弦论概述 英语
string theory

e-mail: ashoke.sen@cern.chString theory and Einstein’s dreamAshoke SenHarish-Chandra Research Institute, Chhatnag Road, Jhusi, Allahabad 211 019, IndiaUnification of the theory of gravitation, as given by Einstein’s general theory of relativity, and the theory of electromagnetism, as formulated by Maxwell, had been Einstein’s dream during the later part of his life. String theory, which is the subject of this article, is an attempt to realize this dream. However in many ways string theory attempts to go beyond Einstein’s dream. String theory attempts to bring all known forces of na-ture – not just gravity and electromagnetism – under one umbrella. It also tries to do so in a manner that is con-sistent with the principles of quantum mechanics – the theory that is necessary for describing the laws of nature at very small distance. Thus string theory is an attempt to provide an all-encompassing description of nature that works at large distances where gravity becomes important as well as small distances where quantum mechanics is important.Keywords: Einstein, gravity, quantum mechanics, string theory.I N this article I shall try to give a very general introduction to string theory (Note 1)1–4. However in order to do so, I must begin by reviewing our current understanding of the ba-sic constituents of matter.The world of elementary particlesAccording to our current understanding, everything that we see around us is made of a few elementary building blocks. Figure 1 gives us a bird’s eye view of our current knowledge of the structure of matter. At the crudest level the building blocks of matter are the individual molecules of various compounds. However there are a very large num-ber of compounds, each with its own characteristic mole-cule. A simpler picture emerged when it was realized that each molecule is made of some smaller building blocks known as atoms. There are about 100 different types of atoms and different molecules differ in their properties because they contain different number of atoms of different types in different arrangements. During the early years of the twentieth century it was realized that atoms are also not the smallest constituents of matter – each atom is made of a central nucleus and a set of electrons revolving around it. Different atoms have different number of elec-trons, but all the electrons found in all atoms have identi-cal properties. In contrast the nuclei of different types of atoms have very different properties. This picture simplified once it was realized that each nucleus can be regarded as being made of even smaller constituents – the proton and the neutron. Different nuclei have different properties be-cause they contain different numbers of protons and neu-trons. Finally, even the protons and neutrons are now known to be made of even smaller constituents called quarks – the proton being made of two up (u ) quarks and one down (d ) quarks, and the neutron of one u and two d quarks. According to our current knowledge, the electrons and the quarks cannot be divided any further. We call them elementary particles.This gives us a very simple picture of the structure of matter, namely everything is made of three different types of ‘elementary particles’ – the electron, the u quark and the d quark. However as we shall see, this is far from a complete picture. As is already evident from Figure 1, the up and down quarks each come in three varieties. Here we have denoted them by u 1, u 2, u 3 and d 1, d 2, d 3, but often they are referred to as red, blue and green type of quarks. We shall refer to this as the colour quantum number al-though this has nothing to do with the colour that we see in everyday life. The quarks inside the proton and neutron continuously change their colour due to a process known as strong interaction that will be discussed soon. There are various other reasons why this picture is not complete. I shall review some of them here.Figure 1. Our current understanding of the building blocks of matter.In order to understand the structure of matter, we need to understand not only the basic constituents of matter, but also the nature of the forces that operate between them. Without this knowledge we shall not have any under-standing of what keeps the quarks bound inside a proton and neutron, or at a larger scale, of what keeps the atoms bound inside a molecule. According to our current knowledge there are four basic types of forces operating between elementary particles: (i) gravitational, (ii) electromagnetic, (iii) strong and (iv) weak. Of these the gravitational and the electromagnetic forces are familiar to us from every-day experience. For example the gravitational force is re-sponsible for earth’s gravity and the motion of the planets around the sun. The electromagnetic force is the cause of lightening in the sky, the force of a magnet, working of various electrical appliances, etc. It is also responsible for binding the electrons and the nuclei inside the atom and the atoms inside a molecule. The strong force operates between quarks and is responsible for binding them inside a proton and a neutron and also for binding the proton and the neutron inside a nucleus. The weak force, being weak, is not responsible for binding any particles; however it is responsible for certain radioactive decays known as β-decay. It turns out that in studying the physics of elementary particles, we can ignore the effect of gravitational force. To see this one can compare the electrostatic force between two protons with the gravitational force between two pro-tons at rest. The result is223622/Grav.Force ~10,Elec.Force /N p p G m r e r −=where G N is the Newton’s constant (6.67 × 10–8 cm 3/g s 2) that controls the strength of the gravitational force between two bodies, m p is the proton mass (1.67 × 10–24 g) and e p is the proton charge (4.8 × 10–10 e.s.u.). Clearly this ratio is extremely small. Similarly all other forces can also be shown to be much larger than the gravitational force.So far we have discussed the elementary particles and the forces operating between them as separate entities, but with the help of quantum theory one can give a unified de-scription of elementary particles, and the forces among the elementary particles. Consider for example the elec-tromagnetic force between two electrons when they pass each other. Due to this force, each particle gets deflected from its original trajectory. This has been depicted in Figure 2. In quantum theory, one provides a different ex-planation of the same phenomenon. Here the deflection takes place because the two electrons exchange a new particle, called photon, while passing near each other (see Figure 3). The photon is capable of carrying some amount of energy and momentum from the first electron to the second electron, thereby causing this deflection (Note 2). We call the photon the mediator of electromagnetic force.Even though it mediates electromagnetic force, the photon it-self is electrically neutral.Thus in the language of quantum theory we can describe a force by specifying the particle(s) which mediate the force. It turns out that the strong force is mediated by eight different particles known as gluons. These particles are all electrically neutral. The quarks inside a proton (and neutron) continuously exchange gluons, and in this process keep changing their colour quantum number. On the other hand the weak force is mediated by three parti-cles, denoted by W +, W – and Z . W + and W – carry +1 and –1 unit of electric charge respectively while Z is neutral. (The unit of electric charge is taken to be the charge carried by a single proton. Thus W + has charge equal to that of a proton, while W – has charge that is equal in magnitude but opposite in sign to that of a proton.)Clearly, we must add the gluons, W +, W – and Z , as well as the photon, to our list of elementary particles. We shall refer to these as the mediator particles. Theoretical analysis shows that for every elementary particle there must also be another elementary particle, known as the antiparticle, that carries exactly the same amount of charge but with opposite sign. Thus for every quark and the electron we have the corresponding anti-quark and the anti-electronFigure 2. Classical picture of the deflection of a pair of electrons via electromagnetic force.Figure 3. Quantum picture of the deflection of a pair of electrons via electromagnetic force.(known as the positron). Fortunately the gluons, the photon and the Z particles are their own anti-particles, whereas W– is the anti-particle of W+ and vice-versa. Thus we do not need to expand our list by including anti-particles of the mediator particles. However this still does not exhaust the list of all elementary particles. Besides the u and d quarks, electrons and mediators and their anti-particles, there are also other elementary particles which are produced by cosmic rays, radioactive decays, collision of high energy particles, etc. They must also be added to the list.Our current list contains about 100 types of elementary particles. Thus the situation would not seem any better than the days when atoms were thought to be the basic constituents of matter. The properties of matter known at that time could be explained in terms of the properties of about 100 types of atoms. There is however a difference – unlike the case of atoms, there is a simple mathematical theory that explains the properties of all the elementary particles. In fact this theory has been so successful that it has come to be known as the ‘standard model’ of elementary particles. This model, in principle, can be used to calculate the result of any experiment that we wish to perform in-volving the elementary particles. So far the standard model has been extremely successful in explaining almost all experimental results.The standard model: Its successes and limitations In this section I shall explain some of the basic properties of the standard model. The basic inputs in this theory are • quantum mechanics,• special theory of relativity, and• laws of electromagnetism and their generalization to strong and weak forces.There is a mathematical framework, known as gauge theory, that includes all these three features. I shall not describe the details of this framework here. It turns out that there are many different consistent gauge theories, one of which describes the theory of elementary particles. This particu-lar theory is known as the standard model.Once the theory is written down, it predicts the outcome of every possible experiment involving elementary particles. (Of course some experimental inputs go in to decide on what is the right theory.) For example the standard model tells us precisely what kind of elementary particles we have in our world. According to this model, the elemen-tary particles in our world fall into four categories:• Quarks u1, u2, u3, d1, d2, d3, c1, c2, c3, s1, s2, s3, t1, t2, t3, b1, b2, b3In this list we recognize the familiar up and down quarks, each coming in three colours. It turns out that nature contains four more types of quarks – charm (c),strange (s), top (t) and bottom (b), each coming in three colours. These four types of quarks are not usually found inside matter but can be produced in highly en-ergetic collision among normal matter. Of the six quarks, the up, charm and top quarks carry 2/3 unit of electric charge, whereas the down, strange and bottom quarks carry –1/3 unit of electric charge. For each quark we also have its anti-quark; we have not listed them sepa-rately here.• Leptons e–, νe, µ–, νµ, τ–, ντIn this list we recognize the electron (e–); the – sign on top is to remind ourselves that the electron carries –1 unit of charge, i.e. charge equal in magnitude but opposite in sign to that carried by the proton. νe – known as the electron neutrino – is a weakly interact-ing chargeless particle. These are so weakly interacting that a neutrino passing through the earth does so experi-encing almost no force. The pair of particles (µ–,νµ) have properties similar to that of the pair (e–, νe) al-though the muon (µ–) is a lot heavier that the electron.Similarly the pair (τ–,ντ) have properties similar to that of (e–, νe), with the tau particle (τ–) being even heavier than a muon. For each lepton we also have an anti-lepton which we have not listed here. For example, the anti-particle of the electron is called the positron and denoted by the symbol e+.• Gauge Bosons gluons: g1, ... g8, Photon: γ, W+, W–, ZThese are the by now familiar mediator particles which have been discussed before. As already mentioned the list is complete without having to add the anti-particles separately.• Higgs Particle φThis is the most mysterious particle in the standard model. Unlike every other particle in the list which has been experimentally observed, the Higgs particle has never been seen in any experiment despite several at-tempts. Nevertheless its existence is predicted by the standard model, and new experiments are being de-signed to look for this particle.The standard model not only gives us a list of elemen-tary particles but also the list of processes that can occur involving these particles. For example in order to explain the electromagnetic force between electrons using the process described in Figure 3, it is necessary to know that an electron can emit a photon. This follows from the mathematical framework that lies behind the standard model. The same mathematical framework also tells us that if in this diagram we replace the electron by an electron neutrino then this is not an allowed process in the stan-dard model; hence a neutrino cannot exchange a photon with another particle. Figure 4 shows another example of a process that can occur in the standard model. This de-scribes the decay of a top quark (t1) into an electron neu-trino (νe), a positron (e+) and a bottom quark (b1). In fact the standard model not only tells us which processes canoccur, but it also gives us precise mathematical formula for calculating the probability of occurrence of any such process. These predictions are then compared with ex-perimental data to test the model.Given the success of the standard model, one might like to conclude that we now have a complete understanding of the elementary constituents of matter. This however is not true. There are several reasons why standard model cannot be the complete theory of elementary particles. I shall review a few of these here.First and foremost, the standard model does not explain the origin of one of the important forces that we observe in nature, namely the gravitational force. In particular the list of particles predicted by the standard model does not contain any particle that mediates gravitational force. The effect of this omission of course is not seen in any of the experiments involving elementary particles since, as observed earlier in this article, the gravitational force between two elementary particles is extremely small compared to the other forces. Nevertheless a complete theory must account for every possible tiny effect that exists in nature. Thus a theory that does not provide an explanation of the gravita-tional force cannot be a complete theory of nature.In order to appreciate the gravity of this problem, let us first take stock of what is known about gravity. Our cur-rent theoretical understanding of the gravitational force is based on the ‘general theory of relativity’ – a theory written down by Einstein almost a hundred years ago. This theory has been enormously successful in explaining all effects related to gravity. Unfortunately this theory is based on the principles of classical mechanics and not of quantum mechanics. Since other forces in nature follow the rules of quantum mechanics, any theory that attempts to explain gravity as well as the other forces of nature must treat gravity according to the rules of quantum mechanics. Hence the general theory of relativity, despite being so successful, cannot be the final story about gravity. In fact the reason that this theory has been so successful so far is that for gravity the difference between the predictions of a classical and the quantum theory is extremely tiny andFigure 4. An allowed process in the standard model. cannot be observed in any of the current experiments. (We say that quantum effects involving gravity are extremely small.)Thus the problem at this stage seems to be to first find a quantum theory of gravity and then combine this with the standard model to arrive at a complete theory of all elementary particles and forces operating between them. At the first sight the problem does not seem unsurmount-able. After all, we normally obtain a quantum theory by first writing down a classical theory and then applying a definite set of rules to turn it into a quantum theory. Why can’t the same thing be done with the general theory of relativity? If one proceeds to do this one does get some encouraging results at first. In particular one finds that like other forces, gravity is also mediated by a new kind of elementary particle. This particle has been given the name graviton. Like the diagram in Figure 3 one will have a diagram where two electrons exchange a graviton, repre-senting the (tiny amount of) deflection of one of the elec-trons due to gravitational force of the other electron.So far everything seems to be proceeding as desired. However one soon runs into a problem with this approach. To understand the origin of this difficulty consider the process shown in Figure 5 involving multiple graviton exchanges. As in the case of the standard model, there are precise mathematical rules for computing the probability ampli-tude of this process in the quantum general theory of rela-tivity. When one applies those rules to calculate this probability amplitude, one finds that the result is infinity!This is clearly a nonsensical answer! In actual practice we know that this probability must be extremely tiny since no experiment has yet seen the effect of gravitational force between elementary particles. Thus there must be something wrong with this theory.In order to appreciate how string theory eventually re-solves this problem, it will be useful to investigate in a little more detail the origin of this problem. You would notice that in a diagram like the one shown in Figure 5 there are ‘interaction vertices’ where three (or more) lines meet. For example in Figure 5 there are four such interactionFigure 5. An infinite contribution to the gravitational scattering of two electrons.vertices. These are the points where something happens. We can regard these points as the basic events which make up the complete process. Each such event takes place at a given point in space at a given time, and in order to calculate the total probability amplitude of the process we must integrate over the location of each event in space as well as in time. It turns out that the integrand, calculated using the rules of quantum theory, diverges (becomes in-finite) when more than two or more such elementary events take place at the same point in space at the same time. This in turn causes the integral to diverge occasionally (Note 3).In any case the final outcome of this complicated analysis is that the standard procedure that has been successful in formulating a quantum theory of strong, weak and elec-tromagnetic forces do not work for gravity, and for this reason it is not easy to incorporate gravity into the stan-dard model.Besides the problem of incorporating gravity, the standard model suffers from other conceptual and technical problems. While it is true that the standard model, once formulated, can predict the results of most experiments involving elementary particles, the formulation of the theory itself requires a lot of input from experiments. For example there are many consistent gauge theories, often labelled by several continuous parameters, and standard model corresponds to one of these theories with a specific choice of the values of these parameters. There is no explanation within the theory as to why this particular gauge theory with this particular choice of parameters should describe our universe. Furthermore the choice of parameters which describes the standard model are not generic, but requires very fine tuning. This is evident from the fact that the theory has some extremely small dimensionless numbers like the ratio of gravitational and electromagnetic force between two elementary particles. For a generic choice of parameters this ratio would be of order one. Finally re-cent experiments show that not all predictions of the standard model are completely correct. In particular, ac-cording to the standard model the neutrinos are zero mass particles, but recent experiments show that neutrinos actually have a tiny but finite mass. This requires a small modification of the gauge theory that describes the standard model.These are some of the reasons why we believe that the standard model is not the final story. In the rest of this article we shall try to see how string theory attempts to address some of these issues.String theoryThe basic idea in string theory is quite simple. It says that the elementary constituents of matter are not point-like objects (particles) but one-dimensional objects. These one-dimensional objects, also known as the fundamental (or elementary) strings, have very specific properties which determine the various modes in which the string can vibrate. However to the present day experimentalists, these strings appear as particles since their size is small compared to the distance scale that can be probed by the most power-ful microscopes available today (Note 4). In particular, different vibrational states of a fundamental string appear to us as different elementary ‘particles’ just as the different modes of vibration of a single musical string can produce different harmonics of a note. Thus in string theory in-stead of having different types of elementary particles we have one single type of elementary string as the basic constituent of matter. Figure 6 shows some of the vibra-tional states of strings. As is evident from this figure, strings can come in two varieties – closed strings which have no boundary and open strings which have two end points forming its two boundaries.Since quantum mechanics and special theory of relativity are two of the basic inputs in the standard model, and since string theory must include the standard model if it is to describe our universe, it is natural to require that string theory also respects the principles of quantum mechanics and special theory of relativity. However one finds that for various technical reasons it is not easy to respect these principles. In fact the only way we can respect these prin-ciples is by formulating string theory not in the usual three-dimensional space but in a hypothetical nine-dimen-sional space (Note 5). Furthermore in this nine-dimen-sional space one can formulate altogether five different types of string theory – known as the Type I, Type IIA, Type IIB, E8 × E8 heterotic and SO(32) heterotic string theories. These five string theories differ from each other in the type of vibrations which the string performs. As a result they have different vibrational states, which is reflected in the spectrum of elementary ‘particles’ that each of these theories produce.Having nine space dimensions instead of three seems to be a serious problem. We shall return to this issue shortly and show that this in fact is not a very serious problem. How-ever, let us leave aside this problem for a moment and discuss some of the good things which string theory pro-vides. First of all one finds that one of the vibrational states of string theory have properties identical to that of a graviton – the mediator of gravitational force. Further-Figure 6. Vibrating closed and open strings.more one finds that string theory calculations do not suf-fer from any infinities of the type we encounter while try-ing to directly quantize general theory of relativity. Thus string theory provides us with a finite quantum theory of gravity!It is instructive to try to understand why the probability amplitudes calculated in string theory are finite. For this we need to look at the Figure 7 describing the process of scattering of two strings. Like in the case of point particle theories, there are definite mathematical rules for calcu-lating the probability amplitude of this process. The point to note is that in this diagram there are no points where specific events (like splitting of a single string into a pair of strings) take place; the diagram is completely smooth everywhere. As a result the divergences in the point particle theories – which arise when two or more such events take place at the same point at the same time – are absent in string theory. This is the intuitive reason why string am-plitudes are finite.At this point we must mention that the graviton is only one of the many vibrational states of an elementary string. In fact the laws of quantum mechanics tells us that a single elementary string has infinite number of vibra-tional states. Since each such vibrational state behaves as a particular type of elementary particle, string theory seems to contain infinite types of elementary particles. This would be in contradiction with what we observe in nature were it not for the fact that most of these elementary particles in string theory turn out to be very heavy, and not observable in present experiments. Thus there is no immediate conflict between what string theory predicts and what we observe in actual experiments. On the other hand these additional heavy elementary particles are abso-lutely essential for getting finite answers in string theory.Let us now return to the issue about the dimension of space-time. Consistency of string theory demands that we can formulate the theory only in 9 space dimensions. How can string theory be relevant for describing nature, which seems to have only 3 space dimension? The an-swer to this question is provided by an old idea known asFigure 7. A process describing a pair of strings scattering from each other. compactification. This idea was pioneered by Kaluza and Klein during the first half of the twentieth century and Einstein himself had been attracted by this idea. We shall illustrate the basic idea by a simple example in which we begin with a world with two space dimensions instead of nine space dimensions. We take the two space coordinates to describe the surface of a cylinder of radius R instead of an infinite plane as shown in Figure 8. All objects (in-cluding light) in this world can move only along the sur-face of the cylinder. Thus if we move along the vertical direction in the figure, then after travelling a certain dis-tance (2πR where R is the radius of the cylinder) we shall traverse the whole circumference of the circle and come back to the original point where we started. We call this a compact direction. In contrast an object can travel along the horizontal direction without ever returning to its origi-nal position and we call this the non-compact direction.Clearly if R is very large (larger than the range of the most powerful telescope) then the two-dimensional space will appear to be infinite in both directions and we would not know that one of the directions is compact. If R is within the visible range, then the two-dimensional crea-tures will start seeing infinite number of images of each object separated by an interval of 2πR since light from any object can reach an observer in many (infinite number of) ways – directly, travelling once around the circumfer-ence, travelling twice around the circumference, etc. This may seem strange from our point of view but will not at all seem strange from the point of view of the two-dimen-sional people living in this world since they would al-ways see their world this way. But now consider the case when R is very small, as shown in Figure 9. Clearly this world looks one dimensional as R→ 0. In fact as long as R is smaller than the resolution of the most powerful micro-scope, the two dimensional people will never know that they have a hidden dimension in their world. To them the world will appear to be one-dimensional.This illustrates the way a universe with a certain number of space dimensions can ‘appear to be’ a universe with less number of dimensions. This idea can be generalized toFigure 8. A two-dimensional space with a compact coordinate.Figure 9. A two-dimensional space with a small compact coordinate.。
Krashen's theory of SLA

2.1 IntroductionStephen Krashen (University of Southern California) is an expert in the field of linguistics, specializing intheories of language acquisition and development. Much of his recent research has involved the study ofnon-English and bilingual language acquisition. During the past 20 years, he has published well over 100books and articles and has been invited to deliver over 300 lectures at universities throughout the UnitedStates and Canada. This is a brief description of Krashen's widely known and well accepted theory ofsecond language acquisition, which has had a large impact in all areas of second language research andteaching since the 1980s. Krashen's theory of second language acquisition consists of five mainhypotheses:l the Acquisition-Learning hypothesis,l the Monitor hypothesis,l the Natural Order hypothesis,l the Input hypothesis,l and the Affective Filter hypothesis.1). The Acquisition-Learning HypothesisKrashen (1982) has a rgued that “Learning cannot become acquisition and that fluency in a second orforeign language is due to what learners have acquired, not what they have learned (Brown, 2000:278).Acquisition, according to Krashen, is a subconscious process whereas learning is an active and consciousprocess involving the memorization of many formal linguistic rules. Krashen believed that second languagelearners should attempt to acquire linguistic rules subconsciously and in a natural way much like a childacquires his or her L1.The Acquisition-Learning distinction is the most fundamental of all the hypotheses in Krashen's theory andthe most widely known among linguists and language practitioners.According to Krashen there are two independent systems of second language performance: 'the acquiredsystem' and 'the learned system'. The 'acquired system' or 'acquisition' is the product of a subconsciousprocess very similar to the process children undergo when they acquire their first language. It requiresmeaningful interaction in the target language - natural communication - in which speakers areconcentrated not in the form of their utterances, but in the communicative act.The 'learned system' or 'learning' is the product of formal instruction and it comprises a conscious processwhich results in conscious knowledge 'about' the language, for example knowledge of grammar rules.According to Krashen 'learning' is less important than 'acquisition'.2). the Monitor HypothesisThe Monitor hypothesis explains the relationship between acquisition and learning and defines theinfluence of the latter on the former. The monitoring function is the practical result of the learned grammar. According to Krashen, the acquisition system is the utterance initiator, while the learning system performs therole of the 'monitor' or the 'editor'. The 'monitor' acts in a planning, editing and correcting function whenthree specific conditions are met: that is, the second language learner has sufficient time at his/her disposal, he/she focuses on form or thinks about correctness, and he/she knows the rule.It appears that the role of conscious learning is somewhat limited in second language performance.According to Krashen, the role of the monitor is - or should be - minor, being used only to correctdeviations from 'normal' speech and to give speech a more 'polished' appearance.Krashen also suggests that there is individual variation among language learners with regard to 'monitor'use. He distinguishes those learners that use the 'monitor' all the time (over-users); those learners whohave not learned or who prefer not to use their conscious knowledge (under-users); and those learnersthat use the 'monitor' appropriately (optimal users). An evaluation of the person's psychological profile canhelp to determine to what group they belong. Usually extroverts areunder-users, while introverts andperfectionists are over-users. Lack of self-confidence is frequently related to the over-use of the 'monitor'.3).The Natural Order Hypothesis:Kr ashen’s Natural Order Hypothesis states that second language learners “acquire the rules of alanguage in a predictable sequence” (Lightbown and Spada,1993:27-28). The Natural Order Hypothesisalso states that even though some of the rules in a language are easy for the learner to memorize, theserules or often most difficult for the learner to acquire. Krashen’s view regarding the natural acquisition ofcertain structures has been supported in morpheme studies and it is from these studies that Krashenattempts to bolster support for his Natural Order Hypothesis.The Natural Order hypothesis is based on research findings (Dulay & Burt, 1974; Fathman, 1975; Makino,1980 cited in Krashen, 1987) which suggested that the acquisition of grammatical structures follows a'natural order' which is predictable. For a given language, some grammatical structures tend to be acquired early while others late. This order seemed to be independent of the learners' age, L1 background, conditions of exposure, and although the agreement between individual acquirers was not always 100% in the studies, therewere statistically significant similarities that reinforced the existence of a Natural Order of languageacquisition. Krashen however points out that the implication of the natural order hypothesis is not that alanguage program syllabus should be based on the order found in the studies. In fact, he rejectsgrammatical sequencing when the goal is language acquisition.4).The Input HypothesisThis is perhaps the most important of Krashen’s five hypotheses. The thrust of the input hypothesis isthat in order for language acquisition to take place, the acquirer must receive comprehensible inputthrough reading or hearing language structures that slightly exceed their current ability (Brown, 2000:278). However, it is important that the acquirer not receive input that exceeds his or her level of competence. It iscrucial that the acquirer receive input that is comprehensible and challenging enough to lead toimprovements in linguistic competence. The input hypothesis has been outlined by Krashen as follows:The input hypothesis makes the following claim: a necessary (but not sufficient) condition to move fromstage i + l is that the acquirer understand input that contains i+ l, where “understand” means that theacquirer is focused on the meaning and not the form of the message (Krashen,1982: 21). Furthermore, it is essential not to focus on explicit grammatical structures or learning activities but rather to occupy classroom time with ac quisition tasks or activities. Krashen argues, “The input hypothesis relates to acquisition, notlearning” (Krashen, 1982:21). In this regard, many EFL/ESL instructors can better serve their studentsSL learning needs by introducing various tasks in the classroom. The input hypothesis also states that anacquirer must not be forced to speak too early. That is, a certain amount of comprehensible input must bebuilt up before the acquirer is required to speak in a classroom (Brown, 2000:278). According to Krashen,many second language learners will go through what is referred to by some as a silent period when learners gradually receive and build enough comprehensible input so that they can start to produce their own structures.The Input hypothesis is Krashen's attempt to explain how the learner acquires a second language. In other words, this hypothesis is Krashen's explanation of how second language acquisition takes place. So, the Input hypothesis is only concerned with 'acquisition', not 'learning'. According to this hypothesis, the learnerimproves and progresses along the 'natural order' when he/she receives second language 'input' that isone step beyond his/her current stage of linguistic competence. For example, if a learner is at a stage 'i',then acquisition takes place when he/she is exposed to 'Comprehensible Input' that belongs to level 'i + 1'.Since not all of the learners can be at the same level of linguistic competence at the same time, Krashen suggests that natural communicative input is the key to designing a syllabus, ensuring in this way that each learner will receive some 'i + 1' input that is appropriate for his/her current stage of linguistic competence.5). The Affective Filter HypothesisFinally, the fifth hypothesis, the Affective Filter hypothesis, embodies Krashen's view that a number of 'affective variables' play a facilitative, but non-causal, role in second language acquisition. These variablesinclude: motivation, self-confidence and anxiety. Krashen claims that learners with high motivation,self-confidence, a good self-image, and a low level of anxiety are better equipped for success in second language acquisition. Low motivation, low self-esteem, and debilitating anxiety can combine to 'raise' theaffective filter and form a 'mental block' that prevents comprehensible input from being used for acquisition.In other words, when the filter is 'up' it impedes language acquisition. On the other hand, positive affect isnecessary, but not sufficient on its own, for acquisition to take place.Comprehensible input will occur during the language acquisition process when that acquirer’s affectivefilter is down or low. The Affective Filter Hypothesis states that:A learner who is tense, angry, anxious, or bored will screen out input, making it unavailable for acquisition.Thus, depending on the learner’s state of mind or disposition, the filter limits what is noticed and what isacquired. The filter will be up or operating when the learner is stressed,self-conscious or unmotivated. Itwill be down when the learner is relaxed or motivated (Lightbown and Spada, 1993: 28).Again, as previously stated, this hypothesis has found a lot of support from those within the EFL/ESL fieldas it makes perfectly good practical sense for instructors to try and create a low-stress, anxiety free andrelaxing language learning environment for those people attempting to acquire a second language. ManyEFL/ESL instructors truly believe that students learn better in environments that are free from stress orwhere they feel uncomfortable.2.2 STRENGTHSEven though Krashen’s innatist model of SLA has received an abundance of criticism, it has been popular with many people teaching in the EFL/ESL fields. The input hypothesis seems to be simple for second language instructors to follow and teachers are able to base some of their teaching methodology on Krashen’s fivehypotheses (Brown, 2000:281).First, Krashen argues that grammar should not be taught in the classroom and that the formal rules orstructures of the language are not necessary to aid in comprehensible input.Krashen has also been attacked on this point to which he states that we use our knowledge of the world as well as context and other extra linguistic devices to aid comprehension and can subconsciously acquire the formal structures through this process:…our assumption has been that we first learn structures then practice using them in communication, andthis is how fluency develops. The input hypothesis says the opposite. It says we acquire by “goin g formeaning” first, and as a result, we acquire structure (Krashen, 1982:21).The writer agrees with Krashen in that learners are better served if instructors can provide learners withopportunities with which to practice the grammar that they have acquired in the classroom. It is alsoimportant for instructors to encourage learners to use context and their knowledge of the world to help learners with comprehension, new vocabulary and grammar.Krashen also advocates the use of communicative tasks in the classroom, with which many professionallanguage teachers also agree. However, at times students, especially adult students who are generallymore cognitively advanced than child learners, can benefit from more explicit grammatical explanations and direct vocabulary definitions.Krashen further argues that it is important for language learners to be exposed to structures that areslightly above their level of ability. Krashen feels that in order for L2 acquisition to occur and for learners toimprove their L2 competence, learners must attempt to understand language that slightly exceeds theircurrent ability:We acquire by understanding language that contains structure a bit beyond our current level ofcompetence (i + l). This is done with the help of context or extra-linguistic information (Krashen, 1982:21).EFL students in Taiwan have studied English grammar extensively. However, it is interesting to note thatmost of these students make routine mistakes on structures which they know the rules for when speaking.Chinese students will, during classroom conversation, regularly omit the s that must be attached to thethird – person singular form of verbs. The students indeed know the rules governing the third – personsingular, as they have studied English grammar ad nauseam in high school.Unfortunately, most of these learners are not able to apply the rules during conversation. Mistakes include, “He live with his sister” or “She go to work at 8 o’clock.” Lightbown (Brown, 2000: 275) states that,“Knowing a language rule does not mean one will be able to use it in communicative interaction.”Chinese students have learned and know many of the formal grammatical rules; however, they have nottruly acquired the structures.These errors may also represent the fossilization phenomenon that occurs in most L2 learners. Thisoccurs during adult L2 acquisition in which the linguistic competence of the L2 learners does not progresswith respect to particular language structures.Lightbown similarly claims that, “For mos t adult learners, acquisition stops –“fossilizes” – before thelearner has achieved native-like mastery of the target language (Brown, 2002:275).2.3 CRITICISMSWhile Krashen’s input hypothesis has received quite a bit of support from practitioners within the EFL field and contributed towards EFL/ESL classroom teaching methodologies, his theoretical views have received some harsh criticisms.For instance, McLaughlin, a psychologist, has attacked Krashen’s Monitor Model by arguing, “Krashenhas not produced any evidence in support of his claims but has simply argued that ‘certain phenomenacan be viewed from the perspective of his theory (Mangubhai, 2003:4.9)”.Similarly, Lightbown and Spada (1998:29) state that, “…the theory has also been seriously criticized forfailing to meet certain minimum standards necessary in scientific research and writing.”Firstly, the Monitor Hypothesis has been attacked primarily because there is no clear evidence to show us ‘monitor’ use (Lightbown and Spada, 1993:27).Scholars have not been able to determine language that has been produced by the learned system and the acquired system.Krashen’s claim that learning does not lead to acquisition is problematic for many scholars as this impliesthat spontaneous language has been acquired and not learned. As a result, Krashen has been accused of providing a circular definition of the terms learned and acquired systems (Lightbown and Spada, 1993:27).Secondly, Krashen has not produced any clear, empirical evidence that more output, written or oral, resultsin more language acquisition. This challenges Krashen’s silent period assertion in which Krashen arguesthat SLA acquirers go through a period of acquiring sufficient comprehensible input in order to finallyproduce intelligible SL utterances.Thirdly, according to Gregg (1984), we need clearer definitions of acquisition/subconscious andlearning/conscious (Brown, 2000: 279). Gregg argues that Krashen has used these terms with a certainsense of recklessness and has argued that it is very difficult to distinguish between what is conscious andwhat is unconscious:Krashen plays fast and loose with his definitions…If unconscious knowledge is capable of being brought toconsciousness, and if conscious knowledge is capable of becoming unconscious – and this seems to be areasonable assumption – then there is no reason whatever to accept Krashen’s claim, in the absence of evidence. And there is an absence of evidence (Brown, 2000:280).Another aspect of Krashen’s input Hypotheses is that h e claims that the use of care – taker talk or foreigner – talk (FT) is beneficial to the learner as it aids comprehension.…foreigner – talk and teacher – talk are not made for the purpose of language teaching, but are madefor the purpose of communication, to help the second language acquirer understand what is being said(Krashen, 1982:25).Many EFL schools view foreigner – talk as ungrammatical and are concerned that students may complainif teachers speak to students using such modified speech. According to Meisel (1980):“Ungrammatical FT is generally felt to imply a lack of respect… However, it may not be the presence ofungrammatical modifications per se that arouse negative responses in learners, but their awareness ofbeing addressed in a sp ecial manner” (cited in Ellis, 2002: 254).Grammatical FT, on the other hand, has been seen as useful in supplying simple input (Ellis, 2002: 254).At any rate, it is widely believed that SL learners need exposure to a broad range of input so that they canincrease their communicative competence.Krashen argues that explicit error-correction has very little or no effect at all upon learner's long-termlinguistic competence. Lightbown (1985) has stated that, “Isolated explicit error correction is usuallyin effective in changing language behavior” (Brown, 2000:276). As Lightbown and Spada (1993:115) point out, “Excessive error correction can have a strong negative effect on motivation.”Finally, there is also some concern that input does not last long enough to promote the development ofconfidence in the acquirer and, as well, we have not seen any evidence indicating as to what the acquirerdoes with the input (Mangubhai, 2003:4.9).In addition, Krashen’s concept of i + l has been attacked as a result of the fact that scholars do not have a very clear understanding of what the various learning stages are in second language acquisition.2.4 The Role of Grammar in Krashen's ViewAccording to Krashen, the study of the structure of the language can have general educational advantages and values that high schools and colleges may want to include in their language programs. It should be clear,however, that examining irregularity, formulating rules and teaching complex facts about the targetlanguage is not language teaching, but rather is "language appreciation" or linguistics.The only instance in which the teaching of grammar can result in language acquisition (and proficiency) iswhen the students are interested in the subject and the target language is used as a medium of instruction. Very often, when this occurs, both teachers and students are convinced that the study of formal grammar isessential for second language acquisition, and the teacher is skillful enough to present explanations in the target language so that the students understand. In other words, the teacher talk meets the requirements forcomprehensible input and perhaps with the students' participation the classroom becomes an environmentsuitable for acquisition. Also, the filter is low in regard to the language of explanation, as the students'conscious efforts are usually on the subject matter, on what is being talked about, and not the medium.This is a subtle point. In effect, both teachers and students are deceiving themselves. They believe that it is the subject matter itself, the study of grammar, that is responsible for the students' progress, but in reality theirprogress is coming from the medium and not the message. Any subject matter that held their interest would do just as well.2.5 SUMMARYKrashen's input hypothesis holds quite a bit of appeal among EFL instructors and has stressed theimportance of communicative tasks in the SLA classroom. As well, the input hypothesis is very easy tounderstand and to apply within the SL classroom.For instance, according to the Affective Filter Hypothesis, SL acquirers are able to make better use of inputif their affective filters are low. EFL students who are relaxed and able to learn English in a stress – freeenvironment will be able to learn better and enjoy their second language learning experience much more.Many EFL/ESL language instructors have seen the merit of the affective-filter hypothesis and strive tomake their classrooms more relaxed for second language learners and to make the language lessons more enjoyable for learners in attempts to reduce the levels of anxiety among students.This aspect of Krashen’s theory, not surprisingly, has received support even from Krashen’s critics. We have also seen through Krashen’s theory that many EFL programs over emphasize the importance ofgrammar and do not utilize enough communicative tasks in order to develop the learners competence.Also, the input hypothesis is simple – perhaps too simple. The simplistic nature of Krashen’s inputhypothesis has led to a fair amount of criticism for not being scientific enough.As mentioned previously, many scholars demand more evidence from Krashen in order to support thevarious claims that he makes under the five central hypotheses which constitute his input hypothesis.For example, Krashen’s definition of the terms acquisition and learning is not satisfactory. Overall,however, the input hypothesis is an interesting and relevant SLA theoretical perspective that has had animpact on SLA learning and EFL/ESL teaching practices.。
弦论与宇宙学

弦论与宇宙学弦论(String Theory)是近年来物理学领域的一项重要研究课题。
它被认为是统一量子力学和爱因斯坦的广义相对论的理论框架。
而宇宙学(Cosmology)则是研究整个宇宙的起源、演化和结构等问题的学科。
本文将探讨弦论与宇宙学之间的关系以及对宇宙起源和演化的启示。
一、弦论基础弦论是一种基本粒子描述的理论,将基本粒子看作是一维的弦状结构,而不再是零维点状结构。
这样一来,物质和力场都可以通过弦的振动模式来描述。
弦论不仅包含了量子力学的规则,还包含了引力的效应,因此具备统一量子力学和引力理论的潜力。
二、弦论与宇宙起源弦论对于解释宇宙的起源提供了一种可能性。
宇宙学家普遍接受的宇宙起源理论是大爆炸理论,即宇宙在一个初始的高温高密度点上产生,然后逐渐膨胀。
然而,大爆炸之前的宇宙是什么样子的呢?弦论提出了一个有趣的观点,即在大爆炸之前宇宙是一个高度弯曲的时空,弦理论则给出了描绘这样一个宇宙的方法。
三、宇宙学观测与弦论验证弦论的一个重要特征是它预测了一些现象,这些现象在目前的宇宙学观测中还没有被观测到。
然而,科学家们认为这些现象可能在未来的观测中被证实,从而验证弦论的有效性。
例如,弦论预测了额外的维度存在,这些维度的大小可能相当微小,因此很难直接观测到。
但是,一些宇宙学实验可能会通过探测引力微弱的效应来间接证实这些额外维度的存在。
四、弦论与黑洞黑洞是宇宙中的一种极端天体,强大的引力使得物质被压缩到极限,形成一个密度无穷大、引力无法逃脱的区域。
弦论对于黑洞的研究也取得了重要的进展。
根据弦论的描述,黑洞并不是完全无法逃离的,而是通过黑洞边界的一些量子效应,信息可能得以逃离黑洞,这与传统的黑洞观点有所不同。
五、宇宙学的开放问题和弦论的应用宇宙学仍然存在许多未解之谜,如宇宙膨胀加速的原因、宇宙中的暗物质和暗能量是什么等。
弦论具有潜在解决这些问题的能力。
例如,弦论中的超弦可以被认为是暗物质的候选者之一,因为它们与普通物质相互作用很弱,难以被观测到。
弦论揭秘:自学向导 网上预览
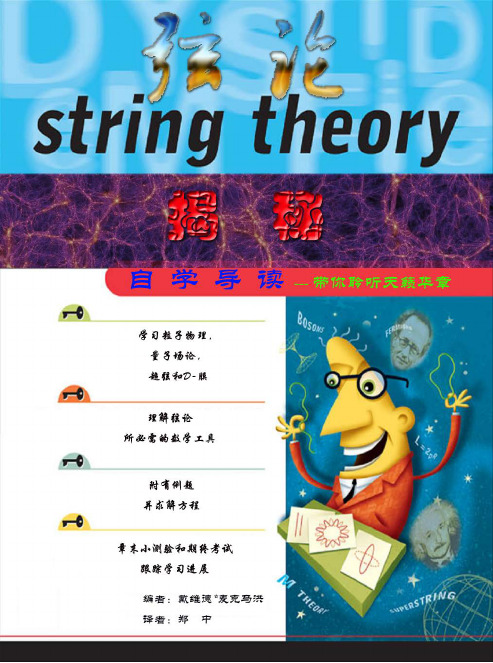
作者简介
戴维德 • 麦克马洪是当代著名的美国物理学家。作为物理研究人员,他曾在圣迪亚 (SaБайду номын сангаасdia) 国家实验室工作过七年。 他是 《线性代数揭秘》 、 《量子力学揭秘》 、 《相对论揭秘》 、 《MATLAB®揭秘》和《复变函数揭秘》等自学导读书籍的作者。
译者简介
郑中,生于 1979 年,四川隆昌人,理学硕士、工程师。本科就读于成都理工大学资源 经济系,后考入中国科学院地球化学研究所,毕业后在矿业公司和资源规划院工作过,现仍 主要从事地质矿产工作。 译者博客:/u/warlong /cqzg
II
目 录
译者序言 作者前言 第一章 导 论(Introduction) 广义相对论快览(A Quick Overview of General Relativity) 量子论快速入门(A Quick Primer on Quantum Theory) 标准模型(The Standard Model) 量子引力场(Quantizing the Gravitational Field) 弦论中一些基本分析(Some Basic Analysis in String Theory) 统一与基本常数(Unification and Fundamental Constants) 弦论概览(String Theory Overview) 弦论类型(Types of String Theories) M论 D膜 高维 小 结 小测验 第二章 经典弦I:运动方程(The Classical String I: Equations of Motion) 相对论性点粒子(The Relativistic Point Particle) 时空中的弦(Strings in Space-Time) 弦的运动方程(Equations of Motion for the String) 波里雅科夫作用量(The Polyakov Action) 数学旁白:欧拉示性数(Mathematical Aside: The Euler Characteristic) 光锥坐标(Light-Cone Coordinates) 波动方程解(Solutions of the Wave Equation) 具有自由端点的开弦(Open Strings with Free Endpoints) 闭弦(Closed String) 具有固定端点的开弦(Open Strings with Fixed Endpoints) 泊松括号公式(Poisson Brackets) 小测验 第三章 经典弦II:对称性与世界面流(The Classical String II: Symmetries and Worldsheet Currents) 能动张量(The Energy-Momentum Tensor) 波里雅科夫作用量的对称性(Symmetries of the Polyakov Action) 庞加莱变换(Poincaré transformations) 再参数化(reparameterization) 外尔变换(Weyl transformation) 转换为平直世界面度规(Transforming to a Flat Worldsheet Metric) 源于庞加莱不变性的守恒流(Conserved Currents from Poincaré Invariance) 哈密顿公式(The Hamiltonian) 小 结 小测验
常用物理英语词汇(全)
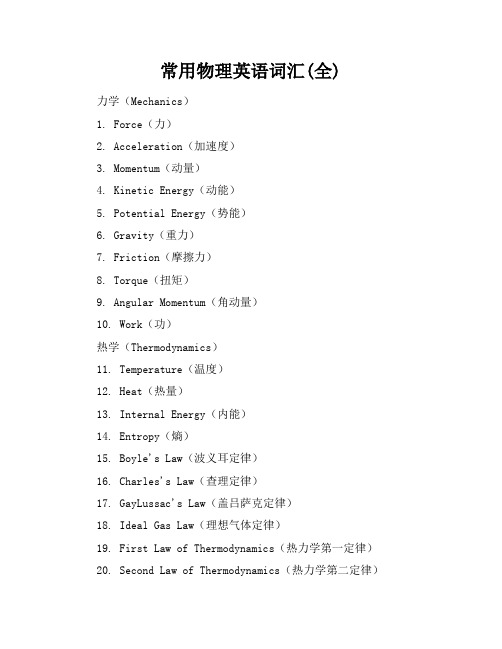
常用物理英语词汇(全)力学(Mechanics)1. Force(力)2. Acceleration(加速度)3. Momentum(动量)4. Kinetic Energy(动能)5. Potential Energy(势能)6. Gravity(重力)7. Friction(摩擦力)8. Torque(扭矩)9. Angular Momentum(角动量)10. Work(功)热学(Thermodynamics)11. Temperature(温度)12. Heat(热量)13. Internal Energy(内能)14. Entropy(熵)15. Boyle's Law(波义耳定律)16. Charles's Law(查理定律)17. GayLussac's Law(盖吕萨克定律)18. Ideal Gas Law(理想气体定律)19. First Law of Thermodynamics(热力学第一定律)20. Second Law of Thermodynamics(热力学第二定律)电磁学(Electromagnetism)21. Electric Charge(电荷)22. Electric Field(电场)23. Magnetic Field(磁场)24. Current(电流)25. Voltage(电压)26. Resistance(电阻)27. Capacitance(电容)28. Inductance(电感)29. Ohm's Law(欧姆定律)30. Ampère's Law(安培定律)光学(Optics)31. Light(光)32. Refraction(折射)33. Reflection(反射)34. Diffraction(衍射)35. Interference(干涉)36. Polarization(偏振)37. Lens(透镜)38. Prism(棱镜)39. Spectrum(光谱)40. Wave Optics(波动光学)现代物理(Modern Physics)41. Quantum Mechanics(量子力学)42. Relativity(相对论)43. Photon(光子)44. Electron(电子)45. Proton(质子)46. Neutron(中子)47. Quark(夸克)48. Black Hole(黑洞)49. Higgs Boson(希格斯玻色子)50. String Theory(弦理论)这些词汇仅为物理学中常用术语的一小部分。
物理专业英语——弦理论
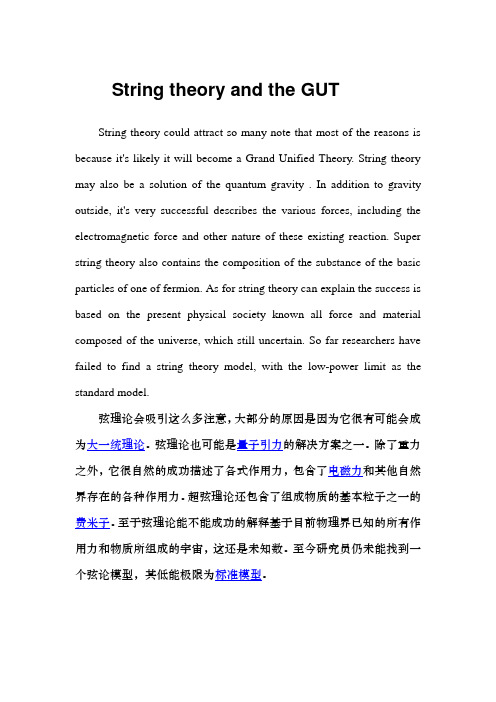
String theory and the GUTString theory could attract so many note that most of the reasons is because it's likely it will become a Grand Unified Theory. String theory may also be a solution of the quantum gravity . In addition to gravity outside, it's very successful describes the various forces, including the electromagnetic force and other nature of these existing reaction. Super string theory also contains the composition of the substance of the basic particles of one of fermion. As for string theory can explain the success is based on the present physical society known all force and material composed of the universe, which still uncertain. So far researchers have failed to find a string theory model, with the low-power limit as the standard model.弦理论会吸引这么多注意,大部分的原因是因为它很有可能会成为大一统理论。
弦理论也可能是量子引力的解决方案之一。
英语文学常识
1. American Romanticism
(1815-1865) Washington Irving (美国文学之父)
The Sketch Book
Rip Van Winkle The Legend of Sleepy Hollow
Hawthorne (象征隐喻手法)
The Scarlet Letter The Birthmark
The Adventure of Tom Sawyer The Adventure of Huckleberry
Finn
Henry James (“美国小说泰斗”) Daisy Miller The Portrait of a Lady
Theodore Dreiser Sister Carrie
Charlotte Bronte Jane Eyre Emily Bronte Wuthering Heights
Thomas Hardy Far from the Madding Crowd Tess of the d’ Urbervilles Jude the Obscure
6. The Modern Period (1901-1980)
2. The Renaissance Period
(1485-1660) (1) 第一时期
Thomas More
(2) “伊丽莎白时代”
Spenser (the poet’s poet) Shepherd’s Calendar
The Faerie Queen
Shakespeare great comedies great tragedies
F. Scott Fitzgerald
The Great Gatsby The Side of Paradise
英语写作论证例子
写作例子科技发展对人类的影响:The place of technology in modern society is a subject of continuing controversy. Key issue includes not only the impact of technology on quotidian life but also the need to control the development and uses of technological innovations so that they benefit all aspects of community.正面:Many technological developments during the last century have helped reduce human suffering. Consider, for instance, technology that enables computers to map Earth’s geographical features from outer space. This technology allows us to locate lands that can be cultivated for feeding malnourished people in third-world countries. And, few would disagree that humanity is the beneficiary of the myriad of 20th century innovations in medicine and medical technology—from prostheses and organ transplants to vaccines and lasers.20th century technological innovation has enhanced the overall standard of comfort level of developed nations. The advent of steel production and assembly-line manufacturing created countless jobs, stimulated economic growth, and supplied a plethora of innovative conveniences. More recently, computers have helped free up out time by performing repetitive tasks: have aided in in the design of safer and more attractive bridges, buildings, and vehicles; and have made possible universal access to information.反面:For every new technological breakthrough that helps reduce human suffering is another that serves primarily to add to that suffering.For example, while some might argue that physics researchers who harnessed the power of the atom have provided us with an alternative source of energy and invaluable “peace-keepers”, this argument flies in the face of hundreds of thousands of innocent people murdered and maimed by atomic blasts, and by nuclear meltdowns. And in fulfilling the promise of “better living through chemistry” research has given us chemical weapons for human slaughter. In short, so-called “advance”that scientific research has brought about often amount to net losses for humanity.Technological development has already brought about environmental problems. Many things these days cause pollution such as cars, air conditioners, refrigerators, factories, etc. All these things together can cause a pollution problem in the society today. Refrigerators and air conditioners are a problem to the zone because of the liquid called Freon--when these liquids are released from damaging the machine, it releases a gas that breaks down the zone layers. In addition, cars also can cause a lot of pollution because of the carbon monoxide it produces. This would cause the air to be bad, cause global warming, and would also cause acid rain.哥白尼日心说:(opposite to “Earth-centric Theory”)Copernicus' theory of heliocentricism is sure to be a great achievement inastronomy, however, his great discovery contradicted the traditional and in-powered theological canon which dictated geocentricism , his book was censored and prohibited from the general public, it was not until several years published in other countries did Copernicus first express his ideas to populace. 利用点:兴趣爱好与成功;质疑权威社会才会前进和发展;真理一开始都是令人难以容忍的,或者至少是令人不快的,而且都是在反对当时的权威思想。
StringTheory弦理论
StringTheory弦理论弦理论,是理论物理的一个分支学科,弦论的一个基本观点是,自然界的基本单元不是电子、光子、中微子和夸克之类的点状粒子,而是很小很小的线状的“弦”(包括有端点的“开弦”和圈状的“闭弦”或闭合弦)。
弦的不同振动和运动就产生出各种不同的基本粒子,能量与物质是可以转化的,故弦理论并非证明物质不存在。
弦论中的弦尺度非常小,操控它们性质的基本原理预言,存在着几种尺度较大的薄膜状物体,后者被简称为“膜”。
直观的说,我们所处的宇宙空间可能是9+1维时空中的D3膜。
弦论是现在最有希望将自然界的基本粒子和四种相互作用力统一起来的理论。
弦理论是一门理论物理学上的学说。
理论里的物理模型认为组成所有物质的最基本单位是一小段“能量弦线”,大至星际银河,小至电子,质子,夸克一类的基本粒子都是由这占有二维时空的“能量线”所组成。
中文的翻译上,一般是译作“弦”。
超弦理论可以解决和黑洞相关的问题。
在弦理论中,基本对象不是占据空间单独一点的基本粒子,而是一维的弦。
这些弦可以有端点,或者他们可以自己连接成一个闭合圈环。
正如小提琴上的弦,弦理论中支持一定的振荡模式,或者共振频率,其波长准确地配合。
编辑弦理论(以及它的升级版超弦理论)认为所有的亚原子粒子都并非是小点,而是类似于橡皮筋的弦 [1] 。
与粒子类型的唯一区别在于弦振动的频率差异 [1] 。
弦理论主要试图解决表面上的不兼容的两个主要物理学理论——量子力学和广义相对论——并欲创造的描述整个宇宙的“万物理论” [1] 。
然而这项理论非常难测试,并需要对我们所描绘的宇宙进行一些调整,也即宇宙一定存在比我们所知的四维空间更多的时空维度 [1] 。
科学家认为这些隐藏的维度可能卷起到非常小以至于我们没有发现它们 [1] 。
模型建立较早时期所建立的粒子学说则是认为所弦理论有物质是由只占一度空间的“点”状粒子所组成,也是目前广为接受的物理模型,也很成功的解释和预测相当多的物理现象和问题,是此理论所根据的“粒子模型”却遇到一些无法解释的问题。
string theory(超弦论)
Thank you for staring at the screen
azicon corperation
EINSTEIN PODOLSKY ROSEN
back to the topic
• In physics, string theory is a theoretical framework in which the point-like particles of particle physics are replaced by multidimensional objects called strings.
string theory: the ultimte theory
azicon coperation
how is string theory developed
• For decades, scientists are trying desperately to come out with a ultimate theory, a theory that can explain everything, from atoms to galaxies. At first, the father of physics, Isaac Newton constructed the logic of motion and the laws of physics. He claimed that everything is predictable, and already set, and unchangeable. And space is like a stage, and events played out like plays, and time’s flowing is unchangeable and remains static and flows at a same rate for everyone.
- 1、下载文档前请自行甄别文档内容的完整性,平台不提供额外的编辑、内容补充、找答案等附加服务。
- 2、"仅部分预览"的文档,不可在线预览部分如存在完整性等问题,可反馈申请退款(可完整预览的文档不适用该条件!)。
- 3、如文档侵犯您的权益,请联系客服反馈,我们会尽快为您处理(人工客服工作时间:9:00-18:30)。
concept
history
significance
nowdays
GMm f R2
GMm f R2
We all kmow this function,but the truth is that :If the distance was extremly short,the force become infinity.
2014-1-4
Hale Waihona Puke concepthistory
significance
nowdays
Fermi national labortory did a experiment and they found an unexplained phenomenon which made the scientist guess thepossibility of another kind of neutrino which could prove the string theory is right.
concept history significance nowdays
Many scientist hold the opinion that string theory was the only way to handle the conflicts between quantum mechanics and gravitation,which was Einstein’s last dream.
I have a dream
concept
history
significance
nowdays
However things are not always goes as plain sailing.
The truth was that string theory could’t be test in laboratory.So that some scientist pointed out that it was only existed in fantasy.At the same time ,we could’t prove that it was wrong.
From where we stand,substance are consist of little dot,but string theory’s principle is line.
concept
history
significance
nowdays
String theory was shaped in 1968 by Gabriele Veneziano,(伽布利耶· 威尼采亚诺)a Italian’s physicist.He merely wanted to find a function to describe the strong interaction between the nucleus.Fortunately ,he found the Euler's Function in a math book which is two hundred years ago.This function was the model of string theory. But the contiual development is Leonard Susskind’s(李奥纳 特· 苏士侃) discovery.
concept
history
significance
nowdays 结论局限
• • •
The end The experiment is never end up,until the truth have been found. The end
The experiment is never end up,until the truth have been found.
弦论
String theorys develop ment history String theorys basic cocept
String theory’ s significa nce The curre nt study
The basic unit of nature is not electronic, photonic, neutrinos pointlike particles and quarks, but tiny linear (" string")。The basic granule are consist of string’s vibration and movement.