1. Introduction
1-introduction (s)(1-简介)

Marketing is so much more than that!(营销远 不止这些)
Packaging(包装) Strategy(策略) Competitive Strategies(竞 Sales, Sales-force 争优势) (销售,销售队伍) Market Penetration(市场 Brand Management 渗透) (品牌管理) Quality(质量)
Meaning:
Creating customer value and satisfaction are at the heart of modern marketing thinking A very simple definition of marketing is the delivery of customer satisfaction at a profit. Goals of marketing are to attract new customers by promising superior value and to keep and grow current customers by delivering satisfaction. Marketing is much more than selling and advertising. It is about understanding and satisfying customer needs.
Logistics(物流) Location(定位) Distribution Channels(分销渠道) Pricing Considerations(价格策略)
Globalization(全球化)
New Product Develop(新产品开发) Service Delivery(服务交付)
初中英语议论文写作 (1)
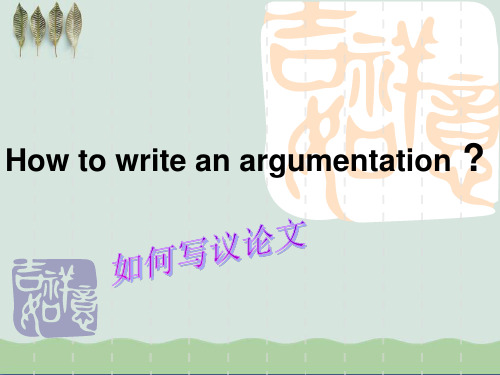
3) Recently we had a heated discussion on… Opinions are various among different people.
Shall we send children to study abroad?
With more and more people becoming rich in re cent years, it is a new tendency for them to
send their children to study abroad. But I don’t think it is a good idea.
Body(正文):
1. Some may hold the view that… because / firstly.. (有些人认为…)
But others have a negative/opposite attitude. From their point of view,…
(有些人却持有不同的观点, 他们认为…)
2. some people are against the idea of … because ….
while others are for the idea of … 3.1) (表顺序)Firstly…. (in the first place,/ first of all)
secondly… thirdly… , the last but not the least , 2)(表递进) what is more/in addition/ furthermore/
1.Introduction+structures

I. Classification of Writings
A. Literature Writings B. Scientific Writings (factual writings)
II. Principles for Good Scientific Writing (1)
Content: original Organization/Structure: complete, coherent Language: correct 文风有独创性;主题 处理好; 中心思想明确; 行文逻辑严密;选辞精确;句子、段落表达 完整;阅读效果好;没有错别字及语法、标 点符号的错误。
Brief & Simple
Though I usually go to the country for a walk during the weekend, I decided to spend the whole day in the city for a change last Sunday. I went to the Tian’anmen Square and the Zhongshan Park. It was so early when I left home that the streets around my neighborhood were deserted. Without the usual crowds and traffic, everything was strangely quiet.
Writing Suggestions:
Plan your writing (audience awareness / target readers). Ignore the language. Write and rewrite. Find readers. Keep writing. Find suitable methods from handbooks and guides. There is many a way to write.
1Introduction
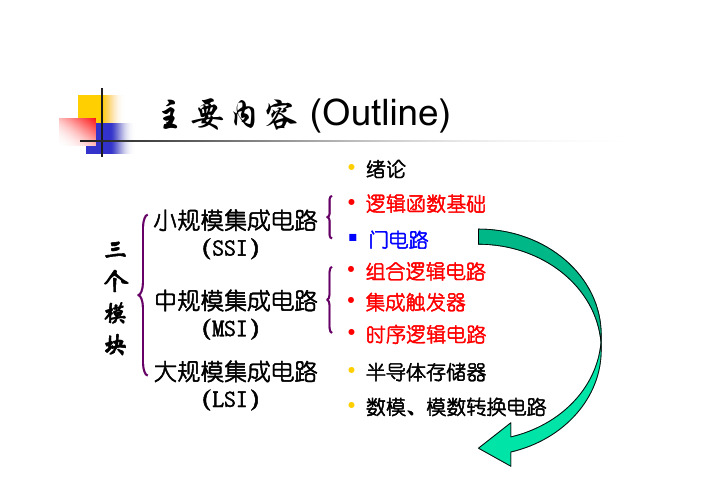
主要内容 (Outline)• 绪论小规模集成电路三(SSI)• 逻辑函数基础 门电路个• 组合逻辑电路模 块中规模集成电路 (MSI)• 集成触发器 • 时序逻辑电路大规模集成电路 • 半导体存储器(LSI)• 数模、模数转换电路绪论 (Introduction)一、数字(digital)信号和模拟(analog)信号 数字量和模拟量 数字电路和模拟电路二、数字信号相关概念 二进制数 Binary Digits 数字信号的逻辑电平 Logic Levels 数字信号波形 Digital Waveforms一、Digital Signal and Analog Signal Digital and Analog Quantities电子 电路 中的 信号模拟信号: 连续analogue signal value数字信号: 离散digital signal valuetime time模拟信号T( C) 30采样信号T( C)sampled3025离散化 2520202 4 6 8 10 12 2 4 6 8 10 12 t (h)A.M.P.M.2 4 6 8 10 12 2 4 6 8 10 12 t (h)A.M.P.M.数字化-表示 为由0、1组成 的二进制码Analog Electronic SystemDigital and Analog Electronic System★ 工作在模拟信号下的电子电路是模拟电路。
研究模拟电路时,注重电路输入、输出信号 间的大小、相位关系。
包括交直流放大器、 滤波器、信号发生器等。
★ 模拟电路中,晶体管一般工作在放大状态。
★ 工作在数字信号下的电子电路是数字电路。
研究数字电路时,注重电路输出、输入间的逻 辑关系。
主要的分析工具是逻辑代数,电路的 功能用真值表、逻辑表达式或波形图表示。
★ 在数字电路中,三极管工作在开关状态, 即工作在饱和状态或截止状态。
1.introduction 简介

Introduction
1、生物化学的概念 2、生物化学的发展简史
3、生物化学的主要研究内容 4、生物化学与其它学科的关系
生物化学的概念
生物化学是研究生命 化学的学科,它在分子水 平上探讨生命的本质,即 研究生物体的分子结构与 功能,物质代谢与调节, 及其在生命活动中的作用。
①吴宪
在血液化学分析方面创 立了血滤液的制备和血糖测定法; ②蛋白质方面,提出了蛋白质变 性学说; ③免疫学方面,研究抗原抗体的 反应机制;
④1965年,首先人工合成具
有生物活性的胰岛素; ⑤1981年,成功合成酵母丙 氨酰tRNA; ⑥完成人类基因组计划工作 的1%。
生物化学的研究内容
静态生物化学 动态生物化学 1、 功能生物化学 分子生物学 2、生物化学的研究采用化 学的原理和方法; 3、生物化学与细胞学、遗传学、 生理学关系密切;
生物化学的发展简史
1、初级阶段
2、发展阶段 3、分子生物学阶段
4、现状
初级阶段
1、对糖类、脂类、氨基酸作
了比较系统的研究; 2、发现了核酸; 3、化学合成了简单多肽; 4、酵母发酵过程中可溶性催 化剂的发现奠定了酶学的基础。
1、生物大分子的结构与功能; 2、物质代谢及其调节; 3、基因信息的传递及其调控。
生物大分子:由某些 基本结构单位按照一定的 顺序和方式连接所形成的 多聚体。 分子量一般在104以上
生物化学与其他学科的关系
1、与农业的关系 2、与工业的关系 3、与药学的关系 4、与医学的关系
与农业的关系
人类基因组计划
科学研究证明,人类的生老病死
都与基因有关,如果能够破译人 类基因的序列和功能,就有可能 真正有效地预防、诊断和治疗各 种疾病。因此,1990年正式启动 的人类基因组计划一开始就引起 了全世界的广泛关注。
Topic 1 Introduction
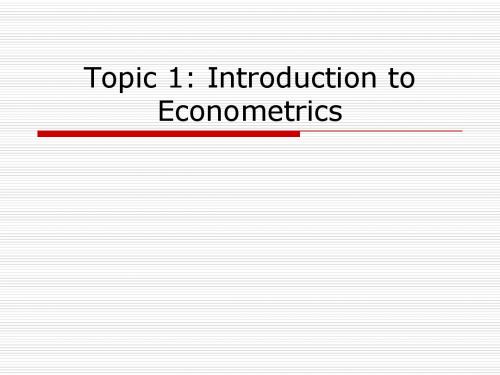
Trygve Haavelmo (Norway)
1980 Nobel Laureates in Economics
Lawrence R. Klein (University of Pennsylvania), “For the creation of econometric models and their application to the analysis of economic fluctuations and economic policies”
Topic 1: Introduction to Econometrics
What is econometrics? Why study econometrics? Types of econometrics Nobel Prize and Econometrics Methodology of econometrics
2. Specification of the Mathematical Model 1) Specification of variables e.g. consumption (income) inflation (money supply of the previous period, GDP growth rate) income (qualification, IQ, EQ, gender, etc.) weight (height, gender, race, age, etc.) * It should be based on economic theory and analysis of economic phenomena * Data availability * The relationship among variables: independence
1. INTRODUCTION
1. INTRODUCTION1.1. WHY USE ELECTRONS?Why should we use an electron microscope? Historically, TEMs were developed because of the limited image resolution in light microscopes, which is imposed by the wavelength of visible light. Only after electron microscopes were developed was it realized that there are many other equally sound reasons for using electrons, most of which are utilized to some extent in a modern TEM. By way of introduction to the topic let's look at how the TEM developed and the pros and cons of using such an instrument.1.1.A. An Extremely Brief HistoryLouis de Broglie (1925) first theorized that the electron had wave-like characteristics, with a wavelength substantially less than visible light. Then Davisson and Germer (1927) and Thompson and Reid (1927) independently carried out their classic electron diffraction experiments which demonstrated the wave nature of electrons. It didn't take long for the idea of an electron microscope to be proposed, and the term was first used in the paper of Knoll and Ruska (1932). In this paper they developed the idea of electron lenses into a practical reality, and demonstrated electron images taken on the instrument shown in Figure 1.1. This was a most crucial step, for which Ruska received the Nobel Prize, somewhat late, in 1986. Within a year of Knoll and Ruska's publication, the resolution limit of the light microscope was surpassed. Ruska, surprisingly, revealed that he hadn't heard of de Broglie's ideas about electron waves and thought that the wavelength limit didn't apply to electrons. TEMs were developed by commercial companies only four years later. The Metropolitan-Vickers EM 1 was the first commercial TEM. It was built in the UK in 1936, but apparently it didn't work very well and regular production was really started by Siemens and Halske in Germany in 1939. TEMs became widely available from several other sources (Hitachi, JEOL, Philips and RCA, inter alia) after the conclusion of World War II.For materials scientists a most important development took place in the late 1940s when Heidenreich (1949) first thinned metal foils to electron transparency. This work was followed up by Bollman in Switzerland and Hirsch and co-workers in Cambridge. Because so much of the early TEM work examined metal specimens, the word "foil" has come to be synonymous with "specimen." In addition, the Cambridge group also developed the theory of electron diffraction contrast with which we can now identify, often in a quantitative manner, all known line and planar crystal defects in TEM images. This theoretical work is summarized in a formidable but essential text often referred to as the "Bible" of TEM (Hirsch et al. 1977). For the materials scientist,practical applications of the TEM for the solution of materials problems were pioneered in the United States by Thomas and first clearly expounded in his text (Thomas 1962). Other materials-oriented texts followed, e.g., Edington (1976) and Thomas and Goringe (1979).Today, TEMs constitute arguably the most efficient and versatile tools for the characterization of materials. If you want to read a history of the TEM, the book by Marton (1968) is a compact, personal monograph and that edited by Hawkes (1985) contains a series of individual reminiscences. Fujita (1986) emphasizes the contribution of Japan to the development of the instrument. The field is now at the point where many of the pioneers have put their memoirs down on paper, or Festschrifts have been organized in their honor (e.g., Cosslett 1979, Ruska 1980, and Hashimoto 1986) which detail their contributions over the decades, and compile some useful overview papers of the field. If you enjoy reading about the history of science, we strongly recommend the review of Fifty Years of Electron Diffraction, edited by Goodman (1981), and Fifty Years of X-ray Diffraction, edited by Ewald (1962). (The spelling of X-ray is discussed in the CBE Manual, 1994.)Figure 1.1. The electron microscope built by Ruska and Knoll in Berlin in the early 1930s.1.1.B. Microscopy and the Concept of ResolutionWhen asked what a "microscope" is, most people would answer that it is an instrument for magnifying things too small to see with the naked eye, and most likely they would be referring to the visible-light microscope. Because of the general familiarity with the concept of the light microscope, we will draw analogies between electron and visible-light microscopes wherever it's instructive.The smallest distance between two points that we can resolve with our eyes is about 0.1-0.2 mm, depending on how good our eyes are, and assuming that there's sufficient illumination to see by. This distance is the resolution or resolving power of our eyes. So any instrument that can show us pictures (or "images" as we'll refer to them) revealing detail finer than 0.1 mm could be described as a microscope, and its highest useful magnification is governed by its resolution. A major attraction to the early developers of the TEM was that, since electrons are smaller than atoms, it would be possible, at least theoretically, to build a microscope that could "see" detail well below the atomic level. The idea of being able to "see" with electrons may be confusing to you. Our eyes are not sensitive to electrons. If a beam of high-energy electrons was aimed into your eye, you would most likely be blinded as the electrons killed the retinal cells, but you wouldn't see anything! So an integral part of any electron microscope is a viewing screen of some form, which translates electron intensity to light intensity, and which we observe or record photographically. We'll discuss these screens and other ways of recording electron images in later chapter.The resolution of a TEM means different things for different functions of the instrument, and we'll discuss them in the appropriate chapters. It's easiest to think of the image resolution in TEM in terms of the classical Rayleigh criterion for light microscopy, which states that the smallest distance that can be resolved, , is given approximately by δβµλ=δsin 61.0 [1.1]In equation 1.1, is the wavelength of the radiation, is the refractive index of the viewing medium, and is the semiangle of collection of the magnifying lens. For the sake of simplicity we can approximate sin (which is sometimes called the numerical aperture) to unity and so the resolution is equal to about half the wavelength of light. For green light in the middle of the visible spectrum, is about 550 nm (5500Å), and so the resolution of a good light microscope is about 300 nm. In TEMs we can approximate the resolution in equation 1.1 to 0.61/ which, as we'll see later, is very small.λµβµβλλβNow although 300 nm is a small dimension to us it corresponds to about 1000 atom diameters, and therefore many of the features that control the properties of materials are on a scale well below the resolution of the light microscope. So there's a real need to image detail down to the atomic level if we want to understand the properties of materials, and that's a major reason why TEMs are so useful.We'll try to use nanometers throughout this book, but you'll find that many microscopists still insist on using Angstroms rather than the SI units. However, the Angstrom is close to the atomic diameter and so is a more convenient unit because it saves us using convoluted phrases like “three tenths of a nanometer.”This limit of light microscopy was well understood at the turn of this century and prompted Ernst Abbe, one of the giants in the field, to complain that "it is poor comfort to hope that human ingenuity will find ways and means of overcoming this limit." (He was right to be so depressed because he died in 1905, some 20 years before de Broglie's ingenuity solved the problem.) Now de Broglie's famous equation shows that the wavelength of electrons is related to their energy, E, and if we ignore relativistic effects we can show approximately (and exactly in Section 1.4 below) that2/122.1~Eλ [1.2]In this equation E is in electron volts (eV) and in nm. Remember that we should be precise in our use of the units V and eV: the former represents the accelerating voltage of the microscope while the latter refers to the energy of the electrons in the microscope. So for a 100-keV electron, we find that ~ 4 pm (0.004 nm), which is much smaller than the diameter of an atom.λλFigure 1.2. A twin boundary in spinel stepping from one {111} plane to another parallel plane. The white dots are columns of atoms. The change in atomic orientation across the twin boundary can be readily seen, even if we do not know what causes the white dots or why, indeed, they are white.We'll see later that we are nowhere near building TEMs that approach this wavelength limit of resolution, because we can't make perfect electron lenses (see Section 2). But progress was rapid after Ruska's early work on lenses and, since the mid-1970s, many commercial TEMs have been capable of resolving individual columns of atoms in crystals, creating the field of "high-resolution transmission electron microscopy," or HRTEM. A typical HRTEM image is shown in Figure 1.2. The advantages of shorter wavelengths led in the 1960s to the development of high voltage electron microscopes (HVEMs), with accelerating potentials between 1 MV and 3 MV . In fact, most of these instruments were used to introduce controlled amounts of radiation damage into specimens in an attempt to simulate nuclear reactor environments, but changes in the emphasis of energy research mean there is not much call for such instruments today. While we can still improve the resolution byincremental amounts, the drive for much better resolution is now no longer paramount and the TEM is developing in other ways. In fact, only one HVEM (1 MV) for HRTEM imaging was constructed in the 1980s and three 1.25-MV machines in the 1990s. Intermediate voltage electron microscopes (IVEMs) were introduced in the 1980s. These TEMs operate at 300 or 400 kV, but still offer very high resolution, close to that achieved at 1 MV.1.1.C. Interaction of Electrons with MatterElectrons are one type of "ionizing radiation," which is the general term given to radiation that is capable of removing one of the tightly bound inner-shell electrons from the attractive field of the nucleus.One of the advantages to using ionizing radiation is that it produces a wide range of secondary signals from the specimen, and some of these are summarized in Figure 1.3. Many of these signals are used in "analytical electron microscopy,'' or AEM, giving us chemical information and a lot of other detail about our samples. AEM uses X-ray energy dispersive spectrometry (XEDS) and electron energy-loss spectrometry (EELS). For example, Figure 1.4A is an X-ray spectrum from a very small region of a TEM specimen showing characteristic peaks which identify the elements present. We can transform such spectra into quantitative data describing elemental changes associated with inhomogeneous microstructures as also shown in Figures 1.4B and C. In contrast, microscopes using nonionizing radiation such as visible light usually only generate light (but not much heat, which is good). AEMs generally offer improved performance at intermediate voltages, similar to HRTEMs.Figure 1.3. Signals generated when a high-energy beam of electrons interacts with a thin specimen. Most of these signals can be detected in different types of TEM. The directions shown for each signal do not always represent the physical direction of the signal but indicate, in a relative manner, where the signal is strongest or where it is detected.In order to get the best signal out of our specimens we have to put the best signal in, and so the electron source is critical. We are now very accomplished in this respect as you'll see in Section 4, so modern TEMs are very good signal-generating instruments. To localize these signals we need to get our TEM to form a very fine electron beam, typically <10 nm and at best <1 nm in diameter. We accomplish this by combining TEM and scanning electron microscope (SEM) technology to create a scanning transmission electron microscope (STEM). The STEM is both the basis for AEMs and a unique scanning imaging microscope in its own right. In fact there are instruments that are only capable of operating in scanning mode and these are sometimes referred to as "dedicated STEMs," or DSTEMs.1.1.D. Depth of FieldThe depth of field of a microscope is a measure of how much of the object we are looking at remains "in focus" at the same time. Like the resolution, this property is governed by the lenses in the microscope. The best electron lens is not a very good one, as we've already mentioned, and has been compared to using the bottom of a Coca-Cola bottle as a lens for light microscopy. To minimize this problem we have to use very small limiting apertures in the lenses, narrowing the beam down to a thin "pencil" of electrons which at most is a few micrometers across. These apertures cut down the intensity of the electron beam, but also act to increase the depth of focus of the images that we produce. Remember that "depth of field" refers to the specimen while "depth of focus" refers to the image.While this large depth of field is chiefly used in the SEM to produce 3D-like images of the surfaces of specimens with large changes in topography, it is also critical in the TEM. It turns out that in the TEM, all of the specimen is usually in focus at the same time, independent of the specimen topography, as long as it's electron transparent! Figure 1.5 shows a TEM image of some dislocations in a crystal. The dislocations appear to start and finish in the specimen, but in fact they are threading their way through the specimen from the top to the bottom, and they remain in sharp focus at all times. Furthermore, we can record the final image at different positions below the final lens of the instrument and it will still be in focus. Compare this with the visible-light microscope where, as you probably know, unless the surface of the specimen is flat to within the wavelength of light, it is not all in focus at the same time. This aspect of TEM gives us both advantages and disadvantages in comparison to the visible-light microscope.A BC Figure 1.4. (A) An X-ray spectrum from asmall biotite crystal showing peaks atenergies that are characteristic of theelements present in the region thatinteracts with the electron beam. Themajor peaks from left to right are for Mg,Al, Si, K, Fe, and the Cu support grid. (B)A TEM image of a precipitate-free zone(PFZ) in an aged Al-16 wt% Ag alloy. (C)The Ag profile across the PFZ in (B),obtained through X-ray spectrometry inthe TEM showing the depletion of Agresponsible for the PFZ formation.Figure 1.5. TEM image of dislocations in GaAs. A band of dislocations threads through the thin specimen from the top to the bottom but remains in focus through the foil thickness.1.1.E. DiffractionThompson and Reid showed that electrons could be diffracted when passing through thin crystals of nickel, and the possibility of combining electron diffraction into TEMs was realized by Kossel and Mollenstedt (1939). Today, electron diffraction is an indispensable part of TEM and is arguably the most useful aspect of TEM for materials scientists. Figure 1.6 shows a TEM diffraction pattern which contains information on the crystal structure, lattice repeat distance, and specimen shape, as well as being a most striking pattern. We'll see that the pattern can always be related to the image of the area of the specimen from which it came, in this case shown in the inset. In addition to the things we just listed, you can conduct a complete crystallographic symmetry analysis of minuscule crystals, including such esoteric aspects as point-group and space-group determination, and at all times the crystallography can be related to the image of your specimen. There is no similar capability on a light microscope because of the relatively large wavelength of visible light.So an electron microscope can produce atomic level images, can generate a variety of signals telling you about your sample chemistry and crystallography, and you can always produce images that are in focus. There are many other good reasons why you should use electron microscopes. We hope they will become evident as you read through this book. At the same time there are many reasons why you should not always seek to solve your problems with the TEM, and it is most important that you realize what the instrument cannot do, as well as knowing its capabilities.Figure 1.6. TEM diffraction pattern from a thin foil of A1-Li-Cu containing various precipitate phases, shown in the inset image. The central spot (X) contains electrons that come directly through the foil and the other spots and lines are diffracted electrons which are scattered from different crystal planes.。
unit_1_Introduction_and_greeting
I will choose two groups to present!
Dialog 1
• • • • • • Read the dialog together. Find out the elements below: Who: Where: When: What:
• Page 6. Act Out.
forms and ceremonies seriously; freedom from constraint or embarrassment 非正式,不拘礼节
a garrulous informality that is explosive, intense and distinctly American. 他29岁,有着深蓝色的眼镜,絮絮叨叨不拘礼节,是个活 泼、热情、典型的美国人。
Part I: Intensive Reading Task1: Topic: Ways Americans greet, introduce and
say goodbye to people.
Section Learning
Main ideas:
1) In the American society, informality is valued very highly. 2) When you greet people who you already know, you are expected to show a certain amount of informality toward them.
e.g: He is 29 years old, with quiet blue eyes and
Section Learning
Language Points:
制药工程_专业英语_Unit_5
2、研发计划 对候选药物是否有可能提供有竞争力的优势方面的评估,首先
需要强调的是应达到一整套的产品目标或目标产品的特性。应当 特别注意竞争者(指药物)之间的差异。随着对有限的处方、医 疗保健费用以及药品经济学的日益关注,这种情况变得越来越重 要(本章稍后讨论)。
A target profile will define the indication(s) that a drug candidate will be develope d for along with goals such as once a day dosing,faster onset of action, better side effect profile than a major competitor. The target profile can be refined and revised as a drug candidate moves through development and new data on the drug candidate or competitors become available. The logical next steps are to defin e the development strategy,for example,which indications to develop first which countries to aim to market the drug in and then to define the core clinical st udies necessary to achieve regulatory approval and commercial success.
雅思写作大作文SimonWritingTask2视频课笔记
Lesson 2: Introductions ---- Four types of questions1. DiscussionSome people think that it is more effective for students to study in groups, while others believe that it isbetter for them to study alone. Discuss both views and give your own opinion.Topic- study in groups or aloneAnswer- sometimes better alone, usually better in a groupPeople have different views about the effectiveness of group study as opposed to working alone. While there are some benefits to studying independently, I believe that group work is usually more productive.2. OpinionSome people believe that unpaid community service should be a compulsory part of high school programmers. To what extent do you agree or disagree?Topic- community service for all teenagersAnswer- 3 choice (agree, disagree, balanced opinion)It is sometimes argued that high school students should be made to do some work in their local communities. (agree) I completely agree that this kind of scheme would be a good idea.(disagree) It is sometimes argued that high school students should be made to do some work in their local communities. In my opinion, it would be wrong to force teenagers to do any kind ofunsalaried work.(want mention both side) It is sometimes argued that high school students should be made to do some work in their local communities. While I disagree with the idea of making such programmers compulsory, I do believe that voluntary community service could benefit young people.3. Problem and solutionSome people reoffend after they have been punished. Why do some people continue to commit crimesafter they have been punished, and what measures can be taken to tackle this problem?Topic- criminals reoffendAnswer- several reasons, a variety of measures (government, communities)It is true that punishments do not always deter criminals from committing more crimes. There are various reasons why offenders repeatedly break the law, but governments could certainly take steps to address this issue.4. Two-part questionAs most people spend a major part of their adult life at work, job satisfaction is an important element of individual well-being. What factors contribute to job satisfaction? How realistic is the expectation of job satisfaction for all workers?Topic- job satisfactionAnswer- several factors, unrealistic / impossibleWork plays a central role in our lives, and we would all like to feel fulfilled professionally. While a variety of factors may lead to job satisfaction, it would be unrealistic to expect everyone to be happy at work.Lesson 3: Main paragraphs ---- Firstly, secondly, finally & Idea, explain, example4 paragraph essay- 2 main body paragraphs - 5 sentences in each- 90 to 100 words each 2 types- Firstly, secondly, finally[ advantages, disadvantages, problems, solutions ] - Idea, explain, example[ one idea, a reason, an opinion ]Some people believe that unpaid community service should be a compulsory part of high school programmers. To what extent do you agree or disagree?Firstly, secondly, finally3-minute plan:-disagree for several reasons-school timetable is full, no time for community service-students’ work in other subjects would be affected-teenagers might not want to do it (reluctant, no motivation) Try to write 5 sentences1. Topic sentences2. Firstly3. Secondly4. FinallyThere are several reasons why I would argue against having compulsory community service for secondary school students. Firstly, the school curriculum is already full with important academic subjects, such as maths, science and languages. For example, I remember having an extremely busy timetable when I was at high school, and it would not have been possible to add to it. Secondly, students’ performance in other subjects would be affected if valuable study time were taken by charity work or neighbourhood improvement schemes. Finally, I believe that teenage students would be reluctant to take part in any programme of obligatory work, and this could lead to poor motivation and even bad behaviour.Idea, explain, example3-minute plan:- voluntary (not compulsory) community service is positive - students more motivated if they can choose- gain work experience, self confidence, skills- good for CVs, career, university admissions, employers3 Try to write 5 sentences IdeaExplain (2 or 3 sentences) ExampleOn the other hand, the opportunity to do voluntary community service could be extremely positive for high school students. By making these programmes optional, schools would ensure that only motivated students took part. These young people would gain valuable experience in an adult working environment, which could help to build their self confidence and enhance their skills. Having such experience and skills on their CVs could greatly improve school leavers’c areer prospects. For example, a period of voluntary work experience might impress a university admissions officer or a future employer.Linking words do not help your “vocabulary” score.Examiners want to see “topic vocabulary”.blue - linking words green - Topic vocabulary red– paraphrasingLesson 4: Conclusions- One sentence - No new information - Paraphrasing to show variety1. Discussion questionIn many cities the use of video cameras in public places is being increased in order to reduce crime,but some people believe that these measures restrict our individual freedom.Do the benefits of increased security outweigh the drawbacks?IntroductionIt is true that video surveillance has become commonplace in many cities in recent years. While I understand that critics may see this as an invasion of privacy, I believe that there are more benefits than drawbacks.ConclusionIn conclusion, I would argue that the advantages of using video security systems in public places dooutweigh the disadvantages.2. Opinion questionFamilies who send their children to private schools should not be required to pay taxes that support thestate education system.To what extent do you agree or disagree with this statement?IntroductionSome people believe that parents of children who attend private schools should not need to contribute to state schools through taxes. Personally, I completely disagree with this view.ConclusionIn conclusion, I do not believe that any financial concessions should be made for people who chooseprivate education.3. Problem and solution questionIn the developed world, average life expectancy is increasing.What problems will this cause for individuals and society?Suggest some measures that could be taken to reduce the impact of ageing populations.IntroductionIt is true that people in industrialised nations can expect to live longer than ever before. Although there will undoubtedly be some negative consequences of this trend, societies can take steps to mitigate these potential problems.ConclusionIn conclusion, various measures can be taken to tackle the problems that are certain to arise as the populations of countries grow older.4. Two-part questionThere are many different types of music in the world today.Why do we need music? Is the traditional music of a country more important than the internationalmusic that is heard everywhere nowadays?IntroductionIt is true that a rich variety of musical styles can be found around the world. Music is a vital part of all human cultures for a range of reasons, and I would argue that traditional music is more important than modern, international music.ConclusionIn conclusion, music is a necessary part of human existence, and I believe that traditional music should be given more importance than international music.Lesson 5: PlanningSome people think that it is more effective for students to study in groups, while others believe that it is betterfor them to study alone.Discuss both views and give your own opinion. 10-minute essay plan, 3 steps:1. Read and understand the question2. Plan your essay structure3. Plan ideas for the 2 main paragraphs1. Read and understand the question - highlight / underline key parts2. Plan your essay structure (4 paragraphs)1. Introduction: topic + answer[ topic ] – study in groups or alone[ answer ] – sometimes better alone, usually better in a group2. Benefits of studying alone3. Benefits of group study (my view)4. Conclusion: repeat answer - both have benefits, but I prefer group3. Plan ideas for the 2 main paragraphs (6 minutes)- brainstorm, note down any ideas you have- develop ideas in detail- keep asking yourself “why?” - think of examples to support your ideas- finally, try to group related ideas (number them)Lesson 6: Opinion essay ---- Do YOU agree or disagree? ( You CAN use “I” ,“my” or “in my opinion” )The money spent by governments on space programmes would be better spent on vital public servicessuch as schools and hospitals. To what extent do you agree or disagree? (275 words, band 9)agree, public services instead of space projectsgovernment spending[ answer ] – 1. Introduction: [ topic ] –2. Explain why “space” spending should be stoppedexpensive (scientists, facilities, equipment),no benefits to normal people, politicians showing power,waste of money when project fail, risk of accidents, death e.g.challenger space shuttle3. Explain why public service spending is bettercheaper e.g. doctors, teachers, police instead of astronautspublic service impact on everyonewe all use schools, hospitals, police, roads etc.reduce poverty, better quality of lifespend on services that benefit us all4. Conclusion: repeat answer – Governments in some countries spend large amounts of money onspace exploration programmes. I completely agree with the ideathat these are a waste of money, and that the funds should beallocated to public services.There are several reasons why space programmes should beabandoned. Firstly, it is extremely expensive to train scientists andother staff involved with space missions, and facilities andequipment also come at a huge cost to the government. Secondly,these programmes do not benefit normal people in our daily lives;they are simply vanity projects for politicians. Finally, manymissions to space fail completely, and the smallest technologicalerror can cost astronauts their lives. The Challenger space shuttledisaster showed us that space travel is extremely dangerous, andin my opinion it is not worth the risk.I believe that the money from space programmes should go to vitalpublic services instead. It is much cheaper to train doctors,teachers, police and other public service workers than it is to trainastronauts or the scientists and engineers who work on spaceexploration projects. Furthermore, public servants do jobs that havea positive impact on every member of society. For example, we alluse schools, hospitals and roads, and we all need the security thatthe police provide. If governments reallocated the money spent onspace travel and research, many thousands of people could belifted out of poverty or given a better quality of life.In conclusion, my view is that governments should spend money onservices that benefit all members of society, and it is wrong towaste resources on projects that do not improve our everyday lives.Lesson 7: Discussion essaySome people think that a sense of competition in children should be encouraged. Others believe that children who are taught to co-operate rather than compete become more useful adults.Discuss both these views and give your own opinion. (270 words, band 9)1. Introduction:benefits of both, co-operation more important [ topic ] –competition or co-operation?[ answer ] –2. Why encourage competition?motivation,work harder, be better than other children,self confidence, independent work, faster progress,competitive situation when leave school e.g. job interviews,prepared for adult life3. Why teach co-operation? (my view)co-operation even more important,e.g. at work (team, follow boss’s instructions, help junior staff),collaboration more useful than winning,better attitude for young people,working togetheraccept both views, co-operation better4. Conclusion: repeat answer – People have different views about whether children should be taught to becompetitive or co-operative. While a spirit of competition can sometimes beuseful in life,I believe that the ability to co-operate is more important.On the one hand, competition can be a great source of motivation forchildren. When teachers use games or prizes to introduce an element ofcompetitiveness into lessons, it can encourage children to work harder tooutdo the other pupils in the class. This kind of healthy rivalry may help to, while pushing them to work independentlybuild children’s self confidenceand progress more quickly. When these children leave school, theirconfidence and determination will help them in competitive situations suchas job interviews. It can therefore be argued that competition should beencouraged in order to prepare children for adult life.On the other hand, it is perhaps even more important to prepare childrenfor the many aspects of adult life that require co-operation. In the workplace,adults are expected to work in teams, follow instructions given by theirsuperiors, or supervise and support the more junior members of staff.Team collaboration skills are much more useful than a competitivedetermination to win. This is the attitude that I believe schools should fosterin young people. Instead of promoting the idea that people are eitherwinners or losers, teachers could show children that they gain more fromworking together.In conclusion, I can understand why people might want to encouragecompetitiveness in children, but it seems to me that a co-operative attitudeis much more desirable in adult life.Lesson 8: Problem and solution essayIn many countries schools have severe problems with student behaviour.What do you think are the causes of this? What solutions can you suggest? (270 words, band 9)1. Introduction:variety of reasons, steps can be taken to tackle [ topic ] –student behaviour in schools[ answer ] – 2. Cause of bad student behaviourparents not strict (too leninent), children don’t accept teachers’ instructions or school rules, teachers’ fault, no control, bad classroom management,influence of celebrities who are bad example3. My suggested solutionsparents set rules for children, use punishments, actions have consequences,schools train teachers and parents, discipline techniques, better communication,famous people act as role modelssummarise the problem and steps4. Conclusion: repeat answer – It is true that the behaviour of school pupils in some parts of the worldhas been getting worse in recent years. There are a variety ofpossible reasons for this, but steps can definitely be taken to tacklethe problem.In my opinion, three main factors are to blame for the way youngpeople behave at school nowadays. Firstly, modern parents tend tobe too lenient or permissive. Many children become accustomed togetting whatever they want, and they find it difficult to accept thedemands of teachers or the limits imposed on them by school rules.Secondly, if teachers cannot control their students, there must be anissue with the quality of classroom management training or supportwithin schools. Finally, children are influenced by the behaviour ofcelebrities, many of whom set the example that success can beachieved without finishing school.Student behaviour can certainly be improved. I believe that thechange must start with parents, who need to be persuaded that it isimportant to set firm rules for their children. When childrenmisbehave or break the rules, parents should use reasonablepunishments to demonstrate that actions have consequences. Also,schools could play an important role in training both teachers andparents to use effective disciplinary techniques, and in improving thecommunication between both groups. At the same time, famouspeople, such as musicians and football players, need to understandthe responsibility that they have to act as role models to children.In conclusion, schools will continue to face discipline problemsunless parents, teachers and public figures set clear rules anddemonstrate the right behaviour themselves.Lesson 9: Two-part essayNews editors decide what to broadcast on television and what to print in newspapers. What factors do you think influence these decisions? Do we become used to bad news, and would it be better if more good news was reported? (285 words, band 9)variety of factors, yes too much bad news1. Introduction: [ topic ] –decisions about news stories[ answer ] – 2. Factors that influence news editorsinterest or attract viewers / readersinform the public, important issue and events, in the public interestpressure from owners, promote political views3. Too much bad news, should report more goodaccustomed to bad news,war, crime, natural, disasters, human suffering,desensitizes us, cynical about the world,prefer positive news, e.g. medical workers, volunteers, kindness,news to inspire usdifficult news choices, more positive4. Conclusion: repeat answer – It is true that editors have to make difficult decisions about which news storiesthey broadcast or publish, and their choices are no doubt influenced by avariety of factors. In my opinion, we are exposed to too much bad news, and Iwould welcome a greater emphasis on good news.Editors face a range of considerations when deciding what news stories tofocus on. Firstly, I imagine that they have to consider whether viewers orreaders will be interested enough to choose their television channel or theirnewspaper over competing providers. Secondly, news editors have aresponsibility to inform the public about important events and issues, and theyshould therefore prioritise stories that are in the public interest. Finally, editorsare probably under some pressure from the owners who employ them. Forexample, a newspaper owner might have particular political views that he orshe wants to promote.It seems to me that people do become accustomed to negative news. We areexposed on a daily basis to stories about war, crime, natural disasters andtragic human suffering around the world. I believe that such repeatedexposure gradually desensitizes people, and we become more cynical aboutthe world and more sceptical that we can do anything to change it. I wouldprefer to see more positive news stories, such as reports of the work ofmedical staff after a natural disaster, or the kindness of volunteers who help intheir communities. This kind of news might inspire us all to lead better lives.In conclusion, it must be extremely difficult for editors to choose which newsstories to present, but I would like to see a more positive approach to this vitalpublic service.。
- 1、下载文档前请自行甄别文档内容的完整性,平台不提供额外的编辑、内容补充、找答案等附加服务。
- 2、"仅部分预览"的文档,不可在线预览部分如存在完整性等问题,可反馈申请退款(可完整预览的文档不适用该条件!)。
- 3、如文档侵犯您的权益,请联系客服反馈,我们会尽快为您处理(人工客服工作时间:9:00-18:30)。
Animal Nutriology
Physiology Reproductive Biology Developmental Biology Genetics
1. Introduction to Zoology
College of Animal Science and Technology
Jlliang Zhang (Ph. D)
1
REang_zoology@ (password: zoology) Office address: Room217, Buliding 3.
Taxonomic Groups
Classification of Man
• • • • • • • Kingdom Phylum Class Order Family Genus Species Animalia Chordata Mammalia Primates Hominidae Homo Homo sapiens
33
Results
• Boy did not get smallpox
34
Conclusion
• Hypothesis supported
35
Aristotle 384 BC
• Classified organisms as either plants or animals
Carolus Linnaeus 1707-1778
Cowpox Makes You Immune to Smallpox 牛痘可以使人对天花产生 免疫
Prediction
• If you are exposed to cowpox, you will be immune to smallpox • 假如种植牛痘,你就可以对天花产生免疫 力
32
Experiment
Theory of Evolution
• Scientists no longer ask if evolution occurs. They study how evolution occurs. • Evolution is the major theory that guides research in Zoology
Ernst Mayr
*
* Archaea (古细菌)
Classification of Living Things
DOMAIN
Bacteria
Archaea
Eukarya
KINGDOM
CELL TYPE
Eubacteria
Prokaryote (原核生物)
Archaebacteria
Prokaryote
Ecology
Zoogeography Biodiversity and Conservation
Paleontology(古生物学)
Etiology(病原学) Evolution
6
Zoology is the scientific study of the
characteristics and classification of
• Hypothesis(假设): A prediction of the
outcome of an experiment • Written: If______________, then _____________, because____________.
Testing Hypotheses
• Observation
– Something you take in with your senses
• Experiment
– Perform CONTROLLED experiments to test repeated observations
– If continued to be accepted………….
• Cell Theory
– All living things are made of cells
Theory of Evolution
• Populations of organisms change over time • Changes result in new species that share a common ancestor.
animals.
7
8
• Zoologists study how animals live, and why they live that way.
9
• Students who study zoology learn a diversity of topics, ranging from the structure and function of the cells that make up animals, through anatomy and physiology, reproduction and development, evolution and behaviour. • This knowledge is important for a range of activities, including biomedical research, agricultural and fisheries practices, the management of natural resources, environmental planning and for understanding the natural world.
• Theory
– Explains laws, hypotheses and facts
• Law
– States what happens
• Hypothesis
– Untested theory
• Fact
– Observation
Major Scientific Theories
• Germ Theory of Disease(生源说)
Evolution is both a fact and a theory
• Fact
– Evolution is documented in the fossil record and has been observed in our lifetime.
• Theory
– How evolution happens
• Theory
– Explains why or how something in nature happens
Which is most important to a scientist?
1. 2. 3. 4.
Fact Hypothesis Law Theory
Theory is the most important
Scientific Theory
• • • • • Principle Tested many times Explains many different phenomena Makes predictions Falsifiable – people are constantly trying to prove wrong and correct “bad”’ science
4
• Zoology is the branch of biology that focuses on
the structure, function, behavior, and evolution of animals.
5
The International Society of Zoological Sciences
10
• Zoology is an amazingly broad discipline, and a degree with zoological knowledge can lead to diverse and interesting careers.
11
Scientific Terminology(术语)
• Swedish Botanist(植物 学家) • Systema Naturae, 10ed
– 1758
• Classification system • Taxonomic groups of related organisms • Binomial nomenclature
– two names – Genus + species – Capitalized, Italics
40
Species
• “Species are groups of actually or potentially interbreeding populations, which are reproductively isolated from other such groups.” • When they reproduce, create FERTILE offspring
Myths To Explain Biological Processes
• Disease caused by evil spirits • 疾病源于魔鬼
25
Edward Jenner 1749-1823
• Smallpox (天花) • Scientific method
26
Observation
Cell walls of Cell walls of No cell walls cellulose (纤 Cell walls of cellulose; or 维素) in some; chitin (几 some have chloroplast chloroplas 丁质) chloroplasts s ts (叶绿体)