ATM和ATR的信号传导通路综述
细胞应激反应的

二、低氧导致的细胞应激反应
1.细胞低氧应激反应的感受器 氧感受器
辅酶II氧化酶。一种膜结合蛋白,可将O2转变为 H2O2。与低氧诱导因子-1 (HIF-1)的活化有关
脯氨酰羟化酶和天冬酰羟化酶正常氧浓度下将 HIF-1维持在一个较低水平或阻断HIF-1的作用
第9页/共34页
9
2024/8/5
2.低氧诱导因子-1
第20页/共34页
20
2024/8/5
氨基酸和葡萄糖缺乏应激的细胞效应
缺乏不严重且时间短时,通过应激反应将细胞 的代谢水平降到最低
缺乏严重时,诱导细胞凋亡
第21页/共34页
21
2024/8/5
第三节 全身应激反应的相关分子
一、急性期反应蛋白 二、热休克蛋白 三、冷休克蛋白
第22页/共34页
22
第11页/共34页
11
2024/8/5
3. 低氧应激的细胞反应
低氧刺激
↓
低氧感受器
↓
HIF-1a表达升高并转位入细胞核
↓
与HIF-1β结合成为有活性的HIF-1分子
↓
募集共激活因子CBP和TIF-2
↓
促进各种缺氧应激效应分子的表达
第12页/共34页
12
2024/8/5
4.其他细胞低氧应激效应分子的作用 Ca2+
第14页/共34页
14
2024/8/5
1.氧化应激的感应和应激信号通路的激活
ROS 活性氧,reactive oxygen species
主要指化学性能比氧活泼的含氧化合物包括:
OH·、O2、H2O2、ROO·、NO、ONOO·等
O-.2
ROS在细胞氧化应激引起的信号传导中的作用
ATR分子通路及其抑制剂抗肿瘤研究进展
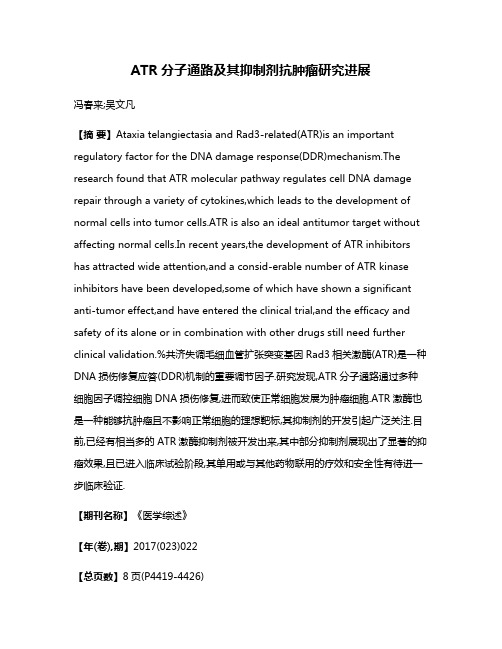
ATR分子通路及其抑制剂抗肿瘤研究进展冯春来;吴文凡【摘要】Ataxia telangiectasia and Rad3-related(ATR)is an important regulatory factor for the DNA damage response(DDR)mechanism.The research found that ATR molecular pathway regulates cell DNA damage repair through a variety of cytokines,which leads to the development of normal cells into tumor cells.ATR is also an ideal antitumor target without affecting normal cells.In recent years,the development of ATR inhibitors has attracted wide attention,and a consid-erable number of ATR kinase inhibitors have been developed,some of which have shown a significant anti-tumor effect,and have entered the clinical trial,and the efficacy and safety of its alone or in combination with other drugs still need further clinical validation.%共济失调毛细血管扩张突变基因Rad3相关激酶(ATR)是一种DNA损伤修复应答(DDR)机制的重要调节因子.研究发现,ATR分子通路通过多种细胞因子调控细胞DNA损伤修复,进而致使正常细胞发展为肿瘤细胞.ATR激酶也是一种能够抗肿瘤且不影响正常细胞的理想靶标,其抑制剂的开发引起广泛关注.目前,已经有相当多的ATR激酶抑制剂被开发出来,其中部分抑制剂展现出了显著的抑瘤效果,且已进入临床试验阶段,其单用或与其他药物联用的疗效和安全性有待进一步临床验证.【期刊名称】《医学综述》【年(卷),期】2017(023)022【总页数】8页(P4419-4426)【关键词】共济失调毛细血管扩张突变基因Rad3相关激酶分子通路;共济失调毛细血管扩张突变基因Rad3相关激酶抑制剂;肿瘤;靶点【作者】冯春来;吴文凡【作者单位】江苏大学药学院,江苏镇江212013;江苏大学药学院,江苏镇江212013【正文语种】中文【中图分类】R322DNA在外部环境和细胞内部的各种因素作用下可不断产生损伤,如体内代谢过程中产生的自由基、DNA在复制和重组过程中自发的错误、环境中的紫外线和离子辐射(ionizing radiation,IR)以及一些化学物质等均能引起DNA损伤,从而导致细胞死亡,有害突变影响细胞活力以及异常细胞行为[1]。
ATM和ATR的信号传导通路综述
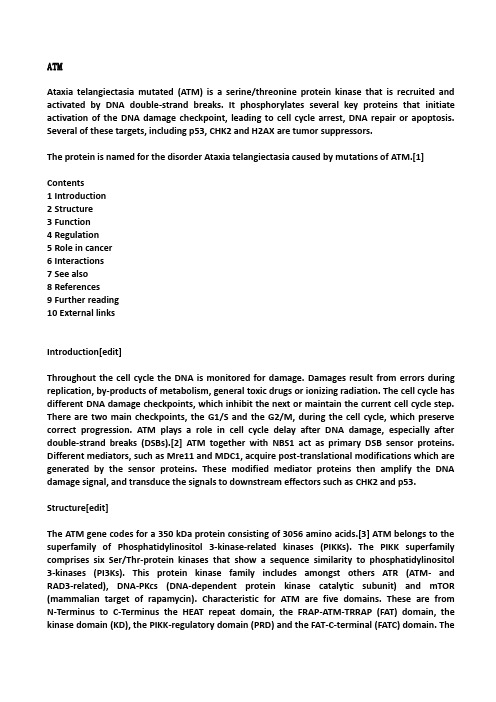
ATMAtaxia telangiectasia mutated (ATM) is a serine/threonine protein kinase that is recruited and activated by DNA double-strand breaks. It phosphorylates several key proteins that initiate activation of the DNA damage checkpoint, leading to cell cycle arrest, DNA repair or apoptosis. Several of these targets, including p53, CHK2 and H2AX are tumor suppressors.The protein is named for the disorder Ataxia telangiectasia caused by mutations of ATM.[1]Contents1 Introduction2 Structure3 Function4 Regulation5 Role in cancer6 Interactions7 See also8 References9 Further reading10 External linksIntroduction[edit]Throughout the cell cycle the DNA is monitored for damage. Damages result from errors during replication, by-products of metabolism, general toxic drugs or ionizing radiation. The cell cycle has different DNA damage checkpoints, which inhibit the next or maintain the current cell cycle step. There are two main checkpoints, the G1/S and the G2/M, during the cell cycle, which preserve correct progression. ATM plays a role in cell cycle delay after DNA damage, especially after double-strand breaks (DSBs).[2] ATM together with NBS1 act as primary DSB sensor proteins. Different mediators, such as Mre11 and MDC1, acquire post-translational modifications which are generated by the sensor proteins. These modified mediator proteins then amplify the DNA damage signal, and transduce the signals to downstream effectors such as CHK2 and p53.Structure[edit]The ATM gene codes for a 350 kDa protein consisting of 3056 amino acids.[3] ATM belongs to the superfamily of Phosphatidylinositol 3-kinase-related kinases (PIKKs). The PIKK superfamily comprises six Ser/Thr-protein kinases that show a sequence similarity to phosphatidylinositol 3-kinases (PI3Ks). This protein kinase family includes amongst others ATR (ATM- and RAD3-related), DNA-PKcs (DNA-dependent protein kinase catalytic subunit) and mTOR (mammalian target of rapamycin). Characteristic for ATM are five domains. These are from N-Terminus to C-Terminus the HEAT repeat domain, the FRAP-ATM-TRRAP (FAT) domain, the kinase domain (KD), the PIKK-regulatory domain (PRD) and the FAT-C-terminal (FATC) domain. TheHEAT repeats directly bind to the C-terminus of NBS1. The FAT domain interacts with ATM's kinase domain to stabilize the C-terminus region of ATM itself. The KD domain resumes kinase activity, while the PRD and the FATC domain regulate it. Although no structure for ATM has been solved, the overall shape of ATM is very similar to DNA-PKcs and is composed of a head and a long arm that is thought to wrap around double-stranded DNA after a conformational change. The entire N-terminal domain together with the FAT domain are predict ed to adopt an α-helical structure, which was found by sequence analysis. This α-helical structure is believed to form a tertiary structure, which has a curved, tubular shape present for example in the Huntingtin protein, which also contains HEAT repeats. FATC is the C-terminal domain with a length of about 30 amino acids. It is highly conserved and consists of an α-helix followed by a sharp turn, which is stabilized by a disulfide bond.[4]Function[edit]A complex of the three proteins Mre11, RAD50 and NBS1 (Xrs2 in yeast), called the MRN complex in humans, recruits ATM to double strand breaks (DSBs) and holds the two ends together. ATM directly interacts with the NBS1 subunit and phosphorylates the histone variant H2AX on Ser139.[5] This phosphorylation generates binding sites for adaptor proteins with a BRCT domain. These adaptor proteins then recruit different factors including the effector protein kinase CHK2 and the tumor suppressor p53. The ATM-mediated DNA damage response consists of a rapid and a delayed response. The effector kinase CHK2 is phosphorylated and thereby activated by ATM. Activated CHK2 phosphorylates phosphatase CDC25A, which is degraded thereupon and can no longer dephosphorylate CDK2-Cyclin, resulting in cell-cycle arrest. If the DSB can not be repaired during this rapid response, ATM additionally phosphorylates MDM2 and p53 at Ser15.[6] p53 is also phosphorylated by the effector kinase CHK2. These phosphorylation events lead to stabilization and activation of p53 and subsequent transcription of numerous p53 target genes including Cdk inhibitor p21 which lead to long-term cell-cycle arrest or even apoptosis.[7]ATM-mediated two-step response to DNA double strand breaks. In the rapid response activated ATM phosphorylates effector kinase CHK2 which phophphorylates CDC25A, targeting it for ubiquitination and degradation. Therefore phosphorylated CDK2-Cyclin accumulates and progression through the cell cycle is blocked. In the delayed response ATM phosphorylates the inhibitor of p53, MDM2, and p53, which is also phosphorylated by Chk2. The resulting activation and stabilization of p53 leads to an increased expression of Cdk inhibitor p21, which further helps to keep Cdk activity low and to maintain long-term cell cycle arrest.[7]The protein kinase ATM may also be involved in mitochondrial homeostasis, as a regulator of mitochondrial autophagy (mitophagy) whereby old, dysfunctional mitochondria are removed.[8] Regulation[edit]A functional MRN complex is required for ATM activation after double strand breaks (DSBs). The complex functions upstream of ATM in mammalian cells and induces conformational changes that facilitate an increase in the affinity of ATM towards its substrates, such as CHK2 and p53.[2] Inactive ATM is present in the cells without DSBs as dimers or multimers. Upon DNA damage, ATMautophosphorylates on residue Ser1981. This phosphorylation provokes dissociation of ATM dimers, which is followed by the release of active ATM monomers.[9] Further autophosphorylation (of residues Ser367 and Ser1893) is required for normal activity of the ATM kinase. Activation of ATM by the MRN complex is preceded by at least two steps, i.e. recruitment of ATM to DSB ends by the mediator of DNA damage checkpoint protein 1 (MDC1) which binds to MRE11, and the subsequent stimulation of kinase activity with the NBS1 C-terminus. The three domains FAT, PRD and FATC are all involved in regulating the activity of the KD kinase domain. The FAT domain interacts with ATM's KD domain to stabilize the C-terminus region of ATM itself. The FATC domain is critical for kinase activity and highly sensitive to mutagenesis. It mediates protein-protein interaction for example with the histone acetyltransferase TIP60 (HIV-1 Tat interacting protein 60 kDa), which acetylates ATM on residue Lys3016. The acetylation occurs in the C-terminal half of the PRD domain and is required for ATM kinase activation and for its conversion into monomers. While deletion of the entire PRD domain abolishes the kinase activity of ATM, specific small deletions show no effect.[4]Role in cancer[edit]Ataxia telangiectasia (AT) is a rare human disease characterized by cerebellar degeneration, extreme cellular sensitivity to radiation and a predisposition to cancer. All AT patients contain mutations in the ATM gene (ATM). Most other AT-like disorders are defective in genes encoding the MRN protein complex. One feature of the ATM protein is its rapid increase in kinase activity immediately following double-strand break formation.[10][11] The phenotypic manifestation of AT is due to the broad range of substrates for the ATM kinase, involving DNA repair, apoptosis, G1/S, intra-S checkpoint and G2/M checkpoints, gene regulation, translation initiation, and telomere maintenance.[12] Therefore a defect in ATM has severe consequences in repairing certain types of damage to DNA, and cancer may result from improper repair. AT patients have an increased risk for breast cancer that has been ascribed to ATM's interaction and phosphorylation of BRCA1 and its associated proteins following DNA damage.[13] Certain kinds of leukemias and lymphomas, including Mantle cell lymphoma, T-ALL, atypical B cell chronic lymphocytic leukemia, and T-PLL are also associated with ATM defects.[14]Interactions[edit]Ataxia telangiectasia mutated has been shown to interact with RAD17,[15][16] RBBP8,[15][17] RAD51,[18] DNA-PKcs,[15][19] RRM2B,[20] FANCD2,[21][22] Nibrin,[15][23] TERF1,[24] BRCA1,[13][15][23][25][26][27][28] Abl gene,[18][24][29] TP53BP1,[30][31] MRE11A,[15][23] P53,[15][32][33][34][35] Bloom syndrome protein,[23][36] SMC1A[37] and RHEB.[38]。
ATM蛋白激酶依赖性信号转导通路研究进展
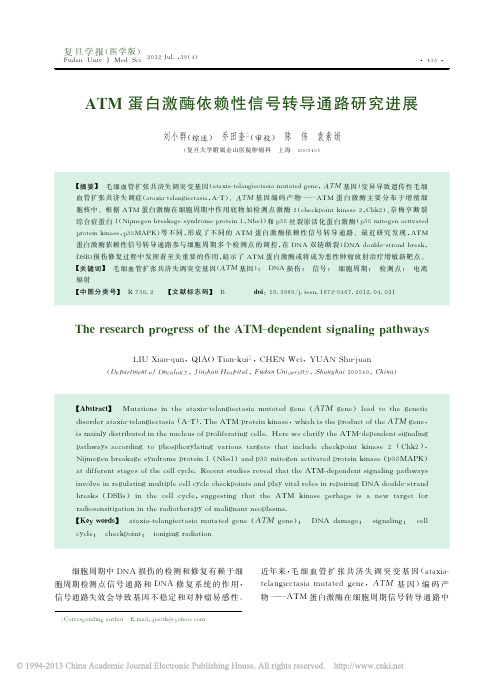
细胞周期中 DNA 损伤 的 检 测 和 修 复 有 赖 于 细 胞周期检测 点 信 号 通 路 和 DNA 修 复 系 统 的 作 用, 信号通路失效会导致基因不稳定和对肿瘤易感性。
近年来,毛 细 血 管 扩 张 共 济 失 调 突 变 基 因 (ataxia- telangiectasia mutated gene,ATM 基 因 )编 码 产 物———ATM 蛋白激酶在细 胞 周 期 信 号 转 导 通 路 中
具体的来说当真核生物细胞受到电离或紫外线辐射所致dna双链损伤时首先需要识别断裂点然后atm蛋白二聚体分子内自动磷酸化并分离成活性的atm蛋白单体诱发下游相应底物发生一系列复杂的磷酸化级联反应最终引起细胞周期如g1dna修复或凋亡阻止错误的遗传信息向子代细胞传递以维持染色体的稳定性和防止恶变
复 旦 学 报 (医 学 版 )
Fudan Univ J Med Sci 2012Jul.,39(4)
细胞信号传导

细胞信号传导细胞信号传导是一种复杂的过程,涉及细胞内和细胞间信息的传递和处理。
信号传导可以通过细胞表面的受体,细胞内的信号转导通路以及细胞间的信号分子进行。
这一过程对于维持生命活动的正常进行至关重要。
一、细胞表面受体的信号传导细胞表面的受体是细胞感知外界信号的主要结构。
主要包括离子通道受体、酪氨酸激酶受体和七膜跨膜受体(G蛋白偶联受体)。
离子通道受体通过调节细胞膜上的离子通道的开启和关闭来传导信号。
酪氨酸激酶受体则通过受体二聚化以及激酶活化来传递信号。
七膜跨膜受体则与G蛋白偶联,通过激活或抑制下游效应分子来传导信号。
二、细胞内信号转导通路的传导一旦受体被激活,信号将通过细胞内的信号转导通路进行传递。
这一过程涉及多种信号分子的相互作用和酶的活化。
例如,当酪氨酸激酶受体被激活后,它会自磷酸化并激活下游信号分子。
这些信号分子会进一步激活一系列的酶级联反应,最终导致信号的扩散和转导。
三、细胞间信号分子的传导细胞间信号分子可以通过细胞间的直接接触或者释放到周围环境中进行传导。
细胞间结合蛋白、细胞间通道以及神经递质都是常见的细胞间信号分子。
这些分子在信号传导中起到桥梁的作用,可以直接传递信息,激活或抑制接受细胞的相应反应。
四、细胞信号传导的调控与调节细胞信号传导过程中的调节和调控非常重要,可以确保信号传导的准确性和平衡性。
细胞内的负调控机制可以通过降解信号分子、阻断通路中的关键酶活性或阻止信号分子与受体的结合来抑制信号传导。
每种细胞信号传导通路都具有精密的调控系统,以确保信号在特定条件下的精确传导。
总结:细胞信号传导是一种复杂而精密的过程,涉及多种信号分子和酶的相互作用。
通过细胞表面受体的激活和细胞内信号转导通路的传递,信号可以被传递到目标细胞或组织,触发特定的生理或病理反应。
细胞信号传导的研究有助于理解疾病的发生机制,并为开发新的治疗方法提供指导。
概述受体酪氨酸激酶介导的信号通路的特点和主要功能

概述受体酪氨酸激酶介导的信号通路的特点和主要功能
受体酪氨酸激酶(RTKS)是细胞表面一大类重要受体家族,当配体与受体结合,导致受体二聚化,激活受体的酪氨酸蛋白激酶活性,随即引起一系列磷酸化级联反应,终至细胞生理和基因表达的改变.RTK-Ras信号通路是这类受体所介导的重要信号通路.其基本模式为:配体→RTK→接头蛋白→GEF →Ras →Raf (MAPKKK) →MAPKK →MAPK →进入细胞核→其他激酶或基因调控蛋白(转录因子)的磷酸化修饰,对基因表达产生多种效应.\x0d组成:该受体家族包括6个亚族.其胞外配体为可溶性或膜结合的多肽或蛋白类激素.还有RTK-Ras 信号通路中各种因子.\x0d特点:(1)激活机制为受体之间的二聚化、自磷酸化、活化自身;(2)没有特定的二级信使,要求信号有特定的结构域;(3)有Ras分子开关的参与;(4)介导下游MAPK的激活\x0d功能:RTKS信号通路主要参与控制细胞生长、分化过程.RTK-Ras信号通路具有广泛的功能,包括调节细胞的增殖分化,促进细胞存活,以及细胞代谢的调节与校正。
细胞信号传导途径研究论文素材
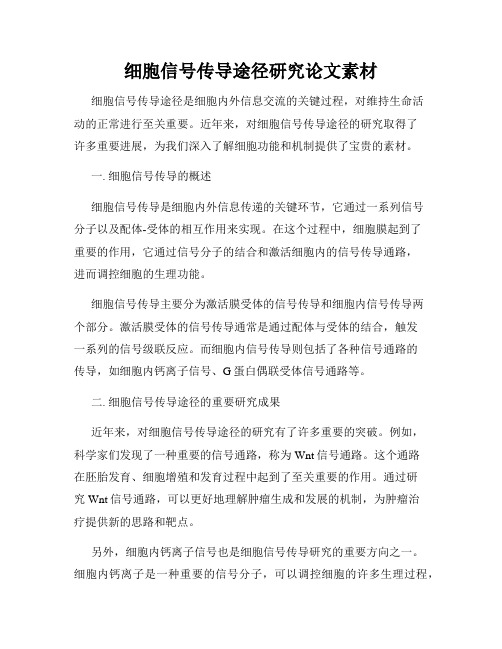
细胞信号传导途径研究论文素材细胞信号传导途径是细胞内外信息交流的关键过程,对维持生命活动的正常进行至关重要。
近年来,对细胞信号传导途径的研究取得了许多重要进展,为我们深入了解细胞功能和机制提供了宝贵的素材。
一. 细胞信号传导的概述细胞信号传导是细胞内外信息传递的关键环节,它通过一系列信号分子以及配体-受体的相互作用来实现。
在这个过程中,细胞膜起到了重要的作用,它通过信号分子的结合和激活细胞内的信号传导通路,进而调控细胞的生理功能。
细胞信号传导主要分为激活膜受体的信号传导和细胞内信号传导两个部分。
激活膜受体的信号传导通常是通过配体与受体的结合,触发一系列的信号级联反应。
而细胞内信号传导则包括了各种信号通路的传导,如细胞内钙离子信号、G蛋白偶联受体信号通路等。
二. 细胞信号传导途径的重要研究成果近年来,对细胞信号传导途径的研究有了许多重要的突破。
例如,科学家们发现了一种重要的信号通路,称为Wnt信号通路。
这个通路在胚胎发育、细胞增殖和发育过程中起到了至关重要的作用。
通过研究Wnt信号通路,可以更好地理解肿瘤生成和发展的机制,为肿瘤治疗提供新的思路和靶点。
另外,细胞内钙离子信号也是细胞信号传导研究的重要方向之一。
细胞内钙离子是一种重要的信号分子,可以调控细胞的许多生理过程,例如细胞的增殖、凋亡、分化等。
通过研究细胞内钙离子信号传导的途径,可以深入了解这些生理过程的调控机制,并为相关疾病的治疗提供新的思路。
三. 细胞信号传导途径的应用前景对细胞信号传导途径的研究不仅有助于我们深入了解细胞的机制,还有广泛的应用前景。
例如,通过干扰特定的信号通路,可以干预肿瘤细胞的生长和扩散,为肿瘤治疗提供新的策略。
同时,了解细胞信号传导途径的机制,也有助于我们开发新的药物和治疗方法,以应对其他相关疾病的挑战。
细胞信号传导途径研究的素材十分丰富多样,科学家们正积极开展相关研究,为我们揭示细胞信号传导的奥秘提供更多的线索。
相信在不久的将来,通过研究细胞信号传导途径,我们将能够更好地理解细胞的功能和机制,为生命科学领域的发展贡献更多的力量。
细胞周期调控和DNA损伤的关系及其作用机制
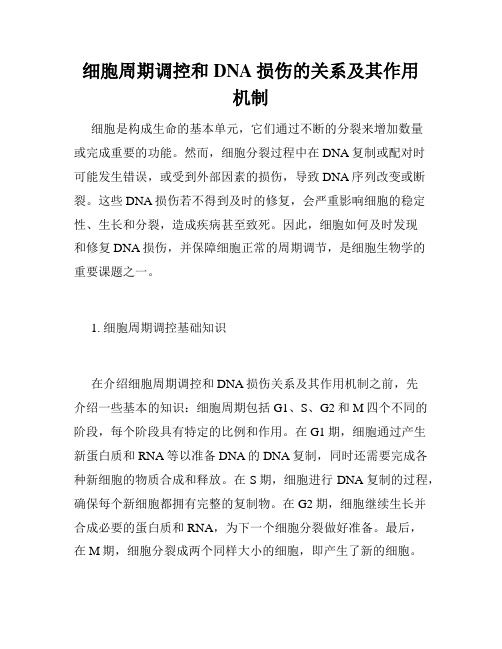
细胞周期调控和DNA损伤的关系及其作用机制细胞是构成生命的基本单元,它们通过不断的分裂来增加数量或完成重要的功能。
然而,细胞分裂过程中在DNA复制或配对时可能发生错误,或受到外部因素的损伤,导致DNA序列改变或断裂。
这些DNA损伤若不得到及时的修复,会严重影响细胞的稳定性、生长和分裂,造成疾病甚至致死。
因此,细胞如何及时发现和修复DNA损伤,并保障细胞正常的周期调节,是细胞生物学的重要课题之一。
1. 细胞周期调控基础知识在介绍细胞周期调控和DNA损伤关系及其作用机制之前,先介绍一些基本的知识:细胞周期包括G1、S、G2和M四个不同的阶段,每个阶段具有特定的比例和作用。
在G1期,细胞通过产生新蛋白质和RNA等以准备DNA的DNA复制,同时还需要完成各种新细胞的物质合成和释放。
在S期,细胞进行DNA复制的过程,确保每个新细胞都拥有完整的复制物。
在G2期,细胞继续生长并合成必要的蛋白质和RNA,为下一个细胞分裂做好准备。
最后,在M期,细胞分裂成两个同样大小的细胞,即产生了新的细胞。
细胞周期调控是一种高级形式的基因表达调控,它通过多个信号途径和分子机制来确保细胞在适当的时刻进入下一个周期,并避免过度分裂或紊乱。
细胞周期调控可以分为内部和外部两种调节方式。
内部调控是由细胞内信号传导通路和蛋白激酶等分子机制来控制的。
外部调控则是受细胞外的生长因素和讯息(比如细胞凋亡)的影响,从而调节细胞周期的进程。
2. DNA损伤的类型和影响DNA损伤是指DNA序列的改变或断裂,这种损伤可以发生在DNA分子的位置(单各核苷酸变异、缺失或增加)或结构(单链或双链断裂)。
环境因素或内源性生理原因都可能会导致DNA损伤,包括直接暴露于毒物、辐射和化学物质等。
另外,DNA损伤可能由一些生理性过程引起,包括细胞呼吸和新陈代谢等。
DNA损伤如果没有被及时修复,会导致染色体缺失、嵌合、倒位等恶性事件。
如果细胞无法检测到或修复这些问题,就会引发DNA复制错误、增加癌症的风险,以及导致细胞凋亡或死亡。