土木专业英语翻译作业
土木工程专业英语(带翻译)

State-of-the-art report of bridge health monitoring AbstractThe damage diagnosis and healthmonitoring of bridge structures are active areas of research in recent years. Comparing with the aerospace engineering and mechanical engineering, civil engineering has the specialities of its own in practice. For example, because bridges, as well as most civil engineering structures, are large in size, and have quite lownatural frequencies and vibration levels, at low amplitudes, the dynamic responses of bridge structure are substantially affected by the nonstructural components, unforeseen environmental conditions, and changes in these components can easily to be confused with structural damage.All these give the damage assessment of complex structures such as bridges a still challenging task for bridge engineers. This paper firstly presents the definition of structural healthmonitoring system and its components. Then, the focus of the discussion is placed on the following sections:①the laboratory and field testing research on the damage assessment;②analytical developments of damage detectionmethods, including (a) signature analysis and pattern recognition approaches, (b) model updating and system identification approaches, (c) neural networks approaches; and③sensors and their optimum placements. The predominance and shortcomings of each method are compared and analyzed. Recent examples of implementation of structural health monitoring and damage identification are summarized in this paper. The key problem of bridge healthmonitoring is damage automatic detection and diagnosis, and it is the most difficult problem. Lastly, research and development needs are addressed.1 IntroductionDue to a wide variety of unforeseen conditions and circumstance, it will never be possible or practical to design and build a structure that has a zero percent probability of failure. Structural aging, environmental conditions, and reuse are examples of circumstances that could affect the reliability and thelife of a structure. There are needs of periodic inspections to detect deterioration resulting from normal operation and environmental attack or inspections following extreme events, such as strong-motion earthquakes or hurricanes. To quantify these system performance measures requires some means to monitor and evaluate the integrity of civil structureswhile in service. Since the Aloha Boeing 737 accident that occurred on April 28, 1988, such interest has fostered research in the areas of structural health monitoring and non-destructive damage detection in recent years.According to Housner, et al. (1997), structural healthmonitoring is defined as“the use ofin-situ,non-destructive sensing and analysis of structural characteristics, including the structural response, for detecting changes that may indicate damage or degradation”[1]. This definition also identifies the weakness. While researchers have attempted the integration of NDEwith healthmonitoring, the focus has been on data collection, not evaluation. What is needed is an efficient method to collect data from a structure in-service and process the data to evaluate key performance measures, such as serviceability, reliability, and durability. So, the definition byHousner, et al.(1997)should be modified and the structural health monitoring may be defined as“the use ofin-situ,nondestructive sensing and analysis of structural characteristics, including the structural response, for the purpose of identifying if damage has occurred, determining the location of damage, estimatingthe severityof damage and evaluatingthe consequences of damage on the structures”(Fig.1). In general, a structural health monitoring system has the potential to provide both damage detection and condition assessment of a structure.Assessing the structural conditionwithout removingthe individual structural components is known as nondestructive evaluation (NDE) or nondestructive inspection. NDE techniques include those involving acoustics, dye penetrating,eddy current, emission spectroscopy, fiber-optic sensors, fiber-scope, hardness testing, isotope, leak testing, optics, magnetic particles, magnetic perturbation, X-ray, noise measurements, pattern recognition, pulse-echo, ra-diography, and visual inspection, etc. Mostof thesetechniques have been used successfullyto detect location of certain elements, cracks orweld defects, corrosion/erosion, and so on. The FederalHighwayAdministration(FHWA, USA)was sponsoring a large program of research and development in new technologies for the nondestructive evaluation of highway bridges. One of the two main objectives of the program is to develop newtools and techniques to solve specific problems. The other is to develop technologies for the quantitative assessment of the condition of bridges in support of bridge management and to investigate howbest to incorporate quantitative condition information into bridge management systems. They hoped to develop technologies to quickly, efficiently, and quantitatively measure global bridge parameters, such as flexibility and load-carrying capacity. Obviously, a combination of several NDE techniques may be used to help assess the condition of the system. They are very important to obtain the data-base for the bridge evaluation.But it is beyond the scope of this review report to get into details of local NDE.Health monitoring techniques may be classified as global and local. Global attempts to simultaneously assess the condition of the whole structure whereas local methods focus NDE tools on specific structural components. Clearly, two approaches are complementaryto eachother. All such available informationmaybe combined and analyzed by experts to assess the damage or safety state of the structure.Structural health monitoring research can be categorized into the following four levels: (I) detecting the existence of damage, (II) findingthe location of damage, (III) estimatingthe extentof damage, and (IV) predictingthe remaining fatigue life. The performance of tasks of Level (III) requires refined structural models and analyses, local physical examination, and/or traditional NDE techniques. To performtasks ofLevel (IV) requires material constitutive information on a local level, materials aging studies, damage mechanics, and high-performance computing. With improved instrumentation and understanding of dynamics of complex structures, health monitoring and damage assessment of civil engineering structures has become more practical in systematic inspection andevaluation of these structures during the past two decades.Most structural health monitoringmethods under current investigation focus on using dynamic responses to detect and locate damage because they are global methods that can provide rapid inspection of large structural systems.These dynamics-based methods can be divided into fourgroups:①spatial-domain methods,②modal-domain methods,③time-domain methods, and④frequency- domain methods. Spatial-domain methods use changes of mass, damping, and stiffness matrices to detect and locate damage. Modal-domain methods use changes of natural frequencies, modal damping ratios, andmode shapesto detect damage. In the frequency domain method, modal quantities such as natural frequencies, damping ratio, and model shapes are identified.The reverse dynamic systemof spectral analysis and the generalized frequency response function estimated fromthe nonlinear auto-regressive moving average (NARMA) model were applied in nonlinear system identification. In time domainmethod, systemparameterswere determined fromthe observational data sampled in time. It is necessaryto identifythe time variation of systemdynamic characteristics fromtime domain approach if the properties of structural system changewith time under the external loading condition. Moreover, one can use model-independent methods or model-referenced methods to perform damage detection using dynamic responses presented in any of the four domains. Literature shows that model independent methods can detect the existence of damage without much computational efforts, butthey are not accurate in locating damage. On the otherhand, model-referencedmethods are generally more accurate in locating damage and require fewer sensors than model-independent techniques, but they require appropriate structural models and significant computational efforts. Although time-domain methods use original time-domain datameasured using conventional vibrationmeasurement equipment, theyrequire certain structural information and massive computation and are case sensitive. Furthermore, frequency- and modal-domain methods use transformed data,which contain errors and noise due totransformation.Moreover, themodeling and updatingofmass and stiffnessmatrices in spatial-domain methods are problematic and difficult to be accurate. There are strong developmenttrends that two or three methods are combined together to detect and assess structural damages.For example, several researchers combined data of static and modal tests to assess damages. The combination could remove the weakness of each method and check each other. It suits the complexity of damage detection.Structural health monitoring is also an active area of research in aerospace engineering, but there are significant differences among the aerospace engineering, mechanical engineering, and civil engineering in practice. For example,because bridges, as well as most civil engineering structures, are large in size, and have quite lownatural frequencies and vibration levels, at lowamplitudes, the dynamic responses of bridge structure are substantially affected by the non-structural components, and changes in these components can easily to be confused with structural damage. Moreover,the level of modeling uncertainties in reinforced concrete bridges can be much greater than the single beam or a space truss. All these give the damage assessment of complex structures such as bridges a still challenging task for bridge engineers. Recent examples of research and implementation of structural health monitoring and damage assessment are summarized in the following sections.2 Laboratory and field testing researchIn general, there are two kinds of bridge testing methods, static testing and dynamic testing. The dynamic testing includes ambient vibration testing and forced vibration testing. In ambient vibration testing, the input excitation is not under the control. The loading could be either micro-tremors, wind, waves, vehicle or pedestrian traffic or any other service loading. The increasing popularity of this method is probably due to the convenience of measuring the vibrationresponse while the bridge is under in-service and also due to the increasing availability of robust data acquisition and storage systems. Since the input is unknown, certain assumptions have to be made. Forced vibration testing involves application of input excitation of known force level at known frequencies. The excitation manners include electro-hydraulic vibrators, forcehammers, vehicle impact, etc. The static testing in the laboratory may be conducted by actuators, and by standard vehicles in the field-testing.we can distinguish that①the models in the laboratory are mainly beams, columns, truss and/or frame structures, and the location and severity of damage in the models are determined in advance;②the testing has demonstrated lots of performances of damage structures;③the field-testing and damage assessmentof real bridges are more complicated than the models in the laboratory;④the correlation between the damage indicator and damage type,location, and extentwill still be improved.3 Analytical developmentThe bridge damage diagnosis and health monitoring are both concerned with two fundamental criteria of the bridges, namely, the physical condition and the structural function. In terms of mechanics or dynamics, these fundamental criteria can be treated as mathematical models, such as response models, modal models and physical models.Instead of taking measurements directly to assess bridge condition, the bridge damage diagnosis and monitoring systemevaluate these conditions indirectly by using mathematical models. The damage diagnosis and health monitoring are active areas of research in recentyears. For example, numerous papers on these topics appear in the proceedings of Inter-national Modal Analysis Conferences (IMAC) each year, in the proceedings of International Workshop on Structural HealthMonitoring (once of two year, at Standford University), in the proceedings of European Conference on Smart materials and Structures and European Conference on Structural Damage AssessmentUsing Advanced Signal Processing Procedures, in the proceedings ofWorld Conferences of Earthquake Engineering, and in the proceedings of International Workshop on Structural Control, etc.. There are several review papers to be referenced, for examples,Housner, et al. (1997)provided an extensive summary of the state of the art in control and health monitoring of civil engineering structures[1].Salawu (1997)discussed and reviewed the use of natural frequency as a diagnostic parameter in structural assessment procedures using vibrationmonitoring.Doebling, Farrar, et al. (1998)presented a through review of the damage detection methods by examining changes in dynamic properties.Zou, TongandSteven (2000)summarized the methods of vibration-based damage and health monitoring for composite structures, especially in delamination modeling techniques and delamination detection.4 Sensors and optimum placementOne of the problems facing structural health monitoring is that very little is known about the actual stress and strains in a structure under external excitations. For example, the standard earthquake recordings are made ofmotions of the floors of the structure and no recordings are made of the actual stresses and strains in structural members. There is a need for special sensors to determine the actual performance of structural members. Structural health monitoring requires integrated sensor functionality to measure changes in external environmental conditions, signal processing functionality to acquire, process, and combine multi-sensor and multi-measured information. Individual sensors and instrumented sensor systems are then required to provide such multiplexed information.FuandMoosa (2000)proposed probabilistic advancing cross-diagnosis method to diagnosis-decision making for structural health monitoring. It was experimented in the laboratory respectively using a coherent laser radar system and a CCD high-resolution camera. Results showed that this method was promising for field application. Another new idea is thatneural networktechniques are used to place sensors. For example,WordenandBurrows (2001)used the neural network and methods of combinatorial optimization to locate and classify faults.The static and dynamic data are collected from all kinds of sensorswhich are installed on the measured structures.And these datawill be processed and usable informationwill be extracted. So the sensitivity, accuracy, and locations,etc. of sensors are very important for the damage detections. The more information are obtained, the damage identification will be conducted more easily, but the price should be considered. That’s why the sensors are determinedin an optimal ornearoptimal distribution. In aword, the theory and validation ofoptimumsensor locationswill still being developed.5 Examples of health monitoring implementationIn order for the technology to advance sufficiently to become an operational system for the maintenance and safety of civil structures, it is of paramount importance that new analytical developments are ultimately verified with appropriate data obtained frommonitoring systems, which have been implemented on civil structures, such as bridges.Mufti (2001)summarized the applications of SHM of Canadian bridge engineering, including fibre-reinforced polymers sensors, remote monitoring, intelligent processing, practical applications in bridge engineering, and technology utilization. Further study and applications are still being conducted now.FujinoandAbe(2001)introduced the research and development of SHMsystems at the Bridge and Structural Lab of the University of Tokyo. They also presented the ambient vibration based approaches forLaser DopplerVibrometer (LDV) and the applications in the long-span suspension bridges.The extraction of the measured data is very hard work because it is hard to separate changes in vibration signature duo to damage form changes, normal usage, changes in boundary conditions, or the release of the connection joints.Newbridges offer opportunities for developing complete structural health monitoring systems for bridge inspection and condition evaluation from“cradle to grave”of the bridges. Existing bridges provide challenges for applying state-of-the-art in structural health monitoring technologies to determine the current conditions of the structural element,connections and systems, to formulate model for estimating the rate of degradation, and to predict the existing and the future capacities of the structural components and systems. Advanced health monitoring systems may lead to better understanding of structural behavior and significant improvements of design, as well as the reduction of the structural inspection requirements. Great benefits due to the introduction of SHM are being accepted by owners, managers, bridge engineers,etc..6 Research and development needsMost damage detection theories and practices are formulated based on the following assumption: that failure or deterioration would primarily affect the stiffness and therefore affect the modal characteristics of the dynamic response of the structure. This is seldom true in practice, because①Traditional modal parameters (natural frequency, damping ratio and mode shapes, etc.) are not sensitive enough to identify and locate damage. The estimation methods usually assume that structures are linear and proportional damping systems.②Most currently used damage indices depend on the severity of the damage, which is impractical in the field. Most civil engineering structures, such as highway bridges, have redundancy in design and large in size with low natural frequencies. Any damage index should consider these factors.③Scaledmodelingtechniques are used in currentbridge damage detection. Asingle beam/girder models cannot simulate the true behavior of a real bridge. Similitude laws for dynamic simulation and testing should be considered.④Manymethods usually use the undamaged structural modal parameters as the baseline comparedwith the damaged information. This will result in the need of a large data storage capacity for complex structures. But in practice,there are majority of existing structures for which baseline modal responses are not available. Only one developed method(StubbsandKim (1996)), which tried to quantify damagewithout using a baseline, may be a solution to this difficulty. There is a lot of researchwork to do in this direction.⑤Seldommethods have the ability to distinguish the type of damages on bridge structures. To establish the direct relationship between the various damage patterns and the changes of vibrational signatures is not a simple work.Health monitoring requires clearly defined performance criteria, a set of corresponding condition indicators and global and local damage and deterioration indices, which should help diagnose reasons for changes in condition indicators. It is implausible to expect that damage can be reliably detected or tracked byusing a single damage index. We note that many additional localized damage indiceswhich relate to highly localized properties ofmaterials or the circumstances may indicate a susceptibility of deterioration such as the presence of corrosive environments around reinforcing steel in concrete, should be also integrated into the health monitoring systems.There is now a considerable research and development effort in academia, industry, and management department regarding global healthmonitoring for civil engineering structures. Several commercial structural monitoring systems currently exist, but further development is needed in commercialization of the technology. We must realize that damage detection and health monitoring for bridge structures by means of vibration signature analysis is a very difficult task. Itcontains several necessary steps, including defining indicators on variations of structural physical condition, dynamic testing to extract such indication parameters, defining the type of damages and remaining capacity or life of the structure, relating the parameters to the defined damage/aging. Unfortunately, to date, no one has accomplished the above steps. There is a lot of work to do in future.桥梁健康监测应用与研究现状摘要桥梁损伤诊断与健康监测是近年来国际上的研究热点,在实践方面,土木工程和航空航天工程、机械工程有明显的差别,比如桥梁结构以及其他大多数土木结构,尺寸大、质量重,具有较低的自然频率和振动水平,桥梁结构的动力响应极容易受到不可预见的环境状态、非结构构件等的影响,这些变化往往被误解为结构的损伤,这使得桥梁这类复杂结构的损伤评估具有极大的挑战性.本文首先给出了结构健康监测系统的定义和基本构成,然后集中回顾和分析了如下几个方面的问题:①损伤评估的室内实验和现场测试;②损伤检测方法的发展,包括:(a)动力指纹分析和模式识别方法, (b)模型修正和系统识别方法, (c)神经网络方法;③传感器及其优化布置等,并比较和分析了各自方法的优点和不足.文中还总结了健康监测和损伤识别在桥梁工程中的应用,指出桥梁健康监测的关键问题在于损伤的自动检测和诊断,这也是困难的问题;最后展望了桥梁健康监测系统的研究和发展方向.关键词:健康监测系统;损伤检测;状态评估;模型修正;系统识别;传感器优化布置;神经网络方法;桥梁结构1概述由于不可预见的各种条件和情况下,设计和建造一个结构将永远不可能或无实践操作性,它有一个失败的概率百分之零。
土木工程专业英语翻译
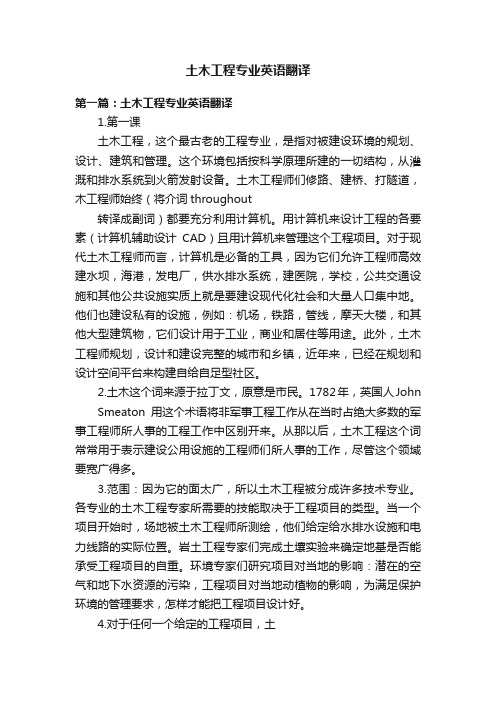
土木工程专业英语翻译第一篇:土木工程专业英语翻译1.第一课土木工程,这个最古老的工程专业,是指对被建设环境的规划、设计、建筑和管理。
这个环境包括按科学原理所建的一切结构,从灌溉和排水系统到火箭发射设备。
土木工程师们修路、建桥、打隧道,木工程师始终(将介词throughout转译成副词)都要充分利用计算机。
用计算机来设计工程的各要素(计算机辅助设计CAD)且用计算机来管理这个工程项目。
对于现代土木工程师而言,计算机是必备的工具,因为它们允许工程师高效建水坝,海港,发电厂,供水排水系统,建医院,学校,公共交通设施和其他公共设施实质上就是要建设现代化社会和大量人口集中地。
他们也建设私有的设施,例如:机场,铁路,管线,摩天大楼,和其他大型建筑物,它们设计用于工业,商业和居住等用途。
此外,土木工程师规划,设计和建设完整的城市和乡镇,近年来,已经在规划和设计空间平台来构建自给自足型社区。
2.土木这个词来源于拉丁文,原意是市民。
1782年,英国人JohnSmeaton用这个术语将非军事工程工作从在当时占绝大多数的军事工程师所人事的工程工作中区别开来。
从那以后,土木工程这个词常常用于表示建设公用设施的工程师们所人事的工作,尽管这个领域要宽广得多。
3.范围:因为它的面太广,所以土木工程被分成许多技术专业。
各专业的土木工程专家所需要的技能取决于工程项目的类型。
当一个项目开始时,场地被土木工程师所测绘,他们给定给水排水设施和电力线路的实际位置。
岩土工程专家们完成土壤实验来确定地基是否能承受工程项目的自重。
环境专家们研究项目对当地的影响:潜在的空气和地下水资源的污染,工程项目对当地动植物的影响,为满足保护环境的管理要求,怎样才能把工程项目设计好。
4.对于任何一个给定的工程项目,土地处理大量的用来制定工程最佳施工方法的数据。
5.结构工程.在这个专业中,土木工程师计划和设计各种各样的结构,包括桥,水坝,发电厂,设备的支柱,海岸工程的特殊结构,美国空间项目,发射塔,巨大的天文射电望远镜,和许多种其它的工程项目。
(完整版)土木工程专业英语翻译
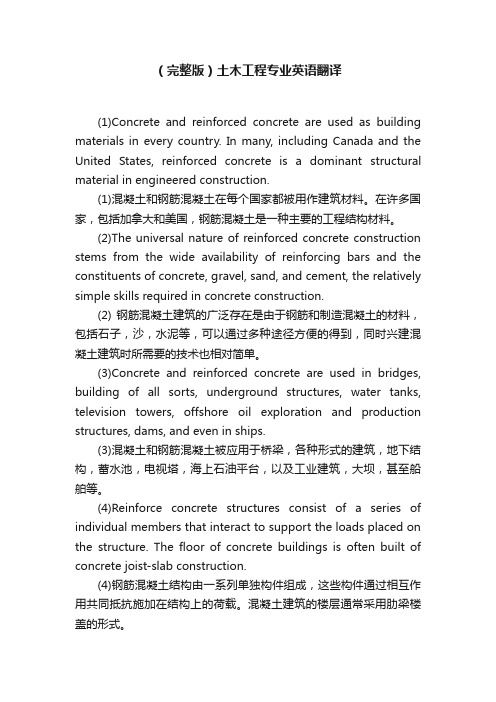
(完整版)土木工程专业英语翻译(1)Concrete and reinforced concrete are used as building materials in every country. In many, including Canada and the United States, reinforced concrete is a dominant structural material in engineered construction.(1)混凝土和钢筋混凝土在每个国家都被用作建筑材料。
在许多国家,包括加拿大和美国,钢筋混凝土是一种主要的工程结构材料。
(2)The universal nature of reinforced concrete construction stems from the wide availability of reinforcing bars and the constituents of concrete, gravel, sand, and cement, the relatively simple skills required in concrete construction.(2) 钢筋混凝土建筑的广泛存在是由于钢筋和制造混凝土的材料,包括石子,沙,水泥等,可以通过多种途径方便的得到,同时兴建混凝土建筑时所需要的技术也相对简单。
(3)Concrete and reinforced concrete are used in bridges, building of all sorts, underground structures, water tanks, television towers, offshore oil exploration and production structures, dams, and even in ships.(3)混凝土和钢筋混凝土被应用于桥梁,各种形式的建筑,地下结构,蓄水池,电视塔,海上石油平台,以及工业建筑,大坝,甚至船舶等。
土建英语作业(中英文对照翻译)
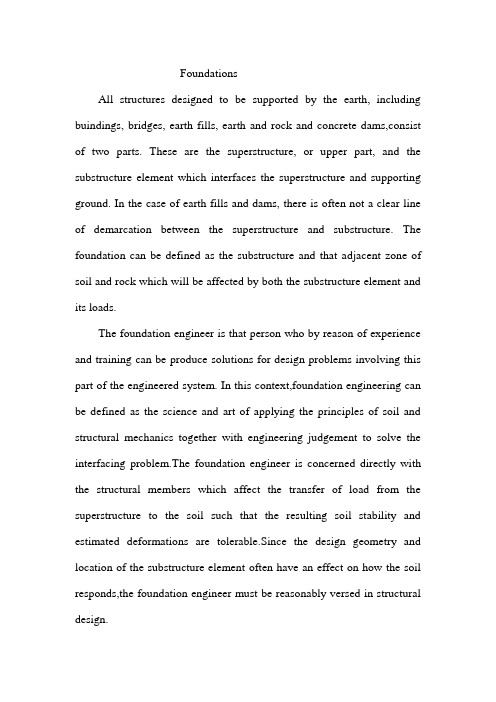
FoundationsAll structures designed to be supported by the earth, including buindings, bridges, earth fills, earth and rock and concrete dams,consist of two parts. These are the superstructure, or upper part, and the substructure element which interfaces the superstructure and supporting ground. In the case of earth fills and dams, there is often not a clear line of demarcation between the superstructure and substructure. The foundation can be defined as the substructure and that adjacent zone of soil and rock which will be affected by both the substructure element and its loads.The foundation engineer is that person who by reason of experience and training can be produce solutions for design problems involving this part of the engineered system. In this context,foundation engineering can be defined as the science and art of applying the principles of soil and structural mechanics together with engineering judgement to solve the interfacing problem.The foundation engineer is concerned directly with the structural members which affect the transfer of load from the superstructure to the soil such that the resulting soil stability and estimated deformations are tolerable.Since the design geometry and location of the substructure element often have an effect on how the soil responds,the foundation engineer must be reasonably versed in structural design.Foundations for structures such as buildings, from the smallest residential to the tallest high-rise, and bridges are for the purpose of transmitting the superstructure load. These loads come from column-type members with stress intensities rangeing from perhaps 140MPa for steel to 10MPa for concrete to the supporting capacity of the soil, which is seldom over 500kPa but more often on the order of 200 to 250kPa.Almost any reasonable structure can be built and safely supported if there is unlimited financing.Unfortunately, in the real situation this is seldom, if ever, the case, and the foundation engineer has the dilemma of making a decision under much less than the ideal condition, Also, even though the mistake may be buried, the results from the error are not and can show up relatively soon-and probably before any statute of limitations expires. These are reported cases where the foundation defects have shown up either during construction of superstructure or immediately thereafter.Since the superstructure is buried, or is beneath the superstructure, in such a configuration that access will be difficult, should foundation inadequacies develop after the superstructure is in place; it is common practice to be conservative. A one or two percent over design, in these areas produces a larger potential investment return than in the superstructure.The designer is always faced with the question of what constitutes asafe , economical design while simultaneously contending with the inevitable natural soil heterogeneity at a site. Nowadays that problem may be compounded by land scarcity requiring reclamation of areas which have been used as sanitary landfills, garbage dumps, or even hazardous waste disposal areas. Still another complicating factor is that the act of construction can alter the soil properties considerably from those used in the initial analyse of the foundation.These factors result in foundation design becoming so subjective and difficult to quantify that two design firms might come up with completely different designs which would perform equally satisfactory. Cost would likely be the distinguishable feature for the preferred design.This problem and the widely differing solutions would depend, for example, on the folling.1)What constitutes satisfactory and tolerable settlement ; how much extra could, or should , be spent to reduce estimated settlements from say 30 to 15mm?2)Has the client been willing to authorize an adequate soil exploration program?what kind of soil variability did the soil borings indicate?Would additional borings actually improve the foundation recommendations?3)Can the buildings be supported by the soil using?4)What are the consequences of a foundation failure in term of public safety? What is the likelihood of a lawsuit if the foundation does notperform adequately?5)Is sufficient money available for the foundation? It is not unheard of that the foundation alone would cost so much that the project is not economically feasible. It may be necessary to abandon the site in favor of one where foundation costs are affordable?6)What is the ability of the local construction force ? It is hardly sensible to design an elaborate foundation if no one can built it, or if it is so different in design that the contractor includes a large "uncertainty" factor in the bid.7)What is the engineering ability of the foundation engineer? While this factor is listed last,this is not of least importance in economical design. Obviously engineers have different levels of capability just as in other professions and in the trades such as carpenters, electricians ,and painters.If the foundation fails because of any cost shaving, the client tends to be quickly lose appreciation for the temporary financial benefit which accrued. At this point , facing heavy damages and a lawsuit, the client is probably in the poorest mental state of all the involved parties. Thus, one should always bear in mind that absolute dollar economics may not produce good foundation engineering.The foundation engineer must look at the entire system,the building purpose, probable service-life loading, type of framing, soil profile,construction methods, and construction costs to arrive at a design that is consistent with the client needs and does not excessively degrade the environment . This must be done with a safety factor which produces a tolerable risk to both the public and the owner.Considering these several areas of uncertainty, it follows that risk and liability insurance for persons engaged in foundation engineering is very costly. In attempts to reduce these costs as well as produces a design , which could be obtained from several engineering firms there is active discussion of having the foundation engineer submit the proposed design to a broad of qualified engineers for a "peer review".中文翻译:地基基础所有由土基础的结构,包括建筑物,桥梁,土堤,土石坝和混凝土坝,都由两部分组成,它们是上部结构或者上面的部分和下部结构单元,下部结构介于上部结构和支撑地层之间。
土木工程专业英语课后习题翻译.doc

(2) Cement gel 水泥凝胶体(4) The stability of the structure 结构稳定 (6) Moisture content 含水量LESSON 4 2、 Translate the following phrases into Chinese /English ・(1) Sustained lotid 长期荷载 (3) Water-cement ratio 水灰比(5) The expansion joint 伸缩缝(7) Cement paste 水泥浆 (8) The coefficient of thermal expansion of concrete 混凝土热膨胀系(9) Pernkinent plastic strain 永久塑性应变(10) The fatigue strength of concrete 混凝土疲劳强度3、Translate the fol lowing sentenee into Chinese ・(l) The cause of the volume changes in concrete can be attributed to changes in moisture content, chemicalretiction of the cement with water, variation in temperature, and applied loads ・ 混凝土的体积变化的原因可以归结为水分含最,水泥和水的化学反应,温度以及施加荷载的变化。
(2) High 一early 一strength and low-heat cement show more shrinkage than nonnal Portland cement. 高早强和低热水泥显示超过普通硅酸盐水泥收缩。
(3) The greater the aggregate content, the snitiller is the shrinkage ・ 骨料含量越大,收缩越小。
土木工程专业英语课后作业翻译
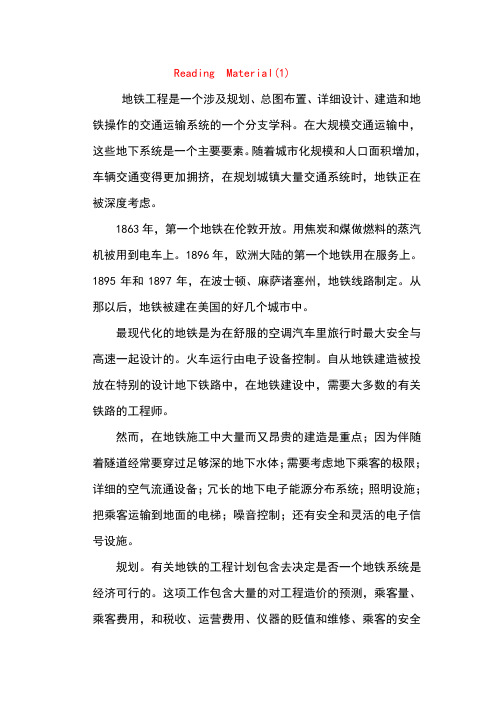
Reading Material(1)地铁工程是一个涉及规划、总图布置、详细设计、建造和地铁操作的交通运输系统的一个分支学科。
在大规模交通运输中,这些地下系统是一个主要要素。
随着城市化规模和人口面积增加,车辆交通变得更加拥挤,在规划城镇大量交通系统时,地铁正在被深度考虑。
1863年,第一个地铁在伦敦开放。
用焦炭和煤做燃料的蒸汽机被用到电车上。
1896年,欧洲大陆的第一个地铁用在服务上。
1895年和1897年,在波士顿、麻萨诸塞州,地铁线路制定。
从那以后,地铁被建在美国的好几个城市中。
最现代化的地铁是为在舒服的空调汽车里旅行时最大安全与高速一起设计的。
火车运行由电子设备控制。
自从地铁建造被投放在特别的设计地下铁路中,在地铁建设中,需要大多数的有关铁路的工程师。
然而,在地铁施工中大量而又昂贵的建造是重点;因为伴随着隧道经常要穿过足够深的地下水体;需要考虑地下乘客的极限;详细的空气流通设备;冗长的地下电子能源分布系统;照明设施;把乘客运输到地面的电梯;噪音控制;还有安全和灵活的电子信号设施。
规划。
有关地铁的工程计划包含去决定是否一个地铁系统是经济可行的。
这项工作包含大量的对工程造价的预测,乘客量、乘客费用,和税收、运营费用、仪器的贬值和维修、乘客的安全的估计的综合分析。
施工。
往往很难安装,地铁的施工明挖法和暗挖法同时存在。
运用明挖法时,一个基坑需要被挖掘。
这经常需要在基坑的每一边搭建支撑杆,另外不同的层面需要大的交叉框架。
敞开的基坑程序限制了工作可能完成的深度。
暗挖法允许地铁安置在很深的地方。
但是由于土的类型或者说所建造的地基的物质,不稳定的状况,巨大的水流量可能会造成运营很昂贵。
地铁的最佳位置的确定需要更仔细研究和考虑的各种植物,包括基础。
在一些大城市地区把地铁建在中央商务区下面和地上的其它地方。
在芝加哥南部的地铁被放在一条州际公路在相对地面公路上中央分隔带的交通空间。
在另一个芝加哥线从中央商务区领导西部,地铁是建在国会街高速公路中央分隔带下面。
土木工程英文翻译
【例1.1】Civil engineering offers a particular challenge , because almost every structure orsystem that is designed and built by civil engineers is unique.One structure rarely duplicatesanother exactly.译文:土木工程提出了特殊的挑战,因为由土木工程师设计建造的每个结构或系统几乎都是唯一的。
一个结构几乎不能完全复制另一个【例1.5】If the structure is saved or returned to its original state , additional foundationsupport must be provided.译文:假如建筑物要加以补救或恢复原貌,对基础做支护加固则是非常必要的。
为了使句子简洁精炼,专业英语中大量使用不定式.动名词、分词。
【例1.6】The total weight being less , it is possible to build much taller buildings.译文:由于总重量减轻,就有可能建造更高的楼房。
【例1.7】All the material forming the crust of the earth likely to be affected by the pressureof structures is divided by engineers into two major groups : rocks and soils.译文:受建筑物压力作用的地壳材料被工程人员分成两组,即岩石和土。
【例1.8】Compared with structural materials , such as steel and timber , soil is difficult to investigate scientifically.译文:与钢材、木材等建筑材料相比较,土研究起来颇为困难。
土木工程专业外语 课文翻译及课后练习答案
专业英语课文翻译Lesson 4Phrases and Expressions1.moisture content 含水量,含湿度; water content 2.cement paste 水泥浆 mortar 3.capillary tension 毛细管张力,微张力 4.gradation of aggregate 骨料级配 coarse fine (crushed stone, gravel) 5.The British Code PC100 英国混凝土规范PC100; nowaday BS8110 6. coefficient of thermal expansion of concrete 混凝土热膨胀系数 7. The B.S Code 英国标准规范 8. sustained load 永久荷载,长期荷载 9. permanent plastic strain 永久的塑性应变 stress 10. crystal lattice 晶格, 晶格 11. cement gel 水泥凝胶体 12. water-cement ratio 水灰比 13. expansion joint 伸缩缝 14. stability of the structure 结构的稳定性 structural stability15. fatigue strength of concrete 混凝土的疲劳强度Volume Changes of ConcreteConcrete undergoes volume changes during hardening. 混凝土在硬结过程中会经历体积变化。
If it loses moisture by evaporation, it shrinks, but if the concrete hardens in water, it expands. 如果蒸发失去水分,混凝土会收缩;但如果在水中硬结,它便膨胀。
土木工程专业英语译文1
1Careers in Civil Engineering土木工程专业Engineering is a profession, which means that an engineer must have a specialized university education. (工程是一个专业,这就是说一个工程师必须受过专业的大学教育) Many government jurisdictions also have licensing procedures which require engineering graduates to pass an examination, similar to the bar examination for a lawyer, before they can actively start on their careers. (许多政府行政区还有签发资格认可的程序,要求工科毕业生在充满自信地开始他们的职业生涯以前要通过一次考试,就象律师必须通过律师资格考试一样)In the university, mathematics, physics, and chemistry are heavily emphasized throughout the engineering curriculum, but particularly in the first two or three years. (在大学里,特别是头二、三年,数学、物理、化学时被重点强调的工科课程) Mathematics is very important in all branches of engineering, so it is greatly stressed. (在所有工程分支中数学都非常重要,所以一向特别强调它) Today, mathematics includes courses in statistics, which deals with gathering, classifying, and using numerical data, or pieces of information. (现在数学课程包括统计学,它是一门研究数据、一些信息的收集、分类和使用的课程) An important aspect of statistical mathematics is probability, which deals with what may happen when there are different factors, or variables, that can change the results of a problem. (统计数学的一个重要部分是概率论,他是研究不同因子或变量对问题所产生的各种结果发生的可能性大小的学科) Before the construction of a bridge is undertaken for example,a statistical study is made of the amount of traffic the bridge will be expected to handle. (例如,在建设一座桥梁前,要对它可能承担的交通量进行一次统计研究) In the design of the bridge, variables such as water pressure on the foundation, impact, the effects of different wind forces, and many other factors must be considered. (在设计这座桥梁时,必须考虑到各个变量,如作用于基础上的水压、冲力、不同风力的影响以及许多其它因素)Because a great deal of calculation is involved in solving these problems, computer programming is now included in almost all engineering curricula. (因为解决这些问题需要进行大量的计算,所以目前计算机程序编制已列入几乎所有工科的课程中) Computers, of course, can solve many problems involving calculations with greater speed and accuracy than a human being can. (诚然计算机能比人更快、更精确地解决许多需要计算的问题) But computers are useless they are given clear and accurate instructions and information-in other words, a good program. (但是除非给他们清楚而准确的指令和信息——换而言之,就是编制良好的程序,否则计算机就毫无用处) In spite of the heavy emphasis on technical subjects in the engineering curriculum, a current trend is to require students to take courses in the social science and the language arts. (尽管在工科的课程设置中重点应放在技术科目上,但是当前的一个趋势还是要求学生学习一些社会科学和语言艺术方面的课程) The relationship between engineering and society is getting closer; it is sufficient, therefore, to say again that the work performed by an engineer affects society in many different and important ways that he or she should be aware of. (工程和社会之间的关系越来越密切,因此有充分理由再次提出,一个工程师的工作在所通晓的许多不同而且重要的方面影响着社会) An engineer also needs a sufficient command of language to be able to prepare reports that are clear and, in many cases, persuasive. (一个工程师还需要能自如地运用语言,能写出条理清楚并在许多情况下具有说服力的报告) An engineer engaged in research will need to able to write up his or her findings for scientific publications. (从事科学研究的工程师要能将他(她)的科研成果写成文章提供给科学刊物)The last two years of an engineering program include subjects within the student’s field of specialization. (最后两年的工科教学计划包括学生所学专业领域内的课程) For the student who is preparing to become a civil engineer, these specialized courses may deal with such subjects as geodetic surveying, soil mechanics, or hydraulics. (对将要成为土木工程师的大学生来说,这些专业课程可能涉及到大地测量、土力学或水力学)Active recruiting for engineers often begins before the student’s last year in the university. (现行的工程师招聘往往在大学生最后一年前就开始进行) Many different corporation and government agencies have competed for the services of engineers in recent years. (近年来,许多不同公司和政府机构竞相争取录用工程师) In the science-oriented society of today, people who have technical training are, of course, in demand. (在当今这个重视科学的社会,当然需要受过技术培训的人才) Young engineers may choose to go into environmental or sanitary engineering, for example, where environmental concerns have created many openings; or they may prefer choose construction firms that specialize in highway work,or they may to work with one of the government agencies that deal with water resource. (年轻的工程师可能选择从事环境或卫生工程,例如环境工程专业为他们提供了许多就业机会;他们也可能选择专门从事高速公路工程施工的工程公司,他们可能更愿意到与水资源有关的政府机构工作) Indeed, the choice is large and varied. (事实上,可供选择的机会是广泛的、多样的)When the young engineer has finally started actual practice, the theoretical knowledge acquired in the university must be applied. (当年轻的工程师最终开始实际的业务工作时,肯定要用到大学里学到的理论知识) He or she will probably be assigned at the beginning to work with a team of engineers. (他(她)在开始时可能被派去和一个工程师小组一起工作) Thus, on-the-job training can be acquired that will demonstrate his or her ability to translate theory into practice to the supervisors. (这样,就能获得实际工作的锻炼,使主管人了解他(她)将理论应用于实践的能力)The civil engineer may work in research, design, construction supervision, maintenance, or even in sales or management. (土木工程师可从事研究、设计、施工管理、维修甚至销售或经营工作) Each of these areas involves different duties, different emphases, and different uses of engineer’s knowledge and experience. (这些领域的每一种工作都有不同的职责、不同的重点和工程师的知识和经验的不同应用)Research is one of the most important aspects of scientific and engineering practice. (科学研究是科学和实践最重要的一个方面) A researcher usually works as a member of a team with other scientists and engineers. (一个科研工作者通常是和其它科学家和工程师一道工作,是小组的成员) He or she is often employed in a laboratory that is financed by government or industry. (他(她)往往在一个由政府或工业企业资助的实验室里工作) Areas of research connected with civil engineering include soil mechanics and soil stabilization techniques, and also the development and testing of new structural materials.( 与土木工程有关的研究领域包括土力学、土加固技术,以及新型结构材料的研制和试验)Civil engineering projects are almost always unique; that is, each has own problems and design features. (土木工程设计几乎都具有独特性,那就是各有其特有的问题和设计特点) Therefore, careful study is given to each project even before design work begins. (因此,甚至设计工作还没有开始之前就要对每项工程进行仔细的研究) The study includes a survey both of topography and subsoil features of the proposed site. (这些研究包括对拟建项目场址地形和地基土特征进行勘测) It also includes a consideration of possible alternatives, such as a concrete gravity dam or an earth-fill embankment dam. (研究还包括要考虑各种可供选择的方案,例如是选用混凝土重力坝还是填土堤坝) The economic factors involved in each of the possible alternatives must also be weighed. (对每种可能方案的经济因素也必须权衡) Today, a study usually includes a consideration of the environmental impact of the project. (现在,一项研究工作通常还包括要考虑这个项目对环境的影响) Many engineers, usually working as a team that includes surveyors, specialists in soil mechanics, and experts in design and construction, are involved in making these feasibility studies. (在进行这些可行性研究时要由许多工程师来完成。
土木工程专业英语翻译
1.1 许多天然物质,如粘土、砂子和岩石,甚至树枝和树叶都已经被用作建筑材料。
Many naturally occurring substances, such as clay, sand, wood and rocks, even twigs and leaves have been used to construct buildings.1.2 砖块是由窑中烧制材料作成的块体,通常由粘土或页岩制成,但也可由炉渣制成。
A brick is a block made of kiln-fired material, usually clay or shale, but also maybe of lower quality mud.1.3 与水混合后,水泥便发生水化反应,并最终形成像石头一样的材料。
After mixing, the cement hydrates and eventually hardens into a stone-like material.1.4 金属可用作大型结构的框架,也可用来装饰建筑物外表。
Metal is used as structural framework for larger buildings such as skyscrapers, or as an external surface covering.1.5 明亮的窗户不但能使光线进入建筑物,而且也能将恶劣气候隔绝于建筑物之外。
Clear windows provided humans with the ability to both let light into rooms while at the same time keeping inclement weather outside.2.1 材料的抗拉强度是一种广延性质,因此它并不因试件尺寸的不同而改变。
Tensile strength is an intensive property and, consequently, does not depend on the side of the test specimen.2.2 屈服强度是材料从弹性变形到塑性变形转化时的应力。
- 1、下载文档前请自行甄别文档内容的完整性,平台不提供额外的编辑、内容补充、找答案等附加服务。
- 2、"仅部分预览"的文档,不可在线预览部分如存在完整性等问题,可反馈申请退款(可完整预览的文档不适用该条件!)。
- 3、如文档侵犯您的权益,请联系客服反馈,我们会尽快为您处理(人工客服工作时间:9:00-18:30)。
桂林理工大学土木与建筑工程学院土木工程专业英语外文翻译,中文翻译姓名:马凤志专业:土木应用班级:10级9班学号:3100510939原文中文翻译The Influence of Concrete Compaction on the Strength of Concrete Filled Steel Tubes压实混凝土对混凝土强度的影响Lin-Hai HanSchool of Civil Engineering, Harbin University of Civil Engineering and Architecture, Haihe Road 202, PO Box 689, Harbin 150090, P.R. China韩林海,哈尔滨建筑大学,土木与建筑工程学院,海河路202号,邮政信箱,689,哈尔滨,150090 中国ABSTRACT: Tests on twenty-one concrete filled steel tubes to investigate the influence of compaction methods on the strength of concrete filled steel tubular members are reported.摘要:测试二十一钢管混凝土试验,研究了钢管对混凝土构件强度压实方法的影响报告。
Two parameters were investigated, including slenderness ratio and load eccentricity.对两个参数进行研究,包括长细比和荷载偏心。
It was found that better compaction of concrete resulted in higher values of the ultimate strength of concrete filled steeltubular members结果发现更好的压实混凝土,能够使钢管混凝土有更高的极限强度值。
The tests show the importance of good concrete compaction for concrete filled steel tubes测试显示良好的混凝土重要性压实的重要性为方钢管混凝土管。
Keywords: concretefilled steel tube, column, strength, deflection, concrete compaction关键字; 钢管混凝土,柱,强度,挠度,混凝土压实1. INTRODUCTION 引言Concrete filled steel tubular columns have been used extensively in Europe, Japan, Australia, China, as well as other countries.在欧洲,日本,澳大利亚,中国,以及其他国家钢管混凝土柱已广泛被使用。
They have proved to be economical in themselves as well as leading to rapid construction and thus additional cost savings.1他们已经是被证明是经济实惠的,同时也能促进快速建设和增加消费储蓄In the past, much progress has been made in studying the bond strength between the steel tube and its core concrete, studiesof how the concrete compaction method influences the strength of concrete filled steel tubular members have been seldom made.2–8在过去的研究中,钢管与核心混凝土之间的粘结强度有了很大进展,但对混凝土的压实方法对钢管混凝土构件强度的研究已经很少了。
2–8These and earlier results show that perhaps the most significant factor influencing the ultimate bond strength might be compaction and the consequent better keying of the concrete with the surface irregularities in the steel.这些和早期的结果表明,也许最重要的因素可能影响最终粘结强度压实以及更好地键控,因而混凝土与钢管中的表面成不规则性In Reference (2), specimens have been tested for different conditions likely to arise in the manufacture of concrete as follows:在参考(2)中,在制造混凝土过程中针对不同的条件下的标本已经过测试,如下所示:1.Aggregate/cement ratio 5.0, dry cured, well compacted witha poker vibrator;1,聚合/水泥比为5.0,干燥固化, 以及压实与插入式振捣器2.Aggregate/cement ratio 5.0, wet cured, well compacted witha poker vibrator;2,骨料/水泥比为5,湿固化,以及压实与插入式振捣器;3.Aggregate/cement ratio 5.5, wet cured, well compacted witha poker vibrator;3,总/水泥比为5.5,湿固化,以及压实与插入式振捣器4.Aggregate/cement ratio5.5, wet cured, lightly compactedwith a poker vibrator4,聚合/水泥比为5.5,湿固化,轻轻压实与插入式振捣器5.Aggregate/cement ratio 5.5, wet cured, well compacted byhand (3 layers with 40 strokes each);5,总/水泥比为5.5,湿固化,用手压实(3层,每组40次)6. Aggregate/cement ratio 5.5, wet cured, lightly compacted by hand (3 layers with 20 strokes each).6,粒料/水泥比5.5,湿固化,轻轻用手压实(3层,每组20次)The test results in Reference (2) show that specimens compacted by mechanical vibration developed higher bond strength than those compacted by hand.参考(2)显示,由机械振动碾压的标本中的测试结果比用手开发粘结强度更高也比那些压实的更高。
The aim of this paper is to investigate if the concrete compaction method influences the strength of concrete filled steel tubular members. Tests on several concrete filled steel tubes for establishing the relationship between strength andcompaction method are reported.本文件的目的是调查如果混凝土压实方法影响钢管混凝土构件的强度。
根据几个混凝土试验根据报告,钢管为建立强度和压实的方法之间的关系。
Several parameters, such as the slenderness ratio l (= 4L/D,L and D are the calculated length and the diameter of the member respectively), as well as the load eccentricity e were investigated.几个参数,如长细比 l (= 4 L/D、 L 和 D 分别计算的长度和直径的成员),以及负载偏心 e 进行了调查。
The tests show the importance of concrete compaction method on the strength of concrete filled steel tubular members. 试验表明,混凝土的压实方法对钢管混凝土构件强度的重要性。
2. EXPERIMENTAL PROGRAM2.实验程序The aim of the experimental study was to determine not only the maximum load capacity of the specimens, but also to investigate the behavorial pattern up to and beyond ultimate load.本实验研究的目的是确定不仅试样的最大负载能力,但也观察到的行为模式超越极限荷载。
The steel had a specified yield strength (f y) as shown in Table 1.该钢具有指定的屈服强度(FY),如表所示 1The concrete mix was designed for a compressive cube strength (f cu) at 28 days of approximately 46.7 MPa。
混凝土配合比设计为一个立方体抗压强度(FCU)在28天约46.7 MPa For each concrete mix batch used, three 100 mm cubes were also cast and cured in conditions similar to the related specimens. 每个使用的混凝土配合比批处理,三个 100 毫米的立方体也强制转换,并固化在条件类似的相关标本。