Nitrogen and potassium dynamics in fertigation systems
吸附在水铁矿,赤铁矿和针铁矿表面的磷酸盐有效性研究

220
Guzman et al. Fe (Fet) by dissolving the sample in 11 M HC1. Dissolved Fe was analyzed by atomic absorption spectrophotometry. The products were examined by X-ray powder diffraction as described by Torrent et al. (1990). Specific surface area was determined by N 2 adsorption (BET method) using a Quantasorb Jr. apparatus. The N2-adsorption data were also used to measure microporosity by the t-plot method (Gregg and Sing, 1982). Transmission electron micrographs (TEM) were obtained with a Jeol JEM-200 CX instrument, after diluting a portion of the stock suspension in acetone, and evaporating a droplet of the resulting suspension on a C-coated Cu grid. Phosphate adsorption curves Phosphate adsorption curves were obtained by adding different amounts of KH2PO 4 to 10-12 suspensions of 10 mg (for F) or 60 mg (for H1, H2, G1, and G2) of Fe (hydr)oxides in the nutrient solution described later. The final volume was 30 mL and the suspensions were shaken at 2 Hz and 298 K for 1 day, and, then, 15 min daily for 11 days. After 1, 3, 6, and 12 days, samples of the suspensions were centrifuged, and P in the supernatant was analyzed (Murphy and Riley, 1962). Final equilibrium concentrations ranged between 0.01 and 10mg P L -1. To describe adsorption, the following modified Freundlich equation (Barrow and Shaw, 1975) was used: X = Acbt d (1)
氮钾钙配施对生菜生长的影响
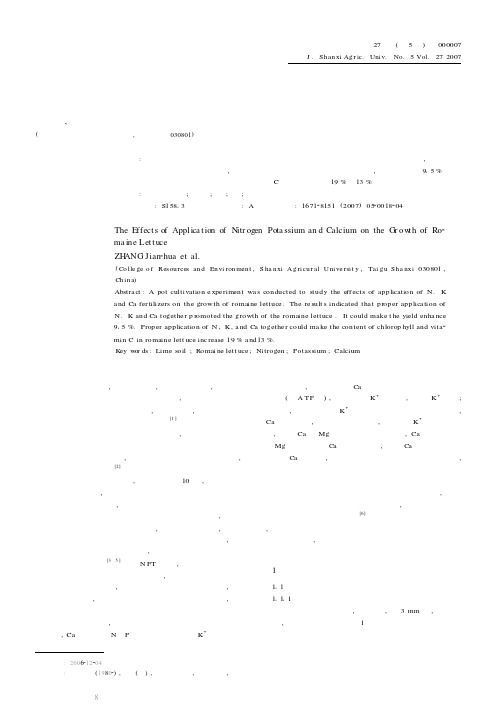
山西农业大学学报第27卷(第5期) 000007J.Shanxi Agr ic.Univ.No.5Vol.272007收稿日期622作者简介张建华(82),女(汉),山西左云人,在读硕士,主要从事植物营养与施肥方面的研究。
氮钾钙配施对生菜生长的影响张建华,卜玉山(山西农业大学资源与环境学院,山西太谷030801)摘 要:采用盆栽试验研究了石灰性土壤施用氮、钾、钙肥对生菜生长的影响。
结果表明,氮钾钙配施对生菜生长有显著的影响,适宜的氮钾钙配比可以促进生菜的生长,使产量提高915%。
适宜的氮钾钙配施可以使生菜叶绿素和维生素C 含量分别提高19%和13%。
关键词:石灰性土壤;生菜;氮;钾;钙中图分类号:S15813 文献标识码:A 文章编号:167128151(2007)0520018204The Effect s of Appl ica t ion of N itr ogen Pota ssium an d Calcium on the G r ow t h of Ro 2ma ine Let t uce ZH ANG Jian 2hua et al.(Colle ge of Resources and Envi ronment ,S ha nxi A gricur al U nive rsit y ,Tai gu Sha nxi 030801,China)Abstra ct :A pot cultivation e xpe riment wa s conducted to study the eff ects of application of N.K a nd Ca fer tilizers on the growth of romaine lettuce.The re sult s indicated tha t proper applica tion of N.K and Ca togethe r p romoted the growth of the romaine lettuce .It could make t he yield enha nce 915%.Proper application of N ,K,a nd Ca togethe r could ma ke the content of chlorop hyll and vita 2min C in romaine lett uce inc rease 19%a nd13%.K ey w o r ds :Lime soil ;Romai ne lett uce ;Nitrogen ;Potassium ;Calcium生菜即叶用莴苣,其质地脆嫩,口感鲜嫩清香,具有清肝利胆和养胃的功效。
鲁米诺_铁氰化钾化学发光体系对盐酸环丙沙星的测定

第29卷第3期2010年3月 分析测试学报FENXI CESH I X UEBAO (J ournal of Ins tru m en talAnal ys i s)V ol 129No 13313~315收稿日期:2009-12-16;修回日期:2010-02-22基金项目:河南省教育厅自然科学研究资助项目(2008B610001,2009A610003)第一作者:周 华(1986-),女,河南中牟人,硕士研究生通讯作者:周艳梅,Te:l 0378-*******,E-m ai:lz houy m@henu 1edu 1cn鲁米诺-铁氰化钾化学发光体系对盐酸环丙沙星的测定周 华1,魏金凤2,周艳梅1,王亚萍1,朱天伟1(1.河南大学 化学化工学院 环境与分析科学研究所,河南 开封 475004;2.河南大学 民生学院,河南 开封 475004)摘 要:环丙沙星对N a OH 介质中的鲁米诺-铁氰化钾的化学发光具有较强的增敏作用,据此建立了一种新的测定痕量环丙沙星药物的流动注射化学发光法。
讨论了介质浓度、发光试剂浓度等因素的影响,在最佳实验条件下,环丙沙星的质量浓度在210@10-5~112@10-4g #L -1范围内与发光强度呈良好线性关系,检出限为112@10-6g #L -1,11次平行测定410@10-5g #L -1环丙沙星的相对标准偏差为312%。
该方法已用于环丙沙星片剂的测定,所得结果与标示值相符。
关键词:化学发光;鲁米诺-铁氰化钾;环丙沙星中图分类号:O 65713;R 917 文献标识码:A 文章编号:1004-4957(2010)03-0313-03do:i 1013969/j 1issn 11004-4957120101031022D eter m i nati on of C i pro fl oxaci n w ith Lu m i no l-Potassi u m Ferricyani deChe m il u m i nescence Syste mZ HOU H ua 1,W E I Jin -feng 2,Z HOU Yan -m ei 1,WANG Y a -ping 1,Z HU T ian -we i1(1.Instit u te o f Env i ron m enta l and A nalytical Sciences ,College o f Che m i stry and Che m i ca l Eng i neering ,H enan U niversity ,K a ifeng 475004,Ch i na ;2.Co llege ofM i nsheng ,H enan U ni v ers it y ,K a ifeng 475004,Chi na)Abstr ac:t B ased on the fact that the che m ilu m i n escence(CL)reaction o f lum i n ol and potassi u m fer -ricyan i d e in a l k ali n e m edi u m cou l d be sensitized by cipro fl o xaci n sign ificantly ,and the che m il u m -inescence i n tensity w as d irectl y proportional to the concentration of cipro fl o xaci n ,a ne w analytica l m ethod w as deve l o ped for the deter m i n ati o n of cipr o floxaci n w ith fl o w i n jecti o n che m il u m inescence .The effect of type o fm edi u m and concentrati o n o f reagents on CL intensity w as i n vesti g ated .Under the opti m u m experi m enta l cond ition,the CL intensity w as proporti o na l to c i p rofl o xac i n concentrati o n over the range of 210@10-5-112@10-4g #L-1w it h a detection li m it of 112@10-6g #L -1.There lative standard dev i a ti o ns(RSDs)of 410@10-5g #L-1c i p rofloxacin w ith 11consecutive m easure -m ents w as found to be 312%.The m ethod w as app li e d in the deter m inati o n o f c i p rofloxacin drug tab -lets ,and the resu lts obta i n ed w ere i n good agree m entw ith that o f the labelled values .Key wor ds :che m il u m i n escence ;lum i n ol-potassi u m ferricyan i d e ;c i p rofloxacin环丙沙星(CF)的化学名为1-环丙-6-氟-104-二氢-4-氧-7[1-哌嗪基]-3-喹啉羧酸,是第三代喹诺酮类药物,对需氧革兰阴性杆菌及绝大多数菌株有较好的抗菌活性[1],在临床上应用广泛。
氮掺杂石墨化炭包覆的铁基催化剂的制备及其费托合成反应性能

ABSTRACT
Fischer‐Tropsch synthesis (FTS) has the potential to be a powerful strategy for producing liquid fuels from syngas if highly selective catalysts can be developed. Herein, a series of iron nanoparticle catalysts encapsulated by nitrogen‐doped graphitic carbon were prepared by a one‐step pyrolysis of a ferric L‐glutamic acid complex. The FeC‐800 catalyst pyrolyzed at 800 °C showed excellent cat‐ alytic activity (239.4 μmolCO gFe–1 s–1), high C5–C11 selectivity (49%), and good stability in FTS. The high dispersion of ferric species combined with a well‐encapsulated structure can effectively inhibit the migration of iron nanoparticles during the reaction process, which is beneficial for high activity and good stability. The nitrogen‐doped graphitic carbon shell can act as an electron donor to the iron particles, thus promoting CO activation and expediting the formation of Fe5C2, which is the key factor for obtaining high C5–C11 selectivity.
无机物英文命名法则知识讲解

无机物英文命名法则Unit 3 The Nomenclature of Inorganic Compounds一、元素与单质的命名“元素”和“单质”的英文意思都是“element”,有时为了区别,在强调“单质”时可用“free element”。
因此,单质的英文名称与元素的英文名称是一样的。
下面给出的既是元素的名称,同时又是单质的名称。
IAH Hydrogen [ˈhaɪdrədʒən] 氢Li Lithium [ˈlɪθiəm] 锂Na Sodium [ˈsodiəm] 钠K Potassium [pə'tæsɪəm] 钾Rb Rubidium [ruˈbɪdiəm] 铷Cs Cesium ['si:zɪəm] 铯Fr Francium [ˈfrænsiəm] 钫IIABe Beryllium [bəˈrɪliəm] 铍Mg Magnesium [mægˈni:ziəm] 镁Ca Calcium [ˈkælsiəm] 钙Sr Strontium [ˈstrɑntiəm] 锶Ba Barium [ˈbeəriəm] 钡Ra Radium [ˈrediəm] 镭IIIAB Boron ['bɔ:rɑ:n] 硼Al Aluminium [ˌæljəˈmɪniəm] 铝Ga Gallium [ˈɡæliəm] 稼In Indium ['ɪndɪəm] 铟Tl Thallium [ˈθæliəm] 铊IV AC Carbon ['kɑ:bən] 碳Si Silicon [ˈsɪlɪkən] 硅Ge Germanium [dʒɚˈmeniəm] 锗Sn Tin [tɪn] 锡Pb Lead [lid] 铅V AN Nitrogen [ˈnaɪtrədʒən] 氮P Phosphorus [ˈfɒsfərəs] 磷As Arsenic [ˈɑ:snɪk] 砷Sb Antimony [ˈæntɪməni] 锑Bi Bismuth [ˈbɪzməθ]铋VIAO Oxygen [ˈɒksɪdʒən] 氧S Sulfur ['sʌlfə] 硫Se Selenium [sɪˈliniəm] 硒Te Tellurium [teˈljʊəriəm] 碲Po Polonium [pəˈləʊniəm] 钋VIIAF Fluorine [ˈflɔ:ri:n] 氟Cl Chlorine [ˈklɔ:ri:n] 氯Br Bromine [ˈbrəʊmi:n] 溴I Iodine [ˈaɪədi:n] 碘At Astatine [ˈæstəti:n] 砹He Helium [ˈhi:liəm] 氦Ne Neon [ˈni:ɑ:n] 氖Ar Argon [ˈɑ:rgɑ:n] 氩Kr Krypton [ˈkrɪptɑ:n] 氪Xe Xenon [ˈzenɑ:n] 氙Rn Radon [ˈreɪdɑ:n] 氡常见过渡金属Fe iron [ˈaɪərn] 铁Cu copper [ˈkɒpə] 铜Hg mercury [ˈmɜ:kjəri] 汞Au gold [gəʊld] 金Mn manganese [ˈmæŋgəni:z] 锰Zn zinc [zɪŋk] 锌Ag silver [ˈsɪlvə] 银单质名称H atomic hydrogen [əˈtɔmik ˈhaidrədʒən] monohydrogen [mɒnəʊ'haɪdrədʒən]O2 oxygen [ˈɒksɪdʒən] dioxygen [daɪ'ɒksɪdʒən]O3 ozone [ˈəʊzəʊn] trioxygenP4 phosphorus tetraphosphorus ['tetrəˈfɒsfərəs]二、阳离子1.单价阳离子单价阳离子直呼其名,即读其元素名称。
紫色土中氮肥、磷肥、钾肥配施对白芷产量及有效成分的影响
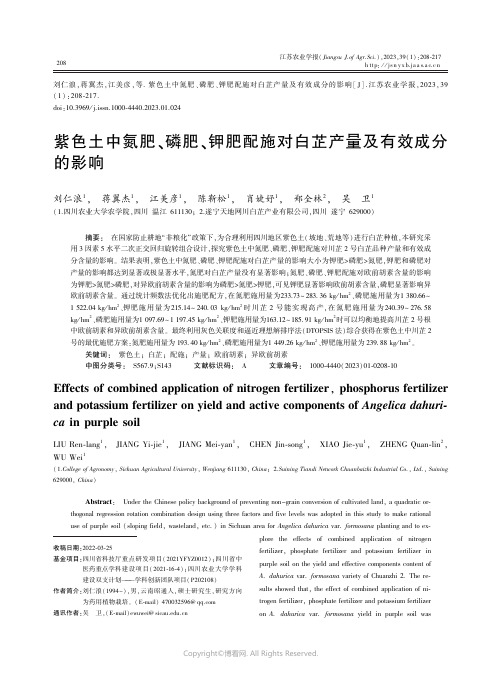
中图分类号: S567.9ꎻS143 文献标识码: A 文章编号: 1000 ̄4440(2023)01 ̄0208 ̄10
Effects of combined application of nitrogen fertilizerꎬ phosphorus fertilizer
and potassium fertilizer on yield and active components of Angelica dahuri ̄
摘要: 在国家防止耕地“非粮化”政策下ꎬ为合理利用四川地区紫色土( 坡地、荒地等) 进行白芷种植ꎬ本研究采
用 3 因素 5 水平二次正交回归旋转组合设计ꎬ探究紫色土中氮肥、磷肥、钾肥配施对川芷 2 号白芷品种产量和有效成
分含量的影响ꎮ 结果表明ꎬ紫色土中氮肥、磷肥、钾肥配施对白芷产量的影响大小为钾肥>磷肥>氮肥ꎬ钾肥和磷肥对
use of purple soil (sloping fieldꎬ wastelandꎬ etc ) in Sichuan area for Angelica dahurica var formosana planting and to ex ̄
plore the effects of combined application of nitrogen
methodꎬ and the application levels of nitrogenous fertilizerꎬ phosphate fertilizer and potassium fertilizer were 193 40 kg / hm2 ꎬ
1 449 26 kg / hm2 and 239 88 kg / hm2 ꎬ respectively
作者简介:刘仁浪(1994-) ꎬ男ꎬ云南昭通人ꎬ硕士研究生ꎬ研究方向
糖厂滤泥发酵制成生物有机肥
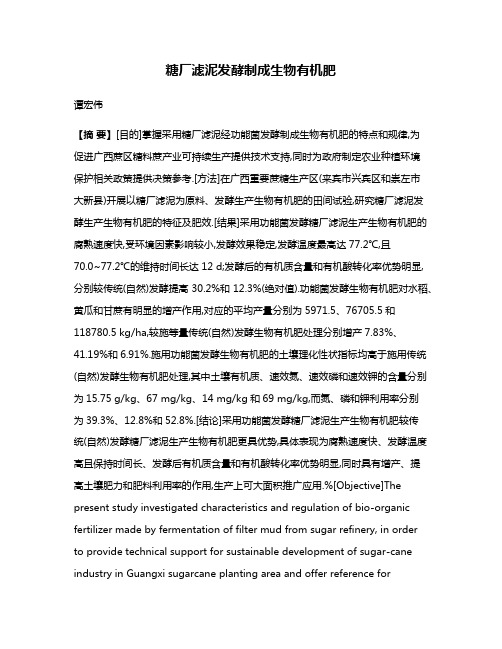
糖厂滤泥发酵制成生物有机肥谭宏伟【摘要】[目的]掌握采用糖厂滤泥经功能菌发酵制成生物有机肥的特点和规律,为促进广西蔗区糖料蔗产业可持续生产提供技术支持,同时为政府制定农业种植环境保护相关政策提供决策参考.[方法]在广西重要蔗糖生产区(来宾市兴宾区和崇左市大新县)开展以糖厂滤泥为原料、发酵生产生物有机肥的田间试验,研究糖厂滤泥发酵生产生物有机肥的特征及肥效.[结果]采用功能菌发酵糖厂滤泥生产生物有机肥的腐熟速度快,受环境因素影响较小,发酵效果稳定,发酵温度最高达77.2℃,且70.0~77.2℃的维持时间长达12 d;发酵后的有机质含量和有机酸转化率优势明显,分别较传统(自然)发酵提高30.2%和12.3%(绝对值).功能菌发酵生物有机肥对水稻、黄瓜和甘蔗有明显的增产作用,对应的平均产量分别为5971.5、76705.5和118780.5 kg/ha,较施等量传统(自然)发酵生物有机肥处理分别增产7.83%、41.19%和6.91%.施用功能菌发酵生物有机肥的土壤理化性状指标均高于施用传统(自然)发酵生物有机肥处理,其中土壤有机质、速效氮、速效磷和速效钾的含量分别为15.75 g/kg、67 mg/kg、14 mg/kg和69 mg/kg,而氮、磷和钾利用率分别为39.3%、12.8%和52.8%.[结论]采用功能菌发酵糖厂滤泥生产生物有机肥较传统(自然)发酵糖厂滤泥生产生物有机肥更具优势,具体表现为腐熟速度快、发酵温度高且保持时间长、发酵后有机质含量和有机酸转化率优势明显,同时具有增产、提高土壤肥力和肥料利用率的作用,生产上可大面积推广应用.%[Objective]The present study investigated characteristics and regulation of bio-organic fertilizer made by fermentation of filter mud from sugar refinery, in orderto provide technical support for sustainable development of sugar-cane industry in Guangxi sugarcane planting area and offer reference forgovernment to formulate agricultural planting and environmental protection policies. [Method]Field trial was conducted in important sugarcane planting area in Guangxi (Xingbin district in Laibin city, Daxin county in Chongzuo city) . Filter mud from sugar refinery was taken as raw materials and fermented into bio-organic fertilizer. Characteristics and fertilizer efficiency of the bio-organic fertilizer were studied.[Result]Bio-organic fertilizer fermented via functional bacteria rotted rapidly, hardly affected by environmental factors. Fermentation effects were stable, fermentation temperature could reach 77.2 ℃, and the temperature maintaining at 70.0-77.2 ℃ for 12 days. Organic matter content and organic acid conversion rate after fermented were 30.2% and 12.3%(abso-lute value) higher than those in traditional(natural) mode. Bio-organic fertilizer fermented via functional bacteria could im-prove yield of rice, cucumber and sugarcane greatly. Average yield of rice was 5971.5 kg/ha, an increase of 7.83% com-pared with the rice applied with bio-organic fertilizer fermented in traditional(natural) mode; average yield of cucumber was 76705.5 kg/ha, an increase of 41.18%, average yield of sugarcane was 118780.5 kg/ha, an increase of 6.91%.After application of bio-organic fertilizer fermented via functional bacteria, physical and chemical properties of soil were superior than those applied with bio-organic fertilizer fermented in traditional(natural) mode. In the soil, organic matter content was 15.75 g/kg, available nitrogen content 67 mg/kg, available phosphorus content 14 mg/kg, available potassium content 69 mg/kg. Utilization efficiency of nitrogen, phosphorus and potassium were 39.3% ,12.8% and 52.8% , respectively.[Conclusion]Bio-organic fertilizer fermented via functional bacteria using filter mud from sugar refinery is better thanfer-mentation in traditional(natural) mode. The advantages include rapid rotting speed, high fermentation temperature and long duration, superior organic matter content and organic acid conversion rate after fermentation. Meanwhile, it can increase the yield, soil fertility and fertilizer utilization efficiency. Therefore, it can be promoted for large scale application.【期刊名称】《南方农业学报》【年(卷),期】2017(048)003【总页数】5页(P428-432)【关键词】糖厂滤泥;发酵;生物有机肥;肥效;特征【作者】谭宏伟【作者单位】广西农业科学院甘蔗研究所, 南宁 530007【正文语种】中文【中图分类】S141.5【研究意义】广西是我国最大的糖业基地,蔗糖产量约占全国的70%(李杨瑞等,2014;覃泽林等,2015;谭芳等,2016)。
含氮、磷、钾三种肥效元素的矿物肥料...(HS 310520)2017 哥伦比亚(75个)进口商排名(按进口额排名)
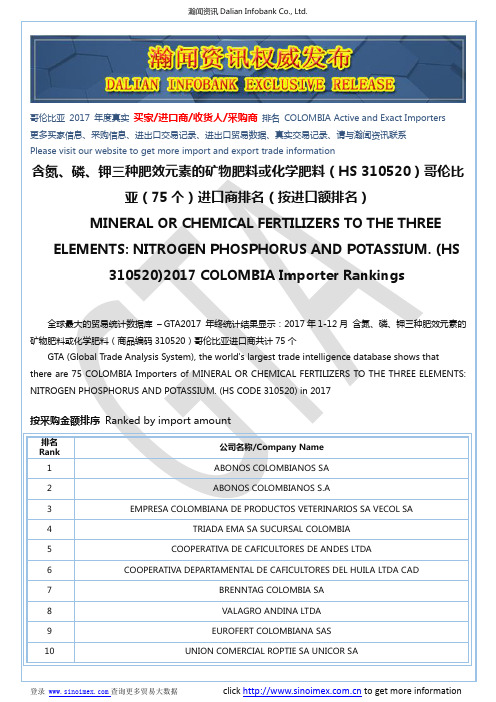
含氮、磷、钾三种肥效元素的矿物肥料或化学肥料(HS 310520)哥伦比 亚(75 个)进口商排名(按进口额排名) MINERAL OR CHEMICAL FERTILIZERS TO THE THREE ELEMENTS: NITROGEN PHOSPHORUS AND POTASSIUM. (HS 310520)2017 COLOMBIA Importer Rankings
按采购金额排序 Ranked by import amount
排名 Rank 1 2 3 4 5 6 7 8 9 10 公司名称/Company Name ABONOS COLOMBIANOS SA ABONOS COLOMBIANOS S.A EMPRESA COLOMBIANA DE PRODUCTOS VETERINARIOS SA VECOL SA TRIADA EMA SA SUCURSAL COLOMBIA COOPERATIVA DE CAFICULTORES DE ANDES LTDA COOPERATIVA DEPARTAMENTAL DE CAFICULTORES DEL HUILA LTDA CAD BRENNTAG COLOMBIA SA VALAGRO ANDINA LTDA EUROFERT COLOMBIANA SAS UNION COMERCIAL ROPTIE SA UNICOR SA
全球最大的贸易统计数据库 – GTA2017 年终统计结果显示:2017 年 1-12 月 含氮、磷、钾三种肥效元素的 矿物肥料或化学肥料(商品编码 310520)哥伦比亚进口商共计 75 个 GTA (Global Trade Analysis System), the world's largest trade intelligence database shows that there are 75 COLOMBIA Importers of MINERAL OR CHEMICAL FERTILIZERS TO THE THREE ELEMENTS: NITROGEN PHOSPHORUS AND POTASSIUM. (HS CODE 310520) in 2017
- 1、下载文档前请自行甄别文档内容的完整性,平台不提供额外的编辑、内容补充、找答案等附加服务。
- 2、"仅部分预览"的文档,不可在线预览部分如存在完整性等问题,可反馈申请退款(可完整预览的文档不适用该条件!)。
- 3、如文档侵犯您的权益,请联系客服反馈,我们会尽快为您处理(人工客服工作时间:9:00-18:30)。
Symposium no. 14Paper no. 1045Presentation: poster Nitrogen and potassium dynamics infertigation systemsSINGH A.K., CHAKRABORTY D., MISHRA P.and SINGH D.K.Water Technology Centre, Indian Agricultural Research Institute, New Delhi-110012, IndiaAbstractWater and nutrients are the two key inputs in agriculture but their use efficiency is low and their injudicious use leads to environmental degradation. Among the various techniques of water application, drip or trickle irrigation is the most efficient method of delivering water to the root zone. Applying fertilizers through irrigation water, particularly through the drip system, termed as fertigation, also provides the most effective way of supplying nutrients to the plant roots. In fact, the supply can be tailored to meet the precise requirement of the plant depending on its growth stage. A considerable amount of work has been done on drip irrigation and also on fertigation, but studies on the dynamics of nutrients in soil after their exit from the emitter are very few. In this paper, results of two separate experiments conducted to study the fate of nitrogen and potassium in soil applied through fertigation have been presented.In both the experiments, water-soluble fertilizer was applied through a drip system (T1). In the second treatment (T2), the fertilizer was applied on soil but water was applied through drip system while in the third treatment (T3) fertilizer was applied on the soil with conventional irrigation method (check basin/furrow). In the experiment in which nitrogen dynamics were studied, the crop was broccoli while potassium dynamics were studied in radish.In the experiment on nitrogen dynamics, it was observed that in the fertigation treatments, ammonium form of nitrogen dominated in the upper soil layers. Almost all the nitrogen applied remained confined to the root zone. In the conventional method of irrigation, the nitrate-nitrogen dominated and a significant amount was leached out. Leaching losses were also observed when the fertilizer was applied on soil and water through a drip system. In the second experiment, in which potassium dynamics were studied, it was observed that in fertigation treatments, potassium was confined to the root zone of the radish crop, while it moved in significant quantities beyond the root zone in the conventional method (furrow irrigation). Movement beyond the root zone was also observed in the soil-based fertilizer application with water through a drip system but to a lesser degree.Keywords:fertigation, nitrogen, potassium, broccoli, radish, water useIntroductionSustainability of any production system requires optimal utilization of resources be it water, fertilizer or soil. Apart from the economic considerations, it is also well known that the adverse effect of injudicious use of water and fertilizers can have far reaching implications on the environment. There is, therefore, a need for technological optionsthat will help in minimizing the use of the precious resources and maximizing crop production without any detrimental impact on the environment. Drip irrigation also referred to as trickle irrigation or microirrigation, represents a definite advancement in irrigation technology with very wide implications (Sivanappan et al., 1972; Magar and Firake, 1991). It is a technique in which water is applied in precise amounts at a rate which matches the plant requirement and also maintains an optimum soil water status around the vicinity of plant roots. This technology has the greatest potential where water is either very expensive or scarce or the soils are sandy, rocky or the terrain is undulating and difficult to level. Poor quality water can also be used without significant yield reductions (Nightingale et al., 1991). As water, labour and land preparation become costlier, this technique of water application is bound to replace conventional systems. Drip irrigation has another advantage because it can also be used to apply any water soluble fertilizer or chemical in precise amounts, as and when required to match the plant needs (Clothier and Saucer, 1988; Bar-Yosef and Sagiv, 1992; Bafna et al., 1993), directly into the root zone of the crop which is referred to as Fertigation or Chemigation. This provides a means of improving nutrient use efficiency as the fertilizer applied remains confined to the root zone of the crop.Considerable amount of work has been conducted on drip irrigation in recent years but studies on the nutrient movement and distribution using drip irrigation are very few. The information on the nutrient dynamics under drip fertigation would be very useful in desiging efficient fertigation systems. In addition to nitrogen, the single most important plant nutrient, potash, a nutrient considered adequate in soils all over the world, has now been reported to be deficient in vast areas in recent years. Bar-Yosef and Sagiv (1985) showed that at the time of maximum nutrient uptake rate by several crops grown under drip irrigation, K must be supplied through the water even when it is in sufficient concentration (as exchangeable ion) in the soil. The fact that in India, the entire requirement of potassic fertilizers are imported signifies the importance of improving the potassium use efficiency through appropriate application methods. In this paper, results of experiments on the movement and distribution of nitrogen and potassium under drip fertigation have been presented and compared with conventional irrigation methods.Materials and MethodsThe studies were carried out on the experimental farm of the Water Technology Centre, Indian Agricultural Research Institute, New Delhi, India situated at 28o 38' N latitude and 77o 10' E longitude and at an altitude of 228.7 meters above mean sea level. The soil is sandy loam classified as Typic Ustocrept with the average pH and EC of the 1:2.5 soil water suspension ratio being 7.51 and 0.48 dSm-1, respectively. The organic carbon content was 0.32 percent. The available N, P and K status of surface soil was 120, 20 and and 170 kg ha-1, respectively. Nitrogen movement was studied in Sprouting Broccoli (Brassica oleracea var. italica L.) variety-Packman, sown in the nursery on 20th October, transplanted in the field on 23rd November, and harvested on 10th February. The plant to plant spacing was 30 cm while the row to row spacing was 60 cm. The recommended fertilizer dose was 200:120:150 of N:P:K kg ha-1. For Potassium studies, radish (Raphanus Sativus L. cv. Pusa Chetaki) was sown at a spacing of 45 cm x 15 cm on March 28, 2000. The recommended dose of fertilizer was 120 kg of N, 75 kg of P and 100 kg of K and harvested on May 18, 2000. The marketable yield wastaken as the total fresh weight at harvest. The following three treatments, replicated four times, were adopted for the study:T1 : Fertigation with fertilizer applied along with irrigation water in the drip system;T2 : Drip irrigation with fertilizer applied on soil; and,T3 : Conventional irrigation [Check basin (Broccoli) and Furrow (Radish)]In drip system, water was applied every alternate day based on the evapotranspiration demand of the crop computed from historical weather data. For check basin/furrow treatment, water was applied on the basis of soil moisture tension value (0.03 MPa approximately) measured by tensiometers installed in the field at 25 cm depth for broccoli and 15 cm depth for radish. All necessary measures were taken to keep the crop pest free.During the crop growth period in broccoli, observations were taken on soil moisture content, ammonium and nitrate concentrations in the various soil layers. Sampling was done from 0-5, 5-10, 10-20, 20-30, 30-50, 50-70 and 70-90 cm depth of the profile at the emitting point, and 15, 30, 45 and 60 cm horizontally away from the emitting point. The drip line was placed mid-way between the two rows of Broccoli plants. Water content of the soil samples was determined gravimetrically. Nitrate-and Ammonical-N was determined in the soil samples collected from the above depths initially, before and after each fertigation and fertilization using Tecator Automated 5020 Flow Injection Analyzer.To study the potassium movement (both horizontal and vertical) under different treatments, soil samples were collected from 0-5, 5-10, 10-20, 20-30, 30-40 cm depth of soil profile at the plant, 10 and 22.5 cm horizontally away from the plant. In case of T1, the soil samples were collected before and after each fertigation and in case of T2 and T3 at 7 DAS, 19 DAS and 31 DAS and at harvest. Concentration of Potassium (K) in the collected soil samples was evaluated using Flame Photometer method.Results and DiscussionSoil water movement and distributionInnumerable studies have been conducted on the soil water uptake and movement under drip and conventional irrigation systems. It has been established that considerable savings in water use can be achieved through drip systems and our results also indicate that at least 30 percent savings in water use can be achieved without affecting the yield. Results on this aspect are not being presented in this paper.Nitrogen movement and distributionNO3-N and NH4-N movement and distribution at different stages for T1, T2 and T3 treatments are presented here under different subheads.NO3-N movement and distributionThe distribution of nitrate-N throughout the profile varied both horizontally and vertically from the emitting point. Before the first fertigation, the nitrate nitrogen was observed to be uniformly distributed throughout the profile. When the soil samples were taken 24 hours after first fertigation, the peak NO3-N concentration below the emitter (16.16 g/g of dry soil) was found to be in 30-50 cm depth whereas for locations 15 cm, 30 cm and 45 cm away from the emitter, the peak was within 10-20 cm depth. The peak concentration was maximum for the point 15 cm from the emitter (32.13 g/g of dry soil) and observed in the 10-20 cm layer. At a distance of 60 cm from emitter the peak (14.3g/g of dry soil) was again found within 30-50 cm depth. For the point below the emitter, the NO3-N distribution at first decreased steadily upto a depth of about 25 cm followed by a sudden increase in the peak concentration in 30-50 cm layer. For 15 and 30 cm horizontal distance from the source there was a decrease in NO3-N concentration from surface to 10 cm depth, then a steep increase in concentration upto a depth of 15 cm (32.13 and 19.87 g/g of dry soil) followed by an almost uniform distribution within 15-25 cm and then a decrease in NO3-N concentration up to 90 cm. For a layer 60 cm away from the emitting point, the concentration gradually increased to a depth of 10-20 cm (17.19 g/g of dry soil) and then decreased up to 30-50 cm layer. Beyond this depth, the concentration was found to be uniform through the profile up to 70-90 cm depth. Again, the decrease in NO3-N concentration from the respective peak concentrations, for all the specified horizontal distances from the emitting point, is steeper for the point immediate to the emitting point than the locations horizontally away from the emitting point.In samples taken 24 hours before 2nd fertigation, the distribution of NO3-N was found to follow a definite pattern (increase in concentration upto a depth, then steep decrease followed by a uniform decrease) for each distance from emitter. For all distances from emitting point, the NO3-N is found to be minimum in the upper 0-10 cm (0.59 to 2.01 g/g of dry soil) and 10-20 cm (0.6-2.98 g/g of dry soil) layer. The concentration below 10-20 cm depth was found to decrease with increase in distance from emitter. The peak concentrations for the point immediate to the emitter and 15 cm from the emitter (26.45 and 18.03 g/g of dry soil) were found in 20-30 cm depth whereas for 30, 45 and 60 cm horizontal distance from emitter, the peak concentrations were 13.41, 15.02 and 11.12 g/g of dry soil, respectively and found in 30-50 cm depth.Twenty four hours after 2nd fertigation, the distribution of NO3-N was almost similar to that at 24 hours after 1st fertigation. The only difference was that the peak NO3-N concentration for the soil profile at a distance of 30 cm from emitting point was in 20-30 cm layer rather than 10-20 cm layer as found at 24 hours after first fertigation. The concentration at surface layer (0-5 cm) was more for the point near the emitter and the point 15 cm away from the emitter (15.47 and 18.62 g/g of dry soil, respectively). It was less for 30 cm from the emitter (9.45 g/g of dry soil) and almost same (7.49 and 6.07 g/g of dry soil) for points 45 cm and 60 cm distance from emitter, as compared to that found 24 hours after 1st fertigation.The peak concentration of NO3-N, was again located at the same depth 24 hours before 3rd fertigation as was observed before 2nd fertigation, but the concentrations were higher at all the points. The concentrations in 50-70 cm depth also increased for all the sampling points as compared to the sampling after the 2nd fertigation but the concentration below 70 cm remained almost the same.The distribution of NO3-N throughout the profile under study 24 hours after 3rd fertigation followed the same trend as 24 hours after 1st and 2nd fertigations. The peak NO3-N concentrations for the points 15 and 45 cm from the emitting point were found at a lower depth (20-30 cm) but for other points the peaks were found at the same depth as observed earlier.The peak NO3-N concentration for the point immediately below the emitter 24 hours before the 4th and last fertigation was more (29.73 g/g of dry soil) as compared to the previous values. The peak concentration of the point 15 cm from the emitter (31.08g/g of dry soil) was significantly higher and more than the point near the emitter. This peak concentration was located at the 30-50 cm layer.The peak NO3-N concentrations for all the points 24 hours after 4th and last fertigation were found at a depth of 30-50 cm except for the point 15 cm from the source which was found in 20-30 cm soil layer and was significantly higher (32.75 g/g of dry soil).At harvesting i.e., 8 days after last fertigation maximum NO3-N concentration was found within 30-50 cm layer (Figure 1). The peak values for the points below the emitter (27.16 g/g of dry soil) and 15 cm from emitter (29.15 g/g of dry soil) was much higher than those of other points (ranging from 12.74 to 14.26 g/g of dry soil).There were marked differences in the distribution of nitrate nitrogen t in the soil profile among fertigation (T1), drip irrigation (T2) and check basin (T3) treatments (Figure 1). For the drip fertigation treatments, the nitrate nitrogen concentration, at 24 hours following fertigation, was less in upper 0-10 cm layer with a peak concentration in 30-50 cm layer for the point immediate to the source (emitting point) and 10-20 cm for other points at different distances from the source. For the fertigation treatments, the maximum NO3-N concentration 19-20 days after each of the fertigations was found to be in 20-30 cm layer.Figure1Distribution of NO3-N and NH4-N in soil profile at harvest for (T1) fertigation, (T2) drip irrigation with soil application of fertilizer and (T3)check basin treatment.In T2, the NO3-N distribution, 24 hours after each dose of fertilizer application decreased steadily without any peak NO3 concentration within the profile under study. Here the maximum concentration was found in the upper 0-10 cm layer and decreasedwith depth. This trend was similar for all the sampling points at different distances from the emitter. The NO3-N was observed to increase 18-20 days after following application of fertilizer with an uniform distribution with 0-30 cm depth and then followed a gradual decrease. This is true for all the locations in the soil profile. The NO3-N concentrations as found in lower depths were more in this treatment as compared to other fertigation treatments, suggesting the possibility of some leaching losses of NO3-N. The NO3-N concentration was significantly higher in surface soil in this treatment than that in fertigation treatments and found to increase with each fertilizer application. In T3 (check basin treatment), NO3-N moved to deeper layer with the advance of the experiment and at the harvest, the NO3-N peak was found in 70-90 cm layer, indicating maximum leaching loss.The significant difference of NO3-N movement and distribution observed between drip fertigation, drip irrigation and check basin treatments a few days following fertigation or fertilization was that the peak concentrations of NO3-N in fertigation treatment was found at some depth in the profile (for the point near the emitter peak was formed at a greater depth than any other points following fertigation), whereas maximum NO3-N concentration in T2 treatments was found in the surface layer gradually decreasing down the profile. The peak concentration for the points near the emitting point and 15 cm from that in T1 treatment was much more than that for other points. This range was not so large for T2 treatment. For check basin treatment, the peak concentration moved to a much lower depth than other treatments with drip system, indicating loss of NO3-N out of the root zone which was found to be 10-50 cm. In both T2 and T3 treatments, urea was applied but for T2, the water was applied through drip whereas in T3, the flood irrigation system was employed. This resulted in the difference in the quantum of water applied at a time between the two treatments following application of fertilizer. Hence, NO3-N moved to a greater depth greater than 50 cm with water in check basin (T3) but in T2, it was confined within 50 cm depth.NH4-N movement and distributionThe NH4-N concentration, 24 hours after each fertigation was found mostly in the upper 0-30 cm soil layer. As NH4 is not mobile and is adsorbed to the soil matrix, its peak concentration (99.45 g/g of dry soil) was in the top 0-10 cm layer, decreased towards the deeper layers upto 40-50 cm layer and then maintained a constant value till 70-90 cm depth. For the point located close to the source, NH4-N was concentration was greater in the 0-10 cm (66.09 g/g of dry soil) than any other point. At the point 15 cm from the emitter the variation in concentration of NH4-N from top layer to the bottom layer of the profile studied was less than that for the point immediate to the source and this difference was significant. For other points, the distributions were uniform throughout the profile, indicating restricted movement of NH4-N.In T2 treatment, NH4-N was concentrated in 0-50 cm layer. In this treatment, the NH4-N concentration was more in the 0-50 cm layer than found in fertigation treatments for both the points next to the emitter and 15 cm from the emitter. In samples taken 24 hours before the next urea application, NH4-N was found more in the upper 50 cm layer than 24 hours after application, indicating urea hydrolysis.In T3 treatment (check basin), NH4-N concentration peak was found in 30-50 cm depth 24 hours after urea application. But 20 days after application, its peak wasobserved at 20-30 cm depth. This indicated conversion of NH4-N to NO3-N in the deeper layers and was reflected in NO3-N profiles.The NH4-N concentration in T1 treatment was maximum in the surface layers i.e., between 0-40 cm layer with peak values in the surface layer, decreasing depth wise. At the start of the experiment, NH4-N concentration in the deeper layer was less. But these differences increased towards the end of the experiment for all fertigation treatments both horizontally and vertically. In T2 treatment, except for the point near the emitter, the NH4-N concentration did not show any marked difference and was same throughout the profile. Figure 1 shows the distribution of NH4-N in the soil profile at harvest in the various treatments.In check basin treatment (T3), the peak was always found between 20-50 cm depth with minimum NH4-N concentration in the surface layer (0-10 cm) which was in sharp contrast to the drip irrigation and fertigation treatments, where maximum NH4-N concentration was always found in the surface layers.The increase in NH4-N concentration immediately in the vicinity of the emitter is a consequence of the hydrolysis of urea (Haynes, 1990). The consistently wet condition around the emitter also ensures that the conversion of NH4-N to NO3-N occurs some distance away from the emitter in a relatively drier zone, where more oxygen is available (Laher and Avnicmelech, 1980). This results in the peak concentration of NO-3N occurring below the soil surface in the layer (30-50 cm) and more than 15 cm away from the emitter. Ammonium ion is absorbed by the soil matrix and, therefore, the maximum concentration changes were confined to the top layer and around the emitter. There was no appreciable change beyond 30 cm away from the emitter up to the third fertilizer application. It is only after the third fertigation that the changes in NH4-N content percolate down to deeper layers. NO3-N ions, on the other hand, are considered very mobile. But unlike T3 (check basin treatment) where the soil is comparatively drier, the higher soil water status in the root zone in T1 and T2 treatments restricts the mobility of NO3 ions to the 20-30 and 30-50 cm layers. These results confirm to the observations of Dalbro and Dorph-Peterson (1976) and Haynes (1990), who have reported that solute penetration is more in an initially dry soil compared to an initially moist soil. Ghuman and Prihar (1980) who reported salt movement under different soil texture also supported these observations.In T1 and T2, the amount of fertilizer applied was identical but as a result of higher amount of water applied in T3, a significant amount of fertilizer was leached down which was reflected in the relatively lesser amounts of NH4-N and NO3-N being retained in the profile. The soil profile was relatively drier in T3 compared to other treatments and this resulted in the conversion of NH4-N to NO3-N the latter being mobile was leached down.It is often reported that nitrate ion being mobile has a tendency to more away from the emitter to the periphery of the water front (Dalbro and Dorph-Peterson, 1976) and not be available to the plant. The distribution of NO3-N in the soil profile has shown that it neither accumulates at the periphery of the wetting zone nor is leached out from the root zone under drip systems. The design of the drip irrigation system coupled with the discharge rate can, therefore, optimize both water and fertilizer use by a crop.Potassium (K) movement and distributionPotassium movement, its horizontal and vertical distribution at harvest in various treatments has been presented in Figure 2. Under the fertigation treatment T1, the distribution of Potassium varied both vertically and horizontally from the emitting point. Before first fertigation (7 DAS) itself the initial Potassium concentration indicated the decreasing trend with respect to the depth. However, before first fertigation the Potassium concentration was found to be fairly uniform with respect to horizontal distance from the emitter. After each fertigation, the similar trend was observed. Thus, in general, the potassium content was found to decrease irrespective of before or after fertigation with depth under all the fertigation treatments (Figure 2). In general, higher concentration of Potassium was found in the upper layers of the soil i.e. at 0-25 cm soil depth and lower concentration of potassium was found in the lower layers of the soil i.e. 25-40 cm soil depth. However, the peak quantity of Potassium under fertigation treatments was always found to be in the soil depth of 0-10 cm at the emitter, 10 cm and 22.5 cm from the emitter irrespective of before or after fertigation.It can also be observed that at the emitter, higher quantity was deposited in the upper layers (0-25 cm depth of soil). But at 10 cm and 22.5 cm distance from the emitter slight decrease in the quantity of Potassium was observed in the upper layers (0-25 cm depth) of the soil. Thus, as the distance from the emitter increased the Potassium quantity was found to decrease. However, the Potassium at lower depths (25-40 cm) remained almost similar and equal to the initial Potassium concentration irrespective of the distance from or at the emitter.K distribution pattern under T2 treatment indicated lower concentration of Potassium in the 0-20 and 30-40 cm depth of soil and higher concentration in the middle layers of soil (i.e. 20-30cm) irrespective of the distance from the emitter. This can be explained in the light of the fact that regular application of irrigation water has taken down the soluble Potassium to the middle layers from the upper layers. However, there was not much difference of Potassium content between that present at emitter and 10 cm from the emitter. As the distance from the emitter increased to 22.5 cm, higher level of Potassium concentration was noticed. This may be due to the depletion of the available Potassium by the plants at the emitter and 10 cm from emitter more than that at 22.5 cm from the plant.The resultant Potassium distribution profiles under T2 treatment indicated lower concentration of Potassium in the 0-20 cm depth and higher concentration in the lower layers of soil i.e. 20-40 cm soil depth irrespective of the distance from the emitter. However, the Potassium content was found to be lower near the plant compared to that present at 10 cm and 22.5 cm from the plant in the upper layers of the soil i.e. in the soil depth range of 0-20 cm. Within the depth range of 0-20 cm, the Potassium content was found to decrease with respect to depth where as that in the depth range of 20-40 cm was found to be fairly uniform with respect to depth (Figure 2). The presence of higher amount of Potassium beyond the crop root zone is not useful to crop and probably lead to more leaching loss of Potassium as a result of flood irrigation by way of furrow irrigation which ultimately resulted into lower yield under T3 treatment compared to other treatments of the present study.Figure2Distribution of potassium in soil under different treatments I: 31 days after sowing; II: at harvest; a, b, c refer to sampling points near the plant, 10 cmand 22.5 cm away from the plants, respectively T1: fertigation; T2: griipirrigation with soil application of fertilizer; and T3: furrow irrigation.Yield and water use efficiencyYields obtained in the various treatments along with the water use efficiency have been presented in Table 1. Fertigation has resulted in higher yields indicating that it is one technology that can enhance both the nutrient and water use efficiency.Table1Yield and water use of broccoli and radish in various treatments.Broccoli RadishTreatmentsYieldkg ha-1WaterappliedmmWater useefficiencykg ha-1 mmYieldkg ha-1WaterappliedmmWater useefficiencykg ha-1 mmT14301217 18.7015200 205 74.15T22343217 10.8711200 205 54.63T31997306 6.5010300 310 33.23ConclusionsThe findings of the foregoing experiments have clearly established that fertigation is definitely advantageous over drip irrigation with soil application of fertilizer and check basin irrigation with broadcast application of fertilizer. Fertigation is, therefore, a technique which economises in water and fertilizer application and increases their use efficiency.ReferencesBafna, AM, S.Y. Daftardar, K.K. Khade, P.V. Patel and R.S. Dhotre. 1993. Utilization of nitrogen and water by tomato under drip irrigation system. J. Water Management 1(1):1-5.Bar-Yosef and B. Saglv. 1985. Potassium supply to the field crops grown under drip irrigation and fertigation, pp. 185-188. In Proceedings, K Symposium, International Potash Research Institute, Praetorian.。