in this field is essential to carry out the various tasks and responsibilities efficiently.The above list of technical terms provides an introductory overview of some of the essential concepts of marine engineering. A thorough understanding of each of these terms and their implications is essential to achieve success in this field.。
- 1、下载文档前请自行甄别文档内容的完整性,平台不提供额外的编辑、内容补充、找答案等附加服务。
- 2、"仅部分预览"的文档,不可在线预览部分如存在完整性等问题,可反馈申请退款(可完整预览的文档不适用该条件!)。
- 3、如文档侵犯您的权益,请联系客服反馈,我们会尽快为您处理(人工客服工作时间:9:00-18:30)。
锅炉系统1 冷凝器1.1 简介欧堡生产的冷凝器是用直管和一个外部密封浮头组成的管壳式冷凝器。
这种冷凝器主要用作废气锅炉,蒸汽加热或洗舱海水加热器的转储冷凝器/冷却器排水。
并取得权威船级社批准。
温度计,排水阀,空气阀,压力表的安装设计为½“BSP内螺纹安装。
这些组件可能是指定的。
而蒸汽或水的控制设备是可选的。
1.2 安装空间要求安装时必须有足够的空间供作清洗,检查或更换管的插入与撤出。
冷凝器必须安置在水平并稳定的表面。
1.3 存储如果冷凝器在安装前要闲置一段时间,应存放在干燥的储藏室里。
如果储藏室潮湿,冷凝器必须放在装有硅胶的包装袋子里。
为了避免破坏,建议冷凝器放在原包装中。
冷凝器已经在交付前做过液压试验。
试验中所用测试媒质含有一定的数额抗腐蚀保护物质。
当冷凝器需闲置的时间较长,建议使用指定产品作为防腐蚀物质1.4 安装冷凝器设计为垂直或水平安装。
在水平安装的情况下,蒸汽喷嘴必须朝上,而冷凝水出口喷嘴朝下。
如果是垂直安装冷凝器,蒸汽入口和海水出口端必须朝上。
排水和空气排放阀必须安装在冷凝器在最低和最高点的中间线的位置。
任何选择性的控制设备必须根据具体指示安装。
步骤A:将冷凝器安装在水平平面上。
步骤B:钻基础固定螺栓孔。
步骤C:将螺栓放入孔中并拧紧。
连接冷凝器步骤D:移除所有的塞子和盲板,然后再连接冷凝器。
步骤E:在连接中确保没有杂质进入。
步骤F:管道连接起来,确保从管道和冷凝器之间没有强制力的产生。
1.5 调试启动前要确保所有连接都牢固地拧紧是很重要的。
同样重要的是,冷凝器和连接管道空气要彻底排出。
步骤A:如果装有安全阀,必须加以调整到最大设计压力或较低。
步骤B:法兰螺栓要拧紧。
拧紧法兰螺栓时始终使用扭矩扳手。
步骤C:运行一小时,停止冷凝器,并重新拧紧所有螺栓。
步骤D:启动阶段,冷凝器的两边都要排出空气,必须认真仔细的检查回路的泄漏。
1.6 性能冷凝器性能须附和传热计算表规定的要求。
,保持指定的蒸汽压力特别重要,因为压力降低可能导致进口速度过大,将会使其产生噪音并损害冷凝器,而较低的压力下会降低热性能。
通常,有效评估冷凝器性能的方法是通过监测评估冷凝水出口温度或海水出口温度。
如果冷凝水温度过高或海水出口温度过低,要么是冷凝器需要清洗或操作参数不正确。
需要检查其工作条件,并采取适当的纠正行动,以使性能恢复所要求的水平。
1.7 在关闭期间的保护在关闭期间发生的腐蚀,主要是由于氧气的存在。
如果存在非蒸馏水,如碱性或海水在冷凝器中,就很容易发生腐蚀。
然而,即使在完全放空的情况下,冷凝器接触到残留的冷凝水仍然会造成腐蚀。
因此,当停止工作时,应当通过使用淡水冲洗海水端,并仔细在两端泄放以保护冷凝器,如果冷凝器需在一个较长时期的停止工作,尤其是在寒冷的冬季。
必须小心清空。
打开空气阀口并检查堵塞情况。
1.8 维护和清洁冷凝器应定期清理,每十二个月或当没有其他原因的情况下而输出下降的时候,应将内部的管子移出清洁。
步骤A:检查是否有水在收集箱或管子中。
步骤B:将内部管束取出后,用有压力的液体进行外部清洗步骤3:将管束的上盖移除后,使用酸性的液体从内部进行清洁,表面的杂质可以用软质尼龙刷去除。
但很重要的一点是进行机械清除时不要破坏管子的表面。
步骤D:安全阀必须每年都要检查其设置点是否正确。
1.9 拆卸和重装管束当需要将管束从冷凝器中取出时,必须拆除海水连接头,拆下蒸汽进口处的水箱,松下其他水箱的连接螺栓需要利用水箱中的顶升螺栓将水箱和管架连接用的紧固O形圈拉开。
在拉出管束前,应使用带状材料缠绕保护管架箱浮动端的表面。
由于管束和包装表面容易损伤,一定要轻拿轻放。
检查管束,水箱和外壳是否遭到破坏或过度磨损,进行必要的维修和清洗后,重新组装冷凝器。
在水箱浮动端的环槽中安装新的O形圈,并涂耐热硅润滑脂,使安装更容易,并可以增加垫材的使用寿命。
然后将管束装入管架中,在插入管束前,管架始终要用包装材料保护。
使抗冲击板块正对蒸汽喷嘴的冲击是十分重要的,不然可能会因为管子被过度侵蚀而发生故障。
安装末端水箱。
取下管架浮动端的包装带,轻轻地将浮动端水箱滑上。
这是极为重要的板块正面临冲击的蒸汽喷嘴,否则可能会失败,使用法兰螺栓确保无偏斜地固定水箱后,装上管子并锁紧螺丝。
垫片表面要清洁并防止损坏,每拆下一个接头都要更换新的垫片。
应当尽量使用原装垫片和垫圈,并保证安装牢固紧密。
需始终使用扭力扳手上紧法兰螺栓。
运行冷凝器一个小时,停止,然后上紧法兰螺栓。
对接头的紧密性进行压力实验。
在运行期间,对冷凝器进行小心的泄放,并对循环回路进行检查是否漏泄。
1.10 备件订货时请注明零件的冷凝器(在铭牌上)的序列号。
需要备件的进一步信息,请联系欧堡工业。
2 化学定量泵2.1 简介化学定量泵是由微处理器控制的电磁定量泵。
该泵能够准确显示液体化学品加药压力管道系统中的流量,并能进行容器的打开和关闭。
化学定量泵的主要组成部分包括操作面板,电源端,液源腔该泵的工作原理是液端定量膜由微处理器控制作动的电磁铁驱动,形成脉冲作用在化学液体上,这就引起吸口端,液体腔和排出端之间产生压力差。
这个压力差将引起吸口和出口的自动阀开启或关闭,从而供应化学液体。
泵的容量(供给速度)取决于行程长度和行程率。
冲程长度使用行程长度调节旋钮在0%和100%间调节。
最佳剂量是设置在30%和100%行程的长度。
行程率10%和100%之间使用的多功能开关进行步进调节,每级为10%。
但在外部模式中,行程率是由电信号控制的。
2.2 安全在安装,调试,运行,维护和修理期间必须始终应遵守遵循以下安全原则请注意,泵的设计不适合用于:(1)气体化学物质或悬浮固体和容易爆炸的危险场所。
(2)在紧急情况下的泵应立即关闭。
断开电源的电源线。
(3)在任何时候都应该能够方便地进行操作和管理。
附近不能有障碍(4)水泵和外围设备只有合格和有授权的维修人员才能对其进行维修和管理。
(5)当做有关泵的工作时,首先要断开电源(6)在做有关泵的工作前,必须泄放出口管路上的压力液体,放空液腔中的液体,并冲洗干净。
(7)务必阅读化学品安全数据。
(8)处理危险或未知的化学物质,务必穿上防护服时(9)如果排出管被封锁,切勿让定量泵运转,因为这可能会导致排出管道破裂。
2.3 安装2.3.1 一般安装化学定量泵通常预装在化工罐上。
因此该泵安装或更换的地方必须遵守以下安装注意事项:(1)化学定量泵必须位于一个环境温度在-10 C至+45之间,在非冷凝的情况下相对湿度不超过92%的环境中(2)泵的泵脚必须牢固地安装在牢固的基准座上,泵运行时不能震动(3)启动和运行时不得超过其最大允许压力。
这种泵的最大吸高为2 mmWC,最大工作压力为16巴。
例如由于化学物质供应系统的位置,导致运行压力超标的情况下,在出口端必须安装一个安全阀,多余的液体回到吸口(4)吸入和排出阀必须安装在垂直位置。
(5)其管子必须具备这样的要求,即如果需要,允许将泵和液腔拆开,在安装时不能产生应力和弯曲,所以只有原装的产品才能达到这种尺寸要求。
(6)吸入管应呈上升趋势并尽可能短以防止气泡的形成。
(7)由于在底部有化学品的沉淀和杂质,底阀的位置要高于它们的位置。
(8)连接到供应系统的出口管的止回阀必须上紧。
2.3.2 电气安装该泵是和主电源的连接通过主电源线。
如果泵连接到电源时与感应元件并联,必须对他们进行电器隔离,防止开关断开时产生的冲击造成损害。
2.4 操作这个化学定量泵的操作面板配有支持泵的设置和运作操作开关,接线端子,和指示器。
该泵可通过手动操作控制或外部控制。
如果泵仅需要手动操作模式时将不会提供可选的终端接到“外部控制”。
当泵需要是通过“外部控制”和/或“浮动开关”控制时,必须提供可选的终端外部控制。
“在化学品罐中装有测量液位的浮动开关,它能够提供液位信号到泵。
由于液位的变化需要安装两个浮动开关到接线端子。
同时泵也需要进一步安装故障监测继电器用于低水位报警。
以下为泵操作指令的完整描述。
如果化学计量泵包括零件或功能,不是用于定量供应系统,这些指令可以忽略。
2.5 泵的基本信息2.5.1 行程长度调节旋钮行程长度可以在0%和100通过调节冲程长度调节旋钮进行连续性的调节。
在技术上只有再现性在调整范围30%至100%可行。
2.5.2 多功能开关多功能开关用来选择操作模式和设置行程率。
下面的操作模式是供多功能开关选择的模式:停止。
外部。
手动(设置在10%的行程步进率)。
测试(自吸功能)。
(1)停止“停止”功能使泵电源未断开也可以停用。
(2)外部外部操作模式允许通过触点或者半导体的外部模式操纵设备单独调节其行程“暂停”功能,可以通过外部终端远程操作使泵停止。
(3)手动操作手动操作模式通过多功能开关对行程率进行10%的步进调整。
(4)测试“测试”功能检查泵的自吸的功能。
开关设置在测试位时,多功能开关“测试”是自动的。
2.5.3 LED指示灯运行和错误状态通过操作面板上的三个指示灯显示:绿色LED指示灯,操作显示:此LED点亮时排出行程被激活。
黄色的LED指示灯,报警指示灯:如果设备装有浮动开关,LED灯亮时,表明液面低于第一触发液面。
红色LED指示灯,故障指示灯:如果装有浮子开关,指示灯亮起来时说明液位测量发生故障。
它闪烁时表示未定义的运行状态2.6 保养.为确保化学定量泵工作安全和性能可靠,必须进行定期保养。
正常保养间隔为三个月。
这是根据其运行时间大约为30%时制定的。
但在使用比较频繁的情况下,保养周期应缩短。
进行以下维护工作:检查泵短期运行时进给率是否正确。
检查排气孔处的化学品是否渗流。
检查排出管与液源端连接是否牢固。
检查排出和吸入阀是否牢固地固定。
检查液源端尤其是排气孔处的水密性。
检查膜片是否损坏检查液源端的固定螺丝是否上紧。
检查泄放阀是否牢牢地固定到位。
检查泄放功能是否正常运行。
检查电气接线有没有破损。