Orientation selectivity in cat primary visual cortex local and global measurement
CorvisST评估高度近视患者角膜生物力学参数及其相关因素

Corvis ST 评估高度近视患者角膜生物力学参数及其相关因素沈如月 叶聪 梁远波 王小洁 周堃 吕帆作者单位:温州医科大学附属眼视光医院 325027第一作者:沈如月(ORCID :0000-0003-1771-5371),Email :shenruyue1992@ 通信作者:吕帆(ORCID :0000-0002-5262-8110),Email :lufan@ 摘要目的:利用Corvis ST 评估高度近视患者角膜生物力学参数及其相关因素。
方法:横断面研究。
选取2017年6-10月就诊于温州医科大学附属眼视光医院的高度近视患者53例,其中男17例,女36例,年龄18~53(33.0±9.6)岁,均取右眼检测数据。
应用Corvis ST 测量角膜生物力学参数,包括第 1次压平速率(A1V )、长度(A1L );第2次压平速率(A2V )、长度(A2L )以及最大凹陷位置的形变幅度(DA )、曲率半径(HCR )、顶点距离(PD )。
比较高度近视患者(-6~-10 D ,23眼)和超高度近视患者(≤-10 D ,30眼)角膜生物力学参数的差异,并分析各参数与年龄、眼压、眼轴长度(AL )、中央角膜厚度(CCT )及视网膜神经纤维层(RNFL )厚度的相关性。
数据采用独立样本t 检验、Pearson 相关进行分析。
结果:高度近视组与超高度近视组之间的A2L 差异具有统计学意义(t =1.95,P =0.043)。
双变量相关分析结果显示眼压与A1V 、PD 、DA 呈负相关(r =-0.56、-0.46、-0.63,P <0.001),与A2V 呈正相关(r =0.37,P =0.005);PD 与AL 呈正相关(r =0.43,P =0.001)。
A1L 、A2L 、A2V 与CCT 呈正相关(r =0.33,P =0.043;r =0.28,P =0.041;r =0.39,P =0.003);角膜压平范围与下方RNFL 厚度呈负相关(r =-0.45,P =0.001)。
细胞生物学C名词解释

细胞生物学C名词解释【1】细胞膜与物质的转运一、名词解释1. cell membrane(细胞膜)也称质膜,是指包围在细胞质外周的一层由蛋白质、脂类和糖类等物质组成的生物膜。
2. biological membrane/biomembrane(生物膜)细胞膜和细胞内各种膜性结构的统称。
3. unit membrane(单位膜)不同的生物膜在电镜下呈现一种较为一致的“两暗夹一明”的3层结构,即电子密度较高的内外两层(2nm×2)夹着电子密度较低的中间层(3.5nm)。
4. amphipathic molecule(两亲性分子/双亲媒性分子)像磷脂分子那样的,既具有亲水的极性头部,又具有疏水的非极性尾部的分子。
5. head group(头部基团)磷脂分子中亲水的小基团位于分子的末端与带负电的磷酸基团一起形成的高度水溶性的结构域,极性很强。
6. integral/intrinsic/transmenbraneprotein(整合/内在/穿膜蛋白)有的膜蛋白通过α-螺旋(也有β-片层)一次或多次穿膜而镶嵌在脂双层中。
7. extrinsic/peripheral protein(外在/周边蛋白)是一类与细胞膜结合疏松(非共价键)、不插入脂双层的蛋白质,分布于质膜的胞内侧或胞外侧。
8. lipid anchored/linked protein(脂锚定/连接蛋白)与外在蛋白类似位于膜的两侧、不穿膜,但以共价键和脂双层中的脂质分子结合。
9. the fluidity of cell membrane(细胞膜的流动性)是指构成细胞膜的膜脂和膜蛋白处于不断的运动状态,是保证细胞正常功能的重要条件。
10. liposome(脂质体)脂质分子在水相中会自发形成脂质双分子层。
为了避免其两端疏水尾部和水的接触,游离端往往可以闭合形成一种自我封闭的稳定的中空结构,称为脂质体。
11. phase transition(相变)由于温度的改变导致膜状态的改变。
大脑的奥秘:神经科学导论答案

大脑的奥秘:神经科学导论答案大脑的奥秘:神经科学导论答案1.1脑与外部世界1【判断题】某些癫痫病人由于外科手术而成为裂脑人,因此他们的大脑可以相互独立工作。
(?)2【判断题】人类的一些高级认知过程都和心脏有关,是心与脑的共同表现形式。
(?)3【判断题】所有具备生命特征的动物都有大脑。
(?)1.2脑科学的应用1【单选题】现代科学技术可以用(C)来控制神经细胞的反应,控制特定的大脑核团。
A、磁波B、信号C、光D、声音2【判断题】别人没有任何方法破解深藏于内心的秘密。
(?) 3【判断题】不同的人脑功能上的显著差别来源于其不同的文化的宗教背景。
(?)4【判断题】大脑的每个半球都包括大脑皮层。
(?)5【判断题】大脑皮层是高级神经活动的物质基础。
(?)1.3打开大脑的“黑盒子”1【单选题】(A)是人脑的最大部分。
A、端脑B、间脑C、中脑D、后脑2【单选题】(C)检测的是血液中含氧量的变化。
A、单电级记录B、功能核磁共振C、内源信号光学成像D、深度脑刺激3【判断题】深度脑刺激可以无损伤地看到大脑的功能活动。
(?) 4【判断题】神经元的细胞体大小为1毫米。
(?)2.1“标准像”与信息传递1【单选题】以下(C)是神经元所不具备的。
A、细胞核B、树突C、细胞壁D、轴突2【单选题】神经元的末端的细小分支叫做(D)。
A、树突B、细胞核C、轴突D、神经末梢3【判断题】每个神经元可以有多个树突。
(?)4【判断题】每个神经元可以有多个轴突。
(?)2.2信息交流的结构单元1【单选题】按照对后继神经元的影响来分类,可分为(A)类。
A、2B、3C、4D、12【单选题】电突触具备(D)特点。
A、突触延迟B、神经递质释放C、前膜与后膜相互独立隔绝D、信号可双向传递3【多选题】神经元按其功能可分为(ABC)。
A、感觉神经元B、联络神经元C、运动神经元D、抑制性神经元4【判断题】神经元具有接受、整合和传递信息的功能。
(?)5【判断题】神经元就是神经细胞。
某大学生物工程学院《普通生物化学》考试试卷(6962)

某大学生物工程学院《普通生物化学》课程试卷(含答案)__________学年第___学期考试类型:(闭卷)考试考试时间:90 分钟年级专业_____________学号_____________ 姓名_____________1、判断题(140分,每题5分)1. 蛋白质合成的方向是从羧基端到氨基端。
()答案:错误解析:2. L抗坏血酸有活性,D抗坏血酸没有活性。
()答案:正确解析:维生素C又称抗坏血酸,有L及D型两种异构体。
其中只有L 型有生理功效,它的还原型和氧化型都有生物活性。
3. 嘧啶核苷酸从头合成途径不涉及天冬酰胺。
()[浙江大学2018研]答案:正确解析:嘧啶核苷酸的从头合成途径中嘧啶环上的各个原子分别来自于CO2、谷氨酰胺和天冬氨酸。
4. 胰高血糖素的作用需要G蛋白,而胰岛素的作用不需要G蛋白。
()答案:错误解析:都需要G蛋白,胰高血糖素需要GS,胰岛素需要Ras。
5. 反转录病毒基因组含有两条RNA链,属于双链RNA病毒。
()答案:错误解析:反转录病毒基因组含有两个拷贝的正链RNA,与带有正负链RNA的双链RNA病毒不同。
6. 在熔解温度时,双链DNA分子会变为无序的单链分子。
()[厦门大学2014研]答案:错误解析:DNA链的核苷酸序列已经固定,在变性时只是双链连接的氢键被破坏。
7. 核苷酸补救途径的特征是所有核苷酸都可以用现成的碱基合成核苷酸。
()[复旦大学2009研]答案:错误解析:核苷酸补救合成途径并不是所有的核苷酸都可以用现成的碱基合成核苷酸,有的只能利用嘧啶核苷或者嘌呤核苷合成。
8. 脯氨酸不能参与α螺旋,它使α螺旋弯曲(bend),在肌红蛋白和血红蛋白的多肽链中,每一个弯曲处并不一定有脯氨酸,但是每个脯氨酸却产生一个弯曲。
()答案:正确解析:9. 细胞膜类似于球蛋白,有亲水的表面和疏水的内部。
()答案:正确解析:10. 膜脂沿膜平面的侧向扩散速度与从一侧到另一侧的旋转扩散速度大体相等。
抗冻蛋白的特性和作用机制
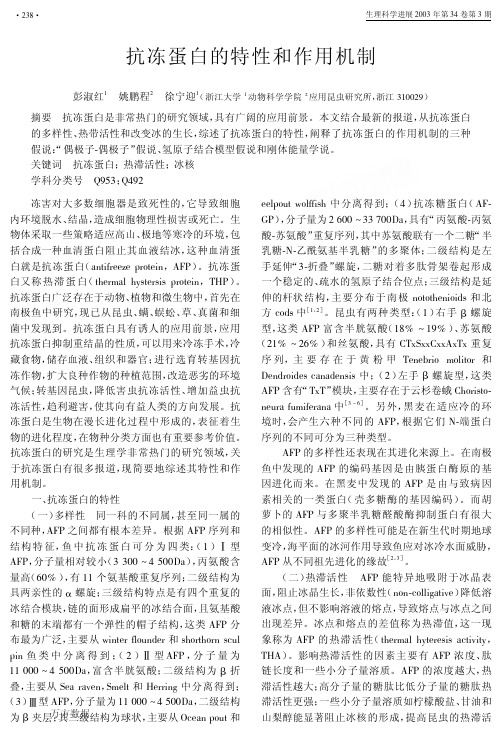
・ J$/・
生理科学进展 J//I 年第 I$ 卷第 I 期
不清楚。!"# 的热滞活性可能是该分子有多个冰结 合位点, 这些位点要么识别不同的冰, 要么在冰晶格 上不同方向有重复表达。这些特征可能增加与冰结 合的频率
[ $]
子, 以便在冰结合面与 !"# 结合的更好。随着科学 技术的发展、 先进科学手段的介入, 对 !"# 与冰晶 作用机制将得到更完善的阐释。 参 考 文 献
・ #$N・
生理科学进展 #%%$ 年第 $* 卷第 $ 期
抗冻蛋白的特性和作用机制
! 彭淑红! " 姚鹏程# " 徐宁迎( 浙江大学 ! 动物科学学院 # 应用昆虫研究所, 浙江 $!%%#& )
摘要" 抗冻蛋白是非常热门的研究领域, 具有广阔的应用前景。本文结合最新的报道, 从抗冻蛋白 的多样性、 热带活性和改变冰的生长, 综述了抗冻蛋白的特性, 阐释了抗冻蛋白的作用机制的三种 假说: “ 偶极子’偶极子” 假说、 氢原子结合模型假说和刚体能量学说。 关键词" 抗冻蛋白;热滞活性;冰核 学科分类号" (&)$ ; (*&# " " 冻害对大多数细胞器是致死性的, 它导致细胞 内环境脱水、 结晶, 造成细胞物理性损害或死亡。生 物体采取一些策略适应高山、 极地等寒冷的环境, 包 括合成一种血清蛋白阻止其血液结冰, 这种血清蛋 白就是抗冻蛋白 ( +,-./01121 304-1.,,567 ) 。抗冻蛋 。 白又称 热 滞 蛋 白 ( -8109+: 8;<-10<.< 304-1.,,=>7 ) 抗冻蛋白广泛存在于动物、 植物和微生物中, 首先在 南极鱼中研究, 现已从昆虫、 螨、 蜈蚣、 草、 真菌和细 菌中发现到。抗冻蛋白具有诱人的应用前景, 应用 抗冻蛋白抑制重结晶的性质, 可以用来冷冻手术, 冷 藏食物, 储存血液、 组织和器官; 进行选育转基因抗 冻作物, 扩大良种作物的种植范围, 改造恶劣的环境 气候; 转基因昆虫, 降低害虫抗冻活性、 增加益虫抗 冻活性, 趋利避害, 使其向有益人类的方向发展。抗 冻蛋白是生物在漫长进化过程中形成的, 表征着生 物的进化程度, 在物种分类方面也有重要参考价值。 抗冻蛋白的研究是生理学非常热门的研究领域, 关 于抗冻蛋白有很多报道, 现简要地综述其特性和作 用机制。 一、 抗冻蛋白的特性 ( 一) 多样性" 同一科的不同属, 甚至同一属的 不同种, 567 之间都有根本差异。根据 567 序列和 结构 特 征, 鱼 中 抗 冻 蛋 白 可 分 为 四 类: (!) !型 567, 分子量相对较小 ( $ $%% ? * )%%@+ ) , 丙氨酸含 量高 ( A%B ) , 有 !! 个氨基酸重复序列; 二级结构为 具两亲性的 " 螺旋; 三级结构特点是有四个重复的 冰结合模块, 链的面形成扁平的冰结合面, 且氨基酸 和糖的末端都有一个弹性的帽子结构, 这类 567 分 布最为广泛, 主要从 C.,-10 /:4D,E10 和 <840-840, <FD: 3., 鱼 类 中 分 离 得 到 ; (#) 分子量为 # 型 567 , !! %%% ? * )%%@+, 富含半胱氨酸; 二级结构为 $ 折 叠, 主要从 G1+ 0+H1, , G91:- 和 >100.,I 中分离得到; ( $) 分子量为 !! %%% ? * )%%@+, 二级结构 %型 567, 万 方数据 为 $ 夹层; 其三级结构为球状, 主要从 JF1+, 34D- 和 11:34D- C4://.<8 中分离得到; (*) 抗冻糖蛋白 ( 56’ K7) , 分子量为 # A%% ? $$ L%%@+, 具有 “ 丙氨酸’丙氨 酸’苏氨酸” 重复序列, 其中苏氨酸联有一个二糖 “半 乳糖’M’乙酰氨基半乳糖” 的多聚体; 二级结构是左 螺旋, 二糖对着多肽骨架卷起形成 手延伸 “ $’折叠” 一个稳定的、 疏水的氢原子结合位点; 三级结构是延 伸的杆状结构, 主要分布于南极 ,4-4-81,.4.E< 和北
某大学生物工程学院《普通生物化学》考试试卷(6632)

某大学生物工程学院《普通生物化学》课程试卷(含答案)__________学年第___学期考试类型:(闭卷)考试考试时间:90 分钟年级专业_____________学号_____________ 姓名_____________1、判断题(140分,每题5分)1. 维生素E是一种抗氧化剂,对线粒体膜上的磷脂有抗自由基的作用。
()[中山大学2004研]答案:正确解析:维生素E极易氧化而保护其他物质不被氧化,是动物和人体中最有效的抗氧化剂,它对抗生物膜磷脂中不饱和脂肪酸的过氧化反应,因而避免脂质中过氧化物产生,保护生物膜的结构和功能。
机体代谢不断产生自由基。
维生素E能捕捉自由基使其吡喃环上酚基失去一个氢原子而形成生育酚自由基。
生育酚自由基又可进一步反应生成非自由基产物——生育醌。
此外维生素E还可与硒协同谷胱甘肽过氧化物酶发挥抗氧化作用。
2. 人体不仅能利用D葡萄糖而且能利用L葡萄糖。
()答案:错误解析:3. 脱氨后的氨基酸碳架,进入各自的分解途径彻底氧化成CO2和H2O,并释放出能量。
()答案:错误解析:4. 对于提纯的DNA样品,测得OD260OD280值小于1.8,则说明样品中含RNA。
()[华东师范大学2007研]答案:错误解析:DNA纯制品的OD260OD280值为1.8,纯RNA的A260A280应为2.0,因此含有RNA则该比值会升高。
5. Rec A蛋白对单链DNA的亲和力高于对双链DNA的亲和力。
()答案:正确解析:Rec A蛋白参与DNA重组和SOS修复,这都需要它与单链DNA结合。
6. 只有单细胞生物中发生的突变可以遗传给后代。
()答案:错误解析:多细胞生物生殖细胞中发生的突变也可以遗传给后代。
7. 氨基甲酰磷酸既可以合成尿素也可以用来合成嘌呤核苷酸。
()[华中农业大学2017研]答案:错误解析:体内催化氨基甲酰磷酸生成的酶有两种,一种是氨基甲酰磷酸合成酶Ⅰ,存在于肝线粒体中,最终产物是尿素;另一种是氨基甲酰磷酸合成酶Ⅱ,存在于各种细胞的细胞液中,反应最终产物是嘧啶。
基于MHSA

doi:10.3969/j.issn.1003-3106.2024.01.010引用格式:张正华,吴宇,金志琦.基于MHSA YOLOv7的小麦赤霉病感染率检测[J].无线电工程,2024,54(1):71-77.[ZHANGZhenghua,WUYu,JINZhiqi.DetectionofGibberellaInfectionRateinWheatBasedonMHSA YOLOv7[J].RadioEngineering,2023,54(1):71-77.]基于MHSA YOLOv7的小麦赤霉病感染率检测张正华,吴 宇,金志琦(扬州大学信息工程学院(人工智能学院),江苏扬州225127)摘 要:在抗病育种中小麦赤霉病感染率是衡量籽粒抗性表型鉴定的重要衡量指标,针对目前小麦赤霉病感染率检测存在检测时间长、硬件成本高以及检测方式破坏植株等问题,设计了一种适用于麦穗籽粒此类小目标检测的深度学习网络模型———MHSA YOLOv7。
通过在原YOLOv7主干网络中融合多头自注意力(Muti HeadSelf Attention,MHSA)机制来提高模型对深层语义特征的提取能力,并使用加权双向特征金字塔网络(BidirectionalFeaturePyramidNetwork,BiFPN)实现模块间的跨层连接,使该模型能够提取和传递更丰富的特征信息。
实验结果表明,MHSA YOLOv7在小麦单穗赤霉病数据集上达到了90.75%的检测精度,相较于原YOLOv7模型,改进后的算法对于麦穗籽粒此类小目标物体具有更强的特征提取能力,检测精度、召回率、F1值、mAP@0.5以及mAP@0.5∶0.95分别提高了0.33%、1.83%、0.011、1.19%以及0.38%,有效满足了小麦赤霉病感染率的精确检测,为实现小麦植株病害走势的长期观测以及小麦籽粒抗性的准确评估提供了技术支持。
关键词:多头自注意力;YOLOv7;目标检测;小麦赤霉病中图分类号:TP391文献标志码:A开放科学(资源服务)标志码(OSID):文章编号:1003-3106(2024)01-0071-07DetectionofGibberellaInfectionRateinWheatBasedonMHSA YOLOv7ZHANGZhenghua,WUYu,JINZhiqi(SchoolofInformationEngineering(SchoolofArtificialIntelligence),YangzhouUniversity,Yangzhou225127,China)Abstract:Indiseaseresistancebreeding,theinfectionrateofgibberellainwheatisanimportantindicatortomeasurethephenotypeidentificationofgrainresistance.Inviewoftheproblemsoflongdetectiontime,highhardwarecostanddamagetoplantsinthedetectionofwheatgibberellainfection,adeeplearningnetworkmodel,orMHSA YOLOv7suitableforthedetectionofsmallobjectssuchaswheateargrainisdesigned.ByintegratingtheMuti HeadSelf Attention(MHSA)mechanismintheoriginalYOLOv7backbonenetwork,themodelcanextractdeepsemanticfeatures,andtheweightedBidirectionalFeaturePyramidNetwork(BiFPN)isusedtorealizethecross layerconnectionbetweenmodules,sothatthemodelcanextractandtransmitricherfeatureinformation.TheexperimentalresultsshowthatMHSA YOLOv7achievesadetectionaccuracyof90.75%onthewheatsingleeargibberelladataset.ComparedwiththeoriginalYOLOv7model,theimprovedalgorithmhasstrongerfeatureextractionabilityforsmallobjectssuchaswheateargrain,andthedetectionAccuracy,Recall,F1score,mAP@0.5andmAP@0.5:0.95areimprovedby0.33%,1.83%,0.011,1.19%and0.38%respectively.Theimprovedalgorithmeffectivelysatisfiestheaccuratedetectionofwheatgibberellainfectionrate,andprovidestechnicalsupportforlong termobservationofwheatdiseasetrendsandaccurateassessmentofwheatgrainresistance.Keywords:MHSA;YOLOv7;objectdetection;wheatgibberella收稿日期:2023-05-15基金项目:2022年江苏省研究生实践创新计划(SJCX22_1708);2021年扬州市级计划-市校合作专项(YZ2021159);2021年扬州市产业前瞻与共性关键技术-产业前瞻研发(YZ2021016)FoundationItem:2022JiangsuProvincalPostgraduatePracticeInnovationPlan(SJCX22_1708);2021YangzhouMunicipalPlan City SchoolCooperationProject(YZ2021159);2021YangzhouCity sIndustrialForesightandCommonKeyTechnologies IndustrialProspectResearchandDevelopment(YZ2021016)信号与信息处理0 引言小麦作为亚洲、欧洲和北美等地区的主要作物,是仅次于玉米和大米的第三大消费谷物[1]。
GABA能神经元

Spontaneous and locomotor-related GABAergic input onto primary afferents in the neonatal ratSilvia Fellippa-Marques,Laurent Vinay and FrancËois ClaracCNRS,UPR Neurobiologie et Mouvements(UPR9011),31chemin Joseph Aiguier,13402Marseille Cx20,France Keywords:antidromic discharges,bicuculline,presynaptic inhibition,spinal cordAbstractThe in vitro brain stem±spinal cord preparation of neonatal rats(0±5days old)was used to examine the contribution of GABA A(g-aminobutyric acid)receptors to the spontaneous and locomotor-related antidromic®ring in the dorsal roots of neonatal rats. Spontaneous bursts of antidromic discharges were generated by the underlying afferent terminal depolarizations reaching spiking threshold.The number of antidromic action potentials increased signi®cantly in saline solution with Cl±concentration reduced to50% of control.Bath application of the GABA A receptor antagonist bicuculline,at low concentrations(1±2m M),or picrotoxin blocked the antidromic discharges in the dorsal roots almost completely.The increase in Cl±conductance was therefore mediated by an activation of GABA A receptors.Increasing the concentration of bicuculline to10±20m M never blocked these discharges further.On the contrary,in half of the preparations,the number of antidromic action potentials was higher in the presence of high concentrations of bicuculline(10±20m M)than in the presence of picrotoxin or low concentrations of bicuculline.This suggests that bicuculline,at high concentrations,may have other effects,in addition to blocking GABA A receptors.Dorsal root®ring was observed during®ctive locomotion induced by bath application of excitatory amino acids and serotonin.A rhythmical pattern was often demonstrated. Bicuculline at low concentrations caused a decrease of the antidromic discharge whereas,at high concentrations,bursts of discharges appeared.A double-bath with a barrier built at the L3level was then used to separate the mechanisms which generate locomotion from those mediating primary afferent depolarizations.Excitatory amino acids and serotonin were perfused in the rostral pool only.Decreasing the concentration of chloride in the caudal bath caused a sharp increase in the number of antidromic action potentials recorded from the L5dorsal root.These discharges,which were modulated in phase with the locomotor rhythm,were blocked by bicuculline.These data demonstrate the existence of a locomotor-related GABAergic input onto primary afferent terminals in the neonatal rat.IntroductionIt is well established that one form of presynaptic inhibition in the vertebrate spinal cord is associated with primary afferent depolariza-tion(PAD,Rudomin,1990;Rudomin et al.,1993;Alvarez-Leefmans et al.,1998).Suprathreshold depolarization triggers discharges that are antidromically conducted into peripheral nerves.This was®rst demonstrated by recording the re¯ex response elicited in dorsal roots, by electrical stimulation of an adjacent dorsal root(`dorsal root re¯ex',Toennies,1938;Barron&Matthews,1938a;see Kerkut& Bagust,1995for review).Antidromic discharges of primary afferents have been observed during locomotion in the cat(®ctive locomotion, Dubuc et al.,1988;Duenas et al.,1990;Gossard et al.,1991;and actual locomotion,Beloozerova&Rossignol,1994;1995;Rossignol et al.,1998)and in the rat(Pilyavskii et al.,1988).A spontaneous antidromic activity in the dorsal roots has been described in the in vitro spinal cord isolated from adult(Bagust et al.,1989;Chen et al., 1993)or young hamsters(Abdul-Razzak et al.,1994;see Kerkut& Bagust,1995for review).Antidromic discharges are also recorded from lumbar dorsal roots in the neonatal rat spinal cord in vitro (Kremer&Lev-Tov,1998;Vinay&Clarac,1999).There is a wealth of evidence suggesting that g-aminobutyric acid (GABA),through the activation of GABA A receptors,plays a major role in the generation of PAD and therefore of antidromic discharges. Axo-axonic interactions between GABAergic terminals and primary afferents have been demonstrated(see Alvarez,1998for review). Activation of GABA A receptors increases membrane conductance to chloride ions,which leads to depolarization of afferent terminals.Both the GABA-evoked depolarization of terminals and PAD are reduced by GABA A receptor antagonists(Eccles et al.,1963;Levy,1975; Rudomin et al.,1981;Curtis&Lodge,1982).In addition,antidromic discharges elicited by stimulation of ventral descending pathways in the neonatal rat spinal cord in vitro are blocked by bath application of bicuculline,a GABA A receptor antagonist(Vinay&Clarac,1999). However,mechanisms other than activation of GABA A receptors have also been suggested to be responsible for PAD.In particular, PAD has been proposed to result from the transient increase in concentration of potassium ions in the extracellular space of the spinal cord,as a consequence of interneuronal activity(Barron& Matthews,1938b;see Vyklicky&Knotkova-Urbancova,1998for review).Support for this idea was provided recently by Kremer& Lev-Tov(1998)who described dorsal root afferent depolarization and antidromic®ring in isolated spinal cords of neonatal rats in the presence of bicuculline.The aim of this study was to identify whether and to what extent the activation of GABA A receptors contributes to the spontaneousCorrespondence:Dr L.Vinay,as above.E-mail:vinay@rs-mrs.frReceived29July1999,revised16September1999,accepted23September1999European Journal o Neuroscience,Vol.12,pp.155±164,2000ãEuropean Neuroscience Associationand locomotor-related antidromic®ring in dorsal roots of neonatal ing the in vitro brain stem±spinal cord preparation,we show that most of the spontaneous antidromic discharges disappear after application of low concentrations of bicuculline or picrotoxin(PTX). We also demonstrate a phasic GABAergic in¯uence on primary afferent terminals during®ctive locomotion.Materials and methodsExperiments were performed on32Wistar rats[from postnatal day0 (P0),de®ned as the®rst24h after birth,to P5].All surgical and experimental procedures were made to minimize animal suffering and conformed to the guidelines from the French Ministry for Agriculture and Fisheries,Division of Animal Rights.The animals were anaesthetized with ether,decerebrated at a postcollicular level and eviscerated.They were pinned down onto a Petri dish continuously perfused with saline solution,containing the following substances(in m M):NaCl,130;KCl,4;CaCl2,3.75;MgSO4,1.3; NaH2PO4,0.58;NaHCO3,25;glucose,10,bubbled with a95%O2±5%CO2mixture,and adjusted to pH7.4.A dorsal craniotomy and laminectomy was performed.The brain stem,spinal cord and lumbar roots were then removed and pinned down with the ventral side up in the recording chamber.Monopolar stainless steel electrodes were placed in contact with the roots and insulated with vaseline,for recording of discharges (bandwidth,70Hz to1kHz).Dorsal root potentials were recorded to investigate the relationships between antidromic discharges and primary afferent depolarizations.For this purpose,dorsal roots were cut~2mm away from the spinal cord and the central stump was incorporated in a suction electrode®lled with normal saline and connected to an AC-coupled ampli®er(bandwidth,0.1Hz to1kHz). Data were collected through an Instrutech(NY,USA)ITC-16MAC interface on a Macintosh Quadra650(software programs from Axon Instruments,CA,USA).The number of action potentials was measured by using a software voltage discriminator(Fig.1B1, arrows)and peak detector.Antidromic discharges occurring sponta-neously in dorsal roots were recti®ed and integrated(time constant 5ms).Sections of traces(duration2s)including the bursts were duplicated,aligned at the burst onset and averaged(25±50bursts)to investigate the effect of low-chloride solutions on the spontaneous bursting dorsal root activity(Fig.3).Fictive locomotion was elicited by bath application of N-methyl-D,L-aspartate(NMA,15±20m M)and serotonin(5-HT,10m M).A program was written to normalize the locomotor cycles and to sample the dorsal root antidromic discharges in100time intervals per locomotor cycle.The onset of the L2or L3ventral root burst on the right side was taken as time t=0for the resampling.A threshold was used for the detection of action potentials and the pattern of dorsal root discharges was identi®ed from30±100consecutive locomotor cycles.The number of antidromic action potentials was averaged over intervals of5±10%of the locomotor cycle(Figs6±8).Averages of the recti®ed ventral root activity were also plotted(Figs6B and8F). Results are presented in the text and®gures in the form of mean6SEM.The Mann±Whitney test was employed for statistical analysis when two groups were compared,and a one-way ANOVA with Tukey post test for more than two groups(Graphpad Prism2 Software,CA,USA).Bicuculline methiodide and PTX were obtained from Sigma(MO, USA).A low-chloride solution was prepared by replacing NaCl in control saline with equimolar amounts of Na isethionate(Vinay& Clarac,1999).ResultsSpontaneous GABAergic antidromic dorsal root discharges Spontaneous activity recorded from lumbar dorsal roots consisted of bursts of action potentials separated by silent periods or continuous ®ring of action potentials(Figs1A and2A1,L5dorsal root). Motoneuron bursting episodes could be recorded from the ipsilateral ventral roots under these conditions.Motor activity,when present, was in phase with dorsal root discharge(Fig.1A).Note that the motor axons in the L3and L5ventral roots on one side burst simultaneously and not in the alternating manner characteristic of®ctive locomotion (Cazalets et al.,1992;Kiehn&Kjaerulff,1996).The interval between bursts was variable within a recording session(Fig.1B1 and B2,note that at least four ranges of intervals could be detected:2±3s;7±10s;16±23s;and28±32s).Recordings of a dorsal root in the neighbouring segment with a suction electrode showed thatF IG.1.Spontaneous bursts in ventral and dorsal roots.(A)Simultaneousrecordings from two ventral roots and one dorsal root on the same side of a P2rat in vitro.(B1)Spontaneous burst activity recorded from the L5dorsal root ofa P4rat.The interval(in s)between two consecutive bursts is indicated belowthe recording.Action potentials were detected by means of peak and leveldetectors(horizontal arrows).(B2)Distribution of intervals between eachaction potential recorded from the dorsal root(same root as in B1;n=670action potentials taken into account)and all the subsequent ones occurringwithin the next1±35s.156S.Fellippa-Marques et al.Ó2000European Neuroscience Association,European Journal of Neuroscience,12,155±164depolarization occurred in phase with the bursts (lower trace in Fig.2A 1and A 2).The number of action potentials in a burst was proportional to the area of the dorsal root potential (Fig.2A 1and 2B,correlation coef®cient,r =0.94).Spontaneous discharges were there-fore generated by PAD reaching ®ring threshold.Discharges were antidromically conducted into the dorsal roots (Fig.2C).We tested whether a Cl ±conductance was involved in generating antidromic action potentials by decreasing the concentration of Cl ±ions in the saline solution (Vinay &Clarac,1999).This should make the reversal potential for Cl ±currents in the afferent terminals become more positive,thereby increasing the amplitude of depolarizations and the probability of ®ring antidromic action potentials (Clarac &Cattaert,1996;Alvarez-Leefmans et al .,1998).The spontaneous dorsal root activity increased after replacing half of the NaCl in the normal solution by Na isethionate (compare Fig.3A 2with Fig.3A 1;Fig.3B).Bursts,after recti®cation and integration,were increased both in amplitude and duration in low Cl ±solutions [Fig.3C;699637ms (n =50)vs.554630ms (n =51)in control;P <0.01].This resulted in a signi®cant increase in the area of the bursts (+66%,P <0.001).A similar increase was observed in the three experiments tested in this way.There was a non-signi®cant trend towards an increase in burst frequency in low Cl ±solutions (Fig.3D,0.18Hz)compared with control (0.16Hz).These effects were reversible within 20±30min after the normal external concentration of Cl ±was restored (Fig.3A 3,B and D).These results indicate that the depolarizations which trigger antidromic action potentials are due to an increase in Cl ±conductance.Bicuculline was applied to determine whether the increase in chloride conductance was mediated by an activation of GABA A receptors.The dorsal root activity was always sharply reduced in the presence of low concentrations of bicuculline (1±2m M ,n =9)as shown in Fig.4by the 3-min-long recordings (compare Fig.4A 3with Fig.4A 1and A 2,and Fig.4A 4and A 5)and the graph with the instantaneous burst frequency plotted against time (Fig.4B).However,a few antidromic discharges persisted in the presence of bicuculline at 1±2m M (Fig.4B).These may be due to non-GABA A receptor-mediated mechanisms or to an uncomplete blockade of GABA A receptors.Increasing the concentration of bicuculline to 10±20m M never blocked these discharges further (n =7).On the contrary,in three preparations,there was a signi®cant (P <0.05)increase in the number of antidromic action potentials per minute in the presence of high concentrations of bicuculline,compared with low concentrations (Fig.4C).Either no effect or a non-signi®cant trend towards an increase was observed in the remaining four experiments.The absence or existence of effects was not related to the age of animals.PTX (10m M ,n =4;20m M ,n =4),another GABA A -receptor antago-nist,was also tested.Antidromic discharges disappeared almost completely in the presence of PTX (Fig.5A 1,A 2and B;Table 1).Surprisingly,instead of decreasing further,the number of antidromic action potentials in the dorsal root increased after addingbicuculline100 ms500 ms100 µV 200 µV DRP area (µV .ms)Spike-triggered averagingN u m b e r o f a n t i d r o m i c a c t i o n p o t e n t i a l sL5 DRL4 DR prox.L4 DR dist.L5 DR (rectified)L4 DRPL4 DRPA 1A 2BC10 msF IG .2.Spontaneous depolarizations of primary afferent terminals and antidromic discharges.(A 1)Simultaneous recordings made from the L5(stainless steel electrode placed in contact with the root)and the L4(suction electrode)dorsal roots showed that discharges were associated with primary afferent depolarizations.(A 2)Averages of the discharges (recti®ed trace)and dorsal root potentials.Recordings were triggered by the action potentials detected in the L5dorsal root (n =276).(B)Number of action potentials in a burst (L5)plotted against the area of the depolarization (above the baseline)recorded from the adjacent dorsal root (L4).Correlation coef®cient of the regression line,r =0.94.(C)Two electrodes were placed in contact with the L4dorsal root to record spontaneous antidromic activity at both proximal (prox.)and distal (dist.)levels.Data recording was triggered by action potentials detected by the distal electrode (averaging of 450events).Action potentials were propagated in a proximo-distal direction with a conduction time of ~4±5ms between the two electrodes (interval between the vertical dotted lines).GABA A receptors and antidromic discharges 157Ó2000European Neuroscience Association,European Journal of Neuroscience ,12,155±164(20m M )to the PTX (20m M )-containing saline solution (Fig.5A 2,A 3and B).This increase was observed for all the dorsal roots recorded during the four experiments tested and was signi®cant in two preparations (Table 1).The effects of bicuculline at 20m M were reversible as shown by the reduction of the antidromic discharges after decreasing the concentration of bicuculline (Fig.4C)or removing bicuculline from the bath (Fig.5B,Table 1).These results show that the spontaneous antidromic activity results almost exclusively from the activation of GABA A receptors.A difference in the blockade of this activity was observed between PTX and bicuculline,suggesting that the latter,at high concentrations,may have other effects,in addition to blocking GABA A receptors.Locomotion-related antidromic dorsal root dischargesDorsal roots were recorded during ®ctive locomotion induced by adding excitatory amino acids and serotonin to the saline solution (n =13).Fictive locomotion was characterized by:(i)the bursts of activity in the right and left ventral roots alternating in a given segment (Fig.6A and B,recti®ed activity);and (ii)the L2/L3ventral roots on one side bursting in phase and the L5ventral root bursting in antiphase (Cazalets et al .,1992;Kiehn &Kjaerulff,1996).Dorsal root ®ring was observed under these conditions in all the experiments.The discharge rarely appeared,at ®rst sight,to be related with the motor activity (Fig.6A,note the irregular ®ring in the L3dorsal root).However,a rhythmical pattern was often demonstrated after detailed analysis including normalization of the locomotor cycles and resampling of the dorsal root antidromic discharges (see Materials and methods).The graph at the bottom of Fig.6B shows a signi®cant decrease in the discharge during the motor burst in the homonymous ventral root on the same side (10±20%of the cycle;see also arrows in Fig.6A).The discharge of seven dorsal roots (four L2,one L3and two L5)recorded in ®ve experiments was analysed.A signi®cant rhythmic modulation of the discharge was observed in ®ve dorsal roots (P <0.05).No characteristic pattern of discharge could be revealed.The contribution of GABA A receptors to these discharges during ®ctive locomotion was evaluated by applying bicuculline (1±20m M ,n =18).At low concentrations (1±2m M ,n =13),bicuculline resulted in a signi®cant (P <0.001)decrease of the antidromic discharge (Fig.7A 2and B 2continuous line;760.5action potentials per cycle,n =30)as compared with control (Fig.7A 1,B 1and B 2dotted line;12.660.6action potentials per cycle,n =30).Bursts of antidromic discharges appeared when the concentration of bicuculline was increased (Fig.7A 3and B 3).In the experiment illustrated in Fig.7,these bursts occurred at the cycle onset (vertical dotted line in Fig.7A 3,time t =0in Fig.7B 3).Such a strong modulation of dorsal root activity was observed in each of the ®ve preparationsperfusedF IG .3.Involvement of chloride conductance in the spontaneous antidromic activity.(A 1±A 3)The spontaneous antidromic activity was enhanced in low-chloride saline solution (A 2)when compared with normal saline (A 1,control and A 3,washout).(B)Mean (6SEM)number of antidromic action potentials in the L5dorsal root in low-chloride saline solution compared with normal saline (control and after washout).(C)Average of 50bursts of antidromic action potentials recorded in normal (control)and low-chloride (50%Cl ±)saline solutions.Traces were recti®ed and integrated (time constant,5ms).The sections of traces including bursts were duplicated and aligned at burst onset before averaging.Note the increase in amplitude and duration in low-chloride saline indicating a higher number of action potentials.(D)A slight,non-signi®cant,increase in the burst frequency was observed in low-chloride saline.158S.Fellippa-Marques et al .Ó2000European Neuroscience Association,European Journal of Neuroscience ,12,155±164with high concentrations of bicuculline (10±20m M ).These ®ndings indicate that antidromic discharges may be recorded from dorsal roots following the activation of the spinal cord by excitatory amino acids and serotonin and the disinhibition by bicuculline.However,they do not give any indication on whether primary afferent terminals receive a phasic input from GABAergic interneurons during ®ctive locomo-tion.Because of the interaction of bicuculline with the locomotor rhythm (Fig.7;Cazalets et al .,1994;Cowley &Schmidt,1995;Kremer &Lev-Tov,1997;see also Bertrand &Cazalets,1999),we separated the mechanisms which generate locomotion from those mediating primary afferent depolarizations.The recording chamber was partitioned into two pools with a barrier built at the L3±L4level so that each pool could be perfused separately (Fig.8A,n =4experiments).The central pattern generators for locomotion were activated by perfusing excitatory amino acids and serotonin only in the rostral bath (Cazalets et al .,1995;1996),and the L5dorsal roots were recorded in the caudal bath in the absence of the tonic pharmacological activation by these two substances.A rhythmic bursting discharge was recorded from the L2ventral roots (Fig.8B±F,top two traces).Antidromic discharges were recorded from the L5dorsal roots in normal saline solution.Only a slight modulation of the discharge with a decreased activity at ~60%of the cycle (Fig.8B,lower trace and Fig.8F continuous trace at the bottom of the graph)could be observed.Decreasing the concentration of chloride in the caudal bath caused a sharp increase in the number of antidromic action potentials recorded from the L5dorsal root (Fig.8C and F,compare traces `B'and `C').In addition,the discharge was strongly modulated with a peak occurring at the end of the cycle (signi®cant difference between the peak and the minimum with P <0.001).Adding bicuculline (1m M )to the low-chloride solution in the caudal bath blocked the discharges almost completely (Fig.8D).The number of action potentials in the different phases of the locomotor cycle was smaller than that in normal saline solution (Fig.8F,compare traces `D'and `B').These effects were reversible (Fig.8E and F,trace `E').Similar results were obtained in the four preparations tested.These data establish the existence of a locomotor-related GABAergic input onto primary afferent terminals in the neonatal rat.DiscussionSpontaneous and locomotor-related GABAergic activity in dorsal root afferentsThe present study has revealed that spontaneous antidromic activity is propagated in dorsal roots of isolated spinal cord preparations (Fig.1).This activity is associated with primary afferentdepolariza-F IG .4.The antidromic activity is due to the activation of GABA A receptors.(A 1±A 5)Spontaneous bursting activity in the L5dorsal root (A 1,A 2;note that each vertical line is a burst)is almost completely blocked by application of bicuculline (2m M )in the saline solution (A 3,note the absence of activity during the 3-min recording).Effects are reversible (A 4,A 5).A 2and A 5are enlargements of the sections of traces between vertical dotted lines in A 1and A 4,respectively.(B)Instantaneous burst frequency before,during and after the application of bicuculline.(C)Mean (6SEM)number of antidromic action potentials in the L4dorsal root in another experiment at two different concentrations of bicuculline (2and 20m M )compared with normal saline (control and after washout of bicuculline).Note the signi®cant (<0.001)increase in the discharge in the presence of 20m M of bicuculline compared with 2m M .This increase is reversible (right 2m M histogram).GABA A receptors and antidromic discharges 159Ó2000European Neuroscience Association,European Journal of Neuroscience ,12,155±164tions (Fig.2)and is due to an increase in chloride conductance (Fig.3).In addition,most of the action potentials disappeared in the presence of bicuculline at low concentrations (1±2m M )or PTX(Figs 4and 5)demonstrating that they are generated by GABAergic mechanisms.High concentrations of bicuculline (10±20m M )caused an increase of the antidromic ®ring when compared with the activity in the presence of either PTX or low concentrations of bicuculline (Figs 4and 5).This may correspond to non-GABAergic effects of bicuculline (see below).60 sBA 1A 2A 3controlPTXPTXPTX +bicuculline PTX 20 µM +bicuculline 20 µMPTX 20 µMcontrolleft L4 DR100N u m b e r o f a c t i o n p o t e n t i a l s / m i n .200300400500600700F IG .5.Bicuculline triggers some spontaneous bursting activity in dorsal roots.(A 1±A 3)Four-minutes-long dorsal root recordings in normal saline solution (A 1),after addition of PTX (A 2),and under both PTX and bicuculline (A 3).(B)The number of antidromic action potentials was decreased signi®cantly (P <0.001)in the presence of GABA A receptor antagonists when compared with control.However,antidromic discharges were signi®cantly (P <0.001)more numerous in the presence of both antagonists than in the presence of PTX alone.The activity decreased after washout of bicuculline (no signi®cant difference under PTX before and after bicuculline;P >0.05).T ABLE 1.One-wayANOVAwith a Tukey's multiple comparison test of antidromic activity in the presence of PTX and bicuculline (BIC)Number of action potentials per minute (+record duration in minutes)Dorsal PTX vs.PTX +BIC PTX +BIC Experiment root Control PTXPTX +BIC PTX (wash)control vs.control vs.PTX 1Right L2234639.3(5)6164.8(12)132614.6(12)*******1Right L4394610.1(7)5468.5(12)190621.8(12)*********2Left L3108622.3(4)860.9(12)2163.7(12)******NS 2Left L5292630.1(5)2061.6(12)3163.1(12)******NS 3Left L515866.2(16)2668.5(12)36617.4(12)561.5(8)******NS 4Right L4371616.3(16)2567.5(12)101629.1(12)43617.6(8)*******4Left L4493622.4(16)56613.4(12)228641.6(12)81622.2(8)*********Data are shown as means 6SEM,with the number of minutes of recording taken into account in parentheses.Four experiments were performed.NS,non-signi®cant;*P <0.05;**P <0.01;***P <0.001.right L3 VRright L3 VR right L3 DRright L3 DRN u m b e r o f a c t i o n p o t e n t i a l sleft L3 VRleft L3 VRcycle (%)ABF IG .6.Locomotor-related modulation of the antidromic discharges.(A)The antidromic discharges in the dorsal root decreased (arrows)shortly after the burst onset in the ventral root on the same side (indicated by vertical dotted lines).(B)Cycles were normalized and onset of the right ventral root burst was taken as time t =0for average.Averaged recti®ed activities in the two opposite ventral roots are shown (n =120cycles).The normalized locomotor cycle is represented twice.Dorsal root activities were sampled over 30cycles and averaged in 100time intervals per locomotor cycle.The number of antidromic action potentials is averaged over intervals of 10%of the locomotor cycle (6SEM).Note the signi®cant (P <0.01)decrease in the discharge at 10±20%of the cycle,when compared with the next interval.160S.Fellippa-Marques et al .Ó2000European Neuroscience Association,European Journal of Neuroscience ,12,155±164The spontaneous activity consisted of periodic bursts and was therefore probably triggered by a centrally generated rhythm.Motor activity sometimes accompanied the dorsal root bursts indicating the existence of neuronal connections which coactivate the motoneurons and the primary afferent terminals.Alternatively,`antidromic'discharges in dorsal roots may have postsynaptic effects in lumbar motoneurons and ®rst-order interneurons.This hypothesis is under investigation.When present,the motor bursts in the L3and L5ventral roots on the same side were not in antiphase,suggesting that the central pattern generator for locomotion was either inactive or active partially (Cazalets et al .,1992;Kiehn &Kjaerulff,1996).The relationships between the network driving the spontaneous activity and the locomotor network remain to be elucidated.Spontaneous rhythmic motor activity is a property of the developing spinal cord (Gu et al .,1994in Xenopus ;O'Donovan &Landmesser,1987;Chub &O'Donovan,1998;Milner &Landmesser,1999in the chick;Nishimaru et al .,1996;Nakayama et al .,1999in the rat).Spontaneous network-driven activity has also been described in other parts of the central nervous system (CNS)during development (see Feller,1999for recent review;Ho &Waite,1999in the trigeminal system;Ben-Ari et al .,1989in the hippocampus;see Wong,1999for review on the visual system).The spontaneous GABAergic dorsal root discharges demonstrated in the present study may play a role in some developmental processes,e.g.central path®nding,axon extension,synapse formation or maturation of the peripheral receptors.However,spontaneous antidromic discharges are not found only in immature systems as they also occur in the adult hamster spinal cord in vitro (Bagust et al .,1989;Chen et al .,1993).Antidromic discharges were recorded during ®ctive locomotion (see also Kremer &Lev-Tov,1998).A phasic modulation of the discharge could be detected in some dorsal root recordings,demonstrating a locomotion-related input to the interneurons which mediate antidromic discharges (Fig.6).The antidromic activity was decreased by perfusion of bicuculline,at low concentrations,over the whole spinal cord preparation.However,the contribution of GABA A receptors to antidromic discharges recorded during ®ctive locomotion could not be identi®ed in such conditions.A direct effect of bicuculline on the dorsal root discharge may,indeed,be masked by a concomitant action on the locomotor rhythm (Cazalets et al .,1994;Cowley &Schmidt,1995;Kremer &Lev-Tov,1997),which may thereby modulate the intensity of antidromic discharges.The existence of a locomotion-related GABAergic input to primary afferent terminals was demonstrated by partitioning the recording chamber into two pools (Fig.8).This avoided actions of bicuculline on the locomotor rhythm.Antidromic discharges were enhanced in low-chloride solution indicating that the amplitude of locomotor-5 sright L3 VR right L5 DRleft L3 VR controlbicuculline 1µMbicuculline 20µMA 1A 2A 3controlcycle (%)N u m b e r o f a c t i o n p o t e n t i a l sN u m b e r o f a c t i o n p o t e n t i a l sN u m b e r o f a c t i o n p o t e n t i a l sB 1F IG .7.Locomotor-related dorsal root bursting activity in the presence of bicuculline.(A 1±A 3)Simultaneous recordings from two opposite ventral roots and one dorsal root in the same segment during ®ctive locomotion induced by bath application of NMA (17m M )and serotonin (10m M )before (A 1)and after the addition of bicuculline at various concentrations (A 2,1m M ;A 3,20m M ).(B 1±B 3)Mean (6SEM)number of action potentials during the different phases of the locomotor cycle (10%-bins;30cycles averaged).The discharge was modulated in normal saline solution (B 1;signi®cant difference between 15%and 35%,and between 35%and 65%of the cycle with P <0.01and P <0.001,respectively).The number of action potentials per cycle was signi®cantly lower in the presence of bicuculline 1m M (B 2,continuous trace)compared with control (B 1,B 2,dotted trace).Phasic discharges were recorded at the cycle onset (0±20%)in the presence of bicuculline 20m M (B 3).GABA A receptors and antidromic discharges 161Ó2000European Neuroscience Association,European Journal of Neuroscience ,12,155±164。
- 1、下载文档前请自行甄别文档内容的完整性,平台不提供额外的编辑、内容补充、找答案等附加服务。
- 2、"仅部分预览"的文档,不可在线预览部分如存在完整性等问题,可反馈申请退款(可完整预览的文档不适用该条件!)。
- 3、如文档侵犯您的权益,请联系客服反馈,我们会尽快为您处理(人工客服工作时间:9:00-18:30)。
Neurosci Bull October 1, 2015, 31(5): 561–571. DOI: 10.1007/s12264-014-1535-7561·Original Article·Orientation selectivity in cat primary visual cortex: local and global measurementTao Xu1,2, Hong-Mei Yan1, Xue-Mei Song2, Ming Li31Key Laboratory for Neuroinformation of Ministry of Education, University of Electronic Science and Technology of China, Chengdu 610054, China2Shanghai Institutes of Biological Sciences, Chinese Academy of Sciences, Shanghai 200031, China3T he Department of Automatic Control, College of Mechatronics and Automation, N ational University of Defense Technology, Changsha 410073, ChinaCorresponding author: Xue-Mei Song. E-mail: xmsong@© Shanghai Institutes for Biological Sciences, CAS and Springer-Verlag Berlin Heidelberg 2015ABSTRACTIn this study, we investigated orientation selectivity in cat primary visual cortex (V1) and its relationship with various parameters. We found a strong correlation between circular variance (CV) and orthogonal-to-preferred response ratio (O/P ratio), and a moderate correlation between tuning width and O/P ratio. Moreover, the suppression far from the peak that accounted for the lower CV in cat V1 cells also contributed to the narrowing of the tuning width of cells. We also studied the dependence of orientation selectivity on the modulation ratio for each cell, which is consistent with robust entrainment of the neuronal response to the phase of the drifting grating stimulus. In conclusion, the CV (global measure) and tuning width (local measure) are signifi cantly correlated with the modulation ratio.Keywords: cat; orientation selectivity; tuning width; circular variance; primary visual cortexINTRODUCTIONOrientation selectivity is an emergent property of neurons in the primary visual cortex (V1). In Hubel and Wiesel’s feed-forward model, orientation selectivity arises from the alignment of the receptive fields of lateral geniculate nucleus (LGN) neurons presynaptic to each simple cell [1, 2]. However, the mechanisms underlying this selectivity are still under debate[3, 4]. There are essentially two models explaining the mechanisms of orientation selectivity, the feed-forward model that relies on the input from the LGN[1, 4-8] and the feedback model that relies on global inhibition to refi ne the selectivity to a weak bias provided by LGN input[3, 9-12]. The complexity of this issue is highlighted by recent studies explaining adaptation and learning-dependent orientation plasticity[13-15]. To address these issues, we studied the variation of orientation selectivity in cat V1 neurons.Previous authors have studied the distribution of orientation tuning width[16, 17] in cat V1, measuring around the peak of the tuning function (local measure). The shape (aspect ratio) and number of receptive fi eld subregions are major factors that affect the tuning width[18-20]. Orientation selectivity is also associated with a broader range of stimulus values (global measure). A study in m a caque V1[21] reported that the orthogonal-to-preferred response ratio (O/P ratio) has a significant effect on the local and global orientation. Further studies[22, 23] have demonstrated that untuned suppression is crucial for generating highly orientation-selective cells in macaque V1. However, the impact of the O/P ratio and the suppression that are far from the optimal on orientation selectivity in cat V1 are unclear.In this study, we measured orientation selectivity with drifting sinusoidal gratings in a large population of cat V1 neurons by using two different quantitative measures, theNeurosci Bull October 1, 2015, 31(5): 561–571 562tuning width and the circular variance (CV). The tuning width is a local measure of tuning around the preferred orientation, whereas the CV is a global measure of the tuning curve[24]. Furthermore, we compared our data in the cat primary visual cortex to the results in macaque V1.MATERIALS AND METHODSAnimal PreparationThis study was performed in strict accordance with the recommendations in the Guideline for the Care and Use of Laboratory Animals from the National Institute of Health. The protocols were specifi cally approved by the Committee on the Ethics of Animal Experiments of the Shanghai Institute for Biological Sciences, Chinese Academy of Sciences (Permit Number: ER-SIBS-621001C).Acute experiments were performed on 12 adult cats of both sexes (the same animals were also used for other parallel projects). Detailed descriptions of animal surgery, anesthesia, and recording techniques can be found in previous studies [25, 26]. Briefl y, cats were anaesthetized prior to surgery with ketamine hydrochloride (30 mg/kg, i.v.), and then tracheal and venous cannulations were performed. After surgery, the animal was placed in a stereotaxic frame for performing a craniotomy and conducting neurophysiological procedures. During recording, anesthesia and paralysis were maintained with urethane (20 mg/kg/h) and gallamine triethiodide (10 mg/kg/h), and glucose (200 mg/kg/h) in Ringer’s solution (3 mL/kg/h). Heart rate, electrocardiography, electroencephalography (EEG), end-expiratory CO2, and rectal temperature were monitored continuously. Anesthesia was considered to be sufficient when the EEG indicated a permanent sleep-like state. Corneal, eyelid, and withdrawal reflexes were tested at appropriate intervals. Additional urethane was given immediately if necessary. The nictitating membranes were retracted and the pupils dilated. Contact lenses and additional corrective lenses were applied to focus the retina on a screen during stimulus presentation. At the end of the experiment, the animal was sacrificed by an overdose of barbiturate administered intravenously.Single-Unit RecordingsExtracellular recordings were made from 168 neurons in the primary visual cortex of anaesthetized cats using tungsten-in-glass microelectrodes with exposed tips 5–10 μm in length, and 1–2 μm in diameter[27]. The electrodes were advanced into the cortex via a step-motor micro-drive (Narishige, Japan) vertically penetrating the cortical layers. The signal was amplified and band-pass filtered (0.3–10 kHz). Spikes were discriminated with a hardware window discriminator and time-stamped with an accuracy of 1 ms using our own data acquisition system. Only well-isolated cells satisfying strict criteria (fi xed shape of action potential and the absence of spikes during the absolute refractory period) for single-unit recordings were collected for further analyses. Spikes were analyzed both during experiments and off-line using standard software packages and customized software written specifi cally for the purpose.Visual StimulationVisual stimuli were generated by a Cambridge Systems VSG graphics board. The stimuli were patches of drifting sinusoidal gratings presented on a high-resolution monitor screen (40 cm × 30 cm) at a 100-Hz vertical refresh rate. The screen was kept at the same mean luminance as the stimulus patches (10 cd/m2). The monitor was placed 57 cm from the cat’s eyes. All recordings were from the area of cortex representing the central 10° of the visual fi eld.ProceduresWhen the single-cell action potentials were isolated, the basic attributes of the cell were measured, including orientation tuning, spatial and temporal frequency tuning, and response function. Each cell was stimulated monocularly via the dominant eye and characterized by measuring its response to conventional drifting sinusoidal gratings (the nondominant eye was occluded).To locate the center of the classical receptive field (CRF), a narrow rectangular sine-wave grating patch (0.5°–1.0° wide × 3.0°–5.0° long at 40% contrast) was moved at successive positions along axes perpendicular or parallel to the optimal orientation of the cell, and the responses to its drift were measured. The grating was set at the optimal orientation and spatial frequency and drifted in the preferred direction at the optimal speed for individual recorded cells. The peak of the response profiles for both axes was defi ned as the center of the CRF.We further confi rmed that the stimulus was positioned in the center of the receptive field by performing anTao Xu, et al. Orientation selectivity in cat V1563occlusion test, in which a mask consisting of a circularblank patch and concentric with the CRF was graduallyincreased in size on a background drifting grating[28, 29]. Ifthe center of the CRF was accurately determined, the maskcurve would begin at the peak, and the response decreaseas more of the receptive field was masked. If the curveobtained with the mask did not begin at the peak value,we considered the stimulus to be offset in relation to thereceptive fi eld center, and the position of the receptive fi eldwas reassessed.Once the center of each cell’s receptive field and theexcitatory receptive field were identified, the orientationtuning of the neuron was re-measured with a 40% contrastgrating at a fixed diameter of the CRF. The orientationtuning curves were obtained using angular steps of 15°.Contrast in the subsequent experiments was selectedto elicit responses that reached ~90% of the saturationresponse for each cell with the center (CRF) contrastresponse function. Our contrast had a range of 20%–70%and a mode of 40%.Modulation RatioThe modulation ratio (MR) of the response to driftingsinusoidal gratings was calculated as MR = F1/F0, whereF0 is the mean firing rate (DC) of the response and F1the magnitude of the first harmonic of the response,corresponding to the temporal frequency of the grating(usually 3 Hz)[30].Data Analysis and StatisticsTo study orientation selectivity across a large populationof neurons, it is useful to have a single number for eachorientation-tuning curve that quantifies the degree ofselectivity of the neuron. We used two different measuresin our analysis. The fi rst measure of selectivity was the CVof the response [24, 31, 32].The CV was calculated from orientation tuning curveas follows. We measured the mean spike rate, rk, inresponse to a grating drifting with angle θk . The angle θkranged from 0° to 360° at equally-spaced intervals. From these data, the CV was defi ned asThe CV ranges from 1 for a completely non-orientated (flat) curve to 0 for an exceptionally oriented curve (zeroresponse at all orientations except the preferred one).The other measure of selectivity we used was the tuning curve width at half-height (WHH) as used previously[24,33]. The orientation tuning curves were fitted with the von Mises distribution:R=Ro+R1e k[cos2 (Ori-Ori p)-1]where R represents the response of the cell as a functionof orientation (Ori), and R1, R, Orip, and k are free parameters[24]. We fit the raw data rather than the mean response at each orientation. The preferred orientation wasdefined as the peak of the fitted function (Orip). The WHH of the fi tted function was used to describe the tuning width, which was calculated as follows:WHH=arccos[(In0.5+k)/k]The two measures, CV and WHH, provide different information about the shape of the tuning curve. CV is a global measure that is influenced by all of the data points on the tuning curve. WHH is a more local measure that depends on the shape of the curve around its peak and is not sensitive at all to the shape of the curve lying below 1/2 of the peak response.The selectivity measures were calculated based on the mean spike rate of the neurons during the response to a visual stimulus. For simple cells, one could also define a similar measure with the first harmonic amplitude (F1) of the response. We did not subtract the spontaneous rate of the responses from the visually-driven responses before the calculation of CV and bandwidth. The statistical signifi cance of the experimental data was evaluated using Student’s t-test. In all analysis, P <0.05 was considered to be statistically signifi cant.RESULTSWe completed quantitative tests and analysis of 168 single neurons at eccentricities within 10° of the visual axis. The majority of the cells were recorded in superfi cial and intermediate layers.WHH and CV in the V1 PopulationThere was a wide variation in orientation selectivity in cat V1 (Fig. 1). The mean WHH was 45.6 ± 26.9°. This was consistent with previous findings on tuning width in cat V1 by Watkins and Berkley[16] and Chen et al. [33], and wasNeurosci Bull October 1, 2015, 31(5): 561–571564also similar to observations in the primary visual cortex of anaesthetized and alert monkeys[35, 36].The distribution over the entire CV range was rather flat. The mean CV was 0.44 ± 0.26, generally consistent with the results in anaesthetized and alert monkey V1[21, 36].To better understand the relationship between CV and WHH, we constructed a scatter plot of CV versus WHH (Fig. 2), and found that they were strongly correlated in catV1 neurons (r = 0.60, P <0.001), also consistent with the results in anaesthetized and alert monkeys [21, 36].The correlation was strong for CV <0.2 where WHHs were <40°; for larger CVs, the correlation was not so high. It is often the case that a narrow WHH is associated with many different CV values.Orientation tuning curves based on spike counts forrepresentative neurons are also displayed in Fig. 2. InFig. 1. Distribution of orientation selectivity in the V1 population. A. Distribution of WHH in the V1 population. The arrow indicates themean WHH of cells. B. Distribution of CV for the V1 population. The arrow indicates the mean of CV of cells.Fig. 2. Relationship between WHH and CV. Left panel, scatterplot of orientation WHH and CV for all neurons in the measured V1population. A–D in the right panel, examples of individual tuning curves in different locations of the scatterplot. The x-axis represents stimulus orientation, from 0 to 180°. The y-axis is the response of the cell in spikes per second. The dashed line represents the spontaneous rate of firing.Tao Xu, et al. Orientation selectivity in cat V1565some cases, the WHHs were similar, while the CVs were quite different (Fig. 2A, B), while in other cases the CVs were similar, but the WHHs were quite different (Fig. 2C, D). This disagreement between CV and WHH indicates that the two measures reflect different aspects of orientation selectivity: WHH depends on the local shape of the tuning curve around the peak, whereas CV weighs the global responses at all orientations.Comparison of CV and WHH with the O/P Ratio Previous experiments[21] in anaesthetized monkeys found that the O/P ratio is strongly correlated with CV,but less with bandwidth. Here, we compared the CV and WHH with the O/P ratio in cat V1. Scatter plots of the O/P ratio versus CV clearly showed that they were strongly correlated (r = 0.78, P <0.001) (Fig. 3A). Besides, there was a lower correlation (r = 0.47, P <0.001) between WHH and O/P ratio (Fig. 3B). These results suggest that the neuronal factors that cause low values of O/P ratios also lead to low values of CV, and these observations are similar to a report in anaesthetized monkeys[21]. Because of the finding that a low response far from the preferred orientation is crucial for a low CV, we next considered the effect of spontaneous activity on CV and WHH.Relationship between Selectivity and Spontaneous ActivityThere was a stronger correlation (r = 0.44, P<0.001)Fig. 3. Relationship between orientation selectivity and orthogonal/preferred orientation response ratio (O/P ratio) or spontaneous fi ring rate. A. Relationship between CV and O/P ratio. There is strong correlation between CV and O/P ratio in all samples. B.Relationship between WHH and O/P ratio. There is a moderate correlation between WHH and O/P ratio. C. Relationship between spontaneous fi ring rate and CV. D. Relationship between WHH and spontaneous fi ring rate.Neurosci Bull October 1, 2015, 31(5): 561–571566between spontaneous firing rate and CV (Fig. 3C) and a weaker correlation (r = 0.38, P <0.001) between spontaneous fi ring rate and WHH (Fig. 3D).CV, WHH, Spontaneous Activity, and the Orthogonal ResponseAdditional analysis of the population data revealed thatother factors determine orientation selectivity besides those that determine spontaneous firing rate. We plotted the orthogonal orientation firing rate versus the spontaneous firing (Fig. 4). Cells with zero spontaneous rates were plotted with y -coordinate 0.1. Cells with zero orthogonal response were plotted with an x -coordinate of 0.1. For this group of neurons (21 cells), both the spontaneous and orthogonal fi ring rates were zero.Cells with low CVs were located above the diagonal, which confirms the role of inhibition of non-preferred orientation in the generation of high orientation selectivity (Fig. 4A). Cells with narrow tuning widths were also located above the diagonal, which implies that cortico-cortical suppression could also contribute to narrowing the tuning width (Fig. 4B).Laminar Distribution of Orientation Selectivity of NeuronsBecause of the uncertainty regarding the exact laminar position of our recording sites, we assigned laminar location by pooling those neurons estimated to be in the super fi cial layers (<600 μm), the intermediate layers (600–1200 μm), and the deep layers (>1200 μm)[37]. We illustrate the difference in laminar distribution focusing on the super fi cial and intermediate layers (because most of the cells were recorded in these layers). We found that the orientationWHH and CV were not uniformly distributed across the cortical layers, with a higher proportion of non-selective cells in the middle layers, and higher orientation selectivity in the super fi cial layers (Fig. 5).A scatter plot of WHH versus depth in the cortex is shown in Fig. 5A and curves that depict descriptive statistical measures of the population data in Fig. 5B. The curves show the moving mean of WHH through the depth of the cortex using a window width of 100 μm. The curve was obtained by selecting, at each cortical depth, all of the data points from cells that were no more than 50 μm above and below and then computing the median of their WHHs. For WHH, there was a clear alteration of orientation selectivity with the layer of origin. Cells in middle layer had broader orientation tuning, while cells in the superficial layer showed sharper orientation tuning.The orientation CV throughout all layers of cat V1, as the laminar scatter plot and statistical measures are shownin Fig. 5C and D. Although the WHH and CV of a single cellFig. 4. Scatter plot for the orthogonal response (x -axis) versus thespontaneous firing rate (y -axis). The diagonal represents when the response at the orthogonal was equal to the spontaneous fi ring rate. A. CV as a function of spontaneous fi ring rate and the response at the orthogonal. The size of each data point corresponds to the CV of the tuning curve of the neuron as illustrated by the scale on the right. Points above the diagonal indicate that the cells have strong orientation selectivity, points below it indicate they have weak orientation selectivity. B. The size of each data point corresponds to the WHH of the tuning curve as illustrated by the scale on the right. The fi gure shows that cells with a narrow WHH are also located above the diagonal.Tao Xu, et al. Orientation selectivity in cat V1567need not agree in their assessment of selectivity (Fig. 2), there was some concordance in the laminar patterns for the two different measures. Cells in the middle layers had higher CVs, while cells in the super fi cial layers had lower CVs.Relationships of CV and WHH with MRV1 neurons differ in the temporal modulation of the response to a drifting sinusoidal grating that is quantified by the F1/F0 ratio [30], with simple cells exhibiting values >1.0, and complex cells having ratios <1.0. However, it has been reported recently that the MR, when derived from the subthreshold membrane potential instead of from spike rate, is unimodally distributed [38]. So, we studied the correlation between measures of orientation selectivity and the MR (continuous distribution).Interestingly, there were almost equal correlations of CV (r = –0.41, P <0.001) and WHH (r = –0.42, P <0.001)with the MR (Fig. 6A, B), different from the results in anaesthetized monkeys [21].Relationships of O/P Ratio and Spontaneous Activity with MRBecause of the indication that the MR is crucial for CV and WHH, we next considered whether MR affects the O/P ratio or spontaneous activity. We found a negative correlation between the two values (r = –0.32, P <0.001) (Fig. 6C). There was also a negative correlation between spontaneous activity and MR (r = –0.36, P <0.001) (Fig. 6D).DISCUSSIONDiversityOur data point to the wide diversity of orientation selectivityFig. 5. Laminar analyses of WHH and CV for the V1 population. A. Plot of WHH against relative cortical depth. Each point represents a cell (n= 168). B. Statistical summary of the scatterplot data in A. The curve represents the mean bandwidth at different cortical depths. A window size of 100 μm, centered at each location, was used. C. Plot of CV against relative cortical depth. D. Statistical summary of the scatter plot data in C. Shaded area denotes ± SD in each bin. The cells located in layers >1800 μm are not shown because their number was small.Neurosci Bull October 1, 2015, 31(5): 561–571 568in the population of cat V1 neurons, especially in the CV data, which are in agreement with the findings in V1 neurons in anaesthetized macaques[21].The factors that control WHH are likely to be different from those determining CV. We found a stronger correlation between CV and the O/P ratio than WHH and the O/P ratio (Fig. 3). Thus, the diversity of the relative heights of the plateau and peak would strongly infl uence the diversity of CV, whereas the aspect ratio of the feedforward input might affect the variability of WHH[1, 18, 19].A previous study reported that suppression far from the peak could account for low values of CV[21, 22]. In the present study, we showed that cortico-cortical suppression also contributed to the narrowing of the WHH (Fig. 4B).MR and Orientation SelectivityWe found that cells with a low MR had a higher CV and WHH than those with a high MR (Fig. 6A, B). Neurons with a high MR are usually called simple cells, and those with a low MR are called complex cells. Previous studies have reported that simple cells have narrower tuning than complex cells in cat V1[16, 17], simple cells have a higher orientation selectivity index (OSI, equal to 1 − CV) than complex cells[36, 37], and the orientation tuning width is linearly related to the OSI (r = 0.54, P <0.001)[39]. These fi ndings are similar to our results.McLaughlin et al. (2000)[40] and Wielaard et al. (2001)[41] proposed that simple cells might receive more intracortical inhibition, which would be consistent with the lower spontaneous fi ring rate and orthogonal response rate. There is another possibility. A recent study based on the model[42] reported that well-defi ned LGN spike trains reduce the noise and elevate the response to the preferred stimulus. It is known that the relay cells are distributed nonrandomly in the LGN of cats and monkeys[43-47]. So the alignment of the receptive fields of relay cells in the LGN[1, 5, 7, 8] could alsoFig. 6. Relationship between modulation ratio and CV, WHH, O/P ratio, or spontaneous rate. A, CV. B, WHH. C, O/P ratio. D, spontaneousrate.Tao Xu, et al. Orientation selectivity in cat V1569explain the lower spontaneous response. However, there is perhaps only one possibility to explain the low spontaneous rate of complex cells: the strong cortico-cortical inhibition.Comparisons with Other SpeciesThe diversity of orientation selectivity in cat V1 reported here is generally consistent with the results from anaesthetized macaque V1[21]. Interestingly, CV and WHH were signifi cantly correlated with the MR, which is different from the results in anaesthetized macaque V1[21]. Our results showed that the neurons of high MR (simple cells) had significantly narrower WHH than the neurons of low MR (complex cells) in cat V1 (Fig. 6B).A type of simple-cell-like, orientation-tuned inhibitory neuron (driven by the thalamus)[48] is the main inhibitory component to form the orientation selectivity of simple cells in cat V1. Although both tuned and global sources of inhibition contribute to the orientation dynamics in monkeys[49], only the interneuron with bordering tuning for orientation has been reported in monkeys[50]. Moreover, in recent years, studies[3, 22, 23] have mainly focused on global inhibition (driven by the cortex) which has a crucial influence on the generation of highly orientation-selective cells (global measure for selectivity - CV) in monkey V1, and the effect of tuned suppression narrowing bandwidth in the most highly tuned cells has seldom been studied[49].Using genetic and imaging tools, recent evidence for orientation selectivity in mouse V1 has not found inhibitory neurons with simple-cell-like RFs, but has found interneurons with complex-cell-like RFs and hardly any orientation selectivity[51]. More evidence shows that the push-pull circuit (in cat V1)[1, 52, 53] cannot be a major synaptic mechanism underlying simple RFs in mouse V1 and the inhibitory circuit (driven by cortex) may contribute in a different way than in cat V1 to form orientation selectivity in simple cells[51, 54, 55].Highly orientation-selective neurons (simple cells in layer 4) are already present in the first stage of cortical processing in cat V1[56], and their generation of orientation tuning relies on a substantial feed-forward mechanism[1, 7, 57, 58]. Although this is true for other carnivores[59], it is not a universal rule. For example, cells in layer 4 of the tree shrew cortex are not tuned to stimulus orientation[60], but the orientation selectivity in layer 2/3 of the tree shrew appears to depend on the axial bias in its feed-forward input from layer 4, further refi ned by the intracortical circuits[61].In primates, the parvo- and magno-cellular pathways remain separate in the LGN. Parvo- and magno-cellular relay cells target different sub-laminae of cortical layer 4C[62]. Cortical cells in the magnocellular stream have orientation-selective responses[63], similar to the organization in cats. However, the poor orientation tuning in the parvocellular stream may be similar to the organization in the tree shrew [62].In future work, it will be necessary to analyze the inhibitory and excitatory input to cells in layer 4C of primates. Perhaps many of the same arguments that we make here for the cat visual cortex might apply to the primate visual cortex.ACKNOWLE D GMENTSThis work was supported by the National Basic Research Development Program of China (2013CB329401), the National Natural Science Foundation of China (91420105, 61105116, 91120013, and 90820301), and Shanghai Municipal Committee of Science and Technology (088014158 and 098014026). We thank Dr. D. A. Tigwell for comments on the manuscript and X. Z. Xu for technical assistance. We also thank Dr. L. Wang and Y. C. Cai for help with the stimulus and analysis programs.Received date: 2014-11-23; Accepted date: 2015-04-13REFERENCES[1] Hubel DH, Wiesel TN. Receptive fi elds, binocular interactionand functional architecture in the cat’s visual cortex. J Physiol 1962, 160: 106–154.[2] Hubel DH, Wiesel TN. Receptive fields and functionalarchitecture of monkey striate cortex. J Physiol 1968, 195: 215–243.[3] Shapley R, Hawken M, Ringach DL. Dynamics of orientationselectivity in the primary visual cortex and the importance of cortical inhibition. Neuron 2003, 38: 689–699.[4] Priebe NJ, Ferster D. Inhibition, spike threshold, and stimulusselectivity in primary visual cortex. Neuron 2008, 57: 482–497.[5] Chapman B, Zahs K, Stryker MP. Relation of cortical cellorientation selectivity to alignment of receptive fields of the geniculocortical afferents that arborize within a single orientation column in ferret visual cortex. J Neurosci 1991, 11: 1347–1358.[6] Nelson S, Toth L, Sheth B, Sur M. Orientation selectivity of。