Pt-石墨烯复合材料
石墨烯复合材料的制备、表征及性能
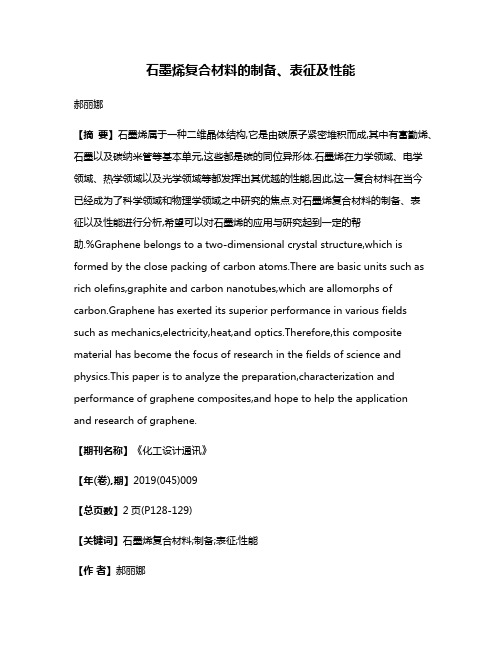
石墨烯复合材料的制备、表征及性能郝丽娜【摘要】石墨烯属于一种二维晶体结构,它是由碳原子紧密堆积而成,其中有富勤烯、石墨以及碳纳米管等基本单元,这些都是碳的同位异形体.石墨烯在力学领域、电学领域、热学领域以及光学领域等都发挥出其优越的性能,因此,这一复合材料在当今已经成为了科学领域和物理学领域之中研究的焦点.对石墨烯复合材料的制备、表征以及性能进行分析,希望可以对石墨烯的应用与研究起到一定的帮助.%Graphene belongs to a two-dimensional crystal structure,which is formed by the close packing of carbon atoms.There are basic units such as rich olefins,graphite and carbon nanotubes,which are allomorphs of carbon.Graphene has exerted its superior performance in various fields such as mechanics,electricity,heat,and optics.Therefore,this composite material has become the focus of research in the fields of science and physics.This paper is to analyze the preparation,characterization and performance of graphene composites,and hope to help the applicationand research of graphene.【期刊名称】《化工设计通讯》【年(卷),期】2019(045)009【总页数】2页(P128-129)【关键词】石墨烯复合材料;制备;表征;性能【作者】郝丽娜【作者单位】齐齐哈尔工程学院,黑龙江齐齐哈尔 161005【正文语种】中文【中图分类】TB332 ;TM53因为石墨烯所具有的二维晶体结构是比较特殊的,所以其纵横比很高、电子迁移率也很高,这就使得石墨烯在储能领域之中的应用前景十分广泛。
石墨烯基复合材料的制备及性能研究
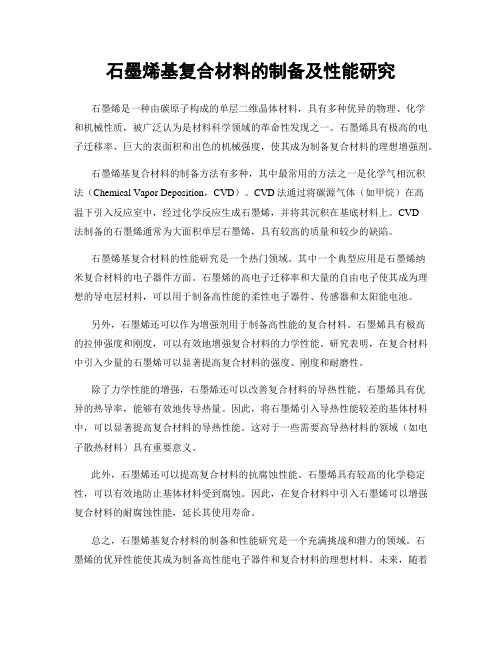
石墨烯基复合材料的制备及性能研究石墨烯是一种由碳原子构成的单层二维晶体材料,具有多种优异的物理、化学和机械性质,被广泛认为是材料科学领域的革命性发现之一。
石墨烯具有极高的电子迁移率、巨大的表面积和出色的机械强度,使其成为制备复合材料的理想增强剂。
石墨烯基复合材料的制备方法有多种,其中最常用的方法之一是化学气相沉积法(Chemical Vapor Deposition,CVD)。
CVD法通过将碳源气体(如甲烷)在高温下引入反应室中,经过化学反应生成石墨烯,并将其沉积在基底材料上。
CVD法制备的石墨烯通常为大面积单层石墨烯,具有较高的质量和较少的缺陷。
石墨烯基复合材料的性能研究是一个热门领域。
其中一个典型应用是石墨烯纳米复合材料的电子器件方面。
石墨烯的高电子迁移率和大量的自由电子使其成为理想的导电层材料,可以用于制备高性能的柔性电子器件、传感器和太阳能电池。
另外,石墨烯还可以作为增强剂用于制备高性能的复合材料。
石墨烯具有极高的拉伸强度和刚度,可以有效地增强复合材料的力学性能。
研究表明,在复合材料中引入少量的石墨烯可以显著提高复合材料的强度、刚度和耐磨性。
除了力学性能的增强,石墨烯还可以改善复合材料的导热性能。
石墨烯具有优异的热导率,能够有效地传导热量。
因此,将石墨烯引入导热性能较差的基体材料中,可以显著提高复合材料的导热性能。
这对于一些需要高导热材料的领域(如电子散热材料)具有重要意义。
此外,石墨烯还可以提高复合材料的抗腐蚀性能。
石墨烯具有较高的化学稳定性,可以有效地防止基体材料受到腐蚀。
因此,在复合材料中引入石墨烯可以增强复合材料的耐腐蚀性能,延长其使用寿命。
总之,石墨烯基复合材料的制备和性能研究是一个充满挑战和潜力的领域。
石墨烯的优异性能使其成为制备高性能电子器件和复合材料的理想材料。
未来,随着对石墨烯制备技术和性能研究的不断深入,相信石墨烯基复合材料将在各个领域展现出更多的应用前景。
石墨烯复合材料的研究及其应用
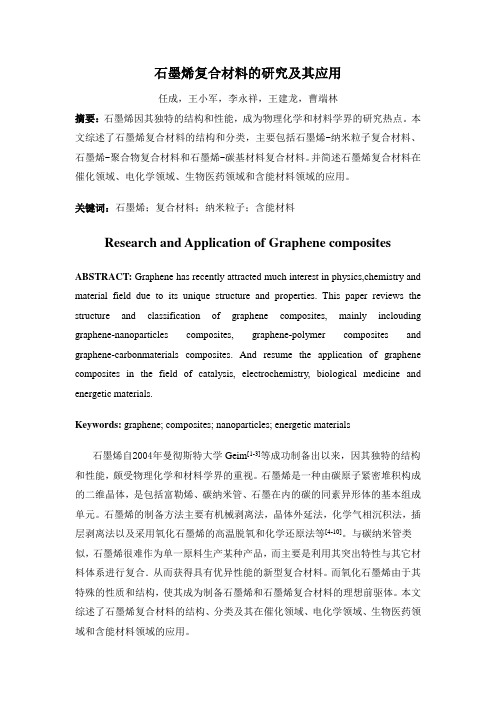
石墨烯复合材料的研究及其应用任成,王小军,李永祥,王建龙,曹端林摘要:石墨烯因其独特的结构和性能,成为物理化学和材料学界的研究热点。
本文综述了石墨烯复合材料的结构和分类,主要包括石墨烯-纳米粒子复合材料、石墨烯-聚合物复合材料和石墨烯-碳基材料复合材料。
并简述石墨烯复合材料在催化领域、电化学领域、生物医药领域和含能材料领域的应用。
关键词:石墨烯;复合材料;纳米粒子;含能材料Research and Application of Graphene compositesABSTRACT: Graphene has recently attracted much interest in physics,chemistry and material field due to its unique structure and properties. This paper reviews the structure and classification of graphene composites, mainly inclouding graphene-nanoparticles composites, graphene-polymer composites and graphene-carbonmaterials composites. And resume the application of graphene composites in the field of catalysis, electrochemistry, biological medicine and energetic materials.Keywords: graphene; composites; nanoparticles; energetic materials石墨烯自2004年曼彻斯特大学Geim[1-3]等成功制备出以来,因其独特的结构和性能,颇受物理化学和材料学界的重视。
Pt/石墨烯-TiO2纳米管的合成及光催化制氢活性
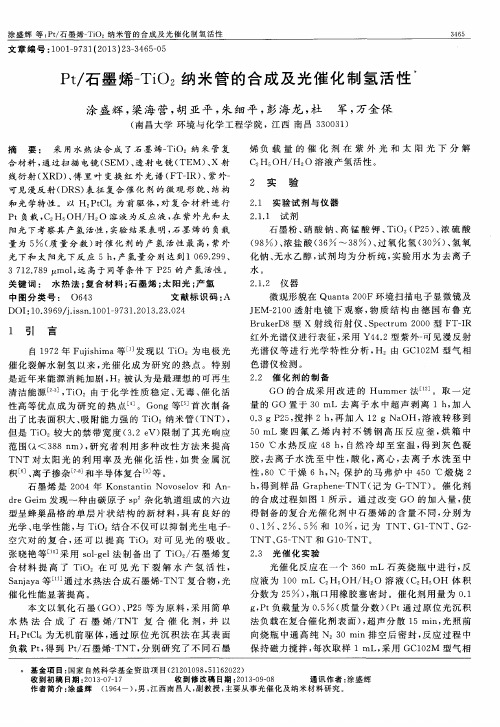
性 高等 优点 成 为 研 究 的热 点 l _ 4 ] 。Go n g等 [ 5 首 次 制备
出了 比表 面积大 、 吸附能 力 强 的 T i O。 纳米管( TNT) ,
0 . 3 g P 2 5 , 搅拌 2 h , 再加入 1 2 g Na OH, 溶 液转 移 到
涂 盛辉 等 : P t / 石 墨烯 一 Ti O 纳 米 管 的合 成 及 光 催 化 制 氢 活 性
文章 编号 : 1 0 0 1 — 9 7 3 1 ( 2 0 1 3 ) 2 3 — 3 4 6 5 — 0 5
P t / 石 墨 烯一 Ti O2 纳 米 管 的合 成 及 光 催 化 制氢 活 性
DoI : 1 0 . 3 9 6 9 / i . i s s n . 1 0 0 1 - 9 7 3 1 . 2 0 1 3 . 2 3 . 0 2 4
2 . 1 . 2 仪 器
微观 形貌 在 Qu a n t a 2 0 0 F环境 扫描 电子显 微镜 及 J E M一 2 1 0 0透射 电镜 下 观察 , 物 质 结 构 由德 国 布 鲁 克
2 . 2 催 化 剂 的 制 备
催 化裂 解水 制 氢 以来 , 光 催 化成 为研 究 的热 点 。特别
是 近年 来能 源消 耗加 剧 , H 被 认 为是 最理 想 的可 再生 清 洁能 源 。 ] , T i O 。由于 化 学 性 质 稳 定 、 无毒 、 催 化 活
GO 的合 成 采 用 改 进 的 Hu mme r 法口 。取 一 定
石墨烯是 2 0 0 4年 Ko n s t a n t i n No v o s e l o v和 An — d r e Ge i m 发 现一种 由碳 原子 s p 杂化 轨道组 成 的六 边 型 呈蜂 巢 晶格 的单 层 片 状 结 构 的 新 材料 , 具 有 良好 的 光学、 电学性 能 , 与 T i O 。 结 合 不仅 可 以抑制 光 生 电子一 空 穴对 的 复 合 , 还可 以提高 T i O 对 可 见 光 的 吸 收 。
石墨烯复合材料研究进展

石墨烯复合材料研究进展摘要:近年来石墨烯因其优良的力学、电学、热学和光学等特性, 且添加到基体材料中可以提高复合材料的性能,拓展其功能,因此石墨烯复合材料的制备成为研究热点之一。
本文介绍了国内外对石墨烯复合材料的研究,对石墨烯复合材料的研究进展及现状进行了详细的介绍,并对石墨烯复合材料的发展趋势进行了展望。
关键词:石墨烯;复合材料;研究进展一、引言石墨烯因其优异的物理性能和可修饰性, 受到国内外学者的广泛关注。
石墨烯的杨氏模量高达1TPa、断裂强度高达130GPa,是目前已知的强度性能最高的材料,同时是目前发现电阻率最小的材料, 只有约10-8Ω·m;拥有很高的电子迁移率,且具有较高的导热系数。
氧化石墨烯作为石墨烯的重要派生物,氧化石墨烯薄片在剪切力作用下很容易平行排列于复合材料中, 从而提高复合材料的性能。
本文总结介绍了几种常见的石墨烯复合材料。
二、石墨烯复合材料(1)石墨烯及氧化石墨烯复合材料膜聚乙烯醇(PVA)结构中有非常多的羟基,因此其能与水相互溶解,溶解效果很好。
GO和PVA都可以在溶液中形成均匀、稳定的分散体系。
干燥成型后,GO在PVA中的分散可以达到分子水平,GO表面丰富的含氧官能团可以与PVA的羟基形成氢键,因此添加少量的GO可以显著提高复合材料的力学性能。
樊志敏[1]等制备出了氧化石墨烯纳米带/TPU复合膜。
通过机械测试显示,当加入氧化石墨烯纳米带的量为2%时,复合薄膜的弹性模量和抗拉强度与不加氧化石墨烯纳米带的纯TPU薄膜相比都得到了非常大的提高,分别提高了160%和123%。
马国富[2]等人发现,在聚乙烯醇(PVA)和氧化石墨烯(GO)复合制备的得复合薄膜中,GO均匀的分散在PVA溶液中,PVA的羟基与GO表面的含氧基团发生相互作用复合而不分相。
加入GO之后,大大提高了复合膜的热稳定性,当加入的GO量为3%时,纳米复合膜力学性能测试出现最大值,此时断裂伸长率也出现了最大值,这表明在此GO含量时复合膜有最佳性能;与不加GO的纯PVA膜相比,当加入的GO量为3%时,耐水性也大大地提高。
【精品文章】几种石墨烯复合材料制备方法及催化应用介绍

几种石墨烯复合材料制备方法及催化应用介绍
石墨烯具有独特的热、电和光学性能,并以高的比表面积性能,使其非常适于用作复合材料的理想载体。
目前,石墨烯基复合材料广泛应用于传感器、新能源、光催化、电容器、生物材料等领域,特别是在在光催化和电催化领域,具有广阔应用前景。
下面小编介绍石墨烯复合材料在催化领域应用。
一、石墨烯/TiO2复合材料
1、石墨烯/TiO2复合材料光催化性能
石墨烯作为TiO2光催化材料的载体,不仅可以提高催化材料的比表面积和吸附性能,还能够抑制TiO2内部光生载流子的复合,降低了电子-空穴对的重组率,从而促进TiO2的光催化性能,提高其利用效率,因此制备TiO2/石墨烯复合材料可以进一步提高材料的光催化活性。
石墨烯/TiO2复合材料光催化机理示意图
2、石墨烯/TiO2复合材料制备方法
目前,石墨烯/TiO2复合材料的制备方法主要有溶胶-凝胶法和水热法等。
两种方法对于石墨烯的前体准备都是通过Hummers法得到氧化石墨烯,然后通过还原手段一步法得到还原氧化石墨烯/TiO2复合材料。
左图:石墨烯结构示意图;右图:氧化石墨烯结构示意图
(1)溶胶-凝胶法
溶胶-凝胶法通常是将钛的前体与氧化石墨烯溶液混合并搅拌均匀,氧化石墨烯通过氢键作用力与钛的前体结合并发生缩合、聚合反应最终形成具有Ti-O-Ti三维网络结构的凝胶,然后经过干燥、焙烧、研磨得到石墨烯。
石墨烯聚合物复合材料的研究现状及前景论文1 推荐
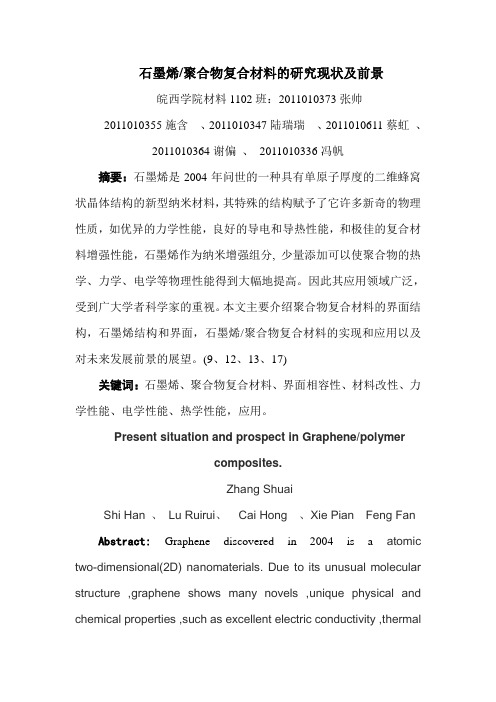
石墨烯/聚合物复合材料的研究现状及前景皖西学院材料1102班:2011010373张帅2011010355施含、2011010347陆瑞瑞、2011010611蔡虹、2011010364谢偏、2011010336冯帆摘要:石墨烯是2004年问世的一种具有单原子厚度的二维蜂窝状晶体结构的新型纳米材料,其特殊的结构赋予了它许多新奇的物理性质,如优异的力学性能,良好的导电和导热性能,和极佳的复合材料增强性能,石墨烯作为纳米增强组分, 少量添加可以使聚合物的热学、力学、电学等物理性能得到大幅地提高。
因此其应用领域广泛,受到广大学者科学家的重视。
本文主要介绍聚合物复合材料的界面结构,石墨烯结构和界面,石墨烯/聚合物复合材料的实现和应用以及对未来发展前景的展望。
(9、12、13、17)关键词:石墨烯、聚合物复合材料、界面相容性、材料改性、力学性能、电学性能、热学性能,应用。
Present situation and prospect in Graphene/polymercomposites.Zhang ShuaiShi Han 、Lu Ruirui、Cai Hong 、Xie Pian Feng Fan Abstract:Graphene discovered in 2004 is a atomic two-dimensional(2D)nanomaterials. Due to its unusual molecular structure ,graphene shows many novels ,unique physical and chemical properties ,such as excellent electric conductivity ,thermalconductivity ,thermal stability.Graphene as nano enhanced components, a small amount of added can make polymer thermal, mechanical, electrical and other physical properties are improved significantly.So its application field widely, have drawn the attention of the many scholars scientists.This paper mainly introduces the interface structure of polymer composite materials, graphene structure and interface, implementation and application of graphene/polymer composites as well as on the outlook for the future development prospect.Key words: Graphene,Polymer composite materials Material modification、Mechanical properties、Electrical performance、Thermal properties、application.一:石墨烯/聚合物的研究现状自年石墨烯发现以来,石墨烯的研究成果层出不穷,其中包括,生活领域,医用领域,电化学领域等。
石墨烯负载铂纳米粒子,抗坏血酸还原

高效、清洁的石墨烯负载的铂纳米团簇的合成在直接甲醇燃料电池的应用摘要石墨烯负载的铂纳米团簇是由一个高效、清洁的方法合成,使用这个方法时石墨烯氧化物和铂离子的前体会因为抗坏血酸而在一步法工艺中降低。
所得到的铂纳米团簇连接的石墨烯复合材料(PtNCs /石墨烯)通过X-射线衍射(XRD),场发射扫描电子显微镜(FE-SEM),透射电子显微镜(TEM)和能量色散X射线光谱(EDS)检测,可以直接显示,Pt纳米团簇可以成功的在石墨烯上形成,并很好地分布在石墨烯薄片的边缘和褶皱上。
进一步的电化学表征——包括循环伏安法(CV)和当前的方法表明:与普通的炭黑Vulcan XC-72和石墨负载Pt纳米团簇相比较,PtNCs /graphene具有明显更高的电催化活性和甲醇电氧化稳定性。
这将导致PtNCs/graphene进一步作为一种新的电极材料在直接甲醇燃料电池(DMFC)中的应用。
1.介绍几十年来,在直接甲醇燃料电池(DMFC)中,一直使用甲醇作为燃料而产生强化利益。
DMFC 与其他的燃料电池相比,主要的优点是它具有便携性、它所需的原料甲醇容易获得、它具有较高的能量密度——一个数量级大于压缩氢气。
然而甲醇交叉和中间物的毒效应,如一氧化碳(CO)对催化剂的毒性影响是影响DMFC在商业市场上应用的主要限制。
减少中间物产生的不良效应和提升催化剂效率是当前研究DMFC的主要问题。
在DMFC的甲醇氧化反应中主要选择铂作为催化剂,是由于它是在这个反应中催化活性最高的纯金属。
但是铂高昂的价格和自然界中的有限供应束缚了它在DMFC中的应用。
而铂不稳定的催化能力会产生具有毒效应的中间产物,是另外一个主要的障碍。
众所周知,催化剂的比活性主要取决于它们的分布和大小。
为了降低铂的负载和提高它的催化活性,可以控制铂的大小在纳米尺寸之类,这个方法也是最有效的。
由于具有很高的表面积体积比,拥有小而窄的分布特征的铂纳米粒子是高效电催化活性材料的理想当选者。
- 1、下载文档前请自行甄别文档内容的完整性,平台不提供额外的编辑、内容补充、找答案等附加服务。
- 2、"仅部分预览"的文档,不可在线预览部分如存在完整性等问题,可反馈申请退款(可完整预览的文档不适用该条件!)。
- 3、如文档侵犯您的权益,请联系客服反馈,我们会尽快为您处理(人工客服工作时间:9:00-18:30)。
This article appeared in a journal published by Elsevier.The attached copy is furnished to the author for internal non-commercial research and education use,including for instruction at the authors institutionand sharing with colleagues.Other uses,including reproduction and distribution,or selling or licensing copies,or posting to personal,institutional or third partywebsites are prohibited.In most cases authors are permitted to post their version of thearticle(e.g.in Word or Tex form)to their personal website orinstitutional repository.Authors requiring further informationregarding Elsevier’s archiving and manuscript policies areencouraged to visit:/copyrightElectrochimica Acta 56 (2011) 2712–2716Contents lists available at ScienceDirectElectrochimicaActaj o u r n a l h o m e p a g e :w w w.e l s e v i e r.c o m /l o c a t e /e l e c t a c taElectrochemical detection of hydroquinone by graphene and Pt-graphene hybrid material synthesized through a microwave-assisted chemical reduction processJing Li a ,b ,Chun-yan Liu a ,∗,Chao Cheng a ,baKey Laboratory of Photochemical Conversion and Optoelectronic Materials of Technical Institute of Physics and Chemistry,Chinese Academy of Sciences,Zhongguancun,Beijing 100190,PR China bGraduate School of the Chinese Academy of Sciences,Beijing 100806,PR Chinaa r t i c l e i n f o Article history:Received 17June 2010Received in revised form 17September 2010Accepted 14December 2010Available online 21 December 2010Keywords:Hydroquinone GraphenePt-graphene hybrid material Electrochemical detectionDifferential pulse voltammetrya b s t r a c tWe have synthesized graphene and Pt-graphene hybrid material by a microwave-assisted chemical reduction process and evaluated their application as electrode materials towards the electrochemical detection of hydroquinone.Graphene modified glass carbon electrode (GCE)showed a good performance for detecting hydroquinone due to the unique properties of graphene which increased the active surface area of the electrode and accelerated the electron transfer.The linear detection range of hydroquinone concentration was 20–115M with a sensitivity of 1.38A M −1cm −2;the detection limit was esti-mated to be 12M (S/N =3).The electrocatalytic activity of the Pt-graphene modified GCE was further improved due to the enhanced electron transfer and the linear detection range was 20–145M with the sensitivity of 3.56A M −1cm −2,detection limit 6M (S/N =3).© 2010 Elsevier Ltd. All rights reserved.1.IntroductionHydroquinone is a phenolic compound which is important in a wide number of biological and industrial processes such as coal–tar production,paper manufacturing and photographic developers,and is considered as an important xenobiotic micropollutant [1].Several analytical methods have been used to detect hydroquinone,including high performance liquid chromatography [2,3],flow injection analysis [4,5],spectrophotometry [6,7],and electrochemi-cal methods [8,9],among which the electrochemical methods have attracted great attentions owing to the advantages such as effi-ciency,simplicity and quick response.In previous work,several carbon-based materials such as mesoporous carbon [10],boron-doped diamond [11],conductive carbon cement [12],carbon fibre [13],and carbon nanotubes (CNTs)[14]have been explored for the electrochemical detection of hydroquinone.Graphene (G),a monolayer of carbon atoms packed into a dense,honeycomb crystal structure as well as being a fundamental build-ing block for fullerenes,carbon nanotubes,and graphite [15],has shown fascinating properties and holds the promise for future carbon-based device architectures [16–19].Recently,graphene-based materials have been explored for electrochemical sensor due to its large surface area,extraordinary electronic transport prop-∗Corresponding author.Tel.:+8601082543573;fax:+8601062554670.E-mail address:cyliu@ (C.-y.Liu).erties,strong mechanical strength,and its lower cost and easier preparation in mass quantities compared with carbon nanotubes,typical examples including detection of glucose by metal deco-rated graphene [20]and nitrogen-doped graphene [21]modified glass carbon electrode,electrochemical detection of paracetamol by graphene modified glass carbon electrode [22],and selective detection of dopamine by graphene modified glass carbon elec-trode [23].However,to the best of our knowledgement,there is no report about the eletrochemical detection of hydroquinone by graphene-based materials.Up to now,numerous methods have been developed to synthe-size graphene such as micromechanical cleavage of graphite [24],chemical vapor deposition technique [25],epitaxial growth on a single-crystal silicon carbide by vacuum graphitization [26],and chemical reduction of graphene oxide [27–31].Among them,the chemical reduction of graphene oxide,involving graphite oxida-tion,exfoliation and reduction,is the most efficient approach to bulk production of graphene-based sheets at low cost.In this con-text,we synthesized graphene and Pt-graphene hybrid material by a microwave-assisted chemical reduction process and evaluated their application to electrochemical detection of hydroquinone.2.Experimental2.1.Preparation of grapheneGraphene was prepared through a microwave-assisted chemi-cal reduction of graphene oxide.In a typical procedure,graphite0013-4686/$–see front matter © 2010 Elsevier Ltd. All rights reserved.doi:10.1016/j.electacta.2010.12.046J.Li et al./Electrochimica Acta56 (2011) 2712–27162713oxide(25mg)prepared from natural graphite powders(Beijing Chemical Factory of China)by Hummer’s method[32]was son-icated in water(50mL)for2h using a JK-300ultrasonic cleaner (300W,40kHz)to achieve a clear,brown dispersion of graphene oxide(0.5mg/mL).5mL of hydrazine hydrate(80%)was added into the graphene oxide and kept stirring for3h at100◦C in a microwave oven with program-control(Sineo MAS-II).The heat-ing power was set to300W at the beginning of the reaction and automatically reduced to as low as about10W when a given tem-perature reached.The stirring rate was set to700rps.The black graphene was obtained after centrifugation,washed with water and ethanol,and naturally dried in air.2.2.Preparation of Pt-graphene hybrid materialThe route to prepare Pt-graphene hybrid material was similar to graphene,except that5mL of hexachloroplatinic acid solution (0.0193M)was added to the graphene oxide solution and kept stirring for15min at room temperature before the addition of hydrazine hydrate.2.3.Preparation of graphene and Pt-graphene modified glass carbon electrodePrior to modification,the glassy carbon electrodes(GCE)of3mm diameter were polished with0.5m alumina powder,washed with deionized water and then dried in air.Graphene-based materials were dispersed in N,N-dimethylformamide(DMF)by sonication for30min to achieve a2mg/mL graphene-DMF suspension.Then, 5L of the suspension was coated onto GCE and allowed to dry in air.2.4.CharacterizationThe AFM images were recorded using a Multimode Nanoscope III a AFM(Veeco Metrology LLC,Santa Barbara,CA).Tapping-mode imaging was applied to provide the largest amount of structural detail of the graphene oxide sheets as well as to prevent transloca-tion of the sheets on the surface by the tip(Veeco MP-11100silicon cantilevers,force constant k=60N/m,radius of curvature r=10nm, and resonance frequency f=300kHz).The sample was prepared by depositing graphene oxide dispersion in water(0.05mg/mL) onto a new cleaved mica surface and dried under vacuum at room temperature.The TEM images were taken with a JEM2100F trans-mission electron microscope,by using an accelerating voltage of 200kV.The samples were dispersed in deionized water by sonica-tion and dropped onto a conventional carbon-coated copper grid. The XRD pattern was obtained with a Bruker D8Focus under Cu K␣radiation at1.54056˚A with a scanning speed of4◦min−1.The XPS measurements were performed on a MICROLAB MK II spec-trophotometer with Mg K␣radiation.UV–vis absorption spectra were recorded with a Shimadzu UV-1601PC spectrophotome-ter.The samples were dispersed in deionized water for optical measurements.Differential pulse voltammetry(DPV)was car-ried out at room temperature with a CHI660C workstation(CH Instruments,Chenhua,Shang-hai,China)connected to a personal computer.A three-electrode configuration was employed,con-sisting of a modified glassy carbon electrode(3mm in diameter) serving as the working electrode,saturated calomel electrode and platinum wire serving as the reference and counter electrodes, respectively.3.Results and discussionAFM images of graphene oxide revealed the presence of sheets with thickness of1.199nm(Fig.1),which wascharacter-Fig.1.AFM images and height profile of graphene oxide.istic of a fully exfoliated graphene oxide sheet[27,33,34].Such thickness was larger than that of single-layer pristine graphene (0.34nm),due to the presence of oxygen-containing functional groups attached on both sides of the graphene sheet and the dis-placement of the sp3-hybridized carbon atoms slightly above and below the original graphene plane[27].The TEM images of graphene sheets and Pt-graphene hybrid material were shown in Fig.2,where the transparent graphene sheets with crumpled silk veil waves on the top of the carbonfilm were observed(Fig.2a),and the rumples was intrinsic to graphene nanosheets[35].A large number of Pt nanoparticles were sup-ported on graphene sheets and few particles resided outside of the support(Fig.2b),which indicated the strong interaction between the Pt nanoparticles and the graphene sheets.The size distribution of Pt nanoparticles was8–45nm(Fig.2c).In Fig.3,graphite showed a sharp diffraction peak at26.2◦cor-responding to(002)plane with d-spacing of0.34nm(Fig.3a). Compared with the graphite,the feature diffraction peak of graphite oxide appeared at10.4◦corresponding to d-spacing of 0.85nm(Fig.3b),which was larger than that of graphite due to the intercalated water molecules between layers[36].After the exfo-liation of graphite oxide by ultrasonic vibration and subsequent chemical reduction,the obtained graphene showed a broadened diffraction peak at24.5◦(Fig.3c),meaning the layers of graphite along c-axis were exfoliated and the carbon sp2bond were restored. The XRD pattern of Pt-graphene hybrid material is shown in Fig.3d. Apart from the peak at24.5◦assigned to graphene,all other peaks can be indexed to Pt nanoparticles(face-centered cubic,JCPDS04-0802).2714J.Li et al./Electrochimica Acta56 (2011) 2712–2716Fig.2.TEM images of (a)graphene sheets and (b)Pt-graphene hybrid material,(c)the size distribution of supported Pt nanoparticles.Compared with graphite oxide,the C 1s XPS spectrum of graphene at 286–289eV corresponding to oxygenated carbon showed a significant decrease (Fig.4a),confirming that most of the epoxide,hydroxyl,and carboxyl functional groups were success-fully removed through the reduction process.This observation was in agreement with that found in previous studies [27,31].Fig.4b represented the XPS signature of the Pt 4f doublet (4f 7/2and 4f 5/2)for the Pt nanoparticles supported on graphene sheets.The Pt 4f 7/2and Pt 4f 5/2peaks appeared at 70.1eV and 73.35eV,respectively,which shifted remarkably to the lower binding energy compared with the standard binding energy of Pt 4f 7/2and Pt 4f 5/2for Pt 0state (70.83eV and 74.23eV)[37]due to the electron transfer from the graphene sheet to Pt nanoparticles.Because the work func-tion of graphene (4.48eV)[38]is smaller than that of Pt (5.65eV)[39],electron transfer from the graphene sheets to Pt nanoparticles would occurred during the formation of the Pt-graphene hybridstructures.Fig.3.XRD patterns of (a)graphite,(b)graphite oxide,(c)graphene,and (d)Pt-graphene hybrid material.The UV–vis spectrum of GO exhibited two characteristic peaks,one was at 230nm corresponding to →*transitions of aromatic C–C bonds,and a shoulder at 303nm was attributed to n →*transitions of C O bonds (Fig.5a)[40].The peak at 230nm was red shift to 268nm after the chemical reduction treatment (Fig.5b and c),which was an indication of the restoration of the electronic conjugation within the G sheets [41].The activity of the graphene modified GCE and Pt-graphene modified GCE towards electrochemical detection of hydroquinone were investigated by differential pulse voltammetry (DPV).The GCE exhibited a weak and broad peak at 0.09V correspondingtoFig.4.(a)C 1s XPS spectra of graphite oxide and graphene,and (b)Pt 4f spectra of Pt-graphene.J.Li et al./Electrochimica Acta 56 (2011) 2712–27162715Fig.5.UV–vis absorption spectra of (a)graphene oxide,(b)graphene,and (c)Pt-graphene hybrid material.the oxidation of hydroquinone (curve a in Fig.6A).The graphene-modified GCE displayed a well-defined peak at 0.002V with a much higher current intensity as compared with the GCE (the curve b in Fig.6A),indicating the enhanced electrocatalytic activ-ity towards hydroquinone,which could be due to the unique properties of graphene that increased the active surface area of the electrode and accelerated the electron transfer via improved conductivity and the good affinity of graphene to hydroquinone.When Pt nanoparticles were combined with graphene,the cur-rent density further increased,maybe due to the enhanced electron transfer in Pt-graphene hybrid system,which was attributed to the charge hopping through the metallic Pt nanoparticles andthe effective charge migration through the graphene.The effec-tive transport of the electrons to the electrode in the Pt-graphene matrix led to the efficient electrocatalytic oxidation of hydro-quinone.We can observe in Fig.6B and C that the peak current increased with the increase of the hydroquinone concentration (from the curve a to f).It could be seen from the curve a in Fig.6D that the current at graphene modified GCE linearly increased with the increase of the concentration of hydroquinone over the 20–115M range with sensitivity of 1.38A M −1cm −2;and the detection limit was estimated to be 12M (S/N =3).For Pt-graphene modified GCE (the curve b in Fig.6D),a lin-ear detection range was from 20M to 145M with sensitivity of 3.56A M −1cm −2,the detection limit 6M (S/N =3).Addi-tionally,the current intensity at the Pt-graphene modified GCE was higher than that at the graphene modified GCE in the whole concentration range.These results indicated that the Pt-graphene modified GCE showed higher current intensity,lower detection limit,and higher sensitivity towards electrochemical detection of hydroquinone compared with the pure graphene modified GCE,possibly due to the enhanced electron transfer in the Pt-graphene hybrid system.The applicability of the graphene modified GCE to the selective detection of hydroquinone in the presence of phenol was stud-ied using DPV.Phenol and hydroquinone showed respectively an oxidation peak at 0.218eV (Fig.7a)and 0.002eV (Fig.7b).For a mixed solution of 0.15mM hydroquinone and 10mM phenol,there were two well-distinguished peaks at the potential of 0.002eV and 0.218eV,corresponding to the oxidation of hydroquinone and phenol,respectively (Fig.7c),indicating that hydroquinone can be selectively detected in the presence of large concentration of phe-nol.Fig.6.DPV obtained at GCE (a),graphene modified GCE (b),and Pt-graphene modified GCE (c),using 115M hydroquinone in 0.05M (pH 7.4)phosphate buffer solution (A).DPV obtained at graphene modified GCE (B)and Pt-graphene modified GCE (C)with various concentration of hydroquinone:(a)20M,(b)29M,(c)65M,(d)83M,(e)115M and (f)145M in 0.05M (pH 7.4)phosphate buffer.DPV conditions:pulse amplitude =0.05V,sample width =0.0167s,pulse width =0.15s,pulse period =0.4s,and quiet time =2s.(D)Calibration plots of background subtracted peak current at graphene modified GCE (a)and Pt-graphene modified GCE (b)versus the concentration of hydroquinone.2716J.Li et al./Electrochimica Acta56 (2011) 2712–2716Fig.7.DPV obtained at graphene modified GCE:(a)10mM phenol,(b)0.15mM hydroquinone,and(c)10mM phenol and0.15mM hydroquinone in0.05M(pH7.4) phosphate buffer.DPV condition:pulse amplitude=0.05V,sample width=0.0167s, pulse width=0.15s,pulse period=0.4s,and quiet time=2s.4.ConclusionsIn conclusion,we have synthesized graphene and Pt-graphene hybrid material through a microwave-assisted chemical reduction process.The resulting graphene and Pt-graphene hybrid materials have been used for the electrochemical detection of hydroquinone. Graphene modified GCE showed a good performance for detect-ing hydroquinone due to the unique properties of graphene,which increased the active surface area of electrode and accelerated the electron pared with the pure graphene modified GCE, the electrocatalytic activity of the Pt-graphene hybrid material was further improved possibly due to the enhanced electron transfer in the Pt-graphene hybrid system.AcknowledgementsThe author thanks the support of Chinese Academy of Sciences and973Program.References[1]H.Cui,C.He,G.J.Zhao,J.Chromatogr.A855(1999)171.[2]C.H.Lin,J.Y.Sheu,H.L.Wu,Y.L.Huang,J.Pharm.Biomed.Anal.38(2005)414.[3]N.A.Penner,P.N.Nesterenko,Analyst125(2000)1249.[4]M.E.Rueda,L.A.Sarabia,A.Herrero,M.C.Ortiz,Anal.Chim.Acta479(2003)173.[5]G.N.Chen,J.S.Liu,J.P.Duan,H.Q.Chen,Talanta53(2000)651.[6]L.P.Jia,A.M.Zhang,Chin.J.Anal.Chem.31(2003)1508.[7]T.Gajewska,A.Kaszuba,Polimery44(1999)690.[8]I.C.Vieira,O.Fatibello-Filho,Talanta52(2000)681.[9]L.H.Wang,Analyst120(1995)2241.[10]Y.Hou,L.P.Guo,G.Wang,J.Electroanal.Chem.617(2008)211.[11]D.Sopchak,ler,Y.Avyigal,R.Kalish,J.Electroanal.Chem.538(2002)39.[12]X.J.Huang,J.J.Pot,W.Th.Kok,Anal.Chim.Acta300(1995)5.[13]R.M.Carvalho,C.Mello,L.T.Kubota,Anal.Chim.Acta420(2000)109.[14]Y.P.Ding,W.L.Liu,Q.S.Wu,X.G.Wang,J.Electroanal.Chem.575(2005)275.[15]A.K.Geim,K.S.Novoselov,Nat.Mater.6(2007)183.[16]T.Takamura,K.Endo,L.Fu,Y.P.Wu,K.J.Lee,T.Matsumoto,Eletrochim.Acta53(2007)1055.[17]S.Gilje,S.Han,M.Wang,K.L.Wang,R.B.Kaner,Nano Lett.7(2007)3394.[18]J.S.Bunch,A.M.van der Zande,S.S.Verbridge,I.W.Frank,D.M.Tanenbaum,J.M.Parpia,H.G.Craighead,P.L.McEuen,Science315(2007)490.[19]A.Sakhaee-Pour,M.T.Ahmadian,A.Vafai,Solid State Commun.147(2008)336.[20]T.T.Baby,S.S.J.Aravind,T.Arockiadoss,R.B.Rakhi,S.Ramaprabhu,Sens.Actu-ators B:Chem.145(2010)71.[21]Y.Wang,Y.Y.Shao,D.W.Matson,J.H.Li,Y.H.Lin,ACS Nano4(2010)1790.[22]X.H.Kang,J.Wang,H.Wu,J.Liu,I.A.Aksay,Y.H.Lin,Talanta81(2010)754.[23]Y.Wang,Y.M.Li,L.H.Tang,J.Lu,J.H.Li,mun.11(2009)889.[24]K.S.Novoselov,A.K.Geim,S.V.Morozov,D.Jiang,Y.Zhang,S.V.Dubonos,I.V.Grigorieva,A.A.Firsov,Science306(2004)666.[25]N.G.Shang,P.Papakonstantinou,M.McMullan,M.Chu,A.Stamboulis,A.Potenza,S.S.Dhesi,H.Machetto,Adv.Funct.Mater.18(2008)3506.[26]A.Charrier,A.Coati,T.Argunova,Y.Garreau,R.Pinchaux,I.Forbeaux,J.M.Debever,M.Sauvage-Simkin,J.M.Themlin,J.Appl.Phys.92(2002)2479. [27]S.Stankovich,D.A.Dikin,R.D.Piner,K.A.Kohlhaas,A.Kleinhammes,Y.Y.Jia,Y.Wu,S.T.Nguyen,R.S.Ruoff,Carbon45(2007)1558.[28]H.L.Guo,X.F.Wang,Q.Y.Qian,F.B.Wang,X.H.Xia,ACS Nano3(2009)2653.[29]G.X.Wang,J.Yang,J.Park,X.L.Gou,B.Wang,H.Liu,J.Yao,J.Phys.Chem.C112(2008)8192.[30]H.L.Wang,J.T.Robinson,X.L.Li,H.J.Dai,J.Am.Chem.Soc.131(2009)9910.[31]Y.Zhou,Q.L.Bao,L.A.L.Tang,Y.L.Zhong,K.P.Loh,Chem.Mater.21(2009)2950.[32]W.Hummers,R.Offeman,J.Am.Chem.Soc.80(1958)1339.[33]S.Stankovich,R.D.Piner,X.Chen,N.Wu,S.T.Nguyen,R.S.Ruoff,J.Mater.Chem.16(2006)155.[34]Y.Xu,H.Bai,G.Lu,C.Li,G.Shi,J.Am.Chem.Soc.130(2008)856.[35]J.C.Meyer,A.K.Geim,M.I.Katsnelson,K.S.Novoselov,T.J.Booth,S.Roth,Nature446(2007)60.[36]A.Buchsteiner,A.Lerf,J.Pieper,J.Phys.Chem.B110(2006)22328.[37]J.F.Moudler,W.F.Stickle,P.E.Sobol,K.D.Bomben,Handbook of X-ray Photo-electron Spectroscopy,PerkinElmer,Eden Prairie,MN,1992.[38]X.J.Wu,X.C.Zeng,Nano Lett.9(2009)250.[39]H.B.Michaelson,J.Appl.Phys.48(1977)4729.[40]J.I.Paredes,S.Villar-Rodil,A.Martınez-Alonso,J.M.D.Tascón,Langmuir24(2008)10560.[41]D.Li,M.B.Muller,S.Gilje,R.B.Kaner,G.G.Wallace,Nat.Nanotechnol.3(2008)101.。