High Gain Free Electron Lasers Driven by Flat Electron Beam
光电技术专业英语词汇

《光电技术》专业英语词汇1.Absorption coefficient 吸收系数2.Acceptance angle 接收角3.fibers 光纤4.Acceptors in semiconductors 半导体接收器5.Acousto-optic modulator 声光调制6.Bragg diffraction 布拉格衍射7.Air disk 艾里斑8.angular radius 角半径9.Airy rings 艾里环10.anisotropy 各向异性11.optical 光学的12.refractive index 各向异性13.Antireflection coating 抗反膜14.Argon-ion laser 氩离子激光器15.Attenuation coefficient 衰减系数16.Avalanche 雪崩17.breakdown voltage 击穿电压18.multiplication factor 倍增因子19.noise 燥声20.Avalanche photodiode(APD) 雪崩二极管21.absorption region in APD APD 吸收区域22.characteristics-table 特性表格23.guard ring 保护环24.internal gain 内增益25.noise 噪声26.photogeneration 光子再生27.primary photocurrent 起始光电流28.principle 原理29.responsivity of InGaAs InGaAs 响应度30.separate absorption and multiplication(SAM) 分离吸收和倍增31.separate absorption grading and multiplication(SAGM) 分离吸收等级和倍增32.silicon 硅33.Average irradiance 平均照度34.Bandgap 带隙35.energy gap 能级带隙36.bandgap diagram 带隙图37.Bandwidth 带宽38.Beam 光束39.Beam splitter cube立方分束器40.Biaxial crystals 双轴晶体41.Birefringent 双折射42.Bit rate 位率43.Black body radiation law 黑体辐射法则44.Bloch wave in a crystal 晶体中布洛赫波45.Boundary conditions 边界条件46.Bragg angle 布拉格角度47.Bragg diffraction condition 布拉格衍射条件48.Bragg wavelength 布拉格波长49.Brewster angle 布鲁斯特角50.Brewster window 布鲁斯特窗51.Calcite霰石52.Carrier confinement 载流子限制53.Centrosymmetric crystals 中心对称晶体54.Chirping 啁啾55.Cladding覆层56.Coefficient of index grating 指数光栅系数57.Coherence 连贯性pensation doping 掺杂补偿59.Conduction band 导带60.Conductivity 导电性61.Confining layers 限制层62.Conjugate image 共轭像63.Cut-off wavelength 截止波长64.Degenerate semiconductor简并半导体65.Density of states 态密度66.Depletion layer 耗尽层67.Detectivity 探测率68.Dielectric mirrors 介电质镜像69.Diffraction 衍射70.Diffraction grating 衍射光栅71.Diffraction grating equation 衍射光栅等式72.Diffusion current 扩散电流73.Diffusion flux 扩散流量74.Diffusion Length 扩散长度75.Diode equation 二极管公式76.Diode ideality factor 二极管理想因子77.Direct recombination 直接复合78.Dispersion 散射79.Dispersive medium 散射介质80.Distributed Bragg reflector 分布布拉格反射器81.Donors in semiconductors 施主离子82.Doppler broadened linewidth 多普勒扩展线宽83.Doppler effect 多普勒效应84.Doppler shift 多普勒位移85.Doppler-heterostructure 多普勒同质结构86.Drift mobility漂移迁移率87.Drift Velocity 漂移速度88.Effective density of states 有效态密度89.Effective mass 有效质量90.Efficiency 效率91.Einstein coefficients 爱因斯坦系数92.Electrical bandwidth of fibers 光纤电子带宽93.Electromagnetic wave 电磁波94.Electron affinity 电子亲和势95.Electron potential energy in a crystal 晶体电子阱能量96.Electro-optic effects 光电子效应97.Energy band 能量带宽98.Energy band diagram 能量带宽图99.Energy level 能级100.Epitaxial growth外延生长101.Erbium doped fiber amplifier 掺饵光纤放大器102.Excess carrier distribution 过剩载流子扩散103.External photocurrent 外部光电流104.Extrinsic semiconductors 本征半导体105.Fabry-Perot laser amplifier 法布里-珀罗激光放大器106.Fabry-Perot optical resonator 法布里-珀罗光谐振器107.Faraday effect 法拉第效应108.Fermi-Dirac function 费米狄拉克结109.Fermi energy 费米能级110.Fill factor 填充因子111.Free spectral range 自由谱范围112.Fresnel’s equations菲涅耳方程113.Fresnel’s optical indicatrix 菲涅耳椭圆球114.Full width at half maximum半峰宽115.Full width at half power半功率带宽116.Gaussian beam 高斯光束117.Gaussian dispersion 高斯散射118.Gaussian pulse 高斯脉冲119.Glass perform玻璃预制棒120.Goos Haenchen phase shift Goos Haenchen相位移121.Graded index rod lens 梯度折射率棒透镜122.Group delay 群延迟123.Group velocity 群参数124.Half-wave plate retarder 半波延迟器125.Helium-Neon laser氦氖激光器126.Heterojunction 异质结127.Heterostructure 异质结构128.Hole 空穴129.Hologram 全息图130.Holography 全息照相131.Homojunction 同质结132.Huygens-Fresnel principle惠更斯-菲涅耳原理133.Impact-ionization 碰撞电离134.Index matching 指数匹配135.Injection 注射136.Instantaneous irradiance 自发辐射137.Integrated optics 集成光路138.Intensity of light 光强139.Intersymbol interference 符号间干扰140.Intrinsic concentration本征浓度141.Intrinsic semiconductors 本征半导体142.Irradiance 辐射SER 激光144.active medium 活动介质145.active region 活动区域146.amplifiers 放大器147.cleaved-coupled-cavity解理耦合腔148.distributed Bragg reflection 分布布拉格反射149.distributed feedback 分布反馈150.efficiency of the He-Ne 氦氖效率151.multiple quantum well 多量子阱152.oscillation condition 振荡条件ser diode 激光二极管sing emission 激光发射155.LED 发光二极管156.Lineshape function 线形结157.Linewidth 线宽158.Lithium niobate铌酸锂159.Load line 负载线160.Loss coefficient 损耗系数161.Mazh-Zehnder modulator Mazh-Zehnder型调制器162.Macrobending loss 宏弯损耗163.Magneto-optic effects 磁光效应164.Magneto-optic isolator 磁光隔离165.Magneto-optic modulator 磁光调制166.Majority carriers 多数载流子167.Matrix emitter 矩阵发射168.Maximum acceptance angle 最优接收角169.Maxwell’s wave equation 麦克斯维方程170.Microbending loss 微弯损耗171.Microlaser 微型激光172.Minority carriers 少数载流子173.Modulated directional coupler 调制定向偶合器174.Modulation of light 光调制175.Monochromatic wave 单色光176.Multiplication region 倍增区177.Negative absolute temperature 负温度系数 round-trip optical gain 环路净光增益179.Noise 噪声180.Noncentrosymmetric crystals 非中心对称晶体181.Nondegenerate semiconductors 非简并半异体182.Non-linear optic 非线性光学183.Non-thermal equilibrium 非热平衡184.Normalized frequency 归一化频率185.Normalized index difference 归一化指数差异186.Normalized propagation constant 归一化传播常数187.Normalized thickness 归一化厚度188.Numerical aperture 孔径189.Optic axis 光轴190.Optical activity 光活性191.Optical anisotropy 光各向异性192.Optical bandwidth 光带宽193.Optical cavity 光腔194.Optical divergence 光发散195.Optic fibers 光纤196.Optical fiber amplifier 光纤放大器197.Optical field 光场198.Optical gain 光增益199.Optical indicatrix 光随圆球200.Optical isolater 光隔离器201.Optical Laser amplifiers 激光放大器202.Optical modulators 光调制器203.Optical pumping 光泵浦204.Optical resonator 光谐振器205.Optical tunneling光学通道206.Optical isotropic光学各向同性的207.Outside vapor deposition管外气相淀积208.Penetration depth 渗透深度209.Phase change 相位改变210.Phase condition in lasers 激光相条件211.Phase matching 相位匹配212.Phase matching angle 相位匹配角213.Phase mismatch 相位失配214.Phase modulation 相位调制215.Phase modulator 相位调制器216.Phase of a wave 波相217.Phase velocity 相速218.Phonon 光子219.Photoconductive detector 光导探测器220.Photoconductive gain 光导增益221.Photoconductivity 光导性222.Photocurrent 光电流223.Photodetector 光探测器224.Photodiode 光电二极管225.Photoelastic effect 光弹效应226.Photogeneration 光子再生227.Photon amplification 光子放大228.Photon confinement 光子限制229.Photortansistor 光电三极管230.Photovoltaic devices 光伏器件231.Piezoelectric effect 压电效应232.Planck’s radiation distribution law 普朗克辐射法则233.Pockels cell modulator 普克尔斯调制器234.Pockel coefficients 普克尔斯系数235.Pockels phase modulator 普克尔斯相位调制器236.Polarization 极化237.Polarization transmission matrix 极化传输矩阵238.Population inversion 粒子数反转239.Poynting vector能流密度向量240.Preform 预制棒241.Propagation constant 传播常数242.Pumping 泵浦243.Pyroelectric detectors 热释电探测器244.Quantum efficiency 量子效应245.Quantum noise 量子噪声246.Quantum well 量子阱247.Quarter-wave plate retarder 四分之一波长延迟248.Radiant sensitivity 辐射敏感性249.Ramo’s theorem拉莫定理250.Rate equations速率方程251.Rayleigh criterion 瑞利条件252.Rayleigh scattering limit 瑞利散射极限253.Real image 实像254.Recombination 复合255.Recombination lifetime 复合寿命256.Reflectance 反射257.Reflection 反射258.Refracted light 折射光259.Refractive index 折射系数260.Resolving power分辩力261.Response time 响应时间262.Return-to-zero data rate 归零码263.Rise time 上升时间264.Saturation drift velocity 饱和漂移速度265.Scattering 散射266.Second harmonic generation 二阶谐波267.Self-phase modulation 自相位调制268.Sellmeier dispersion equation色列米尔波散方程式269.Shockley equation肖克利公式270.Shot noise肖特基噪声271.Signal to noise ratio 信噪比272.Single frequency lasers 单波长噪声273.Single quantum well 单量子阱274.Snell’s law斯涅尔定律275.Solar cell 光电池276.Solid state photomultiplier 固态光复用器277.Spectral intensity 谱强度278.Spectral responsivity 光谱响应279.Spontaneous emission 自发辐射280.stimulated emission 受激辐射281.Terrestrial light 陆地光282.Theraml equilibrium热平衡283.Thermal generation 热再生284.Thermal velocity 热速度285.Thershold concentration 光强阈值286.Threshold current 阈值电流287.Threshold wavelength 阈值波长288.Total acceptance angle 全接受角289.Totla internal reflection 全反射290.Transfer distance 转移距离291.Transit time 渡越时间292.Transmission coefficient 传输系数293.Tramsmittance 传输294.Transverse electric field 电横波场295.Tranverse magnetic field 磁横波场296.Traveling vave lase 行波激光器297.Uniaxial crystals 单轴晶体298.UnPolarized light 非极化光299.Wave 波300.Wave equation 波公式301.Wavefront 波前302.Waveguide 波导303.Wave number 波数304.Wave packet 波包络305.Wavevector 波矢量306.Dark current 暗电流307.Saturation signal 饱和信号量308.Fringing field drift 边缘电场漂移plementary color 补色310.Image lag 残像311.Charge handling capability 操作电荷量312.Luminous quantity 测光量313.Pixel signal interpolating 插值处理314.Field integration 场读出方式315.Vertical CCD 垂直CCD316.Vertical overflow drain 垂直溢出漏极317.Conduction band 导带318.Charge coupled device 电荷耦合组件319.Electronic shutter 电子快门320.Dynamic range 动态范围321.Temporal resolution 动态分辨率322.Majority carrier 多数载流子323.Amorphous silicon photoconversion layer 非晶硅存储型324.Floating diffusion amplifier 浮置扩散放大器325.Floating gate amplifier 浮置栅极放大器326.Radiant quantity 辐射剂量327.Blooming 高光溢出328.High frame rate readout mode 高速读出模式329.Interlace scan 隔行扫描330.Fixed pattern noise 固定图形噪声331.Photodiode 光电二极管332.Iconoscope 光电摄像管333.Photolelctric effect 光电效应334.Spectral response 光谱响应335.Interline transfer CCD 行间转移型CCD336.Depletion layer 耗尽层plementary metal oxide semi-conductor 互补金属氧化物半导体338.Fundamental absorption edge 基本吸收带339.Valence band 价带340.Transistor 晶体管341.Visible light 可见光342.Spatial filter 空间滤波器343.Block access 块存取344.Pupil compensation 快门校正345.Diffusion current 扩散电流346.Discrete cosine transform 离散余弦变换347.Luminance signal 高度信号348.Quantum efficiency 量子效率349.Smear 漏光350.Edge enhancement 轮廓校正351.Nyquist frequency 奈奎斯特频率352.Energy band 能带353.Bias 偏压354.Drift current 漂移电流355.Clamp 钳位356.Global exposure 全面曝光357.Progressive scan 全像素读出方式358.Full frame CCD 全帧CCD359.Defect correction 缺陷补偿360.Thermal noise 热噪声361.Weak inversion 弱反转362.Shot noise 散粒噪声363.Chrominance difference signal 色差信号364.Color temperature 色温365.Minority carrier 少数载流子366.Image stabilizer 手振校正367.Horizontal CCD 水平CCD368.Random noise 随机噪声369.Tunneling effect 隧道效应370.Image sensor 图像传感器371.Aliasing 伪信号372.Passive 无源373.Passive pixel sensor 无源像素传感器374.Line transfer 线转移375.Correlated double sampling 相关双采样376.Pinned photodiode 掩埋型光电二极管377.Overflow 溢出378.Effective pixel 有效像素379.Active pixel sensor 有源像素传感器380.Threshold voltage 阈值电压381.Source follower 源极跟随器382.Illuminance 照度383.Refraction index 折射率384.Frame integration 帧读出方式385.Frame interline transfer CCD 帧行间转移CCD 386.Frame transfer 帧转移387.Frame transfer CCD 帧转移CCD388.Non interlace 逐行扫描389.Conversion efficiency 转换效率390.Automatic gain control 自动增益控制391.Self-induced drift 自激漂移392.Minimum illumination 最低照度393.CMOS image sensor COMS图像传感器394.MOS diode MOS二极管395.MOS image sensor MOS型图像传感器396.ISO sensitivity ISO感光度。
激光等离子体加速机制研究综述
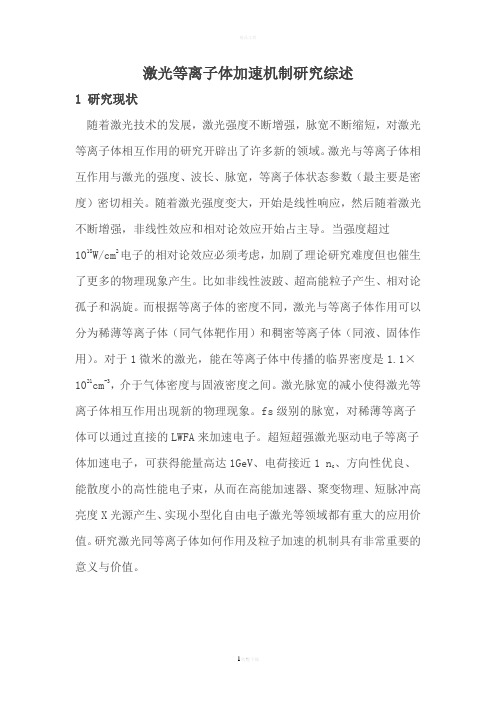
激光等离子体加速机制研究综述1 研究现状随着激光技术的发展,激光强度不断增强,脉宽不断缩短,对激光等离子体相互作用的研究开辟出了许多新的领域。
激光与等离子体相互作用与激光的强度、波长、脉宽,等离子体状态参数(最主要是密度)密切相关。
随着激光强度变大,开始是线性响应,然后随着激光不断增强,非线性效应和相对论效应开始占主导。
当强度超过1018W/cm2电子的相对论效应必须考虑,加剧了理论研究难度但也催生了更多的物理现象产生。
比如非线性波跛、超高能粒子产生、相对论孤子和涡旋。
而根据等离子体的密度不同,激光与等离子体作用可以分为稀薄等离子体(同气体靶作用)和稠密等离子体(同液、固体作用)。
对于1微米的激光,能在等离子体中传播的临界密度是1.1×1021cm-3,介于气体密度与固液密度之间。
激光脉宽的减小使得激光等离子体相互作用出现新的物理现象。
fs级别的脉宽,对稀薄等离子体可以通过直接的LWFA来加速电子。
超短超强激光驱动电子等离子体加速电子,可获得能量高达1GeV、电荷接近1 n c、方向性优良、能散度小的高性能电子束,从而在高能加速器、聚变物理、短脉冲高亮度X光源产生、实现小型化自由电子激光等领域都有重大的应用价值。
研究激光同等离子体如何作用及粒子加速的机制具有非常重要的意义与价值。
图1、激光强度在CPA技术突破后大幅增强首先,激光同等离子体作用的第一步是材料对激光的吸收,除了普通的逆轫致吸收和共振吸收,在高强度相对论激光还有很多吸收机制,比如真空加热,J×B加热,有质动力直接加速离子,鞘场加速等等,下面根据加速粒子不同逐一介绍各种加速机制1979年,Tajima和Dawson提出用强激光脉冲激发等离子体波来加速电子的机制,这就是直接激光尾场加速(LWFA)[1],原理是超强超短激光脉冲在稀薄等离子体中传播时,纵向的非线性力——有质动力(F p=-q2▽a02/4mw2)将电子推开,共振激发出等离子体波(尾波场)。
Laser Principle
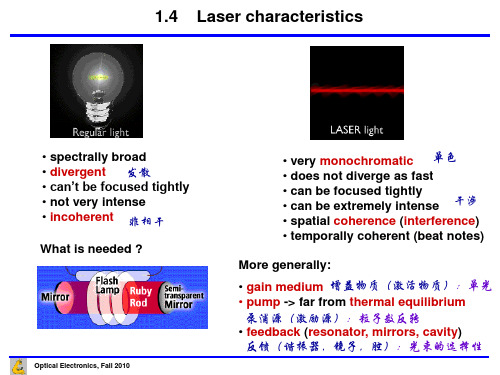
kink in output power 扭结、弯曲
Laser history
Javan invents He-Ne laser Maiman builds first Townes invents and Schawlow and Townes (ruby) LASER builds first MASER propose LASER Spectra introduces first fiber optic communication IBM builds first system Hall buildsAlferov builds first laser printer (Chicago) player Einstein predicts CD Ti:Sapphire laser heterostructure laser semiconductor stimulated emission laser Nakamura builds builds quantum built Faist nanowire laser at UCB blue laser diode cascade laser
Optical Electronics, Fall 2010
Optical Electronics, Fall 2010
Optical Electronics, Fall 2010
Optical Electronics, Fall 2010
Optical Electronics, Fall 2010
laser as amplifier
Input Monitor tap
隔离器
Isolator
隔离器
Isolator Monitor tap Output
大模场光纤
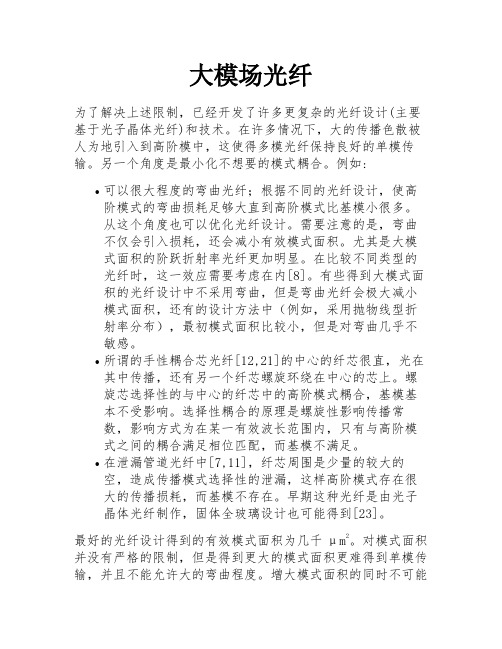
大模场光纤为了解决上述限制,已经开发了许多更复杂的光纤设计(主要基于光子晶体光纤)和技术。
在许多情况下,大的传播色散被人为地引入到高阶模中,这使得多模光纤保持良好的单模传输。
另一个角度是最小化不想要的模式耦合。
例如:•可以很大程度的弯曲光纤;根据不同的光纤设计,使高阶模式的弯曲损耗足够大直到高阶模式比基模小很多。
从这个角度也可以优化光纤设计。
需要注意的是,弯曲不仅会引入损耗,还会减小有效模式面积。
尤其是大模式面积的阶跃折射率光纤更加明显。
在比较不同类型的光纤时,这一效应需要考虑在内[8]。
有些得到大模式面积的光纤设计中不采用弯曲,但是弯曲光纤会极大减小模式面积,还有的设计方法中(例如,采用抛物线型折射率分布),最初模式面积比较小,但是对弯曲几乎不敏感。
•所谓的手性耦合芯光纤[12,21]的中心的纤芯很直,光在其中传播,还有另一个纤芯螺旋环绕在中心的芯上。
螺旋芯选择性的与中心的纤芯中的高阶模式耦合,基模基本不受影响。
选择性耦合的原理是螺旋性影响传播常数,影响方式为在某一有效波长范围内,只有与高阶模式之间的耦合满足相位匹配,而基模不满足。
•在泄漏管道光纤中[7,11],纤芯周围是少量的较大的空,造成传播模式选择性的泄漏,这样高阶模式存在很大的传播损耗,而基模不存在。
早期这种光纤是由光子晶体光纤制作,固体全玻璃设计也可能得到[23]。
最好的光纤设计得到的有效模式面积为几千μm2。
对模式面积并没有严格的限制,但是得到更大的模式面积更难得到单模传输,并且不能允许大的弯曲程度。
增大模式面积的同时不可能不影响单模传输。
原因在于,模式传播涉及到衍射和波导效应的平衡,由于模式面积更大时衍射不可避免的会减弱,平衡也变得对外界干扰越来越敏感。
在采用大模式光纤的高功率光纤激光器和放大器中,热透镜效应会改变模式性质,尤其会减小有效模式面积[29]。
有些情况下采用多丝纤芯可以减弱折射率控制存在的问题,其中光纤纤芯是由一系列二维排列的丝组成的[19]。
ingaas 波长调制 温度

ingaas 波长调制温度English Answer:Introduction.Indium gallium arsenide (InGaAs) is a III-V semiconductor material commonly used in optoelectronic devices due to its wide bandgap and high electron mobility. InGaAs-based devices, such as lasers and photodetectors, exhibit excellent performance characteristics, including high efficiency, low noise, and fast response times. However, the performance of InGaAs devices is sensitive to temperature variations, which can affect their wavelength response, output power, and other key parameters.Temperature Dependence of InGaAs Wavelength.The wavelength of light emitted or detected by an InGaAs device is directly related to the bandgap energy of the material. As temperature increases, the bandgap energyof InGaAs decreases, leading to a redshift in the wavelength. This effect is known as the temperature coefficient of wavelength (TCW). The TCW of InGaAs is typically negative, meaning that the wavelength increases as temperature decreases.The TCW of InGaAs devices can vary depending on the specific material composition and device design. For example, InGaAsP devices typically exhibit a smaller TCW than InGaAs devices due to the presence of phosphorus atoms in the material. Additionally, the TCW can be influenced by the presence of strain in the material, which can alter the bandgap energy.Temperature Effects on InGaAs Device Performance.In addition to affecting the wavelength response, temperature variations can also impact other performance parameters of InGaAs devices. For instance, the output power of an InGaAs laser can decrease with increasing temperature due to increased carrier scattering and reduced gain. The threshold current, which is the minimum currentrequired to achieve lasing, can also increase with temperature.Similarly, the responsivity of an InGaAs photodetector, which is a measure of its ability to convert light into electrical current, can decrease with increasing temperature. This is because the dark current, which is the current that flows through the device in the absence of light, increases with temperature.Temperature Control for InGaAs Devices.To ensure optimal performance and stability of InGaAs devices, it is essential to control the temperature of the device during operation. This can be achieved through various means, such as the use of thermoelectric coolers (TECs), temperature-controlled enclosures, or heat sinks.TECs are semiconductor devices that can generate a temperature difference between two surfaces when anelectric current is applied. By placing a TEC in thermal contact with the InGaAs device, the temperature of thedevice can be precisely controlled. Temperature-controlled enclosures provide a stable thermal environment for the device, while heat sinks help to dissipate heat generatedby the device.Conclusion.The performance of InGaAs devices is sensitive to temperature variations. The wavelength response, output power, and other key parameters can be affected by changesin temperature. Therefore, it is important to control the temperature of InGaAs devices during operation to ensure optimal performance and stability.Chinese Answer:导言。
二维材料异质结高灵敏度红外探测器
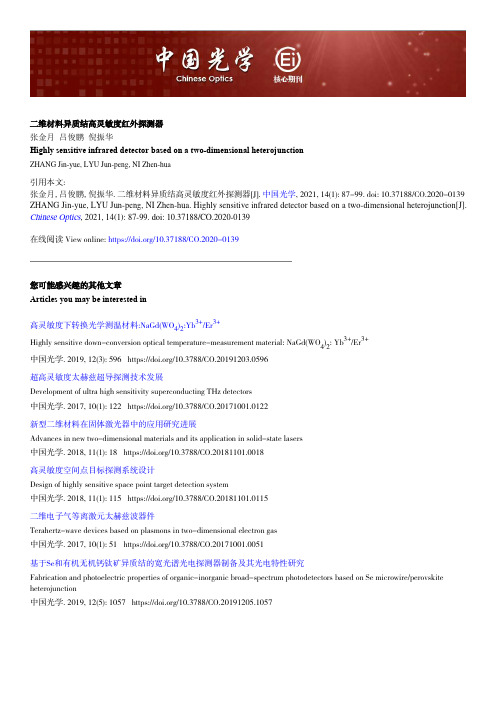
文章编号 2095-1531(2021)01-0087-13
中国光学 Chinese Optics
Vol. 14 No. 1 Jan. 2021
二维材料异质结高灵敏度红外探测器
张金月,吕俊鹏*,倪振华*
(东南大学 物理学院,江苏 南京 211100)
摘要:要想实现弱光探测,需要探测器具有高灵敏度。石墨烯、过渡金属硫化物、黑磷等二维材料因具有宽光谱吸收、带
88
中国光学
第 14 卷
factors affecting sensitivity, we provide a comprehensive review of the strategies that improve the sensitivity of infrared detectors and the development of high-sensitivity infrared detectors based on 2D heterojunctions in recent years. We summarize the figures of merit of these infrared detectors and identify the existing challenges impeding further improvements in sensitivity. Finally, by summarizing the challenges of future improving the sensitivity of infrared detection prospects for strategies to obtain high-sensitivity infrared detectors with good comprehensive performance and commercial applicability are presented with considerations for balancing the detector’s responsivity and response speed, large area two-dimensional heterojunction preparation, heterojunction interface optimization, and so forth. Key words: infrared detection;high sensitivity;2D materials;heterojunction
微波光电子学
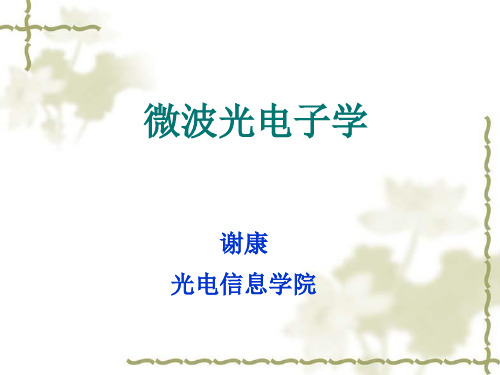
微波光电子学研究光波与微波的相互作用,
主要包括光的微波调制,外差光生微波源, 微波信号的探测,微波器件的光学控制等 领域的机理和技术。 微波光电子学的主要应用领域包括光信息 处理,微波的光载传输,相控阵天线波束 光学实时延迟控制及波束合成。
• 光对微波信号产生、放大与交换的调
控作用,主要是利用光对微波半导体
器件有源层中载流子浓度和运动的激
发与控制;
• 微波对光传输、折射偏振及信号传递
的调ቤተ መጻሕፍቲ ባይዱ作用则利用导光媒质的极化与
载流子分布受微波场变化而导致光导
率、折射与偏振特性的改变。
微波光电子学早期工作 1. Optical sources capable of fast modulation; 2. Suitable transmission media; 3. Fast optical detectors;
Faster response requires high p: • reducing the photon lifetime p short optical cavity, reduced facet reflectivity • increasing the differential gain g0 reduced dimensionality • increasing the photon densities S0 small cavity volumes or short and narrow optical waveguides
3. Fast optical detectors For detection, fast depletion and
avalanche detectors were developed at
第46_届国际红外毫米波与太赫兹会议综述:太赫兹辐射源的最新研究进展

第 21 卷 第 11 期2023 年 11 月Vol.21,No.11Nov.,2023太赫兹科学与电子信息学报Journal of Terahertz Science and Electronic Information Technology第46届国际红外毫米波与太赫兹会议综述:太赫兹辐射源的最新研究进展郑文杰,吴振华*,张晓秋艳*,赵陶,钟任斌,刘頔威,魏彦玉,宫玉彬,胡旻(电子科技大学电子科学与工程学院,四川成都611731)摘要:太赫兹波段介于微波与红外之间,具有许多独特的优势,如对许多物质的穿透能力、较低的光子能量等。
这使得太赫兹技术在生物医学、安全检测、通信等领域具有广泛的应用前景。
太赫兹辐射源是太赫兹技术的核心组件,其发展水平直接决定了太赫兹技术在各个应用领域的性能和前景。
第46届国际红外毫米波与太赫兹会议聚焦了太赫兹辐射源的最新研究成果,展示了在新型辐射源、集成技术及优化设计等方面的重要进展。
本文根据近段时间红外毫米波与太赫兹相关国际关会议报告的内容,总结并展示了不同类型太赫兹辐射源最前沿的研究内容与方向。
这些成果为太赫兹技术在各个应用领域的进一步发展奠定了坚实基础。
关键词:太赫兹技术;辐射源;第46届国际红外毫米波与太赫兹会议中图分类号:O441.4 文献标志码:A doi:10.11805/TKYDA2022042 Review of theReview of the 4646th international conference on infrared millimeter waves and th international conference on infrared millimeter waves and terahertzterahertz:: recent research advances in terahertz radiation sourcesZHENG Wenjie,WU Zhenhua*,ZHANG Xiaoqiuyan*,ZHAO Tao,ZHONG Renbin,LIU Diwei,WEI Yanyu,GONG Yubin,HU Min(School of Electronic Science and Engineering,University of Electronic Science and Technology of China,Chengdu Sichuan 611731,China) AbstractAbstract::The terahertz band is between microwave and infrared and has many unique advantages, such as the ability to penetrate many substances and lower photon energy. This makesterahertz technology promising for a wide range of applications in the fields of biomedicine, securitydetection, and communications. Terahertz radiation source is the core component of terahertztechnology, and its development level directly determines the performance and prospect of terahertztechnology in various applications. The 46th International Conference on Infrared Millimeter Wavesand Terahertz focuses on the latest research results of terahertz radiation sources and demonstratesimportant progress in new radiation sources, integration technology and optimized design. This papersummarizes and shows the cutting-edge research content and directions of different types ofterahertz radiation sources based on the reports of recent international conferences related toinfrared millimeter wave and terahertz. These results lay a solid foundation for the furtherdevelopment of terahertz technology in various applications.KeywordsKeywords::terahertz technology;radiation sources;the 46th international conference on infrared millimeter waves and terahertz1 太赫兹辐射源简介太赫兹(THz)技术,在0.1~10 THz的频率范围内运行,近年来获得了极大的关注,因为它有可能彻底改变各个领域,如成像、通信、安全和感应。
- 1、下载文档前请自行甄别文档内容的完整性,平台不提供额外的编辑、内容补充、找答案等附加服务。
- 2、"仅部分预览"的文档,不可在线预览部分如存在完整性等问题,可反馈申请退款(可完整预览的文档不适用该条件!)。
- 3、如文档侵犯您的权益,请联系客服反馈,我们会尽快为您处理(人工客服工作时间:9:00-18:30)。
LBNL-51615High Gain Free Electron LasersDriven by Flat Electron BeamMing XieLawrence Berkeley National LaboratoryBerkeley,California94720,USAPresented at24th International Free Electron Laser ConferenceArgonne,USA,September9-13,2002To be Published inNuclear Instruments and Methods in Physics Research AThis work was supported by the Director,Office of Science,Office of High Energy and Nuclear Physics,High Energy Physics Division,of the U.S.De-partment of Energy under contract No.DE-AC03-76SF00098..High Gain Free Electron LasersDriven by Flat Electron BeamMing Xie∗Lawrence Berkeley National Laboratory,Berkeley,CA94720,USA AbstractA variational solution of the fundamental eigenmode is presented for high gain free electron lasers driven byflat electron beam having unequal emittances,betatron focusings and beam sizes in two transverse planes.Key words:flat beam,elliptical beam,high gain free electron laserPACS:41.60.Cr1IntroductionThe main objective of this article is to expand our earlier work[1]on the eigenmode solution for high gain FELs into the situation where electron beam distribution is no longer axially symmetrical.Such a beam,generally termed flat beam,may have unequal emittances,betatron focusings,or beam sizes in two transverse planes.Earlier studies on this subject date back more than aWork supported by U.S.DOE under contract No.DE-AC03-76SF00098.∗Corresponding author.Tel.:+1-510-486-5616;fax:+1-510-486-6485Email address:mingxie@(Ming Xie).decade ing a special variational technique[2],the fundamental mode was calculated by Xie[3]for parallel beam having elliptical cross ter, a dispersion equation for the eigenvalue of the fundamental mode was derived using a different technique by Chin et al.[4]for more general cases including unequal emittances and betatron focusings.However,the latter technique was known at birth[4]to introduce a systematic error when approaching the1D limit,thus compromising its accuracy for short wavelength FEL calculations, and furthermore,the dispersion equation was not solved.In this article,we present thefirst generalflat beam solution and specific calculations for the fundamental eigenmode and examine effects offlat beam on high gain FEL performance.The eigenmode equation is formulated forflat beam in section2.The variational solution is derived in section3.Finally, specific calculations for the LCLS case are given in section4.2Eigenmode EquationWe specify the radiationfield by a complex envelope a r(x,z,t)slowly varying with respect to a carrier wave exp(k r z−ωr t),where x≡{x,y},the carrier frequencyωr=ck r=2πc/λr is determined by the resonance condition k r =2γ20k w/(1+a2w),γ0mc2is average electron energy,λw=2π/k w is wiggler period,a w=eB rmsλw/2πmc,and B rms is rms wigglerfield assumed con-stant along wiggler axis.The radiationfield is normalized according to|a r|= eE rms/k r mc2,where E rms(x,z,t)is rms amplitude of the electricfield.Upon introducing Fourier transform byaν=1√2π dθe−iνθ(e iθa r),whereθ=(k r+k w)z−ωr t andν=ω/ωr,the eigenmode of the form aν= a(x)exp(−iµz)can be determined by the mode equation[1,5,6]µ−k w∆ν+12kr∂2∂x2 a(x)=ih16k w L1d∞−∞d2p dη∂F∂η−∞dse−i(µ−ξ)s a(x ),(1)withξ=2k wη−k r2(p2x+k2βx x2+p2y+k2βy y2),x =x cos(kβx s)+p xkβxsin(kβx s),y =y cos(kβy s)+p ykβysin(kβy s),L1d=λw4π 2πhγ30 r e n0λ2w a2w f2B13,whereµis the complex eigenvalue related to our earlier notation[1]byµ= iq/2L1d+k w∆ν,L1d is the1D power gain length,∆ν=ν−1,η=(γ−γ0)/γ0,h=(2/√3)3,fB=1for helical wiggler and f B=J0(χw)−J1(χw)for planarwiggler,χw=a2w/2(1+a2w),r e is the classical radius of electron,n0is the peak electron volume density on the axis,F=F⊥(x,p)F (η)is unperturbed beam distribution function normalized by∞ −∞d2p F⊥(x=0,p)=1,∞−∞dηF (η)=1.Transverse focusing of the beam in wiggler is assumed to have a strength invariant along the axis,thus betatron motion is governed byp x=dxdz,dp xdz=−k2βx x,p y=dydz,dp ydz=−k2βy y,whereβx=1/kβx andβy=1/kβy are constant betafunctions.To perform specific calculations,we use a Gaussian model withF ⊥=12πσx σy k βx k βye−x 2+p 2x /k 2βx2σ2x −y 2+p 2y /k 2βy 2σ2y,F =1√2πσηe −η22σ2η,where σx =√βx εx and σy =βy εy are rms beam sizes matched to the focusingchannel,εx and εy are rms emittances,and σηis relative rms energy ing X ≡{X,Y }≡{x/σx ,y/σy }and τ=s/2L 1d ,Eq.(1)can be expressed in a more compact scaled form2ηdx ∂2∂X 2+2ηdy∂2∂Y 2+¯κa (X )=∞−∞d 2X Π(X ,X )a (X),(2)where ¯κ=κ−ηω,κ=2L 1d µandΠ(X ,X)=−∞τdτhe −Ψ2πΦ,Φ=sin(2√ηdx ηεx τ)sin(2√ηdy ηεy τ),Ψ=iκτ+2η2γτ2+(1+iηεx τ)Ωx 2sin 2(2√ηdx ηεx τ)+(1+iηεy τ)Ωy 2sin 2(2√ηdy ηεy τ),Ωx =X 2+X 2−2XX cos(2√ηdx ηεx τ),Ωy =Y 2+Y 2−2Y Y cos(2√ηdy ηεy τ).There are six scaling parameters in Eq.(2):ηdx =L 1d /2k r σ2x and ηdy =L 1d /2k r σ2y are diffraction parameters;ηεx =2L 1d k r k βx εx and ηεy =2L 1d k r k βy εycharacterize effective spread in longitudinal phase due to emittance and be-tatron focusing,and ηγ=2L 1d k w σηdue to energy spread,respectively;and ηω=2L 1d k w ∆νis a frequency detuning parameter.The 1D power gain length can now be expressed asL 1d=λw4πhI A σx σy k 2w γ3I b a 2w f B13,where I b is beam current and I A =17.05kA is the Alf`v en current.3Variational SolutionNext,we present an approximate solution for the fundamental mode.Accord-ing to the recipe of a special variational technique[2],a variational functional may be constructed from Eq.(2)as∞−∞d2X a(X) 2ηdx∂2∂X2+2ηdy∂2∂Y2+¯κ a(X)=∞−∞d2X d2X a(X)Π(X,X )a(X ).Substituting into the variational functional a trial solution of the forma(X)=exp(−αx X2−αy Y2),whereαx andαy are complex variational parameters to be determined,and applying the variational conditions[2]δκδαx =0,δκδαy=0,to the resulting equation,we obtain three equations from which the eigenvalue κand mode parametersαx andαy can be determined byF1≡¯κ4√αxαy−ηdx2 αxαy−ηdy2αyαx−0−∞τdτhe−f1f2x f2y=0,(3)F2≡−¯κ8αx√αxαy−ηdx4√αxαy+ηdy4αx αyαx+−∞τdτhe−f1f 2x2f2x f2x f2y=0,(4)F3≡−¯κ8αy√αxαy−ηdy4√αxαy+ηdx4αy αxαy+−∞τdτhe−f1f 2y2f2y f2x f2y=0,(5)whereF 2(κ,αx ,αy )=∂F 1(κ,αx ,αy )∂αx,F 3(κ,αx ,αy )=∂F 1(κ,αx ,αy )∂αy,f 1=iκτ+2η2γτ2,f 2x =(1+iηεx τ)2+4αx (1+iηεx τ)+4α2x sin 2(2√ηdx ηεx τ),f 2y =(1+iηεy τ)2+4αy (1+iηεy τ)+4α2y sin 2(2√ηdy ηεy τ),f 2x=∂f 2x ∂αx =4(1+iηεx τ)+8αx sin 2(2√ηdx ηεx τ),f2y=∂f 2y ∂αy=4(1+iηεy τ)+8αy sin 2(2√ηdy ηεy τ).It is noted that in the limit of ηεx =ηεy =ηγ=0,Eqs.(3,4,5)are reduced to the same equations studied earlier [3]for the case of parallel Gaussian beam with elliptical cross section.Given parameter αx and αy ,the mode properties can be determined com-pletely by comparing the mode profilea =exp−αx x 2σ2x −αy y2σ2y,with the usual Gaussian descriptiona =exp−x 2w 2x +ik r x 22R x −y 2w 2y +ik r y 22R y,where w x and w y are mode sizes,R x and R y are radii of phasefront curvature.In particular,we have for mode sizesw x =σx√αxr,w y =σy√αyr,and for far field divergence anglesθx =αxr1+α2xi α2xrλrπσx,θy =αyr1+α2yi α2yrλrπσy.Finally,power gain length of the fundamental mode is related to the eigenvalue by L g =L 1d /κi.5678910L g (m )R εFig.1.L g as a function of R εvaried from 1to 50with βx and βy optimized to minimize L g.20406080100βx a n d βy (m )R εFig.2.Optimized βx and βy as functions of R εvaried from 1to 50.51015202530R σ a n d R wR εFig.3.R σand R w as functions of R εvaried from 1to 50with optimized βx and βy .4LCLS ExamplesGiven the solution of Eqs.(3,4,5),we are now ready to examine effects of flat beam on high gain FEL performance.Let’s first define ratios of emittances,betafunctions,beam sizes and mode sizes respectively byR ε=εx εy ,R β=βx βy ,R σ=σx σy ,R w =w x w y.Consider a scenario in which R εis increased from unity under the constraint that 4D emittance εx εy =ε20is kept constant.At each value of R ε,L g is minimized by varying βx ,βy and frequency detuning.We shall take LCLS nominal design values for all other parameters [7]:λr =1.5˚A ,γ0=28009,I b =3.4kA,γ0ε0=1.5mm-mrad,ση=2×10−4,a planar wiggler with λw =3cm and √2a w =3.7.In this scenario R εis the only free varying parameter.Figure 1shows L g as a function of R εvaried from 1to 50with optimized βx and βy given in Figure 2.At larger value of εx ,focusing has to be relaxed with larger βx to minimize gain reduction due to angular spread.On the otherTable1LCLS ExamplesRε11050γ0εx(mm-mr) 1.5 4.7410.6γ0εy(mm-mr) 1.50.4740.212βx(m)204795βy(m)209.4 6.7σx(µm)3290190σy(µm)32137.1w x(µm)4476120w y(µm)442926θx(µr) 1.6 1.10.83θy(µr) 1.6 2.4 3.1L g(m) 6.0 6.78.3L1d(m) 3.1 3.1 3.3hand,focusing can be enhanced with smallerβy at smaller value ofεy.Figure 3shows Rσand R w as functions of Rεvaried from1to50.Notice the aspect ratio of laser mode is much less than that of electron beam.More details are given in Table1for three cases Rε=1,10,50.5ConclusionsWe have presented an effective solution of the eigenmode for high gain FELs driven by generalflat beam.It is found that the gain length increases with emittance ratio when4D emittance is kept constant,and the rate of increase is rather weak in the LCLS parameter regime if beam focusing is simultaneously optimized.In addition,the aspect ratio of laser mode is much smaller than that of electron beam for larger emittance ratio.Effects offlat beam in other regimes and for other scenarios can be readily evaluated with the solution and methods provided here.References[1]M.Xie,Nucl.Instr.Meth.A445,59and67(2000).[2]M.Xie and D.Deacon,Nucl.Instr.Meth.A250,426(1986).[3]M.Xie,“Theory of Optical Guiding in Free Electron Lasers”,Ph.D.dissertation,Stanford University,(1988).[4]Y.Chin,K.Kim and M.Xie,Phys.Rev.A46,6662(1992).[5]K.Kim,Phys.Rev.Lett.57,1871(1986).[6]L.Yu,S.Krinsky and R.Gluckstern,Phys.Rev.Lett.64,3011(1990).[7]LCLS Design Study Report,SLAC-R-521,(1998).。