2011-10-9胆汁酸的综述
检测血清总胆汁酸用于肝病诊断分析
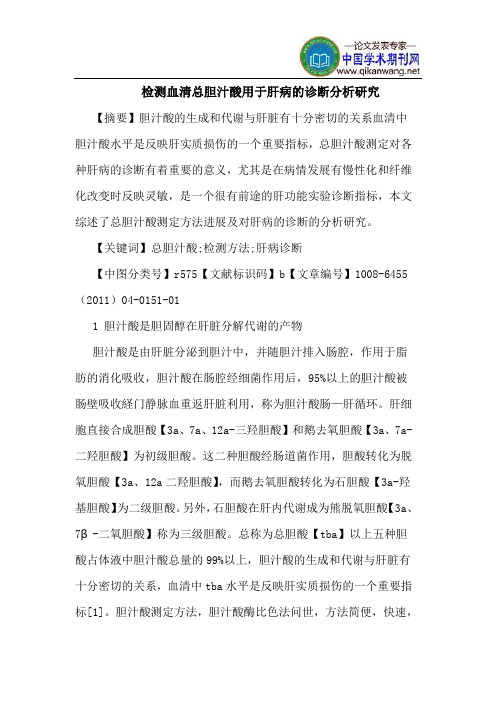
检测血清总胆汁酸用于肝病的诊断分析研究【摘要】胆汁酸的生成和代谢与肝脏有十分密切的关系血清中胆汁酸水平是反映肝实质损伤的一个重要指标,总胆汁酸测定对各种肝病的诊断有着重要的意义,尤其是在病情发展有慢性化和纤维化改变时反映灵敏,是一个很有前途的肝功能实验诊断指标,本文综述了总胆汁酸测定方法进展及对肝病的诊断的分析研究。
【关键词】总胆汁酸;检测方法;肝病诊断【中图分类号】r575【文献标识码】b【文章编号】1008-6455(2011)04-0151-011 胆汁酸是胆固醇在肝脏分解代谢的产物胆汁酸是由肝脏分泌到胆汁中,并随胆汁排入肠腔,作用于脂肪的消化吸收,胆汁酸在肠腔经细菌作用后,95%以上的胆汁酸被肠壁吸收経门静脉血重返肝脏利用,称为胆汁酸肠—肝循环。
肝细胞直接合成胆酸【3a、7a、12a-三羟胆酸】和鹅去氧胆酸【3a、7a-二羟胆酸】为初级胆酸。
这二种胆酸经肠道菌作用,胆酸转化为脱氧胆酸【3a、12a二羟胆酸】,而鹅去氧胆酸转化为石胆酸【3a-羟基胆酸】为二级胆酸。
另外,石胆酸在肝内代谢成为熊脱氧胆酸【3a、7β-二氧胆酸】称为三级胆酸。
总称为总胆酸【tba】以上五种胆酸占体液中胆汁酸总量的99%以上,胆汁酸的生成和代谢与肝脏有十分密切的关系,血清中tba水平是反映肝实质损伤的一个重要指标[1]。
胆汁酸测定方法,胆汁酸酶比色法问世,方法简便,快速,可以用手工操作,也可以进行自动化测定,为临床实验室开展这项检测创造了条件,本文就血清tba测定方法对肝病的诊断作分析研究。
2 酶比色法1974年挪威的skaihegg[2]等应用等电聚焦技术成功地提取了高纯度3a-羟类固醇脱氧酶【3a-hsd】是胆汁酸测定的一种工具酶。
随后,日本第一化学药品株式会社【下称第一化学】用纯制3a-hsd 研制成第一代酶法试剂盒enzabile[10]从此tba测定进入临床检验领域,相继有开发出第二代试剂盒enzabile-2并取消专利权,其特点是双途径通向一指示系统,成倍提高了色素生成,从而提高了消光系数,克服了第一代试剂在低值标本时测定重复性差的缺点,这种方法是目前最简单的胆汁酸测定方法,可以用于手工操作,又可以进行自动化测定,能满足临床实验室大批量的常规工作使用,其不足之处是只能做tba测定,不能去分胆酸的各个组份,但作为临床用于肝功能检测tba能满足使用。
医学类课件胆汁酸类成分.pptx
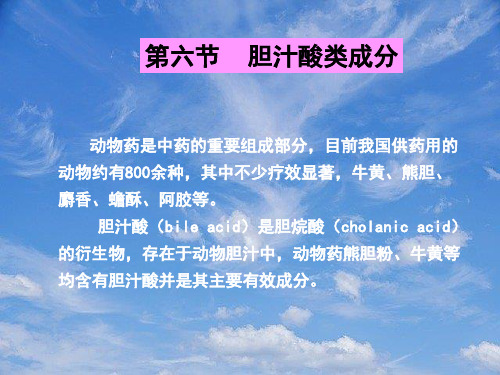
三、检识 除上述颜色反应可用于胆汁酸检 识外,尚可用色谱法检识。 (一)纸色谱 纸色谱法对胆汁酸的分离效果较 好,但展层时间较长。
1、纸色谱的溶剂系统有酸性和碱性两大类。 在酸性溶剂系统中,大多以70%乙酸作固定 相浸渍滤纸,100℃以下干燥,在层析缸中用两相 溶剂饱和平衡,再用不同比例的展开剂展开。 常用的酸性溶剂系统为异丙醚-庚烷(85: 15→10:90)、氯乙烯-庚烷及乙酸异戊酯-庚烷 等。 碱性溶剂系统对胆汁酸的分离能力较差,仅 可作为酸性溶剂系统的补充,常用的有正丙醇-氨 水-水、正丙醇-氨水-乙酸胺-水等。
2、显色剂 10%磷钼酸乙醇液、15%磷酸乙醇
液、10%硫酸乙醇液、间二硝基苯乙醇 液、三氯化锌的氯仿溶液等。
(二)薄层色谱 硅胶薄层色谱广泛用于动物胆汁中的胆汁酸分 离和鉴定。 1、分离游离胆汁酸的展开剂有异辛烷-异戊醚冰乙酸-正丁醇-水(10:5:5:3:1)、异辛烷-乙酸乙 酯-冰乙酸(17:7:5)等。
3-α,6α,7α-OH 猪
α-猪去氧胆酸 (α-hydroxycholic acid)
3-α,6α-OH
猪
β-猪去氧胆酸 (β-hydroxycholic acid)
3-β,6α-OH 3-α,6β -OH
猪,特别在结石 猪
鹅去氧胆酸 (chenodeoxycholic acid)
3-α,7α-OH
(三)气相色谱 气相色谱不能直接分离鉴定结合型胆 汁酸,必须将结合型胆汁酸预先用碱液 (2.5mol/L NaOH)水解成游离胆汁酸, 然后将游离胆汁酸的羧基和羟基分别经甲 酯化和三甲基硅醚化后再进行气相色谱分 离。例如用3%OV-17柱,氮气为流动相, 氢火焰鉴定器检出,可满意地分离熊去氧 胆酸与鹅去氧胆酸,检出灵敏度高。
医学胆汁与胆汁酸的代谢专题课件

➢ 合成过程:复杂
2. 次级胆汁酸在肠道由肠菌作用生成
(1)部位: 小肠下段和大肠 (2)过程: 初级胆汁酸 肠菌
水解、脱羟
次级胆汁酸
3. 胆汁酸肠肝循环概念:
胆汁酸肠肝循环概念: 胆汁酸随胆汁排入肠腔后,约95%可经门静脉重吸 收入肝,在肝内转变为结合胆汁酸,经胆道再次排入肠腔的过程, 称胆汁酸的肠肝 循环(enterohepatic circulation of bile acid) 。
胆汁与胆汁酸的代谢
一、胆汁可分为肝胆汁和胆囊胆汁
肝胆汁 胆道系统 胆囊胆汁
(肝细胞分泌)
(肝胆汁经胆囊浓缩)
胆汁的主要有机成分: 胆汁酸盐(含量最高) 胆固醇 胆色素 多种酶类等
比重 pH 水 固体成分 无机盐 黏蛋白 胆汁酸盐 胆色素 总脂类 胆固醇 磷脂 比重
两种胆汁的百分组成和部分性质
例:胆酸
(2)结合胆汁酸
OH
CONHCH2CH2SO3H
12
例:牛磺胆酸
3
HO
H
7 OH
OH
CONHCH2COOH
12
例:甘氨胆酸
3
HO
H
7 OH
2. 按来源分
初级胆汁酸 ( primary bile acid ) 次级胆汁酸 ( secondary bile acid )
(1)初级胆汁酸:在肝细胞以胆固醇为原料直接合成的胆汁酸,包括胆酸、鹅脱 氧胆酸及其与甘氨酸或牛磺酸的结合产物 (2)次级胆汁酸:在肠菌作用下,第7位α羟基脱氧生成的胆汁酸称为次级胆汁酸, 主要包括脱氧胆酸和石胆酸及其在肝中分别与甘氨酸或牛磺酸的结合产物
初级胆汁酸
胆汁淤积与胆汁酸转运蛋白关系的研究进展
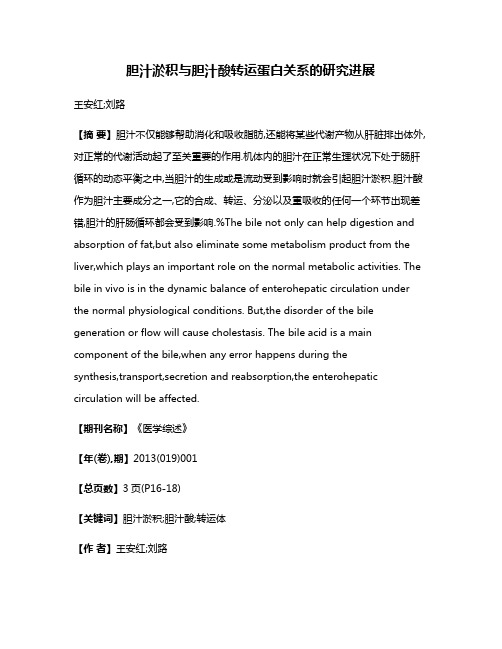
胆汁淤积与胆汁酸转运蛋白关系的研究进展王安红;刘路【摘要】胆汁不仅能够帮助消化和吸收脂肪,还能将某些代谢产物从肝脏排出体外,对正常的代谢活动起了至关重要的作用.机体内的胆汁在正常生理状况下处于肠肝循环的动态平衡之中,当胆汁的生成或是流动受到影响时就会引起胆汁淤积.胆汁酸作为胆汁主要成分之一,它的合成、转运、分泌以及重吸收的任何一个环节出现差错,胆汁的肝肠循环都会受到影响.%The bile not only can help digestion and absorption of fat,but also eliminate some metabolism product from the liver,which plays an important role on the normal metabolic activities. The bile in vivo is in the dynamic balance of enterohepatic circulation under the normal physiological conditions. But,the disorder of the bile generation or flow will cause cholestasis. The bile acid is a main component of the bile,when any error happens during thesynthesis,transport,secretion and reabsorption,the enterohepatic circulation will be affected.【期刊名称】《医学综述》【年(卷),期】2013(019)001【总页数】3页(P16-18)【关键词】胆汁淤积;胆汁酸;转运体【作者】王安红;刘路【作者单位】天津中医药大学中医药研究院,天津市中药药理重点实验室,天津,300193;河南大学药学院,河南,开封,475001【正文语种】中文【中图分类】R966胆汁淤积简称淤胆,是由胆汁生成障碍或胆汁流动障碍所致的一组疾病共同的临床症状,又名胆汁淤积综合征。
2011胆汁酸信号与肝脏代谢和疾病

Hindawi Publishing CorporationJournal of LipidsVolume2012,Article ID754067,9pagesdoi:10.1155/2012/754067Review ArticleBile Acid Signaling in Liver Metabolism and DiseasesTiangang Li and John Y.L.ChiangDepartment of Integrative Medical Sciences,Northeast Ohio Medical University,Rootstown,Ohio,OH44272,USACorrespondence should be addressed to John Y.L.Chiang,jchiang@Received10June2011;Accepted4August2011Academic Editor:William M.PandakCopyright©2012T.Li and J.Y.L.Chiang.This is an open access article distributed under the Creative Commons Attribution License,which permits unrestricted use,distribution,and reproduction in any medium,provided the original work is properly cited.Obesity,diabetes,and metabolic syndromes are increasingly recognized as health concerns worldwide.Overnutrition and insulin resistance are the major causes of diabetic hyperglycemia and hyperlipidemia in humans.Studies in the past decade provide evi-dence that bile acids are not just biological detergents facilitating gut nutrient absorption,but also important metabolic regulators of glucose and lipid homeostasis.Pharmacological alteration of bile acid metabolism or bile acid signaling pathways such as using bile acid receptor agonists or bile acid binding resins may be a promising therapeutic strategy for the treatment of obesity and diabetes.On the other hand,bile acid signaling is complex,and the molecular mechanisms mediating the bile acid effects are still not completely understood.This paper will summarize recent advances in our understanding of bile acid signaling in regulation of glucose and lipid metabolism,and the potentials of developing novel therapeutic strategies that target bile acid metabolism for the treatment of metabolic disorders.1.IntroductionBile acids are produced only in the liver as the end products of cholesterol catabolism[1,2].In addition to the classic function of bile acids in facilitating hepatobiliary secretion of endogenous metabolites and xenobiotics and intestine absorption of lipophilic nutrients,bile acids also play equal-ly important roles in controlling the metabolism of glu-cose and lipids in the enterohepatic system,and energy ex-penditure in peripheral tissues[3,4].Because of such a close association between bile acid signaling and metabolic home-ostasis,targeting bile acid metabolism by using bile acid re-ceptor agonists or bile acid-binding resins have proven to be effective in improving lipid and glucose homeostasis in obesity and diabetes[5].Furthermore,stimulating de novo bile acid synthesis prevented,whereas,disruption of bile acid signaling caused insulin resistance and dyslipidemia in mice, indicating that impaired bile acid homeostasis may likely contribute to the pathogenesis of metabolic disorders[6–9].This paper will summarize recent advances in our un-derstanding of bile acid signaling regulation of glucose and lipid metabolism and the potentials of developing novel ther-apeutic strategies that target bile acid metabolism for the treatment of metabolic disorders.2.Bile Acid SynthesisBile acids are the end product of cholesterol catabolism in the liver[1,10–12].In humans,the bile acid pool consists of primary bile acids cholic acid(CA),chenodeoxycholic acid (CDCA),secondary bile acids deoxycholic acid(DCA),and lithocholic acid(LCA).Primary bile acids are synthesized from cholesterol through two general pathways,the classic pathway and the alternative pathway.Secondary bile acids are derived from primary bile acids in the intestine by bacterial enzymes.Enzymes that catalyze these multistep reactions are located in the endoplasmic reticulum,mitochondria,cyto-sol,and peroxisomes.In humans,the classic pathway is con-sidered as the major bile acid synthetic pathway(accounts for more than90%of total bile acid production)and produces CA and CDCA in approximately equal amounts.Cholesterol 7α-hydroxylase(CYP7A1),a microsomal cytochrome p450 enzyme,catalyzes thefirst and rate-limiting step in the classic pathway[13].The intermediate product in the clas-sic pathway7α-hydroxy-4-cholestene-3-one serves as the common precursor for both CA and CDCA.7α-hydroxy-4-cholestene-3-one can be hydroxylated at C-12position by microsomal sterol12α-hydroxylase(CYP8B1),followed up by several reactions including mitochondrial27-hydroxylase(CYP27A1)to cleave a3-carbon unit and eventually convert-ed to CA.Without12α-hydroxylation,7α-hydroxy-4-choles-tene-3-one is converted to CDCA.Thus,CYP7A1controls the overall rate of bile acid production,while CYP8B1con-trols the CA:CDCA ratio in the bile acid pool.The alter-native pathway(also known as acidic pathway),which is thought to account for less than10%of the total bile acid synthesis in humans,mainly produces CDCA.CYP27A1 catalyzes hydroxylation of cholesterol to27-hydroxycholes-terol and then3β-dihydroxy-5-cholestionic acid[14].Oxys-terol7α-hydroxylase(CYP7B1)then catalyzes the hydroxy-lation reaction at C-7position of these two intermediates, which are subsequently converted to CDCA by the same enzymes in the classic pathway.3.Bile Acid Transport andEnterohepatic CirculationBile acids,once produced in the liver,are transported across the canalicular membrane of the hepatocytes into the bile and stored in the gallbladder.After each meal,gallbladder bile acids are released into the intestinal tract,efficiently reabsorbed in the ileum,and transported back to the liver via portal blood for reexcretion into the bile.This process is referred to as enterohepatic circulation of bile acids[10] (Figure1).Bile acid transporters play important roles in this transport process[15].The biliary excretion of bile acids is the major driving force of bileflow.The bile acid pool size is defined as the total amount of bile acids circulating in the enterohepatic circulation.In humans,bile acid pool consists of CA,CDCA,and DCA in an approximate40:40:20 ratio.In mice,the majority of the CDCA is converted into muricholic acids(MCAs),which are highly soluble and less toxic.Hepatocytes are polarized epithelial cells with basolateral (sinusoidal)and apical(canalicular)membrane domains. Hepatocytes take up bile acids through the basolateral membrane,which is in direct contact with the portal blood plasma,and excrete bile acid at the canalicular membrane into the bile[16].Since the biliary bile acids concentration is about100-to1000-fold higher in the bile than in the hepatocytes,canalicular bile acid transport represents the rate-limiting step in bile formation.Several members of the ATP-binding cassette(ABC)transporter family are responsi-ble for transporting bile acids and other organic compounds across the canalicular membrane against their concentration gradients.The bile salt export pump(BSEP,ABCB11),orig-inally identified as the sister of P-glycoprotein(SPGP),is mainly responsible for bile acid transport at the canalicular membrane[17](Figure1).Mutations in BSEP werefirst identified in patients with progressive familial intrahepatic cholestasis subtype2(PFIC-2).The absence of functional BSEP in the hepatic canalicular membrane and less than1% of normal biliary bile acid concentration found in these pa-tients suggested that BSEP is the major canalicular bile acid transport system[18].Phospholipids are excreted via the phospholipidflippase MDR2(ABCB4)(Figure1),and the major phospholipid in the bile is phosphatidylcholine[19, 20].Biliary-free cholesterol secretion mediated byABCG5/Figure1:Enterohepatic circulation of the bile.Bile acids are synthe-sized from cholesterol in the hepatocytes.CYP7A1regulates the rate-limiting step in the classic bile acid biosynthetic pathway.Bile acids are secreted into the gallbladder via BSEP.Phospholipids are transported via MDR2,and cholesterol is transported by the ABCG5/G8transporters into the bile.In the gallbladder,bile acids, phospholipids,and cholesterol form mixed micelles to solubilize cholesterol and to reduce bile acid toxicity.After meal intake,gall-bladder releases bile into the small intestine where bile acids facil-itate the absorption of dietary lipids and vitamins.At the terminal ileum,most of the bile acids are reabsorbed by ASBT into the en-terocytes,and secreted into the portal circulation via basolateral bile acid transporters Ostα/Ostβ.At the basolateral membrane of the hepatocytes,bile acids are taken up by the NTCP transporter for resecretion into the gallbladder.G8transporters is an important route for hepatic cholesterol elimination.Bile acids,phospholipids,and cholesterol are three major organic solutes of the bile and once secreted, they form mixed micelles to increase cholesterol solubility and reduce their toxicity to the bile duct.Bile acids are conju-gated with taurine or glycine in the peroxisomes and present as bile salts.They cannot cross the hepatocyte membrane and need active transport mechanisms for cellular uptake[21]. Two bile acid transporters,Na+-dependent taurocholate transporter(NTCP)(Figure1)and organic anion trans-porters(OATPs)are responsible for basolateral bile acid transport into the hepatocytes.In the intestine,bile salts are deconjugated,and bacterial 7α-dehydroxylase removed a hydroxy group from C-7and converts CA to DCA and CDCA to LCA.These secondary bile acids are highly toxic.In the intestine lumen,bile acids are reabsorbed mostly at the terminal ileum.Like the hepatic basolateral uptake system,intestinal bile acid uptake is also mainly mediated by the apical sodium-dependent bile salt transporter(ASBT)(Figure1)[22].Once absorbed into the enterocytes,bile acids bind the intestinal bile acid binding protein(I-BABP)and are transported to the basolateral membrane for secretion[23].Recently identified heterodimeric organic solute transporters OSTαand OSTβappeared to be the major basolateral bile acid transport system in the intestine and many other epithelial cells (Figure1)[24].This is supported by studies showing that over-ex-pression of OSTαand OSTβin mice enhanced baso-lateral efflux of taurocholate,while mice lacking ostαshowed marked decreases in intestinal bile acid absorption,serum bile acid concentration,and bile acid pool size.4.Regulation of Bile Acid Synthesis4.1.Bile Acid Feedback Regulation of Its Own Synthesis.It is clear that bile acid synthesis is mainly controlled via the transcriptional regulation of the CYP7A1gene[11].It is well established that the CYP7A1gene is repressed by bile acids.This bile acid feedback repression mechanism allows the liver to efficiently increase or decrease bile acid synthesis in response to changes in bile acid levels and thus to maintain a constant bile acid pool.It is thought that bile acid feedback inhibition of CYP7A1is mediated by the bile acid-activated nuclear receptor farnesoid X receptor(FXR) in the hepatocytes and the enterocytes(Table1).It was first discovered that hepatic FXR induced a negative nuclear receptor small heterodimer partner(SHP),which interacts with and represses the transcriptional activator liver-related homologue-1(LRH-1)that binds to the CYP7A1gene pro-moter and thus inhibit CYP7A1transcription[25].However, the repression of CYP7A1by bile acids and FXR agonist in SHP-deficient mice implies that FXR-SHP-LRH-1cascade is not the only pathway mediating bile acid feedback inhibition of CYP7A1and redundant pathways also exist[26,27]. More recently,FXR was shown to induce intestinefibroblast growth factor15(FGF15)which may act as an endocrine hormone to repress CYP7A1gene transcription via ERK sig-naling activation[28].Direct infusion of recombinant FGF15 into mouse blood circulation or overexpression of FGF15 in mouse livers via an adenovirus expression vector caused marked repression of CYP7A1mRNA expression.The identification of an intestine-initiated endocrine mechanism in mediating bile acid feedback regulation is consistent with the fact of intestine being the major organ for bile ac-id reabsorption and retention.Suchfinding also provides an explanation to a long observed phenomenon that intraduo-denal,but not intravenous,infusion of taurocholic acid repressed CYP7A1mRNA expression in rats[29].In mice lacking functional Ostα/Ostβ,bile acid transport to the liver was reduced and bile acids accumulated in the intestine. Interestingly,these mice showed increased intestine FGF15 expression and reduced liver CYP7A1mRNA and total bile acid pool[30].Furthermore,intestine-specific FXR knockout,but not liver-specific FXR knockout,prevented GW4064repression of liver CYP7A1gene expression in mice[31].These studies collectively suggest that intestine FXR plays a dominant role in mediating bile acid feedback repression of bile acid synthesis.Unfortunately,data on detection of the presence of FGF15protein in the mouse circulation is lacking,and such evidence is needed in order to eventually establish the endocrine mechanism of feedback regulation of bile acid synthesis.Human FGF19shares∼51% amino acid sequence identity with mouse FGF15and is considered as the mouse FGF15orthologue.FGF19has been shown to repress CYP7A1in human hepatocytes[32].In contrast to FGF15that is not detectable in mouse livers and circulation,FGF19mRNA is detectable in human livers and human hepatocytes[32,33].FGF19protein is present in human circulation[34].In human hepatocytes,FGF19is highly inducible by bile acids or FXR agonists[32].Since adenovirus-mediated overexpression of FGF15in mouse liver has been shown to repress CYP7A1,it is likely that bile acid accumulation in human liver may induce FXR/FGF19 pathway to repress CYP7A1in an autocrine manner[32]. Previous studies from us and others showed that bile acids were able to activate FXR-independent cell signaling path-ways to repress the CYP7A1gene[35,36].We recently showed that feeding FXR knockout mice a cholic acid-con-taining diet still repressed CYP7A1despite the absence of FGF15or SHP induction[6].These results indicate that in response to super-physiological concentrations of bile acids,redundant pathways are stimulated to repress bile acid synthesis.These pathways ensure prompt repression of de novo bile acid synthesis in response to elevated bile acid levels in the liver and/or the intestine.4.2.Nutrient Effects on Hepatic Bile Acid Synthesis.Despite the majority of studies being focused on the regulatory roles of bile acids in nutrient metabolism,there is also evidence that nutrition could directly regulate bile acid synthesis.In humans,CYP7A1activity,as determined by a serum surro-gate7alpha-hydroxycholest-4-en-3-one(C4),increases dur-ing postprandial periods during the day and decreases during fasting and at night[37].A metabolomic study also identified bile acids as the most markedly elevated metabolites in hu-man sera after an oral glucose challenge in patients with nor-mal glucose tolerance,but this response was blunted in patients with impaired glucose tolerance[38].Since post-prandial period is the highly active in metabolism and hu-mans undergo fasting-to-feeding cycles a few times a day, these observations could indicate an important link between bile acid synthesis and postprandial nutrient absorption and metabolism.Nutrient-activated signaling such as those by glucose or insulin is by far the most important signaling that regulates postprandial ing primary human hepatocytes,we have demonstrated that insulin rapidly in-duced while glucagon repressed CYP7A1mRNA[39,40].We also reported that glucose induced CYP7A1gene transcrip-tion via inducing histone hyperacetylation in CYP7A1gene chromatin[41].Although our in vitro study provide mech-anistic support for human observations,studies using in vivo mouse models yielded controversial results.It has been shown that PGC-1αacts as a coactivator of HNF4αand induces CYP7A1during fasting in mice[42].Furthermore, CYP7A1mRNA was induced in STZ-treated type-I diabetic rats lacking insulin secretion[43],which has led to the speculation that insulin may repress CYP7A1gene in rats.On the other hand,more recent studies seem to be contradictory to these early observations.First,mouse CYP7A1mRNA ex-pression peaked during the early dark cycle when food in-take was the most active[44,45].Furthermore,restricted feeding during light cycles shifted the peak of CYP7A1Table1:FXR target genes and their function and lipid and glucose metabolism. Gene Tissue Regulation FunctionBile acid metabolismCYP7A1liver down Encodes the rate-limiting enzyme in classic bile acid synthetic pathway BSEP liver up Rate-limiting step in canalicular bile acid transport into the gallbladder NTCP liver down Basolateral bile acid uptake into the hepatocytesOSTα/βintestine up Enterocyte basolateral bile acid secretion into the portal bloodI-BABP intestine up Intracellular bile acid transportFGF15/19intestine up Bile acid synthesis inhibitionSHP liver up Bile acid synthesis inhibitionGlucose metabolismPEPCK liver up GluconeogenesisFGF15/19intestine up Stimulates glycogen synthesis,repress gluconeogenesis Insulin pancreas up Glucose metabolismCholesterol metabolismApoA1liver down HDL metabolismLDLR liver down LDL uptakeABCG5/G8liver up Biliary-free cholesterol secretion SRB1liver up Hepatic HDL uptake,biliary cholesterol secretion PCSK9liver down Induces LDL receptor degradationFatty acid metabolism ApoC II liver up LPL activator ApoC III liver down LPL inhibitor SREBP1liver down LipogenesismRNA expression from dark cycles to light cycles[44].Such evidence seems to imply that liver bile acid synthesis and liver metabolism are coordinately controlled.Further studies are necessary to determine if nutritional regulation of bile acid synthesis may play a role in metabolic homeostasis during fasting to refeeding cycles.5.Bile Acid Regulation of Glucose Metabolism 5.1.FXR and Glucose Metabolism.Diabetes is associated with impaired peripheral glucose clearance and increased hepatic glucose production during fasting,which lead to postpran-dial and fasting hyperglycemia.Initial evidence that bile acids may regulate glucose metabolism came from studies show-ing that FXR agonist induced phosphoenolpyruvate Carbox-ykinase(PEPCK)mRNA expression(Table1)and glucose output in human and rat hepatocytes[46].Treating mice with an FXR agonist also induced hepatic PEPCK mRNA ex-pression in mice in vivo[46].A FXR binding site has been identified in the promoter of PEPCK gene.In contrast, later studies carried out in fxr knockout mice revealed that FXR-deficient mice had insulin resistance and hyperglycemia phenotypes.Administration of a FXR agonist GW4064de-creased serum glucose,increased liver glycogen,and im-proved insulin sensitivity in diabetic db/db mice[7,8].A number of recent studies showed that bile acids and FXR repressed hepatic PEPCK and G6Pase gene expression and thus liver gluconeogenesis.In this case,it is shown that bile acids may induce the repressor SHP,which inhibits PEPCK via inhibiting C/EBP[47],FoxO1[48],and Glucocorticoid receptor[49].Although these liver effects of FXR activation may prevent fasting hyperglycemia,it does not sufficiently explain the increased insulin sensitivity and glucose disposal in FXR agonist-treated mice as determined by glucose and insulin tolerance tests.In a similar study,Cariou et ed hyperinsulinemic euglycemic clamp and demonstrated that FXR-deficiency is associated with decreased whole-body glu-cose disposal,suggesting a role of FXR in regulating periph-eral glucose metabolism[50].FXR is not expressed in muscle but is expressed in white adipose at a very low level.It is noticed that fxr−/−mice had smaller adipocytes,and FXR agonist GW4064treatment increased adipose differentiation and insulin-dependent glucose uptake in3T3-L1cells in vitro.Another study suggests that FXR agonist INT747in-duced adipose differentiation via inducing the expression of adipocyte-related genes including C/EBPαand PPARγ[51].In addition to a role of FXR in adipose,two recent studies provided additional mechanism by which FXR may regulate peripheral glucose homeostasis.These studies re-vealed that FXR is also expressed in pancreaticβcells and positively regulates glucose-dependent insulin secretion [52].It is suggested that FXR activation stimulates insulin gene transcription.On the other hand,FXR activation is associated with increased AKT phosphorylation and GLUT2 translocation to the cell membrane and thus enhances glu-cose uptake into pancreaticβcells and glucose-dependent insulin secretion.Intestine is another major FXR expressing tissue.A recent study showed that FGF15/19,expressed in the intestine and secreted into the blood circulation,acts as a postprandial factor that promotes glycogen synthesis,which may be an important mechanism controlling postprandial glucose metabolism[53].It has been shown that serum FGF19increases during postprandial period in humans,pre-sumably due to increased bile acid signaling[34].Therefore, identification of the regulatory role of FGF15/19in post-prandial glycogen synthesis provides a novel link between bile acid signaling and glucose metabolism.In addition to the nuclear receptor FXR-mediated effects,bile acids have been shown to directly activate hepatic AKT via a Gαi proteincoupled receptor signaling pathway,which stimulated hepat-ic glycogen synthesis[54].Recently,it was further demon-strated that bile acid activation of the Gαi-AKT signaling cascade was involved in the bile acid induction of FXR and SHP and downregulation of gluconeogenic gene expressions in the liver[55].In summary,these studies suggest that bile acid regulation of hepatic glucose metabolism involves com-plex crosstalk between FXR-dependent pathways and FXR-independent signaling pathways.5.2.TGR5and Glucose Metabolism.Bile acids also activatea cell surface G-protein coupled receptor TGR5,which is mainly expressed in the intestine,brown adipose,white adi-pose,and gallbladder.Low levels of TGR5expression has also been detected in liver and skeletal muscle.Upon activation, TGR5leads to intracellular cAMP production and PKA acti-vation.Based on the ability to induce cellular cAMP produc-tion,taurolithocholic acid(TLCA)and LCA show highest potency in activating TGR5with EC50of0.33and0.53μM, respectively,while DCA,CDCA,and CA(in rank order) activate TGR5at higher1–8μM concentrations[56].It is suggested that in brown adipocytes,bile acid activation of TGR5-cAMP-PKA cascade results in induction of down-stream deiodinase,fatty acid oxidation genes,and uncou-pling proteins,which increase energy expenditure and pro-mote weight loss[57].As increased free fatty acid release and cytokine production associated with obesity clearly con-tribute to the development of insulin resistance,bile acids/ TGR5regulation of weight loss certainly could play a role in regulating glucose homeostasis.However,studies in tgr5−/−mice showed that neither under chow condition nor under high fat diet feeding condition did tgr5−/−develop obesity or hyperglycemia[58].The high fat diet feeding effect on insulin sensitivity,as determined by insulin tolerance tests, also seemed to be gender-specific in tgr5−/−mice,with male showing impaired,but female showing improved insulin sensitivity[58].The most potent endogenous ligand for TGR5is TLCA.TLCA is highly toxic and once synthesized, is rapidly metabolized in the intestine and the liver.Under physiological conditions,liver efficiently extracts bile acids from the portal circulation,and bile acid concentration in the systemic circulation is very low.Because these primary and secondary bile acids activate TGR5at a higher EC50,it is possible that TGR5is not activated by physiological con-centration of circulating bile acids outside of the entero-hepatic system.Thus,opposing to the clear pharmacolog-ical benefits of TGR5activation,the physiological role of TGR5in mediating bile acid signaling control of metabolic homeostasis needs to be further investigated.In addition to brown adipose,intestine is another major TGR5expressing ing an enteroendocrine cell line STC-1,Katsuma et al.first demonstrated that bile acids stimulate glucagon like peptide-1(GLP-1)production via TGR5activation[59]. The pharmacological significance of this pathway was then demonstrated by a detailed study carried out by Thomas et al.[60].These authors showed that administration of a potent TGR5agonist INT777raised intracellular ATP/ADP ratio and calcium influx,which leads to enhanced GLP-1 secretion from the intestine.GLP-1is known to promote insulin secretion and thus regulate glucose homeostasis.Be-cause GLP-1mimetics and receptor agonists are currently under clinical development and have shown promise in im-proving glucose homeostasis in diabetes,bile acid-based TGR5agonists may be a potential therapeutic to stimulate GLP-1secretion in diabetic patients[61].In contrast to these studies,bile acid sequestrants,which remove bile acids from the body by binding to bile acids in the intestine and prevent bile acids from being reabsorbed, have been shown to improve insulin sensitivity and lower fasting glucose in both men and several different experimen-tal models[62].Two studies conducted in rats have so far suggested that bile acid sequestrants may improve insulin sensitivity by increasing GLP-1release[63,64].Although the molecular mechanism is still not clear,both studies suggested that such effect is likely bile acid receptor-independent.This is because both studies showed that administration of bile acid sequestrants significantly lowered serum bile acid levels, which was associated with decreased FXR activation in the liver and the intestine.Furthermore,it is shown that blocking intestine bile acid transport using SC-435,an apical sodium-dependent bile acid transport inhibitor,also lowered serum bile acid levels,but did not modulate insulin sensitivity or GLP-1secretion.Thus,it is likely that bile acid sequestrants exert its effect by directly modulating cellular signaling in the intestine rather than by altering circulating bile acid levels or modulating bile acid pool.6.Bile Acids and Lipid Metabolism6.1.Bile Acids and Cholesterol Metabolism.It has been known for a long time that preventing bile acid reabsorption in the intestine by bile acid sequestration increases hepatic CYP7A1 and bile acid synthesis[65].The resulting increase in hepatic cholesterol catabolism caused compensatory induction of LDL receptor(LDLR)and LDL cholesterol(LDL-C)uptake. Because of the activation of this liver pathway,cholestyra-mine has been used to effectively lowering serum cholesterol in human patients.Paradoxically,activation of FXR by its potent agonists,which repress hepatic bile acid synthesis, also decreased serum cholesterol in animal models[7].In wild-type mice,activation of FXR is mainly associated with a reduction of HDL-C,while in hypercholesterolemic animal models,activation of FXR decreases both LDL-C and HDL-C.In vitro,FXR was shown to induce LDLR expression and repress PCSK9,an LDLR inhibitor[66].However, activation of FXR still significantly decreased serum non-HDL cholesterol in ldlr−/−mice[67].Furthermore,CDCA administration has been shown to raise serum LDL-C levels in humans.It remains to be determined whether activation of FXR will provide benefits in lowering LDL-C in men.FXR agonists have been shown to prevent atherosclerosis in various experimental models[68].Serum HDL transports cholesterol from peripheral tissues to the liver for elimination and thus plays a critical role in reverse cholesterol transport and the development of atherosclerosis.However,the role of FXR in regulating HDL metabolism is still under debate because FXR inhibits the hepatic production of apolipopro-tein A1(ApoAI),a key structural component of HDL,andactivation of FXR is associated with decreased serum HDL [69].Nevertheless,a recent study showed that activation of FXR promotes reverse cholesterol transport in mice by inducing hepatic expression of scavenger receptor B1(SR-B1)[70],which is suggested to play a role in both hepatic uptake of HDL-C and biliary secretion of free cholesterol [71].A FXR binding site has been identified in the SR-B1 gene promoter[72].In a recent study,we demonstrated that stimulating de novo bile acid synthesis by transgenic expression of a CYP7A1gene in mouse liver prevented diet-induced hypercholesterolemia[6].Different from CA feed-ing or FXR agonist administration,Cyp7a1-tg mice showed both increased hepatic cholesterol catabolism and bile acid ing this model,we demonstrated that bile acid activation of FXR induces hepatic expression of ABCG5and ABCG8through a common FXRE,which promoted bili-ary-free cholesterol secretion and fecal cholesterol loss.It is well known that cholesterol activation of LXR only induces mouse,but not human CYP7A1gene expression[73].We also showed that cholesterol/LXR signaling only induced ABCG5and ABCG8in mice,but not in primary human hepatocytes[6].These studies suggest that upon hepatic cho-lesterol accumulation,LXR may stimulate cholesterol catab-olism or biliary cholesterol secretion in mouse livers,but not human livers.Thus,it is possible that bile acid/FXR/ABCG5/ G8pathway plays a more important role in maintaining hepatic cholesterol homeostasis in response to increased cho-lesterol levels in humans.6.2.Bile Acids and Fatty Acid Metabolism.It has been known for a long time that serum bile acid and serum triglycer-ides are inversely correlated,suggesting that bile acid nega-tively regulates serum triglycerides[74,75].Current studies suggest that bile acids may lower serum triglycerides by re-pressing both hepatic triglyceride production/secretion and stimulating serum triglyceride clearance.In the liver,it is shown that bile acid activation of FXR repressed LXR-induction of SREBP-1and its target genes in de novo lipo-genesis by inducing the repressor SHP,which not only de-creased hepatic fat accumulation,but also led to reduced he-patic VLDL secretion[76].One study showing both LXR-dependent and FXR-dependent induction of hepatic lipoge-nesis by bile acid sequestrants administration supported this conclusion[68].Thefinding that FXR represses microsomal triglyceride transfer protein(MTP)and thus hepatic VLDL secretion seems to provide additional support that the FXR/SHP pathway reduces hepatic triglyceride output[77]. Genetic knockout of shp in ob/ob mice increased MTP and VLDL secretion[78].Diabetes and obesity are associated with increased hepatic VLDL output.Both increased fatty acid supply to the liver and hepatic insulin resistance may be involved.On the other hand,there are also studies showing that hepatic VLDL secretion was impaired in diabetic mouse models despite increased triglyceride output[79].Further-more,increased VLDL secretion in shp knockout mice seemed to be beneficial in reducing hepatic fat accumulation in ob/ob mice[78].Thus,the bile acid regulation of hepatic VLDL secretion and its in vivo significance seem to be com-plex and may depend on the experimental conditions.Serum triglyceride is cleared after VLDL-triglyceride is hydrolyzed by lipoprotein lipase(LPL)and subsequently taken up by the peripheral tissues.It has been reported that obesity and diabetes are also associated with impaired peripheral triglyceride clearance,contributing to diabetic hypertriglyc-eridemia.Activation of FXR has been shown to induce apolipoprotein CII(ApoCII),which is an LPL activator, and repress apolipoprotein CIII(ApoCIII),which is an LPL inhibitor in the liver[80,81].Increasing ApoCII or decreas-ing ApoCIII stimulates LPL to hydrolyze triglycerides carried by VLDL,thus accelerates serum VLDL clearance upon FXR activation.7.ConclusionExtensive investigations conducted in the past decade have shown that bile acids are important regulators of glucose and lipid metabolism.The identification of bile acid-activated nuclear receptor FXR and cell surface G protein coupled receptor TGR5has significantly advanced our understanding on how bile acid signaling regulates cellular metabolism in physiological and diseased conditions.The identification of these regulatory mechanisms also provided molecular basis for developing bile acid receptor agonists and receptor antagonists for treating human metabolic diseases.On the other hand,conflicting studies in thefield are present,which not only reflects the complex nature of the bile acid signaling in regulation of whole body metabolism,but also implies the difference between physiological role and pharmacological role of bile acid signaling in metabolic control.Furthermore, studies that focus on the regulation of bile acid metabolism in diseased conditions,especially obesity and diabetes,are still insufficient.Future advances in thefield are needed to improve our understanding in the bile acid control of metabolism,which is also critical in developing better drug therapies for the treatment of metabolic disorders. AbbreviationsCA:Cholic acidCDCA:Chenodeoxycholic acidDCA:Deoxycholic acidLCA:Lithocholic acidCYP7A1:Cholesterol7α-hydroxylaseCYP8B1:Microsomal sterol12α-hydroxylaseCYP27A1:Mitochondrial27-hydroxylaseCYP7B1:Oxysterol7α-hydroxylaseMCA:Muricholic acidsABC:ATP-binding cassetteBSEP:Bile salt export pumpSPGP:Sister of P-glycoproteinPFIC:Progressive familial intrahepatic cholestasis MDR:Multidrug resistanceNTCP:Na+-dependent taurocholate transporter OATP:Organic anion transporterASBT:Apical sodium-dependent bile salt transporter I-BABP:Intestinal bile acid binding proteinOST:Organic solute transporterFXR:Farnesoid X receptor。
胆汁酸综述
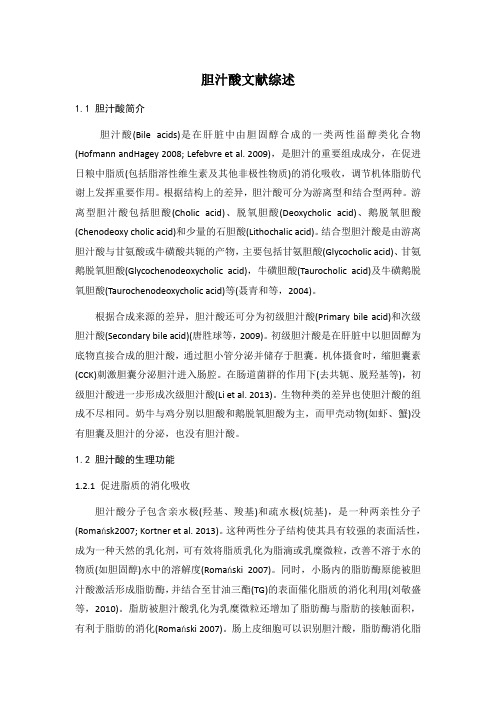
胆汁酸文献综述1.1 胆汁酸简介胆汁酸(Bile acids)是在肝脏中由胆固醇合成的一类两性甾醇类化合物(Hofmann andHagey 2008; Lefebvre et al. 2009),是胆汁的重要组成成分,在促进日粮中脂质(包括脂溶性维生素及其他非极性物质)的消化吸收,调节机体脂肪代谢上发挥重要作用。
根据结构上的差异,胆汁酸可分为游离型和结合型两种。
游离型胆汁酸包括胆酸(Cholic acid)、脱氧胆酸(Deoxycholic acid)、鹅脱氧胆酸(Chenodeoxy cholic acid)和少量的石胆酸(Lithochalic acid)。
结合型胆汁酸是由游离胆汁酸与甘氨酸或牛磺酸共轭的产物,主要包括甘氨胆酸(Glycocholic acid)、甘氨鹅脱氧胆酸(Glycochenodeoxycholic acid),牛磺胆酸(Taurocholic acid)及牛磺鹅脱氧胆酸(Taurochenodeoxycholic acid)等(聂青和等,2004)。
根据合成来源的差异,胆汁酸还可分为初级胆汁酸(Primary bile acid)和次级胆汁酸(Secondary bile acid)(唐胜球等,2009)。
初级胆汁酸是在肝脏中以胆固醇为底物直接合成的胆汁酸,通过胆小管分泌并储存于胆囊。
机体摄食时,缩胆囊素(CCK)刺激胆囊分泌胆汁进入肠腔。
在肠道菌群的作用下(去共轭、脱羟基等),初级胆汁酸进一步形成次级胆汁酸(Li et al. 2013)。
生物种类的差异也使胆汁酸的组成不尽相同。
奶牛与鸡分别以胆酸和鹅脱氧胆酸为主,而甲壳动物(如虾、蟹)没有胆囊及胆汁的分泌,也没有胆汁酸。
1.2 胆汁酸的生理功能1.2.1 促进脂质的消化吸收胆汁酸分子包含亲水极(羟基、羧基)和疏水极(烷基),是一种两亲性分子(Romańsk2007; Kortner et al. 2013)。
这种两性分子结构使其具有较强的表面活性,成为一种天然的乳化剂,可有效将脂质乳化为脂滴或乳糜微粒,改善不溶于水的物质(如胆固醇)水中的溶解度(Romański 2007)。
胆汁酸的合成调控及其在生理与病理中的功能机制

Hereditas (Beijing) 2019年5月, 41(5): 365―374 收稿日期: 2019-01-09; 修回日期: 2019-03-18基金项目:国家自然科学基金项目(编号:91754101)资助[Supported by the National Natural Science Foundation of China (No. 91754101)]作者简介: 刘笑,硕士研究生,专业方向:脂类代谢。
E-mail: 2016202040152@通讯作者:王琰,博士,教授,博士生导师,研究方向:脂类代谢。
E-mail: Wang.y@DOI: 10.16288/j.yczz.19-011网络出版时间: 2019/3/26 9:24:44URI: /kcms/detail/11.1913.R.20190326.0924.001.html综 述胆汁酸的合成调控及其在生理与病理中的功能机制刘笑,王琰武汉大学生命科学学院,细胞稳态湖北省重点实验室,武汉 430072摘要: 胆汁酸是一类胆固醇的代谢物,在机体胆固醇与能量代谢平衡和小肠营养物质吸收等方面起着重要作用。
肝脏是合成胆汁酸的主要场所。
饥饿条件下,胆汁酸从肝脏分泌进入胆管并被储存到胆囊;进食后胆囊收缩,贮存的胆汁酸被排出进入小肠。
在小肠中,95%的胆汁酸会被小肠重新吸收,通过肝门静脉返回肝脏,这一过程被称为胆汁酸的肝肠循环。
胆汁酸一方面作为乳化剂促进小肠中脂类等物质的吸收及转运,同时也作为重要的信号分子与多种受体结合,包括核受体法呢醇X 受体(farnesoid X receptor, FXR)、维生素D 受体(vitamin D receptor, VDR)、孕烷X 受体(pregnane X receptor, PXR)以及细胞膜表面受体G 蛋白偶联受体(cell membrane surface receptor-G protein coupled receptor, TGR5)等,在调节体内胆汁酸的代谢平衡、糖脂代谢与能量代谢平衡等方面发挥重要作用。
胆汁酸的代谢.ppt
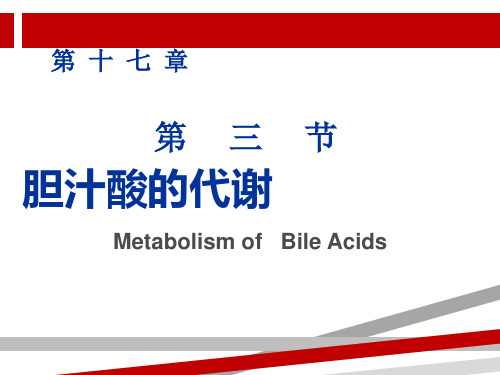
黄疸( jaundice)
概念
高胆红素血症 黄疸 隐形黄疸
种类(按血清胆红素的来源)
溶血性黄疸 (hemolytic jaundice) 肝细胞性黄疸(hepatocellular jaundice) 阻塞性黄疸(obstructive jaundice)
(一)溶血性黄疸
是由于红细胞在单核- 吞噬细胞系统破坏 过多,超过肝细胞的摄取转化和排泄能力,造 成血清游离胆红素浓度过高所致。
游离胆红素:未与葡萄糖醛酸结合的胆红素称为 游离胆红素,又称间接胆红素。
两种胆红素的区别
项目
别名
与葡萄糖醛酸结合 与重氮试剂反应 水中溶解度 经肾随尿排出 通透细胞膜对脑的 毒性作用
游离胆红素 间接胆红素,
血胆红素 未结合 慢或间接反应
小 不能
大
结合胆红素 直接胆红素,
肝胆红素 结合
迅速直接反应 大 能
*转运 在胞浆与配体蛋白结合
内质网
*转化
部位:滑面内质网 反应:结合反应(主要的结合物为UDP葡萄糖
醛酸,UDPGA) 酶:葡萄糖醛酸基转移酶 产物:主要为双葡萄糖醛酸胆红素,另有少
量单葡萄糖醛酸胆红素、硫酸胆红素,统称 为结合胆红素
葡糖醛酸胆红素的生成
胆红素
+ UDP -葡糖醛酸
胆红素葡糖醛酸一酯
➢ 过程
胆固醇(27C) 7α-羟化酶
7α-羟化胆固 醇
结合型初级胆汁酸 初级胆汁酸(24C)
2. 次级胆汁酸的生成与肠肝循环
﹡部位:小肠下段和大肠 ﹡过程
肠菌 初级胆汁酸 水解脱羟 次级胆汁酸
胆汁酸肠肝循环
➢胆汁酸肠肝循环概念 胆汁酸随胆汁排入肠腔后,通过重吸收
- 1、下载文档前请自行甄别文档内容的完整性,平台不提供额外的编辑、内容补充、找答案等附加服务。
- 2、"仅部分预览"的文档,不可在线预览部分如存在完整性等问题,可反馈申请退款(可完整预览的文档不适用该条件!)。
- 3、如文档侵犯您的权益,请联系客服反馈,我们会尽快为您处理(人工客服工作时间:9:00-18:30)。
关于胆汁酸与血糖、血脂及能量代谢关系的研究进展[摘要] 过去胆汁酸仅仅被作为肝脏中来源于胆固醇的两亲性分子,可促进胆固醇、脂溶性维生素和脂质的吸收。
近几十年的研究显示——胆汁酸涉及多种代谢过程(血糖代谢、血脂代谢、能量代谢),与这些过程中的某些基因表达及细胞信号通路的调节有关。
此外,血糖也可以调节胆汁酸的合成过程。
胆汁酸成为代谢综合征、胰岛素抵抗、肥胖的全新研究视角。
近年来,大量的研究显示胆汁酸除了参与食物来源脂质吸收及体内胆固醇的溶解,还作为信号分子在多种代谢过程(包括血糖、血脂及能量代谢)中发挥作用。
本文就胆汁酸的这些新作用及可能涉及的机制作一综述。
1.胆汁酸的概述胆汁酸是胆汁的主要成分,胆汁产生于肝脏而储存于胆囊,经释放进入小肠发挥作用。
作为两性分子,胆汁酸内既含有亲水性的羟基及羧基或磺酸基,又含有疏水性烃核和甲基。
胆汁酸具有较强的界面活性,能降低油水两相间的表面张力,促进脂类乳化;同时扩大脂肪和脂肪酶的接触面,加速脂类的消化。
胆汁酸按结构可以分为两类:一类为游离型胆汁酸,包括胆酸、脱氧胆酸、鹅脱氧胆酸和少量的石胆酸;另一类是上述游离胆汁酸与甘氨酸或牛磺酸结合的产物,称结合型胆汁酸。
从来源上分类可分为初级胆汁酸和次级胆汁酸。
肝细胞内,以胆固醇为原料直接合成的胆汁酸称为初级胆汁酸,包括胆酸和鹅脱氧胆酸。
胆固醇主要的排泄途径即合成胆汁酸,此反应的限速酶是7α-羟化酶(CYP7A1)。
初级胆汁酸在肠道中受细菌作用,进行7α脱羟作用生成的胆汁酸,称为次级胆汁酸,包括脱氧胆酸和石胆酸。
肠道中的各种胆汁酸平均有95%被肠壁重吸收,其余的随粪便排出。
由肠道重吸收的胆汁酸(包括初级和次级胆汁酸;结合型和游离型胆汁酸)均由门静脉进入肝脏,在肝脏中游离型胆汁酸再转变为结合型胆汁酸,再随胆汁排入肠腔。
此过程称为“胆汁酸的肠肝循环”,其生理意义在于使有限的胆汁酸重复利用,促进脂类的消化与吸收。
最近20年来,胆汁酸不断被人们重新认识,作为调节分子而活化肝脏和肠道中存在的核受体(FXR、PXR及VDR)、G蛋白偶联受体TGR5及细胞信号通路(JNK1/2、AKT、ERK1/2)。
这些核受体及信号通路的活化继而改变了许多调节过程(如胆汁酸、血糖、脂肪酸、脂蛋白的合成、代谢、转运过程及能量代谢)中涉及的酶和蛋白质编码基因的表达[1]。
2.胆汁酸与糖代谢作为糖异生和糖原合成的场所,肝脏在调节血糖水平上起重要作用。
PEPCK,即磷酸烯醇式丙酮酸羧激酶,G6Pase,即葡萄糖-6-磷酸酶,以及FBP1,即果糖-1,6-双磷酸酶均是糖异生过程中的关键酶。
糖原合成中的关键酶是糖原合酶,肝糖原的分解是补充血糖、维持血糖水平的一个途径。
2.1 胆汁酸-FXR信号通路与糖代谢FXR是配体活化的转录因子核受体超家族成分,在肝脏和胃肠道均高表达。
胆汁酸对FXR的活化可以上调SHP编码基因的表达,后者对许多转录因子,包括LRX、LRH-1及HNF-4α,起抑制作用。
Yamagata 等人曾报道过:胆汁酸以FXR-SHP依赖的方式抑制糖异生相关基因PEPCK,G6Pase,FBP1的转录[2]。
与上述体外研究结果一致,应用GW4064(一种FXR的激动剂)处理的糖尿病鼠PEPCK和G6Pase基因的表达有所下降。
2.2 胆汁酸-TGR5信号通路与胰岛素抵抗TGR5是G蛋白偶联受体的一种,为细胞膜受体。
很多种类型的胆汁酸可以活化TGR5,导致受体的内化、胞外信号的活化及有丝分裂原活化的蛋白激酶途径,导致胞内cAMP产物合成增加,蛋白激酶A的激活,从而调节细胞的物质代谢和基因表达[3]。
研究发现在体外用石胆酸和脱氧胆酸刺激鼠科动物的肠道细胞,这些细胞以tgr5依赖性的方式来分泌肠源性的肠促胰岛素GLP-1的分泌。
此外,TGR5转基因鼠模型TGR5的过量表达显著改善了高脂食物喂养后血糖耐受性,这可能是与糖负荷后GLP-1及胰岛素大量分泌相关的[4]。
2.3 胆汁酸与糖代谢的小结胆汁酸至少是通过两种机制改变肝细胞内的糖代谢。
其一,活化胰岛素信号通路,发挥类似胰岛素样的活化糖原合酶基因、抑制糖异生基因作用[1]。
胆汁酸种类不同,活化肝细胞内AKT(胰岛素信号通路)的机制也不同:结合胆汁酸主要是通过G蛋白偶联受体,而非结合胆汁酸是通过线粒体内产生的超氧化物离子[5]。
胆汁酸对AKT通路的活化已经在鼠的原代肝细胞中得到证实[5]。
AKT 通路的活化使胆汁酸以类似于胰岛素的方式调节肝脏内的糖代谢,餐后胆汁酸由胆囊释放出来这是一个生理机制,重新回到肝脏的胆汁酸在空腹-进餐的循环中增强了胰岛素信号,有利于血糖的调节。
G蛋白偶联受体活化肝细胞内的胰岛素信号通路可能会成为药物治疗2型糖尿病的新靶点。
其二:通过SHP介导的对转录因子HNF-4α及FOXO1的抑制调节糖异生基因的表达。
编码PEPCK和G6Pase的基因在转录水平受核转录因子FOXO1和HNF-4α的调控。
胆汁酸对SHP基因的活化是通过后者启动子序列中有功能的FXR位点实现的。
FXR的人工合成的配体GW4064处理的糖尿病动物的胰岛素抵抗和高血糖均得到改善[6]。
胆汁酸引起FXR的活化,这对血糖的调节是很重要的。
3. 胆汁酸与脂代谢众所周知,胆汁酸活化的FXR主要是通过影响CYP7A1的表达参与胆固醇代谢调节。
CYP7A1是胆固醇转化为胆汁酸一反应中的限速酶。
在肝内,FXR可诱导抑制性核受体SHP编码基因的表达,后者可结合并使另一核受体同时也是CYP7A1基因反式激活因子的LRH-1失活。
这一级联反应抑制CYP7A1基因的转录,也抑制了胆汁酸的产生(胆固醇的转化)[7]。
除了参与胆固醇的体内平衡,胆汁酸也参与甘油三酯的代谢。
甘油三酯的产生于清除之间的平衡决定了循环中甘油三酯的水平。
饮食来源的脂肪酸和甘油三酯的从头合成主要是在肝脏中进行。
肝脏中甘油三酯的量主要取决于脂肪酸的合成速率,后者很大程度受到转录水平上的PPARα(促进脂肪酸的β氧化)和SREBP-1C(控制脂肪酸的合成)的调节。
目前的研究结果显示:胆汁酸主要是通过FXR,SHP,LXR,SREBP-1C途径影响脂肪酸、甘油三酯和及低密度脂蛋白的合成[8]。
这一模型中,胆汁酸活化FXR, 诱导SHP的表达,后者与LXR相互作用,导致SREBP-1C编码基因的下调。
胆汁酸也可以通过加强低密度脂蛋白的清除与转运降低血浆中甘油三酯的水平。
最后,胆汁酸还可以诱导极低密度脂蛋白的摄取与代谢中所涉及的其他基因的产生。
4. 胆汁酸与能量代谢TGR5表达在棕色脂肪组织中,在能量消耗的调节中起重要作用。
Watanabe 等人首次证实了胆汁酸通过与TGR5结合减轻了高脂饮食鼠的肥胖与胰岛素抵抗,对正常体重和偏瘦的鼠没有产生影响。
胆汁酸的这一代谢效应主要是通过CAMP-PKA依赖的甲状腺激素激活酶D2的基因表达的增加实现的,因为以上效应在缺乏D2基因的鼠是不存在的[9]。
胆汁酸是以剂量依赖的方式增加CAMP的水平,餐后胆汁酸浓度增加,这对于刺激CAMP及D2的产生是必要的。
因此,胆汁酸可能是连接饮食及与其诱导的代谢率的提高的激素信号[10]。
胆汁酸能够组织选择性地增加T3的水平,继而增加能量的消耗这增加了我们对能量平衡及潜在的抑制肥胖的途径的认识。
显然,TGR5配体的发现将为生理学能量代谢的稳定、胰岛素抵抗的改善开辟新的道路。
当然,对于TGR5的生理学作用需要将来进一步的研究。
5. 血糖对CYP7A1基因转录(胆汁酸合成)的调节高血糖可以促进胆汁酸的合成,诱导CYP7A1 基因mRNA的表达。
这主要是通过两种机制。
其一,葡萄糖增加了ATP的量,减少AMP/ATP的值,进而抑制了AMPK的活性。
AMPK为丝氨酸/苏氨酸蛋白激酶,能感受细胞内的营养和能量状态。
在细胞内ATP大量消耗,即AMP/ATP值增加的情况下,AMPK被AMP激活,继而抑制了消耗能量的生物合成过程,如脂肪酸合成、胆固醇合成。
Li 等人已经证明在人类的肝细胞中AMPK抑制了CYP7A1基因的转录和胆汁酸的合成。
血糖对AMPK活性的抑制导致了HNF-4α蛋白的增加,继而诱导了CYP7A1基因的表达。
其二,血糖通过对CYP7A1 染色质进行后天性的乙酰化修饰而诱导CYP7A1的转录。
血糖的增加会同时伴有ATP-柠檬酸裂解酶的增加及乙酰辅酶A的增加,导致了CYP7A1启动子染色质区的组蛋白乙酰化为活性的乙酰化H3 及H4,同时减少了核染色质中H3K9的甲基化,而甲基化有抑制CYP7A1基因转录的作用。
因此糖代谢可能在CYP7A1表达的后天性调节中起主要作用,从而维持胆固醇和胆汁酸的体内平衡[11]。
6.总结综上所述,胆汁酸与糖代谢、脂代谢及能量代谢密切相关;同时血糖也会调节胆汁酸的代谢过程。
对胆汁酸与以上代谢过程中机制的研究,将为正常生理状态和糖尿病状态下物质代谢和能量调节提供新的途径。
参考文献1.Hylemon PB, Zhou H, Pandak WM, Ren S, Gil G, Dent P. Bile acids as regulatory molecules. J Lipid Res. 2009Aug; 50(8):1509-20.2. Yamagata K, Daitoku H, Shimamoto Y, Matsuzaki H, Hirota K, Ishida J,et al. Bile acids regulate gluconeogenic geneexpression via small heterodimer partner-mediated repression of hepatocyte nuclear factor 4 and Foxo1. J Biol Chem.2004 May 28; 279(22):23158-65.3. Staels B, Handelsman Y, Fonseca V. Bile acid sequestrants for lipid and glucose control. Curr Diab Rep. 2011 Feb;11(1):70-7.4. Thomas C, Gioiello A, Noriega L, Strehle A, Oury J, Rizzo G, et al. TGR5-mediated bile acid sensing controlsglucose homeostasis. Cell Metab. 2009 Sep; 10(3):167-77.5. Dent P, Fang Y, Gupta S, Studer E, Mitchell C, Spiegel S, et al. Conjugated bile acids promote ERK1/2 and AKTactivation via a pertussis toxin-sensitive mechanism in murine and human hepatocytes. Hepatology. 2005 Dec;42(6):1291-9.6. Zhang Y, Lee FY, Barrera G, Lee H, Vales C, Gonzalez FJ, et al. Activation of the nuclear receptor FXR improveshyperglycemia and hyperlipidemia in diabetic mice. Proc Natl Acad Sci U S A. 2006 Jan 24;103(4):1006-11.7. Goodwin B, Jones SA, Price RR, Watson MA, McKee DD, Moore LB, et al. A regulatory cascade of the nuclearreceptors FXR, SHP-1, and LRH-1 represses bile acid biosynthesis. Mol Cell. 2000 Sep;6(3):517-26.8. Watanabe M, Houten SM, Wang L, Moschetta A, Mangelsdorf DJ, Heyman RA, et al. Bile acids lower triglyceridelevels via a pathway involving FXR, SHP, and SREBP-1c. J Clin Invest. 2004 May;113(10):1408-18.9. Watanabe M, Houten SM, Mataki C, Christoffolete MA, Kim BW, Sato H, et al. Bile acids induce energyexpenditure by promoting intracellular thyroid hormone activation. Nature. 2006 Jan 26;439(7075):484-9.10. Baxter JD, Webb P. Metabolism: bile acids heat things up. Nature. 2006 Jan 26;439(7075):402-3.11. Li T, Chanda D, Zhang Y, Choi HS, Chiang JY. Glucose stimulates cholesterol 7alpha-hydroxylase genetranscription in human hepatocytes. J Lipid Res. 2010 Apr;51(4):832-42.。