nature 一篇文献的翻译《生物催化工程的第三次浪潮》
bioengineering and translational medicine简介

bioengineering and translational medicine简介《Bioengineering & Translational Medicine》是一本专注于工程生物医学领域的学术期刊。
以下是关于该期刊的简要介绍:
- 该期刊由美国化学工程师协会(AlChE)于2016年创办,现为AlChE会刊,每年出版3期,现在由Wiley出版管理,期刊主编为哈佛大学的Samir Mitragotri 教授。
- 该期刊旨在及时、准确、全面地报道国内外工程生物医学工作者在该领域的科学研究等工作中取得的经验、科研成果、技术革新、学术动态等。
- 该期刊已被多个数据库收录,包括SCIE、BIOSIS Previews、STM Source、PubMed via PMC deposit (NLM)、Biotechnology Source等。
- 该期刊发表的文章类型以研究文章(Article)为主,同时也有综述(Review)、社论(Editorials)等。
- 该期刊主编Samir Mitragotri教授是美国哈佛大学的工程与应用科学教授,也是Bioengineering & Translational Medicine的期刊主编。
他是一位在药物靶向输送、生物医学材料、生物启发工程等领域有深入研究的科学家,已经撰写及合著了210余篇期刊论文,并拥有约150项专利。
总的来说,《Bioengineering & Translational Medicine》是一本在工程生物医学领域具有较高影响力和权威性的学术期刊,为该领域的科研工作者提供了重要的学术交流平台。
2020年在nature catalysis上发表重要成果
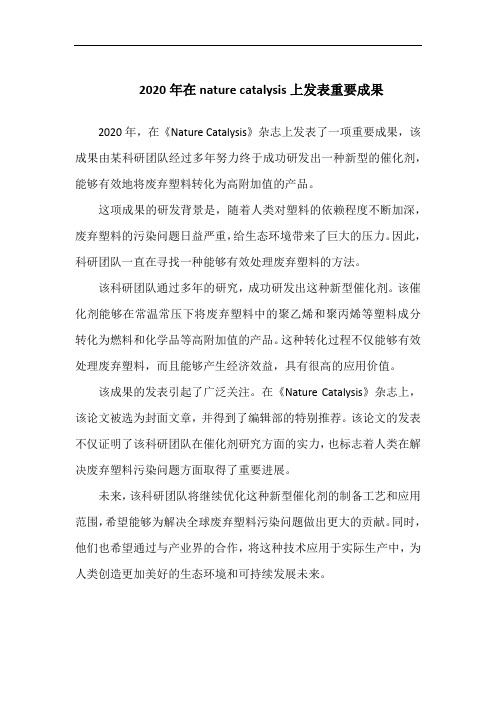
2020年在nature catalysis上发表重要成果
2020年,在《Nature Catalysis》杂志上发表了一项重要成果,该成果由某科研团队经过多年努力终于成功研发出一种新型的催化剂,能够有效地将废弃塑料转化为高附加值的产品。
这项成果的研发背景是,随着人类对塑料的依赖程度不断加深,废弃塑料的污染问题日益严重,给生态环境带来了巨大的压力。
因此,科研团队一直在寻找一种能够有效处理废弃塑料的方法。
该科研团队通过多年的研究,成功研发出这种新型催化剂。
该催化剂能够在常温常压下将废弃塑料中的聚乙烯和聚丙烯等塑料成分转化为燃料和化学品等高附加值的产品。
这种转化过程不仅能够有效处理废弃塑料,而且能够产生经济效益,具有很高的应用价值。
该成果的发表引起了广泛关注。
在《Nature Catalysis》杂志上,该论文被选为封面文章,并得到了编辑部的特别推荐。
该论文的发表不仅证明了该科研团队在催化剂研究方面的实力,也标志着人类在解决废弃塑料污染问题方面取得了重要进展。
未来,该科研团队将继续优化这种新型催化剂的制备工艺和应用范围,希望能够为解决全球废弃塑料污染问题做出更大的贡献。
同时,他们也希望通过与产业界的合作,将这种技术应用于实际生产中,为人类创造更加美好的生态环境和可持续发展未来。
nature catalysis decision sent to author 情况
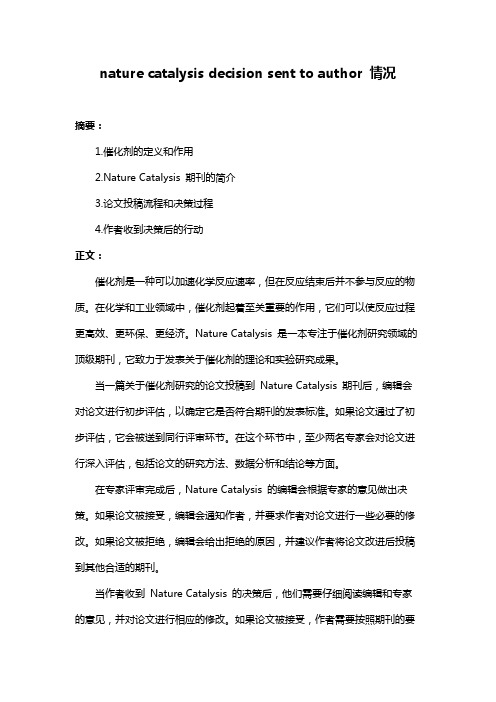
nature catalysis decision sent to author 情况
摘要:
1.催化剂的定义和作用
2.Nature Catalysis 期刊的简介
3.论文投稿流程和决策过程
4.作者收到决策后的行动
正文:
催化剂是一种可以加速化学反应速率,但在反应结束后并不参与反应的物质。
在化学和工业领域中,催化剂起着至关重要的作用,它们可以使反应过程更高效、更环保、更经济。
Nature Catalysis 是一本专注于催化剂研究领域的顶级期刊,它致力于发表关于催化剂的理论和实验研究成果。
当一篇关于催化剂研究的论文投稿到Nature Catalysis 期刊后,编辑会对论文进行初步评估,以确定它是否符合期刊的发表标准。
如果论文通过了初步评估,它会被送到同行评审环节。
在这个环节中,至少两名专家会对论文进行深入评估,包括论文的研究方法、数据分析和结论等方面。
在专家评审完成后,Nature Catalysis 的编辑会根据专家的意见做出决策。
如果论文被接受,编辑会通知作者,并要求作者对论文进行一些必要的修改。
如果论文被拒绝,编辑会给出拒绝的原因,并建议作者将论文改进后投稿到其他合适的期刊。
当作者收到Nature Catalysis 的决策后,他们需要仔细阅读编辑和专家的意见,并对论文进行相应的修改。
如果论文被接受,作者需要按照期刊的要
求进行修改,并提交最终版本的论文。
海洋天然产物喹啉类生物碱的生物活性研究进展
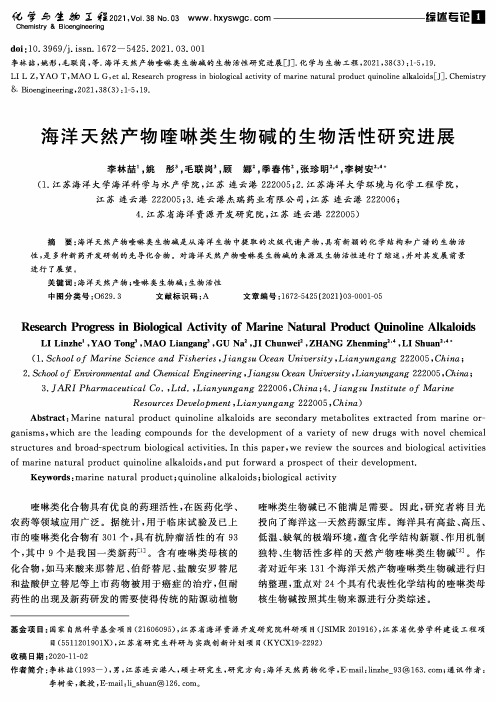
化学鸟住够3終2021,-------------------------------------------------------综施专论―Chemistry&Bioengineeringdoi:10.3969/j.issn.1672-5425.2021.03.001李林拮,姚彤,毛联岗,等.海洋天然产物喳啡类生物碱的生物活性研究进展[J].化学与生物工程,2021,38(3):1-5,19.LI L Z,YAO T?MAO L G,et al.Research progress in biological activity of marine natural product quinoline alkaloids[J].Chemistry &-Bioengineering,2021,38(3):1-5,19.海洋天然产物瞳咻类生物碱的生物活性研究进展李林詰1,姚彤3,毛联岗',顾娜笃季春伟笃张珍明2,",李树安2"(1.江苏海洋大学海洋科学与水产学院,江苏连云港222005;2.江苏海洋大学环境与化学工程学院,江苏连云港222005;3.连云港杰瑞药业有限公司,江苏连云港222006;4.江苏省海洋资源开发研究院,江苏连云港222005)摘要:海洋天然产物摩咻类生物碱是从海洋生物中提取的次级代谢产物,具有新颖的化学结构和广谱的生物活性,是多种新药开发研制的先导化合物。
对海洋天然产物喳咻类生物碱的来源及生物活性进行了综述,并对其发展前景进行了展望。
关键词:海洋天然产物;喳咻类生物碱;生物活性中图分类号:0629.3文献标识码:A文章编号:1672-5425(2021)03-0001-05Research Progress in Biological Activity of Marine Natural Product Quinoline Alkaloids LI Linzhe1,YAO Tong3,MAO Liangang3,GU Na2,JI Chunwei2,ZHANG Zhenming24,LI Shuan24*(1.School of Marine Science and Fisheries^Jiangsu Ocean University^Lianyungang222005,CAzna;2.School of Environmental and Chemical Engineering9Jiangsu Ocean University,Lianyungang222005,C加na;3.J A RI Pharmaceutical Co.,Lul・,Lianyungang2220069China;4.Jiangsu Institute of MarineResources Development9Lianyungang222005^China)Abstract:Marine natural product quinoline alkaloids are secondary metabolites extracted from marine organisms9which are the leading compounds for the development of a variety of new drugs with novel chemical structures and broad-spectrum biological activities.In this paper,we review the sources and biological activities of marine natural product quinoline alkaloids?and put forward a prospect of their development.Keywords:marine natural product;quinoline alkaloids;biological activity嗟咻类化合物具有优良的药理活性,在医药化学、农药等领域应用广泛。
托夫勒的《第三次浪潮》
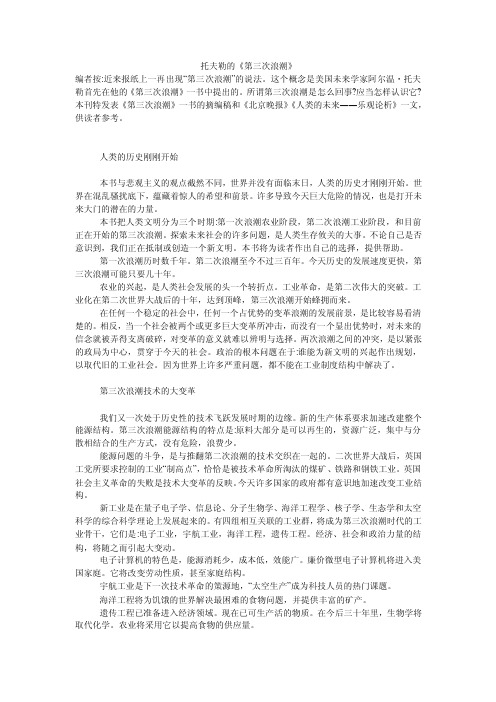
托夫勒的《第三次浪潮》编者按:近来报纸上一再出现“第三次浪潮”的说法。
这个概念是美国未来学家阿尔温・托夫勒首先在他的《第三次浪潮》一书中提出的。
所谓第三次浪潮是怎么回事?应当怎样认识它?本刊特发表《第三次浪潮》一书的摘编稿和《北京晚报》《人类的未来――乐观论析》一文,供读者参考。
人类的历史刚刚开始本书与悲观主义的观点截然不同,世界并没有面临末日,人类的历史才刚刚开始。
世界在混乱骚扰底下,蕴藏着惊人的希望和前景。
许多导致今天巨大危险的情况,也是打开未来大门的潜在的力量。
本书把人类文明分为三个时期:第一次浪潮农业阶段,第二次浪潮工业阶段,和目前正在开始的第三次浪潮。
探索未来社会的许多问题,是人类生存攸关的大事。
不论自己是否意识到,我们正在抵制或创造一个新文明。
本书将为读者作出自己的选择,提供帮助。
第一次浪潮历时数千年。
第二次浪潮至今不过三百年。
今天历史的发展速度更快,第三次浪潮可能只要几十年。
农业的兴起,是人类社会发展的头一个转折点。
工业革命,是第二次伟大的突破。
工业化在第二次世界大战后的十年,达到顶峰,第三次浪潮开始蜂拥而来。
在任何一个稳定的社会中,任何一个占优势的变革浪潮的发展前景,是比较容易看清楚的。
相反,当一个社会被两个或更多巨大变革所冲击,而没有一个显出优势时,对未来的信念就被弄得支离破碎,对变革的意义就难以辨明与选择。
两次浪潮之间的冲突,是以紧张的政局为中心,贯穿于今天的社会。
政治的根本问题在于:谁能为新文明的兴起作出规划,以取代旧的工业社会。
因为世界上许多严重问题,都不能在工业制度结构中解决了。
第三次浪潮技术的大变革我们又一次处于历史性的技术飞跃发展时期的边缘。
新的生产体系要求加速改建整个能源结构。
第三次浪潮能源结构的特点是:原料大部分是可以再生的,资源广泛,集中与分散相结合的生产方式,没有危险,浪费少。
能源问题的斗争,是与推翻第二次浪潮的技术交织在一起的。
二次世界大战后,英国工党所要求控制的工业“制高点”,恰恰是被技术革命所淘汰的煤矿、铁路和钢铁工业。
生物催化作业原文
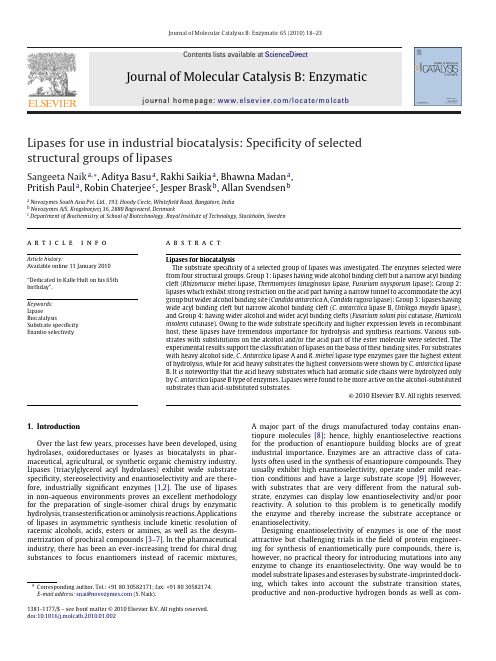
Journal of Molecular Catalysis B:Enzymatic 65 (2010) 18–23Contents lists available at ScienceDirectJournal of Molecular Catalysis B:Enzymaticj o u r n a l h o m e p a g e :w w w.e l s e v i e r.c o m /l o c a t e /m o l c a tbLipases for use in industrial biocatalysis:Specificity of selected structural groups of lipasesSangeeta Naik a ,∗,Aditya Basu a ,Rakhi Saikia a ,Bhawna Madan a ,Pritish Paul a ,Robin Chaterjee c ,Jesper Brask b ,Allan Svendsen baNovozymes South Asia Pvt.Ltd.,193,Hoody Circle,Whitefield Road,Bangalore,India bNovozymes A/S,Krogshoejvej 36,2880Bagsvaerd,Denmark cDepartment of Biochemistry at School of Biotechnology,Royal Institute of Technology,Stockholm,Swedena r t i c l e i n f o Article history:Available online 11 January 2010“Dedicated to Kalle Hult on his 65th birthday”.Keywords:LipaseBiocatalysisSubstrate specificity Enantio selectivitya b s t r a c tLipases for biocatalysisThe substrate specificity of a selected group of lipases was investigated.The enzymes selected were from four structural groups.Group 1:lipases having wide alcohol binding cleft but a narrow acyl binding cleft (Rhizomucor miehei lipase,Thermomyces lanuginosus lipase,Fusarium oxysporum lipase);Group 2:lipases which exhibit strong restriction on the acid part having a narrow tunnel to accommodate the acyl group but wider alcohol binding site (Candida antarctica A,Candida rugosa lipase);Group 3:lipases having wide acyl binding cleft but narrow alcohol binding cleft (C.antarctica lipase B,Ustilago maydis lipase),and Group 4:having wider alcohol and wider acyl binding clefts (Fusarium solani pisi cutinase,Humicola insolens cutinase).Owing to the wide substrate specificity and higher expression levels in recombinant host,these lipases have tremendous importance for hydrolysis and synthesis reactions.Various sub-strates with substitutions on the alcohol and/or the acid part of the ester molecule were selected.The experimental results support the classification of lipases on the basis of their binding sites.For substrates with heavy alcohol side,C.Antarctica lipase A and R.miehei lipase type enzymes gave the highest extent of hydrolysis,while for acid heavy substrates the highest conversions were shown by C.antarctica lipase B.It is noteworthy that the acid heavy substrates which had aromatic side chains were hydrolyzed only by C.antarctica lipase B type of enzymes.Lipases were found to be more active on the alcohol-substituted substrates than acid-substituted substrates.© 2010 Elsevier B.V. All rights reserved.1.IntroductionOver the last few years,processes have been developed,using hydrolases,oxidoreductases or lyases as biocatalysts in phar-maceutical,agricultural,or synthetic organic chemistry industry.Lipases (triacylglycerol acyl hydrolases)exhibit wide substrate specificity,stereoselectivity and enantioselectivity and are there-fore,industrially significant enzymes [1,2].The use of lipases in non-aqueous environments proves an excellent methodology for the preparation of single-isomer chiral drugs by enzymatic hydrolysis,transesterification or aminolysis reactions.Applications of lipases in asymmetric synthesis include kinetic resolution of racemic alcohols,acids,esters or amines,as well as the desym-metrization of prochiral compounds [3–7].In the pharmaceutical industry,there has been an ever-increasing trend for chiral drug substances to focus enantiomers instead of racemic mixtures,∗Corresponding author.Tel.:+918030582171;fax:+918030582174.E-mail address:snai@ (S.Naik).A major part of the drugs manufactured today contains enan-tiopure molecules [8];hence,highly enantioselective reactions for the production of enantiopure building blocks are of great industrial importance.Enzymes are an attractive class of cata-lysts often used in the synthesis of enantiopure compounds.They usually exhibit high enantioselectivity,operate under mild reac-tion conditions and have a large substrate scope [9].However,with substrates that are very different from the natural sub-strate,enzymes can display low enantioselectivity and/or poor reactivity.A solution to this problem is to genetically modify the enzyme and thereby increase the substrate acceptance or enantioselectivity.Designing enantioselectivity of enzymes is one of the most attractive but challenging trials in the field of protein engineer-ing for synthesis of enantiometically pure compounds,there is,however,no practical theory for introducing mutations into any enzyme to change its enantioselectivity.One way would be to model substrate lipases and esterases by substrate-imprinted dock-ing,which takes into account the substrate transition states,productive and non-productive hydrogen bonds as well as com-1381-1177/$–see front matter © 2010 Elsevier B.V. All rights reserved.doi:10.1016/j.molcatb.2010.01.002S.Naik et al./Journal of Molecular Catalysis B:Enzymatic65 (2010) 18–2319Table1Classification of lipases on the basis of structural and physico-chemical properties of scissile fatty acid binding sites.Lipase class Group Active-site propertiesRmL type1Large alcohol binding cleft but a narrowacyl binding cleftCaLA type2Strong restriction on the acid part having anarrow tunnel to accommodate the acylgroup but wider alcohol binding siteCaLB type3Lipases having large acyl binding cleft butnarrow alcohol binding cleftCutinase type4Having wider alcohol and wider acylbinding cleftplete proteinflexibility.[10].Another approach would be to incorporate a desired enantioselectivity to enzymes by directed evolutionary strategy,which comprises iterative cycles of mutation and identification of improved variants by screening or selec-tion[11–14].In one report,CaLA was made enantioselective for 4-nitrophenyl2-methylheptanoate,by using the CASTing(Com-binatorial active-site saturation test)[15],while another that the enantioselectivity of hydantoinase has been inverted towards D,L-5-(2-methylthioethyl)hydantoin by error-prone PCR and fol-lowing saturation mutagenesis[16],and enantioselectivity of a lipase from Pseudomonas aeruginosa was inverted by the combi-nation of error-prone PCR and DNA shuffling[17].Lipases have been classified on the basis of structural and physico-chemical properties of scissile fatty acid binding sites to understand the substrate specificity of lipases[18].In the current study,the substrate specificity of four structural groups of lipases (Table1):Group1:lipases having large alcohol binding cleft but a narrow acyl binding cleft(Rhizomucor miehei lipase(RmL),Ther-momyces lanuginosus lipase(TlL),Fusarium oxysporum lipase(FoL)); Group2:exhibit strong restriction on the acid part having a narrow tunnel to accommodate the acyl group but wider alcohol bind-ing site(Candida antarctica A(CaLA),Candida rugosa lipase(CrL)); Group3:lipases having large acyl binding cleft but narrow alcohol binding cleft(C.antarctica lipase B(CaLB),Ustilago maydis lipase (UmL)),and Group4:having wider alcohol and wider acyl binding cleft(Fusarium solani pisi cutinase(FsC),Humicola insolens cutinase (HiC))was investigated.The work presented here compares the substrate specificity of the various groups of lipases and also looks at the enantioselectivity of CaLB and some of its reported variants,M72L,T103G,and W104H [19].2.Experimental2.1.Strains,culture media and growth conditionsPichia.pastoris CoLS702[Mut−]strain(this strain is a deletion mutant of P.pastoris GS115strain,wherein the AOX1gene has been deleted)was used for heterologous expression of CaLA,CaLB, TlL,CrL-1,HiC and CaLB variants T103G,M72L and W104H.The strains were grown and maintained in YPD medium containing yeast extract(10g/l),peptone(20g/l),and dextrose(20g/l).For expression of the heterologous protein,the Pichia fermentation medium consisted of yeast extract(10g/l),peptone(20g/l),sor-bitol(10g/l),Potassium phosphate buffer(pH6.0,50mM),and yeast nitrogen base without ammonium sulphate and amino acids (3.4g/l).All fermentations were carried out in baffled Erlenmeyer flasks(1l)containing300ml of fermentation medium at27.5◦C and150rpm.The cells were induced after24h of growth with methanol(1%)and the temperature reduced to22◦C.Methanol induction was followed every24h for6days after which the cells were removed by centrifugation at12,000×g for25min,the super-natant wasfiltered through0.2hollowfibrefilter and processed for purification.2.2.Construction of expression plasmidsA P.pastoris vector CoLS789was used for the expression of CaLA, CaLB,TlL,CrL-1,HiC and CaLB variants T103G,M72L and W104H. This in-house vector has a HIS4selection marker,a multiple cloning site,the3 and5 sequences of a AOX1gene of P.pastoris and a pUC origin of replication.The genes with the native peptide signal were sub-cloned under the control of AOX1promoter.The resulting con-structs were used to transform Escherichia coli DH5␣.The plasmids were subjected to DNA sequencing for confirming the sequence.2.3.Preparation of P.pastoris competent cells and transformationYPD100mL(1%(w/v)yeast extract,2%(w/v)peptone,2%(w/v) dextrose)medium was inoculated with a single P.pastoris colony and grown over night at30◦C,200rpm to an OD600of0.8–1.0. Cells were collected by centrifugation(10min,1500×g,and20◦C), washed with50ml of sterile water and suspended in2ml of 100mM LiCl2.The cells were washed twice with100mM LiCl2 and distributed in100l aliquots.To each cell aliquot was added 240L of PEG3350(50%),36L of LiCl2(1M),25L of single stranded DNA and50L of linearized plasmid DNA.The cells were mixed and were incubated at30◦C for30min without shaking and heat shock was given at42◦C for25min.The transformation mix was centrifuged and supernatant was discarded.The transformants were suspended in100L of sterile water spread on SD(Syn-thetic Dropout)plates(1.34%Yeast Nitrogen Base with ammonium sulphate without amino acids),4×10−5%(w/v)biotin,2%(w/v) dextrose)and incubated at30◦C for2–4days.The transformants were selected by their ability to synthesize and utilize histidine. 2.4.PurificationThe purification methods for the various proteins are outlined below.Unless otherwise stated all columns used were of20ml col-umn volume(CV),and equilibration,washing and elution steps consisted to10CV buffer.2.4.1.CaLA and CaLBAmmonium sulphate(0.8M)was added to the fermentation broth and the broth passed through Butyl toyopearl hydropho-bic column which was previously equilibrated with Ammonium acetate(0.8M).The matrix was washed with equilibration buffer and the bound protein was eluted isocratically,first with water and later with ethanol(50%).The eluted protein was dialyzed against HEPES(pH7.0,50mM)and passed through UnoQ(anion exchange). The unbound sample consisted of the purified protein which was concentrated using centrifugal concentrators and used for enzyme assay.2.4.2.TlLSodium chloride(2M)was added to the fermentation broth and the broth passed through Decyl agarose hydrophobic col-umn which had been previously equilibrated with sodium borate buffer(pH9.0,50mM).The matrix was washed with equilibration buffer and the bound protein was eluted isocratically with equi-libration buffer containing Isopropanol(30%).The eluted fraction were pooled,the conductivity was adjusted<7mS/cm and passed through Q-Sepharose(anion exchange)which had been previously equilibrated with sodium borate buffer(pH9.0,50mM).The matrix was washed with equilibration buffer and the bound protein was eluted using a linear gradient of sodium chloride(1M)in equilibra-20S.Naik et al./Journal of Molecular Catalysis B:Enzymatic65 (2010) 18–23Fig.1.The four selected structural classes of lipases.Representatives from each group oriented with superimposed active site.Active-site serine is shown in yellow,and with the acid binding part pointing upwards and the alcohol binding part pointing downwards.For the CrL the acid binding part is a deep tunnel going into the structure.Group 1;RmL (TlL:1GT6),Group 2;CaLA (CrL;1CrL),Group 3;CaLB (CaLB;1TCA),and Group 4;FsC (FsC;1CUS).tion buffer.The eluted protein was pooled,concentrated and used for enzyme assay.2.4.3.CrL-1Ammonium sulphate (0.8M)was added to the fermentation broth and the broth passed through Butyl toyopearl hydropho-bic column which was previously equilibrated with Ammonium acetate (0.8M).The matrix was washed with equilibration buffer and the bound protein was eluted isocratically first with HEPES (pH 7.0,50mM)and later with HEPES (pH 7.0,50mM)contain-ing ethanol (50%).The eluted protein was dialyzed against HEPES (pH 7.0,50mM)and passed through UnoQ (anion exchange).The matrix was washed with equilibration buffer and the bound pro-tein was eluted using a linear gradient of sodium chloride (1M)in equilibration buffer.The eluted protein was pooled,concentrated and used for enzyme assay.2.5.Enzyme assaysThe activity of CaLA,CaLB,TlL,CrL-1,HiC and CaLB variants T103G,M72L and W104H were carried out in HEPES buffer (pH 7.050mM)containing CaCl 2(5mM)and Triton-X-100(0.4%).The reaction was started by the addition of substrate (40mM)and enzyme (0.1mg).All the reactions were performed at 30◦C for 2h,6h,or 24h to get appropriate conversion.The reactions were stopped with 100l HCl (1M)and the reactants extracted with dichloromethane.The organic phase (20l)was diluted with diethylether and analyzed on GC fitted with chiral GC column (Var-ian CP-Chiralsil-DEX CB 10m).Two compounds,one from each substrate categories (A)sub-strates either branched/large on the alcohol part or (B)substrates either branched/large on the acid part were checked for enantiose-lective degradation of chiral isomers.The separation procedure for the two isomers on GC is outlined below.2-ethyl hexyl acetate:Oven T 1=70◦C,T 2=80◦C ( T =1◦C/min),T 3=90◦C ( T =2◦C/min),T 4=150◦C ( T =10◦C/min);Injector T =220◦C;Detector T =250◦C.Carrier N 2,flow rate =0.5ml/min.Ethyl-2-methyl butyrate:Oven T 1=40◦C for 2min,T 2=52◦C ( T =2◦C/min),T 3=150◦C ( T =20◦C/min),Injector T =220◦C;Detector T =250◦C.Carrier N 2,flow rate =1ml/min.Enantioselectivity (E )was calculated using below Equation.The activity is given as percentage conversion of the total amount sub-strate.E =ln {ee p (1−ee s )/(ee s +ee p )}ln {ee p (1+ee s )/(ee s +ee p )}3.Results and discussionLipases have been of significant interest because of their wide substrate specificity.In this study,the specificity of lipases belonging to four structural groups (Table 1)has been exploited over a range of substrates based on the substrate site bind-ing geometry.Fig.1illustrates the four structural classes in this paper.The structural geometry of the lipase is the first indication on the substrate binding possibility in the specific lipase type.Hydrogen bonding,van der Waals contacts and elec-trostatic interactions are the second important factors for the binding possibility.The X-ray structures indicate the potential main structural appearance of the enzyme under activated and inactivated conditions,respectively.In the present work the X-ray structures in the so-called “open”forms are used.For the practical measurements the structural forms may be dif-ferent and of course more dynamic,and for the lipases with lids and flaps which need activation (like TlL,RmL,FoL,CrL,etc.)the experimental conditions have special impact on the binding of substrate to the enzyme.The assay condition has thus been focused to work with both lipases that need activa-tion (CaLA)and those that need little or no activation (FsC and CaLB).In accordance with the above hypothesis,two categories of substrates i.e.fatty acid esters were selected (A)substrates either branched/large on the alcohol part or (B)substrates either branched/large on the acid part.Examples included in first category substrates—Isobutyl propionate,Styrallyl butyrate,2-ethyl-hexyl acetate and Cyclohexyl acetate.Examples included in the second category substrates—Ethyl-2-methyl butyrate,Ethyl-2-ethyl-hexanoate,Ethyl benzoate and ethyl-2-phenylpropionate.One of these substrates –ethyl-2-phenylpropionate is shown in Fig.2,which shows how the substrate –a profen core [20]–docks in a substrate binding pocket of CaLB (Fig.2).Most of the enzymes accept group “A”substrates,however,CaLA type enzymes,which are capable of accepting large alcohol groups,show highest activity.CaLB on the other hand is more strin-gent with respect to alpha carbon branching on the alcohol side as shown by its activity on styrallyl butyrate (Fig.3A).2-ethylhexyl acetate was chosen as a representative substrate for category A substrate and the enantioselectivity of all four groups of enzymes was checked this compound.Most of the enzymes except CaLB could not distinguish between the enantiomers (Fig.4).Docking studies (not shown here)showed that the different enan-tiomers bind in a similar fashion,which makes it hard for theS.Naik et al./Journal of Molecular Catalysis B:Enzymatic65 (2010) 18–2321Fig.2.(A)The acid and alcohol part of the substrate.(B)A profen core compound docked in the active site of CaLB.Fig.3.Enzyme activity on“branched”/“large”substrates on alcohol part(A)and acid part(B).The activity is given in conversion percentage of total amount substrate.Two conditions were chosen:Triton0,1%or Acetone5%,Buffer:50mM Tris-buffer,pH7,Amount of enzyme:0,5mg,Substrate volume:50l,All the reactions were performed in 30◦C and24h.The reactions were stopped with100l1M HCl and the reactants are extracted with dichloromethane.20l of the organic phase was diluted with diethylether and put on the GC.22S.Naik et al./Journal of Molecular Catalysis B:Enzymatic65 (2010) 18–23Fig.3.(Continued ).enzyme to experience any difference.This is probably due to the negligible size differences of the substituents in the branch-ing.CaLB showed very high enantioselectivity on 2-ethyl hexyl acetate.Therefore,it was decided to check the enantioselectivity of CaLB variants T103G,M72L and W104H [19]on the same sub-strate.The CaLB variant T103G which introduced the consensus mutation G-X-S-X-G in CaLB,thereby increasing thermostabil-ity,has shown reduced enantioselectivity towards 2-ethyl hexyl acetate (Fig.4).The CaLB variant W104H in which more space is introduced into the active site has shown 6.7-fold increase in enantioselectivity (Fig.4).The CaLB variant M72L having higher oxidation stability has also shown decrease in the enantioselectiv-ity (Fig.4).In group B substrates,ethyl-2-methyl butyrate was easily hydrolyzed by most of the enzymes (Fig.3B).The analysis based on molecular docking of CrL and TlL with this substrate showed that the R-enantiomer docked in an active fashion while the S docked with the oxygen orientated in the wrong direc-tion.This has also been shown by the results in which CaLA is showing better enantioselectivity than all the other groups (Fig.4).The hydrolysis of all other substrates was enzyme specific.RmL was the only enzyme which was capable of hydrolyzing Ethyl 2-ethylhexanoate (larger branch on acid part)(Fig.3B).This is prob-ably due to the large hydrophobic area of the active site of the RmL.Ethyl benzoate which had benzene ring on acid part was hydrolyzed only by CrL,TlL and CaLB (Fig.3B)whereas phenyl propionic acid ethyl ester having profen core structure was hydrolyzed only by enzymes in the CaLB group (Fig.3B).In conclusion,lipases are found to be more active on the alcohol branched substrates than acid-branched substrates.As depicted in the figures,for alcohol side large substrates,CaLA and RmL type enzymes give the highest extent of hydrolysis,while for large acid substrates the highest conversion was shown by CaLB.It is note-S.Naik et al./Journal of Molecular Catalysis B:Enzymatic65 (2010) 18–2323Fig.4.Enantioselective ratio of CaLA,CaLB,CaLB(T103G),CaLB(M72L)and CaLB (W104H),HiC,TlL and CrL-1on2-ethyl hexyl acetate and Ethyl-2-methyl butyrate.worthy that the large acid substrates which had aromatic side chains were hydrolyzed only by CaLB type of enzymes.4.ConclusionsStructural space for acceptance of substrates of different shapes in lipases from the groups of RmL,CaLA,CaLB and Cutinase has been explored.The RmL group allows large alcohol part substrate,but more limited/narrow acyl binding cleft on the acid part.CaLA group exhibits strong restriction on the acid part having a narrow tunnel to accommodate the acyl group from the acid part of the substrate and a much wider space on the alcohol part allowing highly branched and large groups on the alcohol part.The CaLB group shows more space on the acid part and less on the alcohol part and with restriction on the branching at CA carbon.Cutinase group has a reasonable open space on both the alcohol and the acid part of the substrate.This structural understanding was tested with the8substrates giving an overall result pointing in the same direction as the structural understanding.References[1]K.E.Jaeger,T.Eggert,Curr.Opin.Biotechnol.13(2002)390–397.[2]M.T.Reetz,Curr.Opin.Chem.Biol.6(2002)145–150.[3]S.T.Chen,J.M.Fang,.Chem.62(1997)4349–4357.[4]T.Shibatani,K.Omori,H.Akatsuka,E.Kawai,H.Matsumae,J.Mol.Catal.B.Enzym.10(2000)141–149.[5]H.Bernsmann,M.Gruner,P.Metz,Tetrahedron Lett.41(2000)7629–7633.[6]U.Ader,P.Andersch,M.Berger,U.Goergens,R.Seemayer,M.Schneider,PureAppl.Chem.64(1992)1165–1170.[7]V.Gotor-Fernandez,R.Brieva,V.Gotor,J.Mol.Catal.B Enzym.40(2006)111–120.[8]G.Beck,Synlett(2002)837–850.[9]O.Kirk,T.V.Borchert,C.C.Fuglsang,Curr.Opin.Biotechnol.13(2002)345–351.[10]P.B.Juhl,P.Trodler,S.Tyagi,J.Pleiss,BMC Struct.Biol.9(2009).[11]D.E.Robertson,B.A.Steer,Curr.Opin.Chem.Biol.8(2004)141–149.[12]P.A.Romero,F.H.Arnold,Nat.Rev.Mol.Cell Biol.10(2009)866–876.[13]M.J.Dougherty,F.H.Arnold,Curr.Opin.Biotechnol.20(2009)486–491.[14]J.D.Bloom, F.H.Arnold,A106(Suppl.1)(2009)9995–10000.[15]A.G.Sandstrom,K.Engstrom,J.Nyhlen,A.Kasrayan,J.E.Backvall,Protein Eng.Des.Sel.22(2009)413–420.[16]O.May,P.T.Nguyen,F.H.Arnold,Nat.Biotechnol.18(2000)317–320.[17]D.Zha,S.Wilensek,M.Hermes,E.J.Karl,M.T.Reetz,mun.(2001)2664–2665.[18]J.Pleiss,M.Fischer,R.D.Schmid,Chem.Phys.Lipids93(1998)67–80.[19]S.Patkar,J.Vind,E.Kelstrup,M.W.Christensen,A.Svendsen,K.Borch,O.Kirk,Chem.Phys.Lipids93(1998)95–101.[20]E.Henke,S.Schuster,H.Yang,U.T.Bornscheuer,Monatshefte fur Chemie131(2000)633–638.。
生命科学的第三次革命

⽣命科学的第三次⾰命 苏珊·霍克菲尔德(SusanHockfield)⾃2004年12⽉起任⿇省理⼯学院第16任校长以来,直⼒倡导和推动科学技术以及研究型⼤学在世界事务中发挥重要作⽤ 霍克菲尔德1985年在耶鲁⼤学教授神经⽣物学,1994年被评正教授。
她在耶鲁⼤学度过了19春秋的学术⽣涯,曾经先后担任其研究⽣院院长、学校教务长、学术和⾏政主任等,在⼤学⾥发挥了核⼼领导作⽤。
霍克菲尔德致⼒于⼤脑发育和神经胶质瘤(⼀致命脑癌)的研究,在脑科学研究中开创了单克隆抗体技术的应⽤,并发现了⼀种在早期调节⼤脑神经元结构的蛋⽩质。
最近她发现了在⼤脑肿瘤扩散中起关键作⽤的⼀种基因及其产⽣的蛋⽩质家族,有可能成为脑胶质瘤靶向治疗的治疗靶点。
她在1973年获得美国罗切斯特⼤学(University of Rochester)的⽣物学学⼠。
1979年获得美国乔治城⼤学(Georgetown University)医学院的神经学博⼠。
1979⾄1980年为美国NIH的博⼠后研究员,1980年加盟美国纽约冷泉港实验室,1985⾄1997年任该实验室夏季神经⽣物学研究项⽬主任。
霍克菲尔德博⼠被布朗⼤学、清华⼤学以及沃森⽣物科学学院分别授予荣誉学位,并当选为美国艺术与科学院成员。
下⼀场创新⾰命来⾃⽣命科学、物理科学和⼯程科学领域内正在发⽣的历史性的⼤融合 我很⾼兴能在此与⼤家相聚⼀堂,确实有⼀些值得我们庆贺的事情在发⽣。
美国总统奥巴马最近在美国科学院的讲话中对范围⼴泛的科学与⼯程学的价值坚定地给予了认可,我们不能将其视为⼀席空谈⽽⼀笑置之。
让我们摘引奥巴马总统讲话中的⼀段以共勉之: 在⾦融危机的困难时刻,有⼈认为我们没有⼒量再投资于科学,在个钱要⽤在⼑⼝上的时期,⽀持科学研究在⼀定程度上是⼀种奢侈的花费。
但我本⼈完全不同意这种看法。
科学对于我们的繁荣、安全、健康、环境以及⽣活质量的意义,⽐以往任何时候都为重要。
当代研究生英语 第七单元 B课文翻译
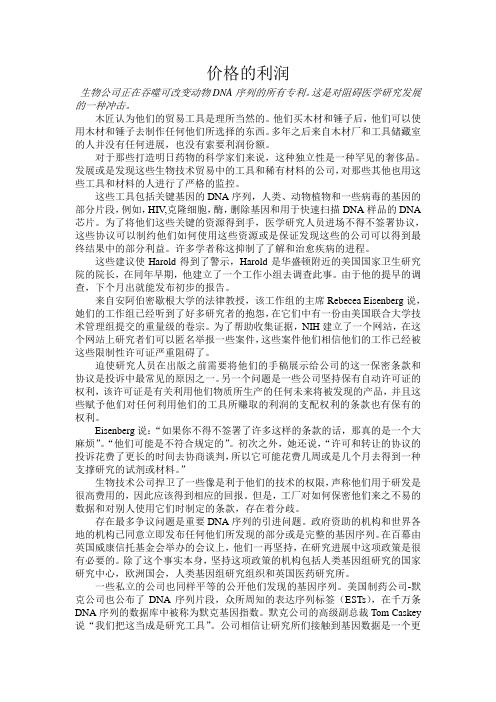
价格的利润生物公司正在吞噬可改变动物DNA序列的所有专利。
这是对阻碍医学研究发展的一种冲击。
木匠认为他们的贸易工具是理所当然的。
他们买木材和锤子后,他们可以使用木材和锤子去制作任何他们所选择的东西。
多年之后来自木材厂和工具储藏室的人并没有任何进展,也没有索要利润份额。
对于那些打造明日药物的科学家们来说,这种独立性是一种罕见的奢侈品。
发展或是发现这些生物技术贸易中的工具和稀有材料的公司,对那些其他也用这些工具和材料的人进行了严格的监控。
这些工具包括关键基因的DNA序列,人类、动物植物和一些病毒的基因的部分片段,例如,HIV,克隆细胞,酶,删除基因和用于快速扫描DNA样品的DNA 芯片。
为了将他们这些关键的资源得到手,医学研究人员进场不得不签署协议,这些协议可以制约他们如何使用这些资源或是保证发现这些的公司可以得到最终结果中的部分利益。
许多学者称这抑制了了解和治愈疾病的进程。
这些建议使Harold得到了警示,Harold是华盛顿附近的美国国家卫生研究院的院长,在同年早期,他建立了一个工作小组去调查此事。
由于他的提早的调查,下个月出就能发布初步的报告。
来自安阿伯密歇根大学的法律教授,该工作组的主席Rebecea Eisenberg说,她们的工作组已经听到了好多研究者的抱怨,在它们中有一份由美国联合大学技术管理组提交的重量级的卷宗。
为了帮助收集证据,NIH建立了一个网站,在这个网站上研究者们可以匿名举报一些案件,这些案件他们相信他们的工作已经被这些限制性许可证严重阻碍了。
迫使研究人员在出版之前需要将他们的手稿展示给公司的这一保密条款和协议是投诉中最常见的原因之一。
另一个问题是一些公司坚持保有自动许可证的权利,该许可证是有关利用他们物质所生产的任何未来将被发现的产品,并且这些赋予他们对任何利用他们的工具所赚取的利润的支配权利的条款也有保有的权利。
Eisenberg说:“如果你不得不签署了许多这样的条款的话,那真的是一个大麻烦”。
- 1、下载文档前请自行甄别文档内容的完整性,平台不提供额外的编辑、内容补充、找答案等附加服务。
- 2、"仅部分预览"的文档,不可在线预览部分如存在完整性等问题,可反馈申请退款(可完整预览的文档不适用该条件!)。
- 3、如文档侵犯您的权益,请联系客服反馈,我们会尽快为您处理(人工客服工作时间:9:00-18:30)。
生物催化工程的第三次浪潮过去的十年里,由于科技的进步,无论是实验室还是工业规模都已确定用具实用性并且环保的生物催化来代替化学合成中的传统的金属催化和有机催化。
DNA测序以及基因合成的关键进展是基于剪切生物催化剂通过蛋白质工程和设计,及将酶整合入新的生物合成途径的能力取得的巨大进步。
为了突出这些成就,在此我们讨论了以酶催化作为关键步骤,将蛋白质-动力学生物催化剂应用于从通用化学品到先进医药中间体的范围。
生物催化是对合成化学中微生物和酶的应用,作为自然界的催化用于新的目的:酶的应用还未涉及到[1-5]。
通过几次技术研究创新的浪潮,目前生物催化领域已达到其企业成熟水平。
图1酶发现的进程及用于确定所需催化剂的蛋白质工程策略理性设计(b)基于蛋白结构(a)或是同源模建识别不同的突变位点,而随机突变(c)与筛选或是选择结合是定向进化实验的基础。
结合这些方法使构建更小型但更智能的数据库(d)成为可能。
现在通过富集培养(e)对酶进行传统筛选已被关键的主题数据库检索(f)代替以指导新型酶或是他们具备的所需特性的确定。
在其初期仍是酶的设计(g)的从头计算(从头合成de novo)。
内部结构指的是通过生物催化不同的浪潮可得到的进行化学物质。
(R)-苯乙醇腈(左)在100年前的植物提取物中已经获得;(1S,3S)-3-氨基环己醇(中)由Novartis公司利用一种固定化酯酶制成;6-氯-2,4,6-3脱氧-D-赤型六吡喃环(右)由DSM用一个设计的醛缩酶耐受高浓度乙醛并且获得高选择性的过程制的。
生物催化的第一次浪潮(图1),始于一个多世纪以前,科学家认识到活细胞的组成成分可以应用于有效地生物转化(相对于几千年已经司空见惯的发酵过程)。
例如,Rosenthaler利用一种植物提取物从苯甲醛和氰化氢合成的(R)-苯乙醇腈[6];发生在微生物细胞内的类固醇的羟化[7]也已知。
较新的例子即洗衣粉中蛋白酶的利用[8]。
葡萄糖异构酶将葡萄糖转化为更甜味的果糖[9],青霉素G酰基转移酶制备半合成抗体[10]。
这些应用关键挑战在于生物催化剂稳定性的限制及诸如此类的缺点主要通过酶的固定化来克服,这也有利于酶的重复利用。
生物催化的第二次浪潮,在20世纪80到90年代,最初的蛋白质动力学技术,代表性的即基于结构的技术,扩大了酶的底物范围以允许异常的合成中间产物的合成。
这一变化将生物催化扩展到医药中间体和精细化学品的制备。
实例包括脂肪酶催化水解手性前体用于合成地尔硫卓(一种治疗血压药物),醇腈酶催化合成醇类对映异构体应用于降胆固醇抑制素药物,脂肪酶催化合成蜡酯类物质例如肉豆蔻醇肉豆蔻酸酯或是十六烷基蓖麻醇酸酯用于化妆品工业,以及腈类水合酶催化水合丙烯腈形成丙烯酰胺用于高分子材料(这类腈类水合酶已在紫红红球菌全细胞中获得)。
除固定化之外,目前的挑战包括优化用于非天然底物的催化剂。
现阶段,生物催化的第三大浪潮开始于20世纪九十年代中后期Pim Stemmer 和Frances Arnold的工作。
他们首创了分子生物学方法,通过达尔文进化论体外实验快速大量地修饰催化剂。
尽管这一术语于1972年的全细胞实验中曾被用过,现在这一方法通常称为定向进化,这一技术的最初方法涉及到在一个蛋白质中氨基酸的随机突变的迭代循环法,随后从酶稳定性提高,底物特异性以及对应选择性的突变体形成的库中筛选法。
讨论到此,今后的发展已经集中于提高定向进化的效率以产生“更智能地”数据库。
工业生物催化主要集中于水解酶,一些酮还原酶(KREDs),以及辅因子再生和在有机溶剂中蛋白质的稳定性研究。
在某些情况下,优化代谢途径;例如,融合不同的自然界菌株的基因于一个新的宿主细胞以产生1,3-丙二醇(形成多聚体的单体),使得将甘油转变为更易被利用的原料葡萄糖成为可能。
由于现在的生物催化浪潮取得的进展,将酶设计成引人瞩目的新功能,例如接受之前的惰性基质(孟鲁司特的KRED或是西他列汀的转氨酶),或是改变形成产物的性质(萜环化酶突变体可作用于不同的萜烯或是氨基酸代谢物使醇类作为生物燃料)。
如今需要新型酶将生物量转换为第二或第三代生物燃料,材料和化学品。
第三大浪潮的主要发展是先进的酶工程(包括定向进化),基因合成,序列分析,生物信息工具和计算机模拟,并且酶改进的理论进展可能比原来预期的要更显著。
工程酶可以在含有60uC的有机溶剂的溶液中保持稳定,可以接受新的底物以及催化新的非天然反应。
目前这一工程可能需要几个月,这样大大扩展潜在的应用。
过去,设计酶化的过程受到酶的限制;目前,酶设计逐渐适应工艺规范。
大约十年前,《Nature》和《Science》的文献综述了第一次和第二次生物催化浪潮,提出了可能带来的第三次浪潮的提示。
现今及时评估第三次浪潮的影响及推测未来十年可能的带来什么进展(框1)。
尽管生物催化涉及到代谢工程和合成生物学,但这些综述重点是针对酶法和全细胞反应。
框1 生物催化应用要求及实例●传统的生物催化,天然产物采用自然反应和途径转变成其他天然产物。
技术要求:尽可能控制自然生物转化;实例:面包和奶酪制作,皮革加工,啤酒和酒发酵,及天然抗生素生成。
●宽底物范围生物催化,化学中间体(非天然产物)通过自然反应和途径转变成其他化学中间体。
技术要求:特定酶的使用(没有干扰活性存在);概念要求:许多酶具有宽的底物范围;实例:采用酯酶和羰基还原酶(乙醇脱氢酶)生产医药中间体。
●多级生物催化,天然产物通过非自然反应和途径转变成燃料,材料和化工原料(非天然产物)。
技术要求:蛋白质工程主要用于稳定性,底物范围和催化反应类型的改变;概念要求:酶可以催化非自然反应,酶的新的组合产生新的途径;实例:用异戊二烯生物合成途径生成燃料分子,氨基酸生物合成燃料乙醇。
适应酶工程的制造工艺为了最大限度降低成本,化工业需要在希望的工艺条件下产生稳定的,选择性的及高产的催化剂。
这样的加工的酶设计先要确定设计目标,例如增加稳定性,可选择性,底物范围,或是通常这些性质的结合。
2000年,第三大浪潮前,只有很少的策略可以满足这些目标。
酶的固定化可以增加蛋白的稳定性,但是稳定性的增加幅度较温和而且往往不能满足大多数化学转化。
定向进化也有可能满足这些目标,但仍然缓慢,因为它需要对大型数据库进行建立和筛选,而且这些数据库中大部分突变体是活性降低甚至没有活性的。
大幅改进的例子很少与产业相关。
低速意味着进化的蛋白仅包含一些变化,因此,酶性质仅稍微得到改变。
尽管几百年来酶法工艺已经应用于工业,但大部分设计的酶和全细胞从遗传学上已被最低限度的改变了。
通过蛋白质工程策略结合自由能(△G)设计目标所需的结构改变这一推理需要利用更集中的数据库。
如果设计目标并非在于开始酶的作用,那么大变化的自由能是必要的。
对蛋白质伸展及反应机理的力学和动力学的理解确定达到设计目标的设计策略。
最终,结构分析(从定性的检测到大量的计算机模拟的差异)可以确定必须改变的区域和氨基酸。
需要高的自由能变化的目标将同样需要结构上更广泛的变化。
过去的十年里,我们对蛋白质和有效地定向进化策略属相的理解都加深,可能酶学性质发生巨大改变。
总的来说,酶工程仍将是通过运用各种解决目前问题可能的方法,对研究成果进行收集,而不是例如用在那些土木,电器,软件或是化学工程的学科的定量的方法。
这些实验研究转化为动力学原理将需要运用自由能与设计目标产物结合成需要的结构变化(图2)。
性质的巨大转变需要自由能的较大转变。
例如,稳定性的明显改变将需要折叠-伸展平衡时更多的自由能变化。
(甚至蛋白质不可逆的伸展起始于一个可逆的部分伸展。
)对蛋白质分子生物学的理解暗示着策略可得到改善。
例如,表面残基促进折叠-伸展平衡,并且在环区增加一个脯氨酸会降低伸展形式的熵。
这些策略代替了随机突变(大部分具有更差的性质)巨大的数据库,而是包含高比例具有活性且潜在的改进的突变体组成的更小的,更集中的蛋白质数据库(图1)。
最后,通过估算各种反应(表面离子对或是增加脯氨酸对熵变的贡献)的强度,研究者可以估算出达到目标所需的变化。
目前很少有研究人员明确的应用基于自由能的方法来计算蛋白质自由能策略,但是将实验研究转变入动力学原理需要一种定量的方法。
新的改进的方法过去的十年里,DNA技术和生物信息学主要进展已经为生物催化领域提供了关键性的支持。
这些工具已经促进了自然资源中新型酶的发现,并且大体上加速了目前生物催化剂的重新设计。
先进的DNA技术新一代的DNA测序技术已可以大规模且相当低成本地进行平行序列的分析。
然而,2002年人类基因组序列分析的成本估计为70,000,000美元,2012年成本已大大降低了1,000倍,低于10,000美元(参考34),Life Technologies 公司,Illumina 公司和Oxford Nanopore Technologies公司已宣布能够在几个小时内对人类全基因组完成测序的测序设备的设计经在2012年晚些推出,这将使每一个基因组的成本降低至少于1,000美元。
不同环境的有机体的全基因组序列,或是环境中的不可培养的有机体(宏基因组)的DNA样本,都已建立了丰富的资源,以供在其中搜索新型生物催化剂[35],而且会继续进行。
运用Illumina技术进行大规模的高通量测序(10,000,000序列读取)也促进了对蛋白质序列-功能关系的探索和了解[36]。
低成本DNA合成已代替基因组DNA的分离成为蛋白质工程的开端。
全基因DNA合成可以进一步为宿主生物体优化密码子,将分子总体结构例如启动子,终止子,增强子,限制性位点等引入到合适的位点。
DNA合成应用传统的亚磷酰胺化学法,但是优化的反应条件已经提高了配对效率,这样增加了聚合物整体质量和数量使得序列可以甚至达到200-250个核苷酸长度。
并行DNA合成应用光刻和喷墨印刷技术进一步降低成本并实现快速合成[37]。
DNA合成也已被用于染色体DNA的整个部分甚至用于代谢途径工程的全基因组的合成[38]。
全基因合成也可被用于合成高质量DNA数据库,范围从小型,集中,饱和位点数据库到大型,综合性的基因库。
自定义的基因甚至基因库正成为类似如今研究实验室使用的试剂和溶剂的商业化化学品。
生物信息学新型工具对实验进展进行补充,生物信息学工具已经成为现代蛋白质工程的一个主要部分[39]。
大的酶家族和同源性搜索的多序列比对中已经确定具有相似催化活性的基因,导致新型的,强有力的生物催化剂[40]。
相同的序列信息可以重建原始的生物催化剂[40],它可能具有更广泛的底物范围和催化多功能性(见下文)。
多序列比对确定每个位点最常见的氨基酸(共有序列)和氨基酸替换以得到功能稳定的酶。
这一数据有助于具有高比例催化活性突变体的小型数据库的设计。
这些数据库已被用于稳定性、催化功能增强及立体选择性改变的生物催化剂的发现[41]。