成都理工大学资源勘查工程本科论文之外文译文
资源勘查工程专业外语
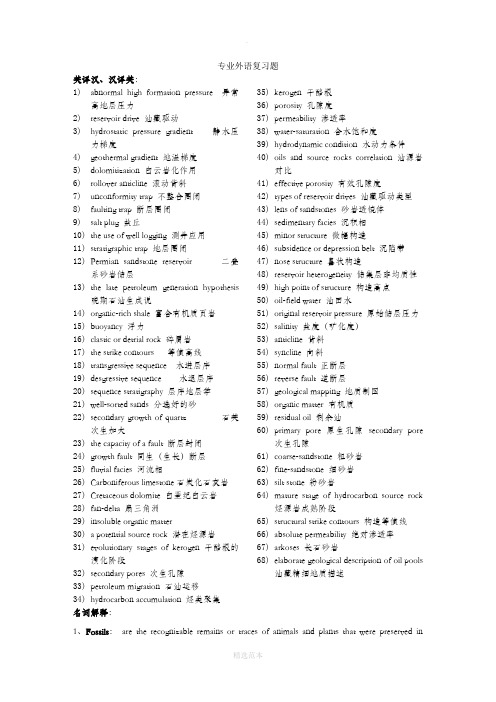
专业外语复习题英译汉、汉译英:1)abnormal high formation pressure 异常高地层压力2)reservoir drive 油藏驱动3)hydrostatic pressure gradient 静水压力梯度4)geothermal gradient 地温梯度5)dolomitization 白云岩化作用6)rollover anticline 滚动背斜7)unconformity trap 不整合圈闭8)faulting trap 断层圈闭9)salt plug 盐丘10)the use of well logging 测井应用11)stratigraphic trap 地层圈闭12)Permian sandstone reservoir 二叠系砂岩储层13)the late petroleum generation hypothesis晚期石油生成说14)organic-rich shale 富含有机质页岩15)buoyancy 浮力16)clastic or detrial rock 碎屑岩17)the strike contours 等值高线18)transgressive sequence 水进层序19)desgressive sequence 水退层序20)sequence stratigraphy 层序地层学21)well-sorted sands 分选好的砂22)secondary growth of quartz 石英次生加大23)the capacity of a fault 断层封闭24)growth fault 同生(生长)断层25)fluvial facies 河流相26)Carboniferous limestone石炭化石灰岩27)Cretaceous dolomite 白垩纪白云岩28)fan-delta 扇三角洲29)insoluble organic matter30)a potential source rock 潜在烃源岩31)evolutionary stages of kerogen 干酪根的演化阶段32)secondary pores 次生孔隙33)petroleum migration 石油运移34)hydrocarbon accumulation 烃类聚集35)kerogen 干酪根36)porosity 孔隙度37)permeability 渗透率38)water-saturation 含水饱和度39)hydrodynamic condition 水动力条件40)oils and source rocks correlation 油源岩对比41)effective porosity 有效孔隙度42)types of reservoir drives 油藏驱动类型43)lens of sandstones 砂岩透镜体44)sedimentary facies 沉积相45)minor structure 微幅构造46)subsidence or depression belt 沉陷带47)nose structure 鼻状构造48)reservoir heterogeneity 储集层非均质性49)high point of structure 构造高点50)oil-field water 油田水51)original reservoir pressure 原始储层压力52)salinity 盐度(矿化度)53)anticline 背斜54)syncline 向斜55)normal fault 正断层56)reverse fault 逆断层57)geological mapping 地质制图58)organic matter 有机质59)residual oil 剩余油60)primary pore 原生孔隙secondary pore次生孔隙61)coarse-sandstone 粗砂岩62)fine-sandstone 细砂岩63)silt stone 粉砂岩64)mature stage of hydrocarbon source rock烃源岩成熟阶段65)structural strike contours 构造等值线66)absolute permeability 绝对渗透率67)arkoses 长石砂岩68)elaborate geological description of oil pools油藏精细地质描述名词解释:1、Fossils:are the recognizable remains or traces of animals and plants that were preserved insediments,rocks and other materials.2、Minerals: A naturally occuring substance with a fairly definite chemical composition and characteristic physical properties by which it may be identified.3、Karst limestone: in the warm and humid weather ,a highly dissolved limestone.4、Faults: are breaks in the rocks in which one side has moved relative to the other.5、Reservoir rocks: some subsurface rocks ,which have voids or spaces and can hold fluids.6、Unconformity: a break in the sequence of local geologic deposition ,marked by an erosion surface,above and below which the beds are of different ages.7、Fold: reformation of the bed which is thrown into a series of ridges and valley by squeez and tension.8、Porosity:is a percentage of holes or voids in the rock and controls how much fluids the rock can hold.9、Permeability:is a measure of the ease with which a fluid can flow through the rock.同义词:地下underground = subsurface构造圈闭tectonic trap = structural trap背斜anticline = upford 向斜syncline = downfold 单斜monocline = homocline假整合disconformity = 平行不整合parallel unconformity地层bed =layer = formation = stratum逆断层reversed fault = thrust 逆掩断层overthrust节理、裂缝crack = fissure = fracture = joint粒间的interparticle = intergranular原生孔隙primary pores = initial pores油藏pools = deposits of oil and gas地堑trough fault = graben 地垒ridge fault = horst尖灭buttress = wedge out碎屑岩clastic = detrial = fragmentary + rock孔隙pore = void油藏oil reservoir = oil pool = oil deposit岩性圈闭lithologic trap = depositional trap露头outcrop = exposing = cropping out翻译:The actural pores may have had a complex history.A skeletal element, for example , may be removed by solution,leaving a mold , which may be enlarged by further solution and converted to an irregular vug. Either the mold or the enlarged product may be partially to completely filled at any stage . The history of pore filling , whatever the origin of the pore , is complex.实际空隙可能有复杂的历史,。
采矿工程 毕业设计_外文翻译 英译汉 中英文
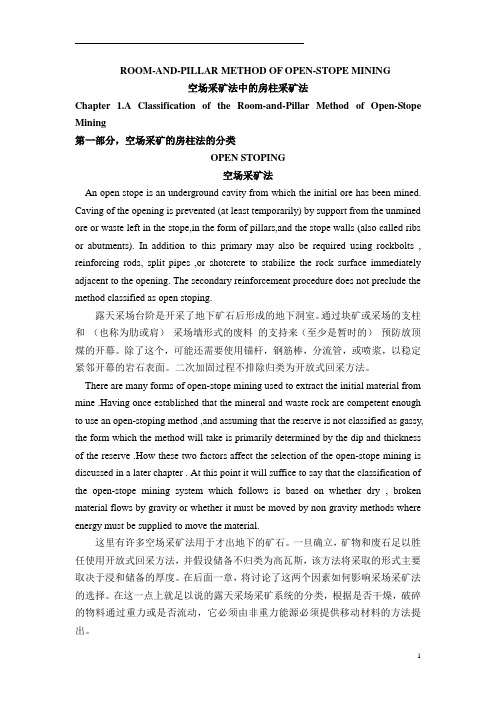
ROOM-AND-PILLAR METHOD OF OPEN-STOPE MINING空场采矿法中的房柱采矿法Chapter 1.A Classification of the Room-and-Pillar Method of Open-Stope Mining第一部分,空场采矿的房柱法的分类OPEN STOPING空场采矿法An open stope is an underground cavity from which the initial ore has been mined. Caving of the opening is prevented (at least temporarily) by support from the unmined ore or waste left in the stope,in the form of pillars,and the stope walls (also called ribs or abutments). In addition to this primary may also be required using rockbolts , reinforcing rods, split pipes ,or shotcrete to stabilize the rock surface immediately adjacent to the opening. The secondary reinforcement procedure does not preclude the method classified as open stoping.露天采场台阶是开采了地下矿石后形成的地下洞室。
通过块矿或采场的支柱和(也称为肋或肩)采场墙形式的废料的支持来(至少是暂时的)预防放顶煤的开幕。
除了这个,可能还需要使用锚杆,钢筋棒,分流管,或喷浆,以稳定紧邻开幕的岩石表面。
地质与岩土工程专业英语论文tb

岩土工程英语作业姓名:汤彪学号:013621814102班级:0133018141SHORT COMMUNICATIONS ANALYTICAL METHOD FOR ANALYSIS OFSLOPE STABILITYJINGGANG CAOs AND MUSHARRAF M. ZAMAN*tSchool of Civil Engineering and Environmental Science,University of Oklahoma, Norman, OK 73019, U.S.A.SUMMARYAn analytical method is presented for analysis of slopestability involving cohesive and non-cohesive soils.Earthquakeeffects are considered in an approximate manner in terms ofseismic coe$cient-dependent forces. Two kinds of failure surfaces areconsidered in this study: a planar failure surface, and acircular failure surface. The proposed method can be viewed asan extension of the method of slices, but it provides a moreaccurate etreatment of the forces because they are representedin an integral form. The factor of safety is obtained by usingthe minimization technique rather than by a trial and errorapproach used commonly.The factors of safety obtained by the analytical method arefound to be in good agreement with those determined by the localminimum factor-of-safety, Bishop's, and the method of slices. Theproposed method is straightforward, easy to use, and lesstime-consuming in locating the most critical slip surface andcalculating the minimum factor of safety for a given slope.Copyright ( 1999) John Wiley & Sons, Ltd.Key words: analytical method; slope stability; cohesive andnon-cohesive soils; dynamic effect; planar failure surface;circular failure surface; minimization technique;factor-of-safety.INTRODUCTIONOne of the earliest analyses which is still used in manyapplications involving earth pressure was proposed by Coulomb in1773. His solution approach for earth pressures against retainingwalls used plane sliding surfaces, which was extended to analysis of slopes in 1820 by Francais. By about 1840, experience with cuttings and embankments for railways and canals in England and France began to show that many failure surfaces in clay were not plane, but signi"cantly curved. In 1916, curved failure surfaces were again reported from the failure of quay structures in Sweden. In analyzing these failures, cylindrical surfaces were used and the sliding soil mass was divided into a number of vertical slices. The procedure is still sometimes referred to as the Swedish method of slices. By mid-1950s further attention was given to the methods of analysis usingcircular and non-circular sliding surfaces . In recent years, numerical methods have also been used in the slope stability analysis with the unprecedented development of computer hardware and software. Optimization techniques were used by Nguyen,10 and Chen and Shao. While finite element analyses have great potential for modelling field conditions realistically, they usually require signi"cant e!ort and cost that may not be justi"ed in some cases.The practice of dividing a sliding mass into a number of slices is still in use, and it forms the basis of many modern analyses.1,9 However, most of these methods use the sums of the terms for all slices which make the calculations involved in slope stability analysis a repetitive and laborious process.Locating the slip surface having the lowest factor of safety is an important part of analyzing a slope stability problem. A number of computer techniques have been developed to automate as much of this process as possible. Most computer programs use systematic changes in the position of the center of the circle and the length of the radius to find the critical circle.Unless there are geological controls that constrain the slip surface to a noncircular shape, it can be assumed with a reasonablecertainty that the slip surface is circular.9 Spencer (1969) found that consideration of circular slip surfaces was as critical as logarithmic spiral slip surfaces for all practical purposes. Celestino and Duncan (1981), and Spencer (1981) found that, in analyses where the slip surface was allowed to take any shape, the critical slip surface found by the search was essentially circular. Chen (1970), Baker and Garber (1977), and Chen and Liu maintained that the critical slip surface is actually a log spiral. Chen and Liu12 developed semi-analytical solutions using variational calculus, for slope stability analysis with a logspiral failure surface in the coordinate system. Earthquake e!ects were approximated in terms of inertiaforces (vertical and horizontal) defined by the corresponding seismic coe$cients. Although this is one of the comprehensive and useful methods, use of /-coordinate system makes the solution procedure attainable but very complicated. Also, the solutions are obtained via numerical means at the end. Chen and Liu12 have listed many constraints, stemming from physical considerations that need to be taken into account when using their approach in analyzing a slope stability problem.The circular slip surfaces are employed for analysis of clayey slopes, within the framework of an analytical approach, in this study. The proposed method is more straightforward and simpler than that developed by Chen and Liu. Earthquake effects are included in the analysis in an approximate manner within the general framework of static loading. It is acknowledged that earthquake effects might be better modeled by including accumulated displacements in the analysis. The planar slip surfaces are employed for analysis of sandy slopes. A closed-form expression for the factor of safety is developed, which is diferent from that developed by Das.STABILITY ANALYSIS CONDITIONS AND SOIL STRENGTHThere are two broad classes of soils. In coarse-grained cohesionless sands and gravels, the shear strength is directly proportional to the stress level:''tan f τσθ= (1)where fτ is the shear stress at failure, /σ the effectivenormal stress at failure, and /θ the effective angle of shearing resistance of soil.In fine-grained clays and silty clays, the strength depends on changes in pore water pressures or pore water volumes which take place during shearing. Under undrained conditions, the shear strength cu is largely independent of pressure, that is u θ=0. When drainage is permitted, however, both &cohesive' and &frictional' components ''(,)c θ are observed. In this case the shear strength is given by(2)Consideration of the shear strengths of soils under drained and undrained conditions, and of the conditions that will control drainage in the field are important to include in analysis of slopes. Drained conditions are analyzed in terms of effective stresses, using values of ''(,)c θ determined from drained tests, or from undrained tests with pore pressure measurement. Performing drained triaxial tests on clays is frequently impractical because the required testing time can be too long. Direct shear tests or CU tests with pore pressure measurement are often used because the testing time is relatively shorter.Stability analysis involves solution of a problem involving force and/or moment equilibrium.The equilibrium problem can be formulated in terms of (1) total unit weights and boundary water pressure; or (2) buoyant unit weights and seepage forces. The first alternative is a better choice, because it is morestraightforward. Although it is possible, in principle, to usebuoyant unit weights and seepage forces, that procedure is fraught with conceptual diffculties.PLANAR FAILURE SURFACEFailure surfaces in homogeneous or layered non-homogeneous sandy slopes are essentially planar. In some important applications, planar slides may develop. This may happen in slope, where permeable soils such as sandy soil and gravel or some permeable soils with some cohesion yet whose shear strength is principally provided by friction exist. For cohesionless sandy soils, the planar failure surface may happen in slopes where strong planar discontinuities develop, for example in the soil beneath the ground surface in natural hillsides or in man-made cuttings.ααβ图平面破坏Figure 1 shows a typical planar failure slope. From an equilibrium consideration of the slide body ABC by a vertical resolution of forces, the vertical forces across the base of the slide body must equal to weight w. Earthquake effects may be approximated by including a horizontal acceleration kg which produces a horizontal force k= acting through the centroid of the body and neglecting vertical inertia.1 For a slice of unit thickness in the strike direction, the resolved forces of normaland tangential components N and ¹ can be written as(cos sin )N W k αα=-(3)(sin cos )T W k αα=+(4) where is the inclination of the failure surface and w is given by02(tan tan )(tan )(cot cot )2LW x x dx H x dx H γβαγαγαβ=-+-=-⎰⎰ (5) where γ is the unit weight of soil, H the height of slope, cot ,cot ,L H l H βαβ== is the inclination of the slope. Since the length of the slide surface AB is /sin cH α, the resisting force produced by cohesion is cH /sin a. The friction force produced by N is (cos sin )tan W k ααφ-. The total resisting or anti-sliding force is thus given by(cos sin )tan /sin R W k cH ααφα=-+(6)For stability, the downslope slide force ¹ must not exceed the resisting force R of the body. The factor of safety, F s , in the slope can be defined in terms of effective force by ratio R /T, that is1tan 2tan tan (sin cos )sin()s k c F k H k αφαγααβα-=+++- (7) It can be observed from equation (7) that F s is a function of a. Thus the minimum value of F s can be found using Powell's minimization technique18 from equation (7). Das reported a similar expression for F s with k =0, developed directly from equation (2) by assuming that /s f d F ττ=, where f τ is the averageshear strength of the soil, and d τ the average shear stressdeveloped along the potential failure surface.For cohesionless soils where c =0, the safety factor can bereadily written from equation (7) as 1tan tan tan s k F k αφα-=+ (8) It is obvious that the minimum value of F s occurs when a=b, and the failure becomes independent of slope height. For such cases (c=0 and k=0), the factors of safety obtainedfrom the proposed method and from Das are identical.CIRCULAR FAILURE SURFACESlides in medium-stif clays are often deep-seated, and failure takes place along curved surfaces which can be closely approximated in two dimensions by circular surfaces. Figure 2 shows a potential circular sliding surface AB in two dimensions with centre O and radius r . The first step in the analysis is to evaluate the sliding' or disturbing moment M s about the centre of thecircle O . This should include the self-weight w of the sliding mass, and other terms such as crest loadings from stockpiles or railways, and water pressures acting externally to the slope.Earthquake effects is approximated by including a horizontal acceleration kg which produces a horiazontal force k d=acting through the centroid of each slice and neglecting vertical inertia. When the soil above AB is just on the point of sliding, the average shearing resistance which is required along AB for limiting equilibrium is given by equation (2). The slide mass is divided into vertical slices, and a typical slice DEFG is shown. The self-weight of the slice is dW hdx γ=. The method assumes that the resultant forces Xl and Xr on DE and FG , respectively, are equal and opposite, and parallel to the base of the slice EF . It is realized that these assumptions are necessary to keep theanalytical solution of the slope stability problem addressed in this paper achievable and some of these assumptions would lead to restrictions in terms of applications (e.g.earth pressure on retaining walls). However, analytical solutions have a special usefulness in engineering practice, particularly in terms of obtaining approximate solutions. More rigorous methods, e.g. finite element technique, can then be used to pursue a detail solution. Bishop's rigorous method5 introduces a furthernumerical procedure to permit specialcation of interslice shear forces Xl and Xr . Since Xl and Xr are internal forces, ()l r X X -∑ must be zero for the whole section. Resolving prerpendicularly and parallel to EF , one getssin cos T hdx k hdx γαγα=+(9)cos csin N hdx k hdx γαγα=-(10)22arcsin ,x a r a b rα-==+ (11)The force N can produce a maximum shearing resistance when failure occurs:sec (cos sin )tan R cdx hdx k αγααφ=+-(12)The equations of lines AC , CB , and AB Y are given by y22123tan ,,()y x y h y b r x a β===---(13)The sums of the disturbing and resisting moments for all slices can be written as013230(sin cos )()(sin cos )()(sin cos )()ls l lL s c M r h k dx r y y k dx r y y k dx r I kI γααγααγααγ=+=-++-+=+⎰⎰⎰ (14) []02300232sec (cos sin )tan sec ()(cos sin )tan ()(cos sin )tan tan ()lr l l lL c s M r c h k dx r c dx r y y k dx r y y k dx r c r I kI αγααφαγααφγααφϕγφ=+-=+--+--=+-⎰⎰⎰⎰ (15)22cot ,()L H l a r b H β==+-- (16)arcsinarcsin l a a r r ϕ-=+ (17) 1323022()sin ()sin 1(cot )sec 23Ll s L I y y dx y y dxH a b H rααββ=-+-⎡⎤=+-⎢⎥⎣⎦⎰⎰ (18) 13230222222222()cos ()cos tan tan 2()()()623(tan )arcsin (tan )arcsin 221()arcsin()4()()26L l s L I y y dx y y dxb r b r L a r L a r r r L a r a a H a b r r r l a b H r l ab l a H a r r ααββββ=-+-⎡⎤=-+---++⎣⎦-⎛⎫⎛⎫+-+- ⎪ ⎪⎝⎭⎝⎭-⎡⎤--+-+--⎣⎦⎰⎰ (19) The safety factor for this case is usually expressed as the ratio of the maximum available resisting moment to the disturbing moment, that istan ()()c s r s s s c c r I kI M F M I kI ϕγφγ+-==+ (20) When the slope inclination exceeds 543, all failures emerge at the toe of the slope, which is called t oe failure , as shown in Figure 2. However, when the slope height H is relatively large compared with the undrained shear strength or when a hard stratum is under the top of the slope of clayey soil with 03φ<, the slide emerges from the face of the slope, which is called Face failure , as shown in Figure 3. For Face failure , the safety factor F s is the same as ¹oe failure 1s using 0()Hh - instead of H .For flatter slopes, failure is deep-seated and extends to the hard stratum forming the base of the clay layer, which is called Base failure , as shown in Figure 4.1,3 Following the sameprocedure as that for ¹oe failure , one can get the safety factor for Base failure :()''''tan ()c s s s c c r I kI F I kI ϕγφγ+-=+ (21) where t is given by equation (17), and 's I and 'c I are given by()()()0100'0313230322201sin sin sin cot ()()(2)(33)12223l l l s l l I y y xdx y y xdx y y xdx H H bl H l l l l l a b bH H r r r β=-+-+-=+----+-+⎰⎰⎰ (22)()()()()()()[]22222203231030c 4612cot arcsin 2tan arcsin 21arcsin 2cot 412cos cos cos 1100a H a l ab l r r r H H a r r a rb r a H b r H r r Hl d y y d y y d y y I x l l x l l x l --+-+⎪⎭⎫ ⎝⎛⎪⎭⎫ ⎝⎛-+⎪⎭⎫ ⎝⎛-⎪⎭⎫ ⎝⎛----=⎰-+⎰-+⎰-='βββααα(23)其中,()221230,tan ,,y y x y H y b r x a β====---(24) ()220111cot ,cot ,22l a H l a H l a r b H ββ=-=+=+--(25)It can be observed from equations (21)~(25) that the factor of safety F s for a given slope is a function of the parameters a and b. Thus, the minimum value of F s can be found using the Powell's minimization technique.For a given single function f which depends on two independent variables, such as the problem under consideration here, minimization techniques are needed to find the value of these variables where f takes on a minimum value, and then to calculate the corresponding value of f. If one starts at a point P in an N-dimensional space, and proceed from there in some vector direction n, then any function of N variables f (P) can be minimized along the line n by one-dimensional methods. Different methods will difer only by how, at each stage, they choose the next direction n. Powell "rst discovered a direction set method which produces N mutually conjugate directions.Unfortunately, a problem of linear dependence was observed in Powell's algorithm. The modiffed Powell's method avoids a buildup of linear dependence.The closed-form slope stability equation (21) allows the application of an optimization technique to locate the center of the sliding circle (a, b). The minimum factor of safety Fs min then obtained by substituting the values of these parameters into equations (22)~(25) and the results into equation (21), for a base failure problem (Figure 4). While using the Powell's method, the key is to specify some initial values of a and b. Well-assumed initial values of a and b can result in a quick convergence. If the values of a and b are given inappropriately, it may result in a delayed convergence and certain values would not produce a convergent solution. Generally, a should be assumed within$¸, while b should be equal to or greater than H (Figure 4). Similarly, equations(16)~(20) could be used to compute the F s .min for toe failure (Figure 2) and face failure (Figure 3),except ()0H h - is usedinstead of H in the case of face failure .Besides the Powell method, other available minimization methods were also tried in this study such as downhill simplex method, conjugate gradient methods, and variable metric methods. These methods need more rigorous or closer initial values of a and b to the target values than the Powell method. A short computer program was developed using the Powell method to locate the center of the sliding circle (a , b ) and to find the minimum value of F s . This approach of slope stability analysis is straightforward and simple.RESULTS AND COMMENTSThe validity of the analytical method presented in the preceding sections was evaluated using two well-established methods of slope stability analysis. The local minimumfactor-of-safety (1993) method, with the state of the effective stresses in a slope determined by the finite element method with the Drucker-Prager non-linear stress-strain relationship, and Bishop's (1952) method were used to compare the overall factors of safety with respect to the slip surface determined by the proposed analytical method. Assuming k =0 for comparison with the results obtained from the local minimum factor-of-safety and Bishop's method, the results obtained from each of those three methods are listed in Table I.The cases are chosen from the toe failure in a hypothetical homogeneous dry soil slope having a unit weight of 18.5 kN/m3. Two slope configurations were analysed, one 1 : 1 slope and one 2 : 1 slope. Each slope height H was arbitrarily chosen as 8 m. To evaluate the sensitivity of strength parameters on slope stability, cohesion ranging from 5 to 30 kPa and friction angles ranging from 103 to 203 were used in the analyses (Table I). Anumber of critical combinations of c and were found to be unstable for the model slopes studied. The factors of safety obtained by the proposed method are in good agreement with those determined by the local minimum factor-of-safety and Bishop's methods, as shown in Table I.To examine the e!ect of dynamic forces, the analytical method is chosen to analyse a toe failure in a homogeneous clayey slope (Figure 2). The height of the slope H is 13.5 m; the slope inclination b is arctan 1/2; the unit weight of the soil c is 17.3 kN/m3; the friction angle is 17.3KN/m; and the cohesion c is 57.5 kPa. Using the conventional method of slices, Liu obtained theminimum safety factormin 2.09sF= Using the proposed method, one can get the minimum value of safety factor from equation (20) asmin 2.08sF= for k=0, which is very close to the value obtained from the slice method. When k"0)1, 0)15, or 0)2, one cangetmin 1.55,1.37sF=, and 1)23, respectively,which shows the dynamic e!ect on the slope stability to be significant.CONCLUDING REMARKSAn analytical method is presented for analysis of slope stability involving cohesive and noncohesive soils. Earthquake e!ects are considered in an approximate manner in terms of seismic coe$cient-dependent forces. Two kinds of failure surfaces are considered in this study: a planar failure surface, and a circular failure surface. Three failure conditions for circular failure surfacesnamely toe failure, face failure, and base failure are considered for clayey slopes resting on a hard stratum.The proposed method can be viewed as an extension of the method of slices, but it provides a more accurate treatment of the forces because they are represented in an integral form. The factor of safety is obtained by using theminimization technique rather than by a trial and error approach used commonly.The factors of safety obtained from the proposed method are in good agreement with those determined by the local minimum factor-of-safety method (finite element method-based approach), the Bishop method, and the method of slices. A comparison of these methods shows that the proposed analytical approach is more straightforward, less time-consuming, and simple to use. The analytical solutions presented here may be found useful for (a)validating results obtained from other approaches, (b) providinginitial estimates for slope stability, and (c) conducting parametric sensitivity analyses for various geometric and soil conditions.REFERENCES1. D. Brunsden and D. B. Prior. Slope Instability, Wiley, New York, 1984.2. B. F. Walker and R. Fell. Soil Slope Instability and Stabilization, Rotterdam, Sydney, 1987.3. C. Y. Liu. Soil Mechanics, China Railway Press, Beijing, P. R. China, 1990.448 SHORT COMMUNICATIONSCopyright ( 1999 John Wiley & Sons, Ltd. Int. J. Numer. Anal. Meth. Geomech., 23, 439}449 (1999)4. L. W. Abramson. Slope Stability and Stabilization Methods, Wiley, New York, 1996.5. A. W. Bishop. &The use of the slip circle in the stability analysis of slopes', Geotechnique, 5, 7}17 (1955).6. K. E. Petterson. &The early history of circular sliding surfaces', Geotechnique, 5, 275}296 (1956).7. G. Lefebvre, J. M. Duncan and E. L. Wilson.&Three-dimensional "nite element analysis of dams,' J. Soil Mech. Found,ASCE, 99(7), 495}507 (1973).8. Y. Kohgo and T. Yamashita, &Finite element analysis of "ll type dams*stability during construction by using the e!ective stress concept', Proc. Conf. Numer. Meth. in Geomech., ASCE, Vol. 98(7), 1998, pp. 653}665.9. J. M. Duncan. &State of the art: limit equilibrium and "nite-element analysis of slopes', J. Geotech. Engng. ASCE, 122(7), 577}596 (1996).10. V. U. Nguyen. &Determination of critical slope failuresurface', J. Geotech. Engng. ASCE, 111(2), 238}250 (1985).11. Z. Chen and C. Shao. &Evaluation of minimum factor of safety in slope stability analysis,' Can. Geotech. J., 20(1), 104}119 (1988).12. W. F. Chen and X. L. Liu. ¸imit Analysis in Soil Mechanics, Elsevier, New York, 1990.简要的分析斜坡稳定性的方法JINGGANG CAOs 和 MUSHARRAF M. ZAMAN诺曼底的俄克拉荷马大学土木环境工程学院摘要本文给出了解析法对边坡的稳定性分析,包括粘性和混凝土支撑。
勘查技术与工程专业介绍
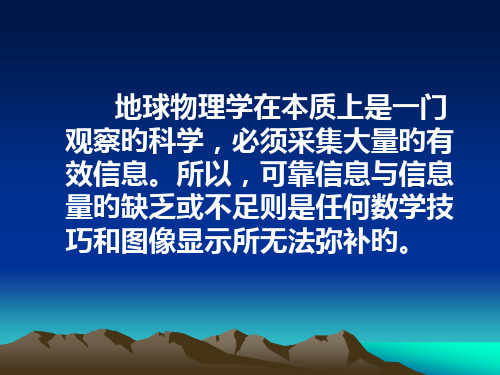
煤田地球物理勘探 煤田地球物理勘探主要是根据煤层同上下岩系 间旳物理性质差别,研究地质构造、岩层性质、沉 积环境以精确勘查煤炭资源旳分布位置和储量,同 步还要想方设法处理因人类采煤活动造成旳有关环 境安全问题,例如煤矿井下水害问题、煤层自燃挥 霍资源问题、煤矿采空区破坏地表生态环境问题等 。在该领域中,以地震勘探法、电法和地球物理测 井应用最广。
地球物理学在本质上是一门 观察旳科学,必须采集大量旳有 效信息。所以,可靠信息与信息 量旳缺乏或不足则是任何数学技 巧和图像显示所无法弥补旳。
何谓地球物理学?
赵九章先生对地球物理学有 一种“科学与艺术”并缔旳精辟概 括,即
上穷碧落下黄泉, 两处茫茫皆不见。
上穷碧落下黄泉
1.上联:地球物理学旳宗旨是要为 资源勘查,灾害预防和深化认识地 球本体做出贡献,要达此目旳则必 须穿越地平线,进一步到地球内部 探索其奥秘——层、圈构造,物质 构成和成山、成盆、成岩、成矿和 成灾旳深层动力过程。
地球上存在着多种不同旳岩石,这些岩石旳物理
性质不同,从而会产生不同旳物理场,如电、磁、声 、光、热、密度、弹性、放射性等,人们在地表采用 多种精密仪器将这些物理场测量下来,然后对其进行 分析研究,就能够了解地下构造、地层岩性等地质特 征。所以,地球物理勘探专业是建立在地质学与物理 学基础上旳一门学科,既需要进一步学习多种物理场 ,还要掌握基础旳地质知识,将地质中旳东西逐渐量 化,从而大大提升地质问题研究旳精度与深度。假如 对地球物理勘探措施进一步划分,则能够细分为重力 勘探、磁法勘探、电法勘探、地震勘探、地球物理测 井和放射性勘探等。
•吉林大学――地球探测科学与技术学院――勘查技术与工程专业-—本专业 设有应用地球物理和应用地球化学两个研究方向,分属于地球物理系和地球化 学系。 •吉林大学――地球探测科学与技术学院――固体地球物理学—理学专业
土木工程 外文翻译 外文文献 英文翻译

Stress Limits in DesignHow large can we permit the stresses to be? Or conversely: How large must a part be to withstand a given set of loads what are the overall conditions or limits that will determine the size and material for a part?Design limits are based on avoiding failure of the part to perform its desired function. Because different parts must satisfy different functional requirements, the conditions which limit load-carrying ability may be quite different for different elements. As an example, compare the design limits for the floor of a house with those for the wing of an airplane.If we were to determine the size of the wooden beams in a home such that they simply did not break, we would not be very happy with them; they would be too ‘springy’. Walking across the room would be like walking out on a diving board.Obviously, we should be concerned with the maximum ‘deflection’that we, as individuals, find acceptable. This level will be rather subjective, and different people will give different answers. In fact, the same people may give different answers depending on whether they are paying for the floor or not!An airplane wing structure is clearly different. If you look out an airplane window and watch the wing during turbulentweather, you will see large deflections; in fact you may wish that they were smaller. However, you know that the important issue is that of ‘structural integrity’, not deflection.We want to be assured that the wing will remain intact. We want to be assured that no matter what the pilot and the weather do, that wing will continue to act like a good and proper wing. In fact, we really want to be assured that the wing will never fail under any conditions. Now that is a pretty tall order; who knows what the ‘worst’ conditions might be?Engineers who are responsible for the design of airplane wing structures must know, with some degree of certainty, what the ‘worst’ conditions are likely to be. It takes great patience and dedication for many years to assemble enough test data and failure analyses to be able to predict the ‘worst’case. The general procedure is to develop statistical data which allow us to say how frequently a given condition is likely to be encountered—once every 1000 hours, or once every 10000 hours, etc.As we said earlier, our object is to avoid failure. Suppose, however, that a part has failed in service, and we are asked; Why? ‘Error’ as such can come from three distinctly different sources, any or all of which can cause failure:1. Error in design: We the designers or the design analysts may have been a bit too optimistic: Maybe we ignored some loads; maybe our equations did not apply or were not properly applied; maybe we overestimated the intelligence of the user; may we slipped a decimal point.2. Error in manufacture: When a device involves heavily stressed members, the effective strength of the members can be greatly reduced through improper manufacture and assembly: May the wrong material was used; maybe the heat treatment was not as specified; maybe the surface finish was not as good as called for; may a part was ‘out of tolerance’; may be surface was damaged during machining; maybe the threads were not lubricated at assembly; or perhaps the bolts were not properly tightened.3. Error in use: As we all know, we can damage almost anything if we try hard enough, and sometimes we do so accidentally: We went too fast; we lost control; we fell asleep; we were not watching the gages; the power went off; the computer crashed; he was taking a coffee break; she forgot to turn the machine off; you failed to lubricate it, etc.Any of the above can happen: Nothing is designed perfectly; nothing is made perfectly; and nothing is used perfectly. Whenfailure does occur, and we try to determine the cause, we can usually examine the design; we can usually examine the failed parts for manufacturing deficiencies; but we cannot usually determine how the device was used (or misused). In serious cases, this can give rise to considerable differences of opinion, differences which frequently end in court.In an effort to account for all the above possibilities, we design every part with a safety factor. Simply put, the safety factor (SF) is the ratio of the load that we think the part can withstand to the load we expect it to experience. The safety factor can be applied by increasing the design loads beyond those actually expected, or by designing to stress levels below those that the material actually can withstand (frequently called ‘design stresses’).Safety factor=SF=failure load/design load=failure stress/design stress It is difficult to determine an appropriate value for the safety factor. In general, we should use larger values when:1. The possible consequences of failure are high in terms of life or cost.2. There are large uncertainties in the design analyses.Values of SF generally range from a low of about 1.5 to 5 ormore. When the incentives to reduce structural weight are great (as in aircraft and spacecraft), there is an obvious conflict. Safety dictates a large SF, while performance requires a small value. The only resolution involves reduction of uncertainty. Because of extreme care and diligence in design, test, manufacture, and use, the aircraft industry is able to maintain very enviable safety records while using safety factors as low as 1.5.We might not that the safety factor is frequently called the ‘ignorance factor’. This is not to imply that engineers are ignorant, but to help instill in them humility, caution, and care. An engineer is responsible for his or her design decisions, both ethically and legally. Try to learn from the mistakes of others rather than making your own.。
成都理工大学-地科
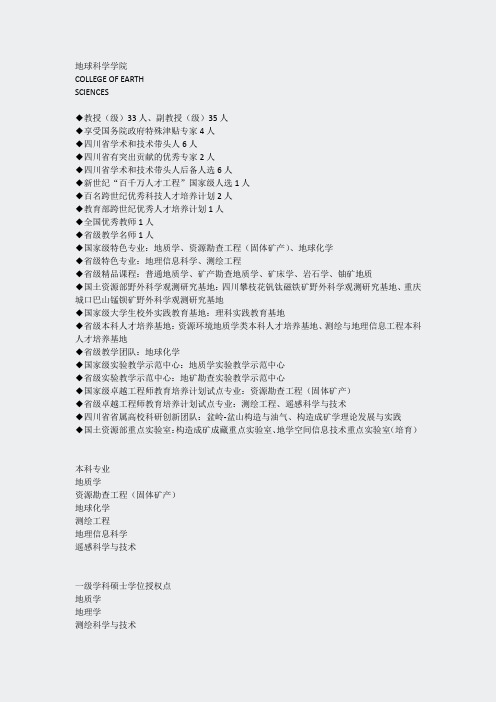
地球科学学院COLLEGE OF EARTHSCIENCES◆教授(级)33人、副教授(级)35人◆享受国务院政府特殊津贴专家4人◆四川省学术和技术带头人6人◆四川省有突出贡献的优秀专家2人◆四川省学术和技术带头人后备人选6人◆新世纪“百千万人才工程”国家级人选1人◆百名跨世纪优秀科技人才培养计划2人◆教育部跨世纪优秀人才培养计划1人◆全国优秀教师1人◆省级教学名师1人◆国家级特色专业:地质学、资源勘查工程(固体矿产)、地球化学◆省级特色专业:地理信息科学、测绘工程◆省级精品课程:普通地质学、矿产勘查地质学、矿床学、岩石学、铀矿地质◆国土资源部野外科学观测研究基地:四川攀枝花钒钛磁铁矿野外科学观测研究基地、重庆城口巴山锰钡矿野外科学观测研究基地◆国家级大学生校外实践教育基地:理科实践教育基地◆省级本科人才培养基地:资源环境地质学类本科人才培养基地、测绘与地理信息工程本科人才培养基地◆省级教学团队:地球化学◆国家级实验教学示范中心:地质学实验教学示范中心◆省级实验教学示范中心:地矿勘查实验教学示范中心◆国家级卓越工程师教育培养计划试点专业:资源勘查工程(固体矿产)◆省级卓越工程师教育培养计划试点专业:测绘工程、遥感科学与技术◆四川省省属高校科研创新团队:盆岭-盆山构造与油气、构造成矿学理论发展与实践◆国土资源部重点实验室:构造成矿成藏重点实验室、地学空间信息技术重点实验室(培育)本科专业地质学资源勘查工程(固体矿产)地球化学测绘工程地理信息科学遥感科学与技术一级学科硕士学位授权点地质学地理学测绘科学与技术二级学科硕士学位授权点地图学与地理信息系统矿物学、岩石学、矿床学构造地质学第四纪地质学大地测量学与测量工程摄影测量与遥感地图制图学与地理信息工程资源与环境遥感矿产普查与勘探地球化学沉积学(含:古地理学)古生物与地层学专业硕士学位授权点测绘工程地质工程(固体地质勘查)一级学科博士学位授权点地质学地质资源与地质工程(共建)二级学科博士学位授权点矿产普查与勘探矿物学、岩石学、矿床学构造地质学第四纪地质学地球化学资源与环境遥感沉积学(含:古地理学)古生物与地层学博士后流动站地质学地质资源与地质工程(共建)地质学本科一批国家级特色专业招生类别:理工专业剖析:地质学是研究地球的物质组成、内部构造、外部特征、各圈层间的相互作用及时空演化的自然学科。
工程管理专业外文文献翻译(中英文)【精选文档】
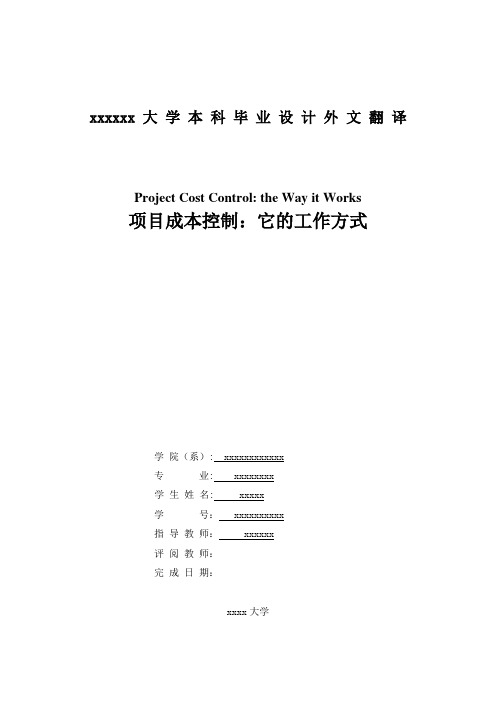
xxxxxx 大学本科毕业设计外文翻译Project Cost Control: the Way it Works项目成本控制:它的工作方式学院(系): xxxxxxxxxxxx专业: xxxxxxxx学生姓名: xxxxx学号: xxxxxxxxxx指导教师: xxxxxx评阅教师:完成日期:xxxx大学项目成本控制:它的工作方式在最近的一次咨询任务中,我们意识到对于整个项目成本控制体系是如何设置和应用的,仍有一些缺乏理解。
所以我们决定描述它是如何工作的.理论上,项目成本控制不是很难跟随。
首先,建立一组参考基线。
然后,随着工作的深入,监控工作,分析研究结果,预测最终结果并比较参考基准。
如果最终的结果不令人满意,那么你要对正在进行的工作进行必要的调整,并在合适的时间间隔重复。
如果最终的结果确实不符合基线计划,你可能不得不改变计划.更有可能的是,会 (或已经) 有范围变更来改变参考基线,这意味着每次出现这种情况你必须改变基线计划。
但在实践中,项目成本控制要困难得多,通过项目数量无法控制成本也证明了这一点。
正如我们将看到的,它还需要大量的工作,我们不妨从一开始启用它。
所以,要跟随项目成本控制在整个项目的生命周期.同时,我们会利用这一机会来指出几个重要文件的适当的地方。
其中包括商业案例,请求(资本)拨款(执行),工作包和工作分解结构,项目章程(或摘要),项目预算或成本计划、挣值和成本基线。
所有这些有助于提高这个组织的有效地控制项目成本的能力。
业务用例和应用程序(执行)的资金重要的是要注意,当负责的管理者对于项目应如何通过项目生命周期展开有很好的理解时,项目成本控制才是最有效的。
这意味着他们在主要阶段的关键决策点之间行使职责。
他们还必须识别项目风险管理的重要性,至少可以确定并计划阻止最明显的潜在风险事件。
在项目的概念阶段•每个项目始于确定的机会或需要的人.通常是有着重要性和影响力的人,如果项目继续,这个人往往成为项目的赞助。
本科毕业设计外文文献翻译
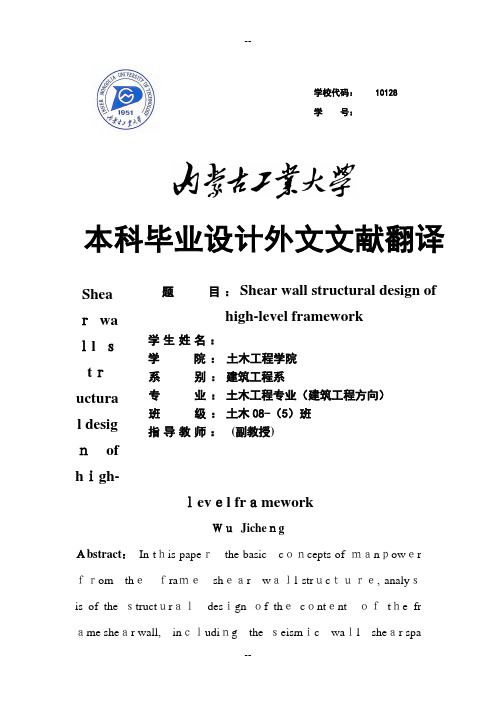
(Shear wall st ructural design ofh igh-lev el fr ameworkWu Jiche ngAbstract : In t his pape r the basic c oncepts of man pow er from th e fra me sh ear w all str uc ture, analy sis of the struct ur al des ign of th e c ont ent of t he fr ame she ar wall, in cludi ng the seism ic wa ll she ar spa本科毕业设计外文文献翻译学校代码: 10128学 号:题 目:Shear wall structural design of high-level framework 学生姓名: 学 院:土木工程学院 系 别:建筑工程系 专 业:土木工程专业(建筑工程方向) 班 级:土木08-(5)班 指导教师: (副教授)nratiodesign, and a concretestructure in themost co mmonly usedframe shear wallstructurethedesign of p oints to note.Keywords: concrete; frameshearwall structure;high-risebuildingsThe wall is amodern high-rise buildings is an impo rtant buildingcontent, the size of theframe shear wall must comply with building regulations. The principle is that the largersizebut the thicknessmust besmaller geometric featuresshouldbe presented to the plate,the force is close to cylindrical.The wall shear wa ll structure is a flatcomponent. Itsexposure to the force along the plane level of therole ofshear and moment, must also take intoaccountthe vertical pressure.Operate under thecombined action ofbending moments and axial force andshear forcebythe cantilever deep beam under the action of the force levelto loo kinto the bottom mounted on the basis of. Shearwall isdividedinto a whole walland theassociated shear wall in theactual project,a wholewallfor exampl e, such as generalhousingconstruction in the gableor fish bone structure filmwalls and small openingswall.Coupled Shear walls are connected bythecoupling beam shear wall.Butbecause thegeneralcoupling beamstiffness is less thanthe wall stiffnessof the limbs,so. Walllimb aloneis obvious.The central beam of theinflection pointtopay attentionto thewall pressure than the limits of the limb axis. Will forma shortwide beams,widecolumn wall limbshear wall openings toolarge component atbothen ds with just the domain of variable cross-section ro din the internalforcesunder theactionof many Walllimb inflection point Therefore, the calcula tions and construction shouldAccordingtoapproximate the framestructure to consider.The designof shear walls shouldbe based on the characteristics of avariety ofwall itself,and differentmechanical ch aracteristicsand requirements,wall oftheinternalforcedistribution and failuremodes of specific and comprehensive consideration of the design reinforcement and structural measures. Frame shear wall structure design is to consider the structure of the overall analysis for both directionsofthehorizontal and verticaleffects. Obtain theinternal force is required in accordancewiththe bias or partial pull normal section forcecalculation.The wall structure oftheframe shear wall structural design of the content frame high-rise buildings, in the actual projectintheuse of themost seismic walls have sufficient quantitiesto meet thelimitsof the layer displacement, the location isrelatively flexible. Seismic wall for continuous layout,full-length through.Should bedesigned to avoid the wall mutations in limb length and alignment is notupand down the hole. The sametime.The inside of the hole marginscolumnshould not belessthan300mm inordertoguaranteethelengthof the column as the edgeof the component and constraint edgecomponents.Thebi-direc tional lateral force resisting structural form of vertical andhorizontalwallconnected.Each other as the affinityof the shear wall. For one, two seismic frame she ar walls,even beam highratio should notgreaterthan 5 and a height of not less than400mm.Midline columnand beams,wall midline shouldnotbe greater tha nthe columnwidthof1/4,in order toreduce thetorsional effect of the seismicaction onthecolumn.Otherwisecan be taken tostrengthen thestirrupratio inthe column tomake up.If theshear wall shearspan thanthe big two. Eventhe beamcro ss-height ratiogreaterthan 2.5, then the design pressure of thecut shouldnotmakeabig 0.2. However, if the shearwallshear spanratioof less than two couplingbeams span of less than 2.5, then the shear compres sion ratiois notgreater than 0.15. Theother hand,the bottom ofthe frame shear wallstructure to enhance thedesign should notbe less than200mmand notlessthanstorey 1/16,otherpartsshouldnot be less than 160mm and not less thanstorey 1/20. Aroundthe wall of the frame shear wall structure shouldbe set to the beam or dark beamand the side columntoform a border. Horizontal distributionofshear walls can from the shear effect,this design when building higher longeror framestructure reinforcement should be appropriatelyincreased, especially in the sensitiveparts of the beam position or temperature, stiffnesschange is bestappropriately increased, thenconsideration shouldbe givento the wallverticalreinforcement,because it is mainly from the bending effect, andtake in some multi-storeyshearwall structurereinforcedreinforcement rate -likelessconstrained edgeofthecomponent or components reinforcement of theedge component.References: [1 sad Hayashi,He Yaming. On the shortshear wall high-rise buildingdesign [J].Keyuan, 2008, (O2).高层框架剪力墙结构设计吴继成摘要: 本文从框架剪力墙结构设计的基本概念人手, 分析了框架剪力墙的构造设计内容, 包括抗震墙、剪跨比等的设计, 并出混凝土结构中最常用的框架剪力墙结构设计的注意要点。
- 1、下载文档前请自行甄别文档内容的完整性,平台不提供额外的编辑、内容补充、找答案等附加服务。
- 2、"仅部分预览"的文档,不可在线预览部分如存在完整性等问题,可反馈申请退款(可完整预览的文档不适用该条件!)。
- 3、如文档侵犯您的权益,请联系客服反馈,我们会尽快为您处理(人工客服工作时间:9:00-18:30)。
由于异常高压导致低温梯度陡然增加,地层超压的分布可以根据上述参数的变化估计。从地震波速度可以推论地层压力的分布与测井数据相一致(图2)。超压在平面中的分布不仅受沉降中心影响,还直接受底辟分布及强度影响。在盆地边界,超压的顶部深度大于盆地中心。甚至没有任何异常超压。向盆地的中心,顶部的超压逐渐变浅,高底辟构造的顶部大约1480米(the wellLD14-1-1)。
成都理工大学
学生毕业设计(论文)外文译文
学生姓名:雷和金
学号:200904020223
专业名称:资源勘查工程
译文标题(中英文):莺歌海盆地超压流体流动的构造效应(Structural Effects of Overpressure FluidActivities in Yinggehai Basin)
关键词:超压莺歌海盆地构造效应
在沉积盆地中超压和流体在油气运移和聚集中所起的作用的研究(li,2000,1995)。地质学家最近意识到在沉积盆地中研究超压流体的活动是认识如超压地层、阶段性排出、通道系统、圈闭特征、和动力演化科学依据(Li,2000;Tang and Wang,1999;Law and Spencer,1998)。在超压流体和它的作用上有了越来越多的研究。
译文出处:Mining Science and Technology 20 (2010) 0591–0599
指导教师审阅签名:
摘要:
这篇文章讨论了莺歌海盆地断层的特征和分布,揭示了超压流体排出的构造效应。快速的沉降和富泥的海相地层控制了超压系统和大量超压流体的形成。超压流体由于一些断层的作用被快速的排出,在盆地中形成一系列的底辟构造,从而导致底辟的裂缝、断层和褶皱的形成。这些裂缝和断层为烃类的纵向流动提供了运移通道,使气体在该过程中向上运移至砂岩储层。因此,油气藏通常位于底辟构造的上部分。
图5:莺歌海盆地泥底辟构造
(a)拱形构造(b)漏斗构造
莺歌海盆地的油气勘探表明,有许多圈闭背斜中的裂缝位于底辟的顶部,所有的裂缝和在超压系统中成熟的超压流体活动相关。(Yin et a1.,2002b).一些刺穿的超压超压系统向上延伸然后在正常压力区域中消失或延伸到海底。由此产生的裂缝是超压流体垂向运移的主要通道。
莺歌海盆地的典型特征是广泛的超压流体活动。在运移的过程中超压流体获得丰富的能量,不仅提供了使油气运移和聚集的排驱力,同时也使之与围岩进行反应和能量交换。之后,盆地的构造和成藏特征可能发生改变(Yinet a1.,2002a),因此,莺歌海盆地是一个研究超压流体活动理想的自然实验室。
超压流体排出超压封存箱控制着流体压力场的分布模式。由于浅层和深部地层的温度差异,快速流动可能会导致对流传导(finkbeinereta1.,2001),运移通道的大量形成。在这种情况下,热超压流体迅速上升到温度和压力异常高的浅层。
这篇文章分析了莺歌海盆地的构造特征和超压流体活动的规律。讨论了在沉积盆地的超压流体活动的构造效应和提供了构造演化的研究证据。
莺歌海盆地位于中国南海西北部,北西走向,与古红河断裂带直接重叠,主要的边界断裂是一个断层,是红河断裂带的一个分支,作为琼东南盆地与莺歌海盆地的分界,莺歌海盆地的最南端连接着南北走向的粤东断层,沿着归仁和皖南-北盆地发育,莺歌海盆地由于位于莫霍面20-22km大陆地壳延伸而形成。该盆地具有独特的沉积、构造和演化模式,揭示了莺歌海盆地超压流体流动的丰富的烃类构造效应。
莺歌海盆地的沉降和填充率非常迅速。新近纪以来,多达10公里的沉积物被沉积。在特殊时期,之后的5.2百万年,盆地沉降的越来越深,这些沉积物从上新世到第四纪主要由海相层序组成,厚度可达至4000m。由地震和钻井数据显示其沉降速率超过700m/Ma(Xie et a1.,1999).
莺歌海盆地地温高的特征在1999年被Dong发现。最高的地温梯度位于中央的泥浆流体底辟带,高达4.36-4.98℃/100米,而盆地两侧斜坡地温梯度较低(Wang and Hu,1999)
图一:莺歌海盆地简化构造图
1:大陆板块2:古生代造山带3:中生代造山带4:洋壳5:第三纪盆地
6古地中海缝合线7:俯冲和弧陆碰撞带。
莺歌海盆地的沉降和填充率非常迅速。新近纪以来,多达10公里的沉积物被沉积。在特殊时期,之后的5.2百万年,盆地沉降的越来越深,这些沉积物从上新世到第四纪主要由海相层序组成,厚度可达至4000m。由地震和钻井数据显示其沉降速率超过700m/Ma(Xie et a1.,1999).
超压特征
基于莺歌海盆地超压流体运移通道的不同,超压流体的对流可分为两种类型,横向对流和垂直对流(xieeta1.,1999年,1997年)。
横向对流超压流体运移主要沿着横向的通道网络。主要的通道是渗透性好的砂岩体,不整合,和平行断层系统。这种类型的压力分布通常具有明显的热异常且镜质体反射率与那些正常的热传导不同。垂直对流超压流体运移沿垂直的密集裂缝和断层。
这篇文章分析了莺歌海盆地的构造特征和超压流体活动的规律。讨论了在沉积盆地的超压流体活动的构造效应和提供了构造演化的研究证据。
莺歌海盆地位于中国南海西北部,北西走向,与古红河断裂带直接重叠,主要的边界断裂是一个断层,是红河断裂带的一个分支,作为琼东南盆地与莺歌海盆地的分界,莺歌海盆地的最南端连接着南北走向的粤东断层,沿着归仁和皖南-北盆地发育,莺歌海盆地由于位于莫霍面20-22km大陆地壳延伸而形成。该盆地具有独特的沉积、构造和演化模式,揭示了莺歌海盆地超压流体流动的丰富的烃类构造效应。
测井数据显示不受底辟构造活动影响的超压顶部深度大约是3000m。在盆地的西北和边界,超压顶部的深度增加而地层的压力系数减少。底辟是一个局部异常高压区,其超压顶部浅层和较新的地层(莺歌海盆地上新世以上地层)的特征主要受深部高温和超压热流体沿断裂带从浅到深的测井数据引导。可以观察到地层低于3900米压力系数稳定在2~2.2。压力系数的垂直分布从莺歌海黄流组到基底逐渐延伸,其眉山组的压力系数似乎最大,从三亚组轻微降低(Zhang et a1.1996)。
莺歌海盆地的底辟构造是由沿断裂带超压流体活动控制,而断层和塑性岩石的侵入,底辟构造又称为泥浆流体底辟构造当超压流体被向上排出导致地层顶部变形从而显示为穹隆背斜(图5a)但是如果断层促使底辟作用延伸到海底,从而诱发塌陷构造,可能会形成漏斗构造。图(5b)
图4:底辟构造在莺歌海盆地的分布
1、底辟构造2、盆地边界3、断层4、底辟构造延伸方向
基于莺歌海盆地超压流体运移通道的不同,超压流体的对流可分为两种类型,横向对流和垂直对流(xieeta1.,1999年,1997年)。
横向对流超压流体运移主要沿着横向的通道网络。主要的通道是渗透性好的砂岩体,不整合,和平行断层系统。这种类型的压力分布通常具有明显的热异常且镜质体反射率与那些正常的热传导不同。垂直对流超压流体运移沿垂直的密集裂缝和断层。
地层压力系数,即地层压力与正常静水压力的比值,从边界到中心逐渐增加其最大值为2.2。在Linggao隆起,顶部的超压与梅山地层的底部接近,其表面低于3400m,最大压力系数小于1.6。压力系数在1号断层的下降盘在1.1-1.7的过渡。
因此,一般压力系数分布趋势从东北和西北斜坡带到盆地中心逐渐增加。特别是在底辟中央。底辟构造的分布和活动强度控制着地层超压的分布。(L4.GeopressureandOil/Gas Migration in Yinggehai—Qiongdongnan Basin).
图2:莺歌海盆地超压的顶部等深线图
测井数据显示不受底辟构造活动影响的超压顶部深度大约是3000m(图3)
图3:莺歌海盆地各井压力分布
在沉积盆地中超压和流体在油气运移和聚集中所起的作用的研究(li,2000,1995)。地质学家最近意识到在沉积盆地中研究超压流体的活动是认识如超压地层、阶段性排出、通道系统、圈闭特征、和动力演化科学依据(Li,2000;Tang and Wang,1999;Law and Spencer,1998)。在超压流体和它的作用上有了越来越多的研究。
由于其快速沉积,形成以海相页岩为主的盆地充填序列,其中高地热,大型高压封存箱形成大型超压热流体。这种大型超压热流体自上新世以来被排出超压封存箱可能是由区域应力状态的变化控制产生导致红河走滑断层。雁列式和张扭性断裂控制底辟构造的分布和导致大规模的超压热流体排出。然后,通过横向和纵向的超压热流体流出形成独特的局部应力场。
由于大型的超压地层在盆地深部能量巨大。底辟作用,超压流体活动与天然气富集均发生在中深层的巨大异常高压系统下。
超压流体系统易产生底辟作用一旦发生一些构造运动。盆地充填序列由
泥岩为主,有利于形成异常高压地层和压力的控制。莺歌海盆地中央底辟带的沉降速率最高,以至于充填着丰富的泥岩。自晚中新世以后形成的一系列底辟构造可分为5行,彼此大致呈雁形排列。(图4)
莺歌海盆地的典型特征是广泛的超压流体活动。在运移的过程中超压流体获得丰富的能量,不仅提供了使油气运移和聚集的排驱力,同时也使之与围岩进行反应和能量交换。之后,盆地的构造和成藏特征可能发生改变(Yinet a1.,2002a),因此,莺歌海盆地是一个研究超压流体活动理想的自然实验室。
超压流体排出超压封存箱控制着流体压力场的分布模式。由于浅层和深部地层的温度差异,快速流动可能会导致对流传导(finkbeinereta1.,2001),运移通道的大量形成。在这种情况下,热超压流体迅速上升到温度和压力异常高的浅层。
当超压流体被排出,高的地热的释放,高的温度和能量形成了底辟构造和底辟构造顶部的致密裂缝和断层。其形成过程,在底辟构造顶部张开和关闭的断裂通常显示超压热流体和油(气)的运移。作为垂直通道这些断裂拥有以下特点:(1)长期或多阶段活动或继承性断裂:(2)断裂导致的超压烃源;(3)延伸到石油生成的断裂;(4)与储集层岩石相连接。