土木工程外文翻译70683
土木工程专业外文翻译
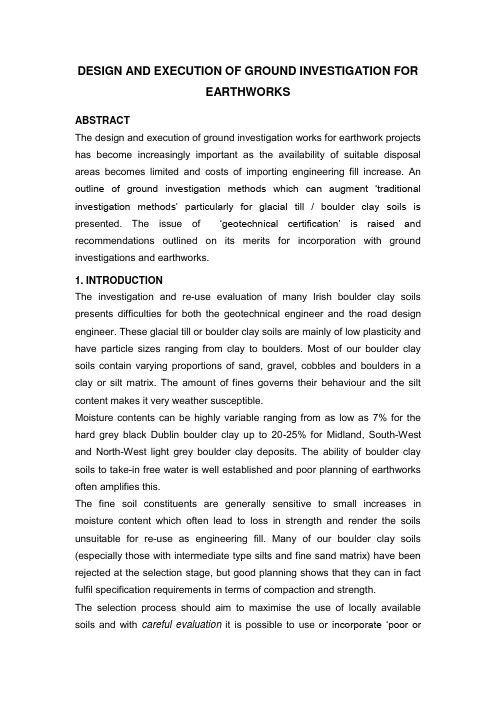
DESIGN AND EXECUTION OF GROUND INVESTIGATION FOREARTHWORKSABSTRACTThe design and execution of ground investigation works for earthwork projects has become increasingly important as the availability of suitable disposal areas becomes limited and costs of importing engineering fill increase. An outline of ground investigation methods which can augment ‘traditional investigation methods’ particularly for glacial till / boulder clay soils is presented. The issue of ‘geotechnical certification’ is raised an d recommendations outlined on its merits for incorporation with ground investigations and earthworks.1. INTRODUCTIONThe investigation and re-use evaluation of many Irish boulder clay soils presents difficulties for both the geotechnical engineer and the road design engineer. These glacial till or boulder clay soils are mainly of low plasticity and have particle sizes ranging from clay to boulders. Most of our boulder clay soils contain varying proportions of sand, gravel, cobbles and boulders in a clay or silt matrix. The amount of fines governs their behaviour and the silt content makes it very weather susceptible.Moisture contents can be highly variable ranging from as low as 7% for the hard grey black Dublin boulder clay up to 20-25% for Midland, South-West and North-West light grey boulder clay deposits. The ability of boulder clay soils to take-in free water is well established and poor planning of earthworks often amplifies this.The fine soil constituents are generally sensitive to small increases in moisture content which often lead to loss in strength and render the soils unsuitable for re-use as engineering fill. Many of our boulder clay soils (especially those with intermediate type silts and fine sand matrix) have been rejected at the selection stage, but good planning shows that they can in fact fulfil specification requirements in terms of compaction and strength.The selection process should aim to maximise the use of locally available soils and with careful evaluation it is possible to use o r incorporate ‘poor ormarginal soils’ within fill areas and embankments. Fill material needs to be placed at a moisture content such that it is neither too wet to be stable and trafficable or too dry to be properly compacted.High moisture content / low strength boulder clay soils can be suitable for use as fill in low height embankments (i.e. 2 to 2.5m) but not suitable for trafficking by earthwork plant without using a geotextile separator and granular fill capping layer. Hence, it is vital that the earthworks contractor fully understands the handling properties of the soils, as for many projects this is effectively governed by the trafficability of earthmoving equipment.2. TRADITIONAL GROUND INVESTIGATION METHODSFor road projects, a principal aim of the ground investigation is to classify the suitability of the soils in accordance with Table 6.1 from Series 600 of the NRA Specification for Road Works (SRW), March 2000. The majority of current ground investigations for road works includes a combination of the following to give the required geotechnical data:▪Trial pits▪Cable percussion boreholes▪Dynamic probing▪Rotary core drilling▪In-situ testing (SPT, variable head permeability tests, geophysical etc.) ▪Laboratory testingThe importance of ‘phasing’ the fieldwork operations cannot be overstressed, particularly when assessing soil suitability from deep cut areas. Cable percussion boreholes are normally sunk to a desired depth or ‘refusal’ with disturbed and undisturbed samples recovered at 1.00m intervals or change of strata.In many instances, cable percussion boring is unable to penetrate through very stiff, hard boulder clay soils due to cobble, boulder obstructions. Sample disturbance in boreholes should be prevented and loss of fines is common, invariably this leads to inaccurate classification.Trial pits are considered more appropriate for recovering appropriate size samples and for observing the proportion of clasts to matrix and sizes of cobbles, boulders. Detailed and accurate field descriptions are therefore vitalfor cut areas and trial pits provide an opportunity to examine the soils on a larger scale than boreholes. Trial pits also provide an insight on trench stability and to observe water ingress and its effects.A suitably experienced geotechnical engineer or engineering geologist should supervise the trial pitting works and recovery of samples. The characteristics of the soils during trial pit excavation should be closely observed as this provides information on soil sensitivity, especially if water from granular zones migrates into the fine matrix material. Very often, the condition of soil on the sides of an excavation provides a more accurate assessment of its in-situ condition.3. ENGINEERING PERFORMANCE TESTING OF SOILSLaboratory testing is very much dictated by the proposed end-use for the soils. The engineering parameters set out in Table 6.1 pf the NRA SRW include a combination of the following:▪Moisture content▪Particle size grading▪Plastic Limit▪CBR▪Compaction (relating to optimum MC)▪Remoulded undrained shear strengthA number of key factors should be borne in mind when scheduling laboratory testing:▪Compaction / CBR / MCV tests are carried out on < 20mm size material.▪Moisture content values should relate to < 20mm size material to provide a valid comparison.▪Pore pressures are not taken into account during compaction and may vary considerably between laboratory and field.▪Preparation methods for soil testing must be clearly stipulated and agreed with the designated laboratory.Great care must be taken when determining moisture content of boulder clay soils. Ideally, the moisture content should be related to the particle size andhave a corresponding grading analysis for direct comparison, although this is not always practical.In the majority of cases, the MCV when used with compaction data is considered to offer the best method of establishing (and checking) the suitability characteristics of a boulder clay soil. MCV testing during trial pitting is strongly recommended as it provides a rapid assessment of the soil suitability directly after excavation. MCV calibration can then be carried out in the laboratory at various moisture content increments. Sample disturbance can occur during transportation to the laboratory and this can have a significant impact on the resultant MCV’s.IGSL has found large discrepancies when performing MCV’s in the field on low plasticity boulder clays with those carried out later in the laboratory (2 to 7 days). Many of the aforementioned low plasticity boulder clay soils exhibit time dependant behaviour with significantly different MCV’s recorded at a later date – increased values can be due to the drainage of the material following sampling, transportation and storage while dilatancy and migration of water from granular lenses can lead to deterioration and lower values.This type of information is important to both the designer and earthworks contractor as it provides an opportunity to understand the properties of the soils when tested as outlined above. It can also illustrate the advantages of pre-draining in some instances. With mixed soils, face excavation may be necessary to accelerate drainage works.CBR testing of boulder clay soils also needs careful consideration, mainly with the preparation method employed. Design engineers need to be aware of this, as it can have an order of magnitude difference in results. Static compaction of boulder clay soils is advised as compaction with the 2.5 or 4.5kg rammer often leads to high excess pore pressures being generated – hence very low CBR values can result. Also, curing of compacted boulder clay samples is important as this allows excess pore water pressures to dissipate.4. ENGINEERING CLASSIFICATION OF SOILSIn accordance with the NRA SRW, general cohesive fill is categorised in Table 6.1 as follows:▪2A Wet cohesive▪2B Dry cohesive▪2C Stony cohesive▪2D Silty cohesiveThe material properties required for acceptability are given and the design engineer then determines the upper and lower bound limits on the basis of the laboratory classification and engineering performance tests. Irish boulder clay soils are predominantly Class 2C.Clause 612 of the SRW sets out compaction methods. Two procedures are available:▪Method Compaction▪End-Product CompactionEnd product compaction is considered more practical, especially when good compaction control data becomes available during the early stages of an earthworks contract. A minimum Target Dry Density (TDD) is considered very useful for the contractor to work with as a means of checking compaction quality. Once the material has been approved and meets the acceptability limits, then in-situ density can be measured, preferably by nuclear gauge or sand replacement tests where the stone content is low.As placing and compaction of the fill progresses, the in-situ TDD can be checked and non-conforming areas quickly recognised and corrective action taken. This process requires the design engineer to review the field densities with the laboratory compaction plots and evaluate actual with ‘theoretical densities’.5. SUPPLEMENTARY GROUND INVESTIGATION METHODS FOR EARTHWORKSThe more traditional methods and procedures have been outlined in Section 2. The following are examples of methods which are believed to enhance ground investigation works for road projects:▪Phasing the ground investigation works, particularly the laboratory testing▪Excavation & sampling in deep trial pits▪Large diameter high quality rotary core drilling using air-mist or polymer gel techniques译文:土方工程的地基勘察与施工摘要:当工程场地的处理面积有限且填方工程费用大量增加时,土方工程的地基勘察设计与施工已逐渐地变得重要。
土木工程 外文资料翻译(通用)

淮阴工学院毕业设计外文资料翻译学院:建筑工程学院专业:土木工程(路桥方向)姓名:石洋学号:1081401526外文出处:工程力学杂志(用外文写)Journal of Engineering Mechanics 附件: 1.外文资料翻译译文;2.外文原文。
注:请将该封面与附件装订成册。
附件1:外文资料翻译译文Timoshenko 和剪切模型梁的动力学研究Noël Challamel1摘要:古典Timoshenko 梁模型和剪切梁模型常用于建筑行为模型都剪稳定性或动态分析。
该技术关注的是两种模型间的大量弯曲剪切刚度值的问题。
这是以两种模型分析研究了简支梁。
获得大量弯曲剪切刚度值的渐进解。
在一般情况下,实验在考虑大弯剪刚度值参数时证明该剪切梁模型不能从Timoshenko 模型中推断出来,这只是达到特定的几何参数在目前的例子。
作为结论,剪切模型的能力近似Timoshenko 模型,因为大量弯曲剪切刚度参数是坚定的依赖于横截面在边界状态下的材料和几何特性。
关键词:横波,结构力学,动态模型,脑电图仪,比较研究。
引言:经典的Timoshenko 梁模型和剪切梁模型经常被用来模拟建筑物的剪切稳定性和动态特性。
该技术关注的是两种模型间的大量弯曲剪切刚度值的问题。
2004年Aristizabal-Ochoa 通过考虑大量无维参数来比较这两种模型出一种关系,屈服于剪切刚度参数。
这项科学证据表明一个简单的例子这个参数可能不足以联系这两种理论。
Timoshenko 模型动态方程: Timoshenko 模型的控制方程是:x∂θ∂EI -)θ-x ∂y ∂(G A -t ∂θ∂r m 0x∂θ∂G A x ∂y ∂G A -t ∂∂m 22S 222S 22S 2y 2==+ (1) 这种横梁只在杨氏模量和横断面剪切模量下用均匀的弹性材料制成的。
它的横向的横截面是带有一个用A S 和一个重要的惯性矩表示的有效的剪切区域双重对称的I =Ar 2。
土木工程外文翻译70683
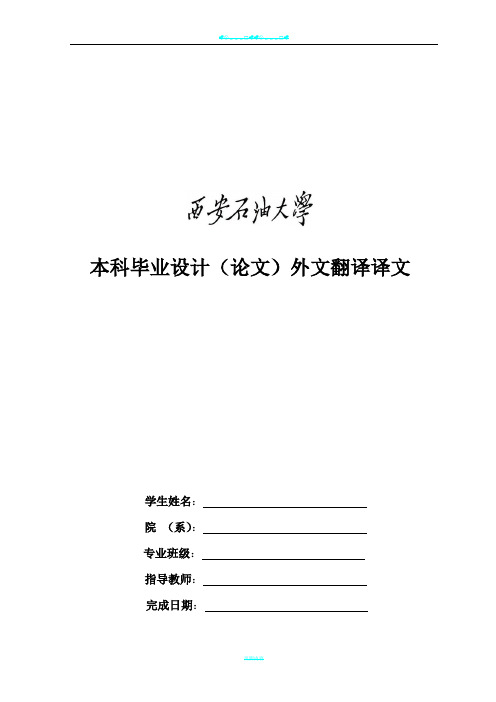
本科毕业设计(论文)外文翻译译文学生姓名:院 (系):专业班级:指导教师:完成日期:钢筋混凝土填充框架结构对拆除两个相邻的柱的响应作者: 美国波士顿东北大学,斯奈尔 设计中心收稿日期: 年 月 日,修整后收稿日期 年 月 日,录用日期 年 月 日,网上上传日期 年 月 日。
摘要:本文是评价圣地亚哥旅馆对同时拆除两根相邻的外柱的响应问题,圣地亚哥旅馆是个 层钢筋混凝土填充框架结构。
结构的分析模型应用了有限元法和以此为基础的分析模型来计算结构的整体和局部变形。
分析结果跟实验结果非常吻合。
当测量的竖向位移增加到为四分之一英寸(即 )的时候,结构就发生连续倒塌。
通过实验分析方法评价和讨论随着柱的移除而产生的变形沿着结构高度上的发展和荷载动态重分配。
讨论了轴向和弯曲的变形传播的不同。
结构横向和纵向的三维桁架在填充墙的参与下被认为是荷载重分配的主要构件。
讨论了两种潜在的脆性破坏模型(没有拉力加强的梁的脆断和有加筋肋的梁的挤出)。
分析评价了结构对额外的重力和无填充墙时的响应。
有限责任公司对此文保留所有权利。
关键词:连续倒塌;荷载重分配;对荷载抵抗能力;动态响应;非线性分析;脆性破坏。
介绍:作为减小由于结构的局部损坏而造成大量伤亡的可能性措施的一部分,美国总务管理局【 】和国防部【 】出台了一系列制度来评价结构对连续倒塌的抵抗力。
【 】定义连续倒塌为,由原始单元的局部破坏在单元间的扩展最终造成结构的整体或不成比例的大部破坏。
通过 和 【 】建议的方法, 定义了两种一般模型来减小结构设计时连续倒塌效应产生的损害,它们分为直接和间接的设计方法。
一般建筑规范和标准用增加结构的整体性的间接设计方法。
间接设计法也应用于美国国防部的降低连续倒塌设计和未归档设备标准中。
尽管间接设计法可以降低连续破坏的风险【 , 】,对基于此法设计的结构破坏后的表现的判断是不容易实现的。
有一种基于直接设计的方法通过研究瞬间消除受载构件,比如柱子,对结构的影响来评价结构的连续倒塌。
土木工程概论外文翻译.doc

外文原文 2Civil engineering introduction papersAbstract: the civil engineering is a huge discipline, but the main one is building, building whether in China or abroad, has a long history, long-term development process. The world is changing every day, but the building also along with the progress of science and development. Mechanics findings, material of update, ever more scientific technology into the building. But before a room with a tile to cover the top of the house, now for comfort, different ideas, different scientific, promoted the development of civil engineering, making it more perfect.Key words: civil engineering;Architecture;Mechanics;Materials Civil engineering is building various projects collectively. It was meant to be and "military project" corresponding. In English the history of Civil Engineering, mechanical Engineering, electrical Engineering, chemical Engineering belong to Engineering, because they all have Min Yong Xing. Later, as the project development of science and technology, mechanical, electrical, chemical has gradually formed independent scientific, to Engineering became Civil Engineering of specialized nouns. So far, in English, to Engineering include water conservancy project, port engineering, while in our country, water conservancy projects and port projects also become very close and civil engineering relatively independent branch. Civil engineering construction of object, both refers to that built on the ground, underground water engineering facilities, also refers to applied materials equipment and conduct of the investigation, design and construction, maintenance, repair and other professional technology.Civil engineering is a kind of with people's food, clothing, shelter and transportation has close relation of the project. Among them with "live" relationship is directly. Because, to solve the "live" problem,must build various types of buildings. To solve the "line, food and clothes" problem both direct side, but also a indirect side. "Line", must build railways, roads, Bridges, "Feed", must be well drilling water, water conservancy, farm irrigation, drainage water supply for the city that is direct relation. Indirectly relationship is no matter what you do, manufacturing cars, ships, or spinning and weaving, clothing, or even production steel, launch satellites, conducting scientific research activities are inseparable from build various buildings, structures and build all kinds of project facilities.Civil engineering with the progress of human society and development, yet has evolved into large-scale comprehensive discipline, it has out many branch, such as: architectural engineering, the railway engineering, road engineering, bridge engineering, special engineering structure, water and wastewater engineering, port engineering, hydraulic engineering, environment engineering disciplines. [1] Civil engineering as an important basic disciplines, and has its important attributes of: integrated, sociality, practicality, unity. Civil engineering for the development of national economy and the improvement of people's life provides an important material and technical basis, for many industrial invigoration played a role in promoting, engineering construction is the formation of a fixed asset basic production process, therefore, construction and real estate become in many countries and regions, economic powerhouses.Construction project is housing planning, survey, design, construction of the floorboard. Purpose is for human life and production provide places.Houses will be like a man, it's like a man's life planning environment is responsible by the planners, Its layout and artistic processing, corresponding to the body shape looks and temperament, is responsible by the architect, Its structure is like a person's bones and life expectancy, the structural engineer is responsible, Its water, heating ventilation and electrical facilities such as the human organ and the nerve, is by the equipment engineer is responsible for. Also like nature intact shaped like people, in the city I district planning based on build houses, and is the construction unit, reconnaissance unit, design unit of various design engineers and construction units comprehensive coordination and cooperation process.After all, but ,is structural stress body reaction force and the internal stress and how external force balance. Building to tackle, also must solve the problem is mechanical problems. We have to solve the problem of discipline called architectural mechanics. Architectural mechanics have can be divided into: statics, material mechanics and structural mechanics three mechanical system. Architectural mechanics is discussion and research building structure and component in load and other factors affecting the working condition of, also is the building of intensity, stiffness and stability. In load, bear load and load of structure and component can cause the surrounding objects in their function, and the object itself by the load effect and deformation, and there is the possibility of damage, but the structure itself has certain resistance to deformation and destruction of competence, and the bearingcapacity of the structure size is and component of materials, cross section, and the structural properties of geometry size, working conditions and structure circumstance relevant. While these relationships can be improved by mechanics formula solved through calculation.Building materials in building and has a pivotal role. Building material is with human society productivity and science and technology improves gradually developed. In ancient times, the human lives, the line USES is the rocks and trees. The 4th century BC, 12 ~ has created a tile and brick, humans are only useful synthetic materials made of housing. The 17th century had cast iron and Sheltie later, until the eighteenth century had Portland cement, just make later reinforced concrete engineering get vigorous development. Now all sorts of high-strength structural materials, new decoration materials and waterproof material development, criterion and 20th century since mid organic polymer materials in civil engineering are closely related to the widely application. In all materials, the most main and most popular is steel, concrete, lumber, masonry. In recent years, by using two kinds of material advantage, will make them together, the combination of structure was developed. Now, architecture, engineering quality fit and unfit quality usually adopted materials quality, performance and using reasonable or not have direct connection, in meet the same technical indicators and quality requirements, under the precondition of choice of different material is different, use method of engineering cost has direct impact.In construction process, building construction is and architectural mechanics, building materials also important links. Construction is to the mind of the designer, intention and idea into realistic process, from the ancient JuChao place to now skyscrapers, from rural to urban country road elevated road all need through "construction" means. A construction project, including many jobs such as dredging engineering, deep foundation pit bracing engineering, foundation engineering, reinforced concrete structure engineering, structural lifting project, waterproofing, decorate projects, each type of project has its own rules, all need according to different construction object and construction environment conditions using relevant construction technology, in work-site. whenever while, need and the relevant hydropower and other equipment composition of a whole, each project between reasonable organizing and coordination, better play investment benefit. Civil engineering construction in the benefit, while also issued by the state in strict accordance with the relevant construction technology standard, thus further enhanceChina's construction level to ensure construction quality, reduce the cost for the project.Any building built on the surface of the earth all strata, building weight eventually to stratum, have to bear. Formation Support building the rocks were referred to as foundation, and the buildings on the ground and under the upper structure of self-respect and liable to load transfer to the foundation of components or component called foundation. Foundation, and the foundation and the superstructure is a building of three inseparable part. According to the function is different, but in load, under the action of them are related to each other, is the interaction of the whole. Foundation can be divided into natural foundation and artificial foundation, basic according to the buried depth is divided into deep foundation and shallow foundation. , foundation and foundation is the guarantee of the quality of the buildings and normal use close button, where buildings foundation in building under loads of both must maintain overall stability and if the settlement of foundation produce in building scope permitted inside, and foundation itself should have sufficient strength, stiffness and durability, also consider repair methods and the necessary foundation soil retaining water and relevant measures. [3]As people living standard rise ceaselessly, the people to their place of building space has become not only from the number, and put forward higher requirement from quality are put car higher demands that the environment is beautiful, have certain comfort. This needs to decorate a building to be necessary. If architecture major engineering constitutes the skeleton of the building, then after adornment building has become the flesh-and-blood organism, final with rich, perfect appearance in people's in front, the best architecture should fully embody all sorts of adornment material related properties, with existing construction technology, the most effective gimmick, to achieve conception must express effect. Building outfit fix to consider the architectural space use requirement, protect the subject institutions from damage, give a person with beautiful enjoying, satisfy the requirements of fire evacuation, decorative materials and scheme of rationality, construction technology and economic feasibility, etc. Housing construction development and at the same time, like housing construction as affecting people life of roads, Bridges, tunnels has made great progress.In general civil engineering is one of the oldest subjects, it has made great achievements, the future of the civil engineering will occupy in people's life moreimportant position. The environment worsening population increase, people to fight for survival, to strive for a more comfortable living environment, and will pay more attention to civil engineering. In the near future, some major projects estimated to build, insert roller skyscrapers, across the ocean Bridges, more convenient traffic would not dream. The development of science and technology, and the earth is deteriorating environment will be prompted civil engineering to aerospace and Marine development, provide mankind broader space of living. In recent years, engineering materials mainly is reinforced concrete, lumber and brick materials, in the future, the traditional materials will be improved, more suitable for some new building materials market; especially the chemistry materials will promote the construction of towards a higher point. Meanwhile, design method of precision, design work of automation, information and intelligent technology of introducing, will be people have a more comfortable living environment. With the development of the theory and new materials, the emergence of the application of computer, high-tech introduction to wait to will make civil engineering have a new leap.This is a door needs calm and a great deal of patience and attentive professional. Because hundreds of thousands, even thousands of thousands of lines to building each place structure clearly reflected. Without a gentle state of mind, do what thing just floating on the surface, to any a building structure, to be engaged in business and could not have had a clear, accurate and profound understanding of, the nature is no good. In this business, probably not burn the midnight oil of courage, not to reach the goal of spirit not to give up, will only be companies eliminated.This is a responsible and caring industry. Should have a single responsible heart - I one's life in my hand, thousands of life in my hand. Since the civil, should choose dependably shoulder the responsibility.Finally, this is a constant pursuit of perfect industry. Pyramid, spectacular now: The Great Wall, the majestic... But if no generations of the pursuit of today, we may also use the sort of the oldest way to build this same architecture. Design a building structure is numerous, but this is all experienced centuries of clarification, through continuous accumulation, keep improving, innovation obtained. And such pursuit, not confined in the past. Just think, if the design of a building can be like calculation one plus one equals two as simple and easy to grasp, that was not for what? Therefore, a civil engineer is in constant of in formation. One of the most simple structure, theleast cost, the biggest function. Choose civil, choosing a steadfast diligence, innovation, pursuit of perfect path.Reference:[1] LuoFuWu editor. Civil engineering (professional). Introduction to wuhan. Wuhan university of technology press. 2007[2] WangFuChuan, palace rice expensive editor. Construction engineering materials. Beijing. Science and technology literature press. 2002.[3] jiang see whales, zhiming editor. Civil engineering introduction of higher education press. Beijing, 1992.中文翻译2土木工程概论摘要:土木工程是一门庞大大学科,主要的部分是建筑,无论在国内还是在国外,都有着悠久的历史发展过程。
外文翻译 土木工程 英文文献 文献翻译

外文翻译土木工程英文文献文献翻译外文原文Stage of construction cost controlConstruction enterprises in engineering construction of a construction project cost management is the foundation of the enterprise survival and the development and the core of the construction stage does well the cost control to achieve the purpose of increasing earnings is the project activities more important link, this paper will carry on the elaboration to this question, so that in enterprise production and management play a directive role.So in the project construction cost control what are the content? The author through 10 years of work experience, and analysis has the following aspects:contract aspects: according to construction drawing, contracting contract as the basis, according to the requirements of the contract project, quality, progress index, compiled in detail the construction organization design, this as the basis of cost plan. The project is in the contract and the existence of the change of component project,report to. As far as possible increases the project income. Use contract rights granted reasonable increase income and reduce expenditure.technical aspects: first of all, according to the actual situationof construction site, scientific planning of the construction site layout, to reduce the waste and save money to create conditions; Basedon its technical superiority, fully mobilize the enthusiasm of management personnel, and carry out the mention reasonable suggestion activities, the expansion of nearly may cost control of scope and depth.quality and security; In strict accordance with the engineering technical specifications and rules of safe operation management, reduce and eliminate quality and safety accidents, make all sorts of loss is reduced to the minimum.machinery management: according to the requirements of project scientific, reasonable selection of machinery, give full play to the mechanical performance; Be reasonable arrangement construction in order to improve the utilization rate of the machinery, reduce machine fee cost; Regular maintenance machinery, improve the integrity rate of the machinery, provide guarantee for the whole progress. For the rent mustbe the mechanical equipment, to improve market research touch bottom the material aspects: material purchasing should be abided by "quality, low price and short distance of the principle of" approach to correct materials measurement, serious acceptance, the maximum limit reduced purchasingmanagement in the process of consumption. According to the construction schedule science organization the use of material plan, avoid downtime should phenomenon; Material drawing shall be strictly controlled, regular inventory, grasps the actual1consumption and the progress of the projects contrast data; For inthe recovery turnover materials, sorting, completed with timely and exits, like this is advantageous to the turnover use and reduce thelease fees, and reduce the cost.and administrative management: first to streamline management institutions, avoid overstaffing, reduce unnecessary salary expenses; Control business expenses and so on each unproductive spending Numbers. The administrative office of the materials with property, all on thecard USES, prevent damage and loss,and financial aspects: the financial department is an important part of the cost control, mainly through the spending review all the expenses, balance scheduling funds and establishing various auxiliary records and hard working with all department cost implementation method such as the inspection and supervision, and the engineering cost analysis of all-round and provide feedback to decision-making departments, in order to take effective measures to correct the deviation of the project cost.More from seven aspects of simple described the content of the responsibility cost management, so in the construction of how tospecific implementation, which we need to master the dynamic control of the construction project cost.In short, the construction project cost control is a complicated system engineering. Construction project cost control, the need for flexible use of, the actual operation should adjust measures to local conditions, different project size, different construction enterprise,different management system have differences, but no matter how construction enterprise to manage production is the consumption of human resources, material resources and cost, guidance, supervision and regulation and restrictions2译文施工阶段成本控制建筑施工企业在工程建设中实行施工项目成本管理是企业生存和发展的基础和核心,在施工阶段搞好成本控制,达到增收节支的目的是项目经营活动中更为重要的环节,本文将对这一问题进行论述,以便在企业的生产经营中起指导作用。
土木工程外文翻译(外文)框架

4.1 INVESTIGATION OF STRUCTURAL BEHA VIORInvestigating how structures behave is an important part of structural design: it provides a basis for ensuring the adequacy and safety of a design, In this section I discuss structural investigation in general. As I do throughout this book. I focus on material relevant to structural design tasks.Purpose of InvestigationMost structures exist because they are needed. Any evaluation of a structure thus must begin with an analysis of how effectively the structure meets the usage requirements.Designers must consider the following three factors:●Functionality. or the general physical relationships of the structure'sform. detail. durability. fire resistance. deformation resistance. and so on.●Feasibility. including cost. availability of materials and products. andpracticality of construction.●Safety. or capacity 10 resist anticipated loads.MeansAn investigation of a fully defined structure involves the following:1. Determine the structure's physical being-materials, form, scale.orientation. location. support conditions, and internal character and detail.2. Determine the demands placed on the structure-that is. loads.3. Determine the structure's deformation limits.4. Determine the structure's load response-how it handles internal forcesand stresses and significant deformations.5. Evaluate whether the structure can safely handle the requiredstructural tasks.Investigation may take several forms. You can●Visualize graphically the structure's deformation under load.●Manipulate mathematical models.●Test the structure or a scaled model, measuring its responses to loads. When precise quantitative evaluations are required. use mathematical models based on reliable theories or directly measure physical responses. Ordinarily. mathematical modeling precedes any actual construction-even of a test model. Limit direct measurementto experimental studies or to verifying untestedtheories or design methods.Visual AidsIn this book, I emphasize graphical visualization; sketches arc invaluable learning and problem-solving aids. Three types of graphics are most useful: the free-body diagram. the exaggerated profile of a load-deformed structure. and the scaled pial.A free-body diagram combines a picture of an isolated physical clemen I with representations of all external forces. The isolated clement may be a whole structure or some part of it.For example. Figure 4.1a shows an entire structure-a beamand-eolumn rigid bent-and the external forces (represented by arrows). which include gravity. wind. and the reactive resistance of the supports (called the reactions). Note: Such a force system holds the structure in static equilibrium.Figure 4.lb is a free-body diagram of a single beam from the bent. Operating on the beam are two forces: its own weight and the interaction between the beam ends and the columns 10 which the beam is all ached. These interactions are not visible in the Ireebody diagram of the whole bent. so one purpose of the diagram for the beam is to illustrate these interactions. For example. note that the columns transmit to theendsofthe beams horizontal and vertical forces as well as rotational bending actions.Figure 4.lc shows an isolated portion ofthe beam length. illustrating the beam's internal force actions. Operating on this free body arc its own weight and the actions of the beam segments on the opposite sides of the slicing planes. since it is these actions that hold the removed portion in place in the whole beam.Figure 4.ld. a tiny segment. or particle. of the beam material is isolated, illustrating the interactions between this particle and those adjacent to it. This device helps designers visualize stress: in this case. due to its location in the beam. the particle is subjected to a combination of shear and linear compression stresses.An exaggerated profile of a load-deformed structure helps establish the qualitative nature of the relationships between force actions and shape changes. Indeed. you can infer the form deformation from the type of force or stress. and vice versa.FIGURE 4.1Free-body diagrams.For example. Figure shows {he exaggerated deformation of the bent in Figure 4.1 under wind loading. Note how you can determine the nature of bending action in each member of the frame from this figure. Figure 4.2b shows the nature of deformation of individual particles under various types of stress.FIGURE 4.2 Structural deformationThe scaled plot is a graph of some mathematical relationship or real data. For example, the graph in Figure 4.3 represents the form of a damped ibration of an elastic spring. It consists of the plot of the displacements against elapsed time t. and represents the graph of the expression.FIGURE 4.3 Graphical plot of a damped cyclic motion.Although the equation is technically sufficient to describe the phenomenon, the graph illustrates many aspects of the relationship. such as the rate of decay of the displacement. the interval of the vibration. the specific position at some specific elapsed time. and so on..4.2 METHODS OF INVESTIGATION AND DESIGNTraditional structural design centered on the working stress method. a method now referred to as stress design or allowable stress design (ASD). This method. which relies on the classic theories of elastic behavior, measures a design's safety against two limits: an acceptable maximum stress (called allowable working stress) and a tolerable extent of deformation (deflection. stretch. erc.). These limits refer to a structure's response to service loads-that is. the loads caused by normal usage conditions. The strength me/hod, mean-while, measures a design's adequacy against its absolute load limit-that is. when the structure must fail.To convincingly establish stress. strain. and failure limits, tests were performed extensively in the field (on real structures) and laboratories (on specimen prototypes. or models). Note: Real-world structural failures are studied both for research sake and to establish liability.In essence. the working stress method consists of designing a structure to work at some established percentage of its total capacity. The strength methodconsists of designing a structure tofail. but at a load condition well beyond what it should experience. Clearly the stress and strength methods arc different. but the difference is mostly procedural.The Stress Method (ASD)The stress method is as follows:1. Visualize and quantify the service (working) load conditions asintelligently as possible. You can make adjustments by determiningstatistically likely load combinations (i.e , dead load plus live load pluswind load). considering load duration. and so on.2. Establish standard stress. stability, and deformation limits for thevarious structural responses-in tension. bending, shear, buckling.deflection, and so on.3. Evaluate the structure's response.An advantage of working with the stress method is that you focus on the usage condition (real or anticipated). The principal disadvantage comes from your forced detachment from real failure conditions-most structures develop much different forms of stress and strain as they approach their failure limits.The Strength Method (LRFD)The strength method is as follows:1. Quantify the service loads. Then multiply them by an adjustmentfactor'( essentially a safety factor) to produce thejaclOred load.2. Visualize the various structural responses and quantify the structure'sultimate (maximum, failure) resistance in appropriate terms(resistance to compression, buckling. bending. etc.). Sometimes thisresistance is subject to an adjustment factor, calledtheresistancefacror. When you employ load and resistance factors.the strength method is now sometimes called foad andresistancefaaor design (LRFD) (see Section 5.9).3. Compare the usable resistance ofthe structu re to the u ltirnatcresistance required (an investigation procedure), or a structure with anappropriate resistance is proposed (a design procedure).A major reason designers favor the strength method is that structural failure is relatively easy to test. What is an appropriate working condition is speculation. In any event, the strength method which was first developed for the design of reinforced concrete structures, is now largely preferred in all professional design work.Nevertheless, the classic theories of clastic behavior still serve as a basisfor visualizing how structures work. But ultimate responses usually vary from the classic responses, because of inelastic materials, secondary effects, multi mode responses, and so on. In other words, the usual procedure is to first consider a classic, elastic response, and then to observe (or speculate about) what happens as failure limits are approached.。
土木工程专业外文翻译--土木工程

外文原文:Civil EngineeringCivil engineering is the planning, design, construction, and management of the built environment. This environment includes all structures built according to scientific principles, from irrigation and drainage systems to rocket launching facilities.Civil engineers build roads, bridges, tunnels, dams, harbors, power plants, water and sewage systems, hospitals, schools, mass transit, and other public facilities essential to modern society and large population concentrations. They also build privately owned facilities such as airport, railroads, pipelines, skyscrapers, and other large structures designed for industrial, commercial, or residential use. In addition, civil engineers plan, design, and build complete cities and towns, and more recently have been planning and designing space platforms to self-contained communities.The word civil derives from the Latin for citizen. In 1782, Englishman John Seaton used the term to differentiate his nonmilitary engineering work from that of the military engineers who predominated at the time. Since then, the term civil engineer has often been used to refer to engineers who build public facilities, although the field is much broader.Scope Because it is so broad, civil engineering is subdivided into a number of technical specialties. Depending on the type of project, the skills pf many kinds of civil engineer specialties may be needed. When a project begins, the site is surveyed and mapped by civil engineers who experiment to determine if the earth can bear the weight of project. Environmental specialists study the project’s impact on the local area, the potential for air and groundwater pollution, the project’s impact on local animal and plant life, and how the project can be designed to meet government requirements aimed at protecting the environment. Transportation specialists determine what kind of facilities are needed to ease the burden on local roads and other transportation networks that will result from the completed project. Meanwhile, structural specialists raise preliminary data to make detailed designs, plans, and specifications for the project. Supervising and coordinating the work of these civil engineer specialists, from beginning to end of the project, are the construction management specialists. Based on information supplied by the other specialists, construction management civil engineers estimate quantitiesand costs of materials and subcontractors, and perform other supervisory work to ensure the project is completed on time and as specified.Many civil engineers, among them the top people in the field, work in design. As we have seen, civil engineers work on many different kinds of structures, so it is normal practice for an engineer to specialize in just one kind. In designing buildings, engineers often work as consultants to architectural or construction firms. Dams, bridges, water supply systems, and other large projects ordinarily employ several engineers whose work is coordinated by a system engineer who is in charge of the entire project. In many cases, engineers from other disciplines are involved. In a dam project, for example, electrical and mechanical engineers work on the design of the powerhouse and its equipment. In other cases, civil engineers are assigned to work on a project in another field; in the space program, for instance, civil engineers were necessary in the design and construction of such structures as launching pads and rocket storage facilities.Throughout any given project, civil engineers make extensive use of computers. Computes are used to design the project’s various elements (computer-aided design, or CAD) and to manger it. Computers are a necessity for the modern civil engineer because they permit the engineer to efficiently handle the large quantities of data needed in determining the best way to construct a project.Structural engineering In this specialty, civil engineers plan and design structures of all types, including bridges dams, power plants, supports for equipment, special structures for offshore projects, the United States space program, transmission towers, giant astronomical and radio telescopes, and many other kinds of projects.Using computers, structural engineers determine the forces a structure must resist, its own weight, wind and hurricane forces temperature changes that expand or contract construction materials, and earthquakes. They also determine the combination of appropriate materials: steel, concrete, plastic, stone, asphalt, brick, aluminum, or other construction materials.Water resources engineering Civil engineers in this specialty deal with all aspects of the physical control of water. Their projects help prevent floods, supply water for cities and for irrigation, manage and control rivers and water runoff, and maintain beaches and other waterfront facilities. In addition, they design and maintain harbors, canals, and locks, build huge hydroelectric dams and smaller dams and water impoundments of all kinds, help design offshorestructures, and determine the location of structures affecting navigation.Geotechnical engineering Civil engineers who specialize in this filed analyze the properties of soils and rocks that support structures and affect structural behavior. They evaluate and work to minimize the potential settlement of buildings and other structures that stems from the pressure of their weight on the earth. These engineers also evaluate and determine how to strengthen the stability of slopes and how to protect structures against earthquakes and the effects of groundwater.Environmental engineering In this branch of engineering, civil engineers design, build, and supervise systems to provide safe drinking water and to prevent and control pollution of water supplies, both on the surface and underground. They also design, build, and supervise projects to control or eliminate pollution of the land and air. These engineers build water and wastewaters treatment plants, and design air scrubbers and other devices to minimize or eliminate air pollution caused by industrial processes, incineration, or other smoke-producing activities. They also work to control toxic and hazardous wastes through the construction of special dump sites or the neutralizing of toxic and hazardous substances. In addition the engineers design and manage sanitary landfills to prevent pollution of surrounding land.Transportation engineering Civil engineers working in this specialty build facilities to ensure safe and efficient movement of both people and goods. They specialize in designing and maintaining all types of transportation facilities, highways and streets, mass transit systems, railroads and airfields ports and harbors. Transportation engineers apply technological knowledge as well as consideration of the economic, political, and social factors in designing each project. They work closely with urban planners since the quality of the community is directly related to the quality of the transportation system.Pipeline engineering In this branch of civil engineering, engineers build pipelines and related facilities, which transport liquids, gases, or solids ranging from coal slurries (mixed coal and water) and semi liquids wastes, to water, oil and various types pf highly combustible and noncombustible gases. The engineers determine pipeline design, the economic and environmental impact of a project on regions it must traverse, the type pf materials to be used-steel, concrete, plastic, or combinations of various materials, installation techniques, methods for testing pipeline strength, and controls for maintaining proper pressure and rate of flow of materials being transported. When hazardous materials are being carried, safety is a major consideration as well.Construction engineering Civil engineers in this field oversee the construction of a project from beginning to end. Sometimes called project engineers, they apply both technical and managerial skills, including knowledge of construction methods, planning, organizing, financing, and operating construction projects. They coordinate the activities of virtually everyone engaged in the work: the surveyors, workers who lay out and construct the temporary roads and ramps, excavate for the foundation, build the forms and pour the concrete; and workers who build the steel frame-work. These engineers also make regular progress reports to the owners of the structure.Construction is a complicated process on almost all engineering projects. It involves scheduling the work and utilizing the equipment and the materials so that coats are kept as low as possible. Safety factor must also be taken into account, since construction can be very dangerous. Many civil engineers therefore specialize in the construction phase.Community and urban planning Those engaged in this area of civil engineering may plan and develop communities within a city, or entire cities. Such planning involves far more than engineering considerations; environmental, social, and economic factors in the use and development of land and natural resources are also key elements. They evaluate the kinds of facilities needed, including streets and highways, public transportation systems, airports, and recreational and other facilities to ensure social and economic as well as environmental well-being.Photogrammetry, surveying, and mapping The civil engineers in this specialty precisely measure the Earth’s surface to obtain reliable information for locating and designing engineering projects. This practice often involves high-technology methods such as satellite and aerial surveying, and computer processing of photographic imagery. Radio signals from satellites, scanned by laser and sonic beams, are converted to maps to provide very accurate measurements for boring tunnels, building highways and dams, plotting flood control and irrigation projects, locating subsurface geologic formations that may affect a construction project and a host of other building uses.Other specialties Three additional civil engineering specialties that are not entirely within the scope of civil engineering teaching.Engineering research Research is one of the most important aspects of scientific and engineering practice. A researcher usually works as a member of a team with other scientists and engineers. He or she is often employed in alaboratory that financed by government or industry. Areas of research connected with civil engineering include soil mechanics and soil stabilization techniques, and also the development and testing of new structural materials.Engineering management Many civil engineers choose careers that eventually lead to management. Others are also to start their careers in management positions. The civil engineer manager combines technical knowledge with an ability to organize and coordinate worker power, materials, machinery, and money. These engineers may work in government municipal, county, state, or federal; in the U.S.Army Corps of Engineers as military or civilian management engineers; or in semiautonomous regional or city authorities or similar organization. They may also manage private engineering firms ranging in size from a few employees to hundreds.Engineering teaching The civil engineer who chooses a teaching career usually teaches both graduate and undergraduate students in technical specialties. Many teaching civil engineers engage in basic research that eventually leads to technical innovations in construction materials and methods. Many also serve as consultants on engineering projects, or on technical boards and commissions associated with major projects.中文译文:土木工程土木工程是指对建成环境的规划、设计、建造、管理等一系列活动。
土木工程外文翻译-原文
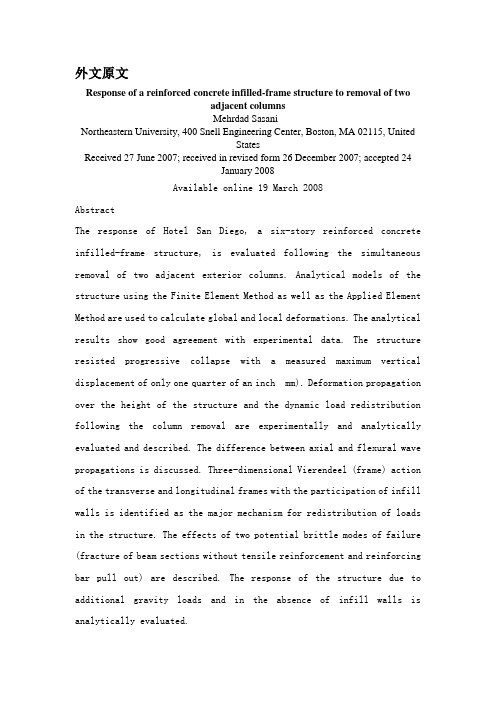
外文原文Response of a reinforced concrete infilled-frame structure to removal of twoadjacent columnsMehrdad Sasani_Northeastern University, 400 Snell Engineering Center, Boston, MA 02115, UnitedStatesReceived 27 June 2007; received in revised form 26 December 2007; accepted 24January 2008Available online 19 March 2008AbstractThe response of Hotel San Diego, a six-story reinforced concrete infilled-frame structure, is evaluated following the simultaneous removal of two adjacent exterior columns. Analytical models of the structure using the Finite Element Method as well as the Applied Element Method are used to calculate global and local deformations. The analytical results show good agreement with experimental data. The structure resisted progressive collapse with a measured maximum vertical displacement of only one quarter of an inch mm). Deformation propagation over the height of the structure and the dynamic load redistribution following the column removal are experimentally and analytically evaluated and described. The difference between axial and flexural wave propagations is discussed. Three-dimensional Vierendeel (frame) action of the transverse and longitudinal frames with the participation of infill walls is identified as the major mechanism for redistribution of loads in the structure. The effects of two potential brittle modes of failure (fracture of beam sections without tensile reinforcement and reinforcing bar pull out) are described. The response of the structure due to additional gravity loads and in the absence of infill walls is analytically evaluated.c 2008 Elsevier Ltd. All rights reserved.Keywords: Progressive collapse; Load redistribution; Load resistance; Dynamic response; Nonlinear analysis; Brittle failure1.IntroductionThe principal scope of specifications is to provide general principles and computational methods in order to verify safet y of structures. The “safety factor ”, which according t o modern trends is independent of the nature and combination of the materials used, can usually be defined as the rati o between the conditions. This ratio is also proportional to the inverse of the probability ( risk ) of failure of th e structure.Failure has to be considered not only as overall collapse o f the structure but also as unserviceability or, according t o a more precise. Common definition. As the reaching of a “limit state ”which causes the construction not to acco mplish the task it was designed for. There are two categori es of limit state :(1)Ultimate limit sate, which corresponds to the highest value of the load-bearing capacity. Examples include local buckli ng or global instability of the structure; failure of some sections and subsequent transformation of the structure intoa mechanism; failure by fatigue; elastic or plastic deformati on or creep that cause a substantial change of the geometry of the structure; and sensitivity of the structure to alte rnating loads, to fire and to explosions.(2)Service limit states, which are functions of the use and durability of the structure. Examples include excessive defo rmations and displacements without instability; early or exces sive cracks; large vibrations; and corrosion.Computational methods used to verify structures with respect to the different safety conditions can be separated into: (1)Deterministic methods, in which the main parameters are co nsidered as nonrandom parameters.(2)Probabilistic methods, in which the main parameters are co nsidered as random parameters.Alternatively, with respect to the different use of factors of safety, computational methods can be separated into:(1)Allowable stress method, in which the stresses computed un der maximum loads are compared with the strength of the mat erial reduced by given safety factors.(2)Limit states method, in which the structure may be propor tioned on the basis of its maximum strength. This strength, as determined by rational analysis, shall not be less than that required to support a factored load equal to the sum of the factored live load and dead load ( ultimate state ).The stresses corresponding to working ( service ) conditions with unfactored live and dead loads are compared with pres cribed values ( service limit state ) . From the four poss ible combinations of the first two and second two methods, we can obtain some useful computational methods. Generally, t wo combinations prevail:(1)deterministic methods, which make use of allowable stresses . (2)Probabilistic methods, which make use of limit states. The main advantage of probabilistic approaches is that, at l east in theory, it is possible to scientifically take into account all random factors of safety, which are then combine d to define the safety factor. probabilistic approaches depend upon :(1) Random distribution of strength of materials with respect to the conditions of fabrication and erection ( scatter of the values of mechanical properties through out the structu re ); (2) Uncertainty of the geometry of the cross-section sand of the structure ( faults and imperfections due to fab rication and erection of the structure );(3) Uncertainty of the predicted live loads and dead loads acting on the structure; (4)Uncertainty related to the approx imation of the computational method used ( deviation of the actual stresses from computed stresses ). Furthermore, proba bilistic theories mean that the allowable risk can be based on several factors, such as :(1) Importance of the construction and gravity of the damage by its failure; (2)Number of human lives which can be thr eatened by this failure; (3)Possibility and/or likelihood of repairing the structure; (4) Predicted life of the structure. All these factors are related to economic and social consi derations such as:(1) Initial cost of the construction;(2) Amortization funds for the duration of the construction;(3) Cost of physical and material damage due to the failure of the construction;(4) Adverse impact on society;(5) Moral and psychological views.The definition of all these parameters, for a given saf ety factor, allows construction at the optimum cost. However, the difficulty of carrying out a complete probabilistic ana lysis has to be taken into account. For such an analysis t he laws of the distribution of the live load and its induc ed stresses, of the scatter of mechanical properties of mate rials, and of the geometry of the cross-sections and the st ructure have to be known. Furthermore, it is difficult to i nterpret the interaction between the law of distribution of strength and that of stresses because both depend upon the nature of the material, on the cross-sections and upon the load acting on the structure. These practical difficulties ca n be overcome in two ways. The first is to apply different safety factors to the material and to the loads, without necessarily adopting the probabilistic criterion. The second i s an approximate probabilistic method which introduces some s implifying assumptions ( semi-probabilistic methods ) . Aspart of mitigation programs to reduce the likelihood of mass casualties following local damage in structures, the General Services Administration [1] and the Department of Defense [2] developed regulations to evaluate progressive collapse resistance of structures. ASCE/SEI 7 [3] defines progressive collapse as the spread of an initial local failure fromelement to element eventually resulting in collapse of an entire structure or a disproportionately large part of it. Following the approaches proposed by Ellinwood and Leyendecker [4], ASCE/SEI 7 [3] defines two general methods for structural design of buildings to mitigate damage due to progressive collapse: indirect and direct design methods. General building codes and standards [3,5] use indirect design by increasing overall integrity of structures. Indirect design is also used in DOD [2]. Although the indirect design method can reduce the risk of progressive collapse [6,7] estimation of post-failure performance of structures designed based on such a method is not readily possible. One approach based on direct design methods to evaluate progressive collapse of structures is to study the effects of instantaneous removal of load-bearing elements, such as columns. GSA [1] and DOD [2] regulations require removal of one load bearing element. These regulations are meant to evaluate general integrity of structures and their capacity of redistributing the loads following severe damage to only one element. While such an approach provides insight as to the extent to which the structures are susceptible to progressive collapse, in reality, the initial damage can affect more than just one column. In this study, using analytical results that are verified against experimental data, the progressive collapse resistance of the Hotel San Diego is evaluated, following the simultaneous explosion (sudden removal) of two adjacent columns, one of which was a corner column. In order to explode the columns, explosives were inserted into predrilled holes in the columns. The columns were then well wrapped with a few layers of protective materials. Therefore, neither air blast nor flying fragments affected the structure.2. Building characteristicsHotel San Diego was constructed in 1914 with a south annex added in 1924. The annex included two separate buildings. Fig. 1 shows a south view of the hotel. Note that in the picture, the first and third stories of the hotel are covered with black fabric. The six story hotel had a non-ductile reinforced concrete (RC) frame structure with hollow clay tile exterior infill walls. The infills in the annex consisted of two withes (layers) of clay tiles with a total thickness of about 8 in (203 mm). The height of the first floor was about 190–800 m). The height of other floors and that of the top floor were 100–600 m) and 160–1000 m), respectively. Fig. 2 shows the second floor of one of the annex buildings. Fig. 3 shows a typical plan of this building, whose responsefollowing the simultaneous removal (explosion) of columns A2 and A3 in the first (ground) floor is evaluated in this paper. The floor system consisted of one-way joists running in the longitudinal direction (North–South), as shown in Fig. 3. Based on compression tests of two concrete samples, the average concrete compressive strength was estimated at about 4500 psi (31 MPa) for a standard concrete cylinder. The modulus of elasticity of concrete was estimated at 3820 ksi (26 300 MPa) [5]. Also, based on tension tests of two steel samples having 1/2 in mm) square sections, the yield and ultimate tensile strengths were found to be 62 ksi (427 MPa) and 87 ksi (600 MPa), respectively. The steel ultimate tensile strain was measured at . The modulus of elasticity of steel was set equal to 29 000 ksi (200 000 MPa). The building was scheduled to be demolished by implosion. As part of the demolition process, the infill walls were removed from the first and third floors. There was no live load in the building. All nonstructural elements including partitions, plumbing, and furniture were removed prior to implosion. Only beams, columns, joist floor and infill walls on the peripheral beams were present.3. SensorsConcrete and steel strain gages were used to measure changes in strains of beams and columns. Linear potentiometers were used to measure global and local deformations. The concrete strain gages were in (90 mm) long having a maximum strain limit of ±. The steel strain gages could measure up to a strain of ±. The strain gages could operate up to a several hundred kHz sampling rate. The sampling rate used in the experiment was 1000 Hz. Potentiometers were used to capture rotation (integral of curvature over a length) of the beam end regions and global displacementin the building, as described later. The potentiometers had a resolution of about in mm) and a maximum operational speed of about 40 in/s m/s), while the maximum recorded speed in the experiment was about 14 in/sm/s).4. Finite element modelUsing the finite element method (FEM), a model of the building was developed in the SAP2000 [8] computer program. The beams and columns are modeled with Bernoulli beam elements. Beams have T or L sections with effective flange width on each side of the web equal to four times the slab thickness [5]. Plastic hinges are assigned to all possible locations where steel bar yielding can occur, including the ends of elements as well as the reinforcing bar cut-off and bend locations. The characteristics of the plastic hinges are obtained using section analysesof the beams and columns and assuming a plastic hinge length equal to half of the section depth. The current version of SAP2000 [8] is not able to track formation of cracks in the elements. In order to find the proper flexural stiffness of sections, an iterative procedure is used as follows. First, the building is analyzed assuming all elements are uncracked. Then, moment demands in the elements are compared with their cracking bending moments, Mcr . The moment of inertia of beam and slab segments are reduced by a coefficient of [5], where the demand exceeds the Mcr. The exterior beam cracking bending moments under negative and positive moments, are 516 k in kN m) and 336 k in kN m), respectively. Note that no cracks were formed in the columns. Then the building is reanalyzed and moment diagrams are re-evaluated. This procedure is repeated until all of the cracked regions are properly identified and modeled.The beams in the building did not have top reinforcing bars except at the end regions (see Fig. 4). For instance, no top reinforcement was provided beyond the bend in beam A1–A2, 12 inches away from the face of column A1 (see Figs. 4 and 5). To model the potential loss of flexural strength in those sections, localized crack hinges were assigned at the critical locations where no top rebar was present. Flexural strengths of the hinges were set equal to Mcr. Such sections were assumed to lose their flexural strength when the imposed bending moments reached Mcr.The floor system consisted of joists in the longitudinal direction (North–South). Fig. 6 shows the cross section of a typical floor. In order to account for potential nonlinear response of slabs and joists, floors are molded by beam elements. Joists are modeled with T-sections, having effective flange width on each side of the web equal to four times the slab thickness [5]. Given the large joist spacing between axes 2 and 3, two rectangular beam elements with 20-inch wide sections are used between the joist and the longitudinal beams of axes 2 and 3 to model the slab in the longitudinal direction. To model the behavior of the slab in the transverse direction, equally spaced parallel beams with 20-inch wide rectangular sections are used. There is a difference between the shear flow in the slab and that in the beam elements with rectangular sections modeling the slab. Because of this, the torsional stiffness is setequal to one-half of that of the gross sections [9].The building had infill walls on 2nd, 4th, 5th and 6th floors on the spandrel beams with some openings . windows and doors). As mentioned before and as part of the demolition procedure, the infill walls in the 1st and 3rd floors were removed before the test. The infill walls were made of hollow clay tiles, which were in good condition. The net area of the clay tiles was about 1/2 of the gross area. The in-plane action of the infill walls contributes to the building stiffness and strength and affects the building response. Ignoring the effects of the infill walls and excluding them in the model would result in underestimating the building stiffness and strength.Using the SAP2000 computer program [8], two types of modeling for the infills are considered in this study: one uses two dimensional shell elements (Model A) and the other uses compressive struts (Model B) as suggested in FEMA356 [10] guidelines.. Model A (infills modeled by shell elements)Infill walls are modeled with shell elements. However, the current version of the SAP2000 computer program includes only linear shell elements and cannot account for cracking. The tensile strength of the infill walls is set equal to 26 psi, with a modulus of elasticity of 644 ksi [10]. Because the formation ofcracks has a significant effect on the stiffness of the infill walls, the following iterative procedure is used to account for crack formation:(1) Assuming the infill walls are linear and uncracked, a nonlinear time history analysis is run. Note that plastic hinges exist in the beam elements and the segments of the beam elements where moment demand exceeds the cracking moment have a reduced moment of inertia.(2) The cracking pattern in the infill wall is determined by comparingstresses in the shells developed during the analysis with the tensile strength of infills.(3) Nodes are separated at the locations where tensile stress exceeds tensile strength. These steps are continued until the crack regions are properly modeled.. Model B (infills modeled by struts)Infill walls are replaced with compressive struts as described in FEMA 356 [10] guidelines. Orientations of the struts are determined from the deformed shape of the structure after column removal and the location of openings.. Column removalRemoval of the columns is simulated with the following procedure. (1) The structure is analyzed under the permanent loads and the internal forces are determined at the ends of the columns, which will be removed.(2) The model is modified by removing columns A2 and A3 on the first floor. Again the structure is statically analyzed under permanent loads. In this case, the internal forces at the ends of removed columns found in the first step are applied externally to the structure along with permanent loads. Note that the results of this analysis are identical to those of step 1.(3) The equal and opposite column end forces that were applied in the second step are dynamically imposed on the ends of the removed column within one millisecond [11] to simulate the removal of the columns, and dynamic analysis is conducted.. Comparison of analytical and experimental resultsThe maximum calculated vertical displacement of the building occurs at joint A3 in the second floor. Fig. 7 shows the experimental andanalytical (Model A) vertical displacements of this joint (the AEM results will be discussed in the next section). Experimental data is obtained using the recordings of three potentiometers attached to joint A3 on one of their ends, and to the ground on the other ends. The peak displacements obtained experimentally and analytically (Model A) are in mm) and in mm), respectively, which differ only by about 4%. The experimental and analytical times corresponding to peak displacement are s and s, respectively. The analytical results show a permanent displacement of about in mm), which is about 14% smaller than the corresponding experimental value of in mm).Fig. 8 compares vertical displacement histories of joint A3 in the second floor estimated analytically based on Models A and B. As can be seen, modeling infills with struts (Model B) results in a maximum vertical displacement of joint A3 equal to about in mm), which is approximately 80% larger than the value obtained from Model A. Note that the results obtained from Model A are in close agreement with experimental results (see Fig. 7), while Model B significantly overestimates the deformation of the structure. If the maximum vertical displacement were larger, the infill walls were more severely cracked and the struts were more completely formed, the difference between the results of the two models (Models A and B) would be smaller.Fig. 9 compares the experimental and analytical (Model A) displacement of joint A2 in the second floor. Again, while the first peak vertical displacement obtained experimentally and analytically are in good agreement, the analytical permanent displacement under estimates the experimental value.Analytically estimated deformed shapes of the structure at the maximumvertical displacement based on Model A are shown in Fig. 10 with a magnification factor of 200. The experimentally measured deformed shape over the end regions of beams A1–A2 and A3–B3 in the second floorare represented in the figure by solid lines. A total of 14 potentiometers were located at the top and bottom of the end regions of the second floor beams A1–A2 and A3–B3, which were the most critical elements in load redistribution. The beam top and corresponding bottom potentiometerrecordings were used to calculate rotation between the sections where the potentiometer ends were connected. This was done by first finding the difference between the recorded deformations at the top and bottom of the beam, and then dividing the value by the distance (along the height of the beam section) between the two potentiometers. The expected deformed shapes between the measured end regions of the second floor beams are shown by dashed lines. As can be seen in the figures, analytically estimated deformed shapes of the beams are in good agreement with experimentally obtained deformed shapes.Analytical results of Model A show that only two plastic hinges are formed indicating rebar yielding. Also, four sections that did not have negative (top) reinforcement, reached cracking moment capacities and therefore cracked. Fig. 10 shows the locations of all the formed plastic hinges and cracks.。
- 1、下载文档前请自行甄别文档内容的完整性,平台不提供额外的编辑、内容补充、找答案等附加服务。
- 2、"仅部分预览"的文档,不可在线预览部分如存在完整性等问题,可反馈申请退款(可完整预览的文档不适用该条件!)。
- 3、如文档侵犯您的权益,请联系客服反馈,我们会尽快为您处理(人工客服工作时间:9:00-18:30)。
本科毕业设计(论文)外文翻译译文学生姓名:院(系):专业班级:指导教师:完成日期:钢筋混凝土填充框架结构对拆除两个相邻的柱的响应作者:Mehrdad Sasani 美国波士顿东北大学,斯奈尔400设计中心MA02115收稿日期:2007年7月27日,修整后收稿日期2007年12月26日,录用日期2008年1月24日,网上上传日期2008年3月19日。
摘要:本文是评价圣地亚哥旅馆对同时拆除两根相邻的外柱的响应问题,圣地亚哥旅馆是个6层钢筋混凝土填充框架结构。
结构的分析模型应用了有限元法和以此为基础的分析模型来计算结构的整体和局部变形。
分析结果跟实验结果非常吻合。
当测量的竖向位移增加到为四分之一英寸(即6.4mm)的时候,结构就发生连续倒塌。
通过实验分析方法评价和讨论随着柱的移除而产生的变形沿着结构高度上的发展和荷载动态重分配。
讨论了轴向和弯曲的变形传播的不同。
结构横向和纵向的三维桁架在填充墙的参与下被认为是荷载重分配的主要构件。
讨论了两种潜在的脆性破坏模型(没有拉力加强的梁的脆断和有加筋肋的梁的挤出)。
分析评价了结构对额外的重力和无填充墙时的响应。
Elsevier有限责任公司对此文保留所有权利。
关键词:连续倒塌;荷载重分配;对荷载抵抗能力;动态响应;非线性分析;脆性破坏。
1. 介绍:作为减小由于结构的局部损坏而造成大量伤亡的可能性措施的一部分,美国总务管理局【1】和国防部【2】出台了一系列制度来评价结构对连续倒塌的抵抗力。
【3】定义连续倒塌为,由原始单元的局部破坏在单元间的扩展最终造成结构的整体或不成比例的大部破坏。
通过Ellingwood 和Leyendecker【4】建议的方法,ASCE/SEI 7定义了两种一般模型来减小结构设计时连续倒塌效应产生的损害,它们分为直接和间接的设计方法。
一般建筑规范和标准用增加结构的整体性的间接设计方法。
间接设计法也应用于美国国防部的降低连续倒塌设计和未归档设备标准中。
尽管间接设计法可以降低连续破坏的风险【6,7】,对基于此法设计的结构破坏后的表现的判断是不容易实现的。
有一种基于直接设计的方法通过研究瞬间消除受载构件,比如柱子,对结构的影响来评价结构的连续倒塌。
美国防部和国家事务管理局的规章是要求去除一个受荷构件,考虑其影响。
这样的规范目的是评价结构的整体性和结构的一个单元出现严重的毁坏时的分荷能力。
这种方法是研究结构受连续倒塌的影响的程度,但是事实上初始结构损伤的影响不止局限于某一根柱子。
在本论文中,应用通过实验证实的分析结果,评价圣地亚哥旅馆抵抗连续破坏的能力,实验中瞬间移除两个相邻的柱子,其中一个柱是拐角柱。
为了爆除这两个柱子,将炸药放在预先在柱子上钻的孔里面。
柱子然后再用几层保护材料包裹好,以避免爆炸时的冲击波和碎片影响结构的其他部分。
2. 建筑的特性圣地亚哥旅馆建造于1914年,在1924年又向南扩展了一部分,此部分包括两个分离的结构。
图.1是从南边看旅馆的样子。
注意这张照片,旅馆的第一和第三层被用黑色的布蒙了起来。
这个六层的旅馆是无延性的钢筋混凝土框架结构,其中还有由空心砖构成的填充外墙。
扩展部分的填充墙有两层共203mm厚。
第一层的楼高为6.0m,其他楼盖高为3.2m,顶楼高度为5.13m。
图.2为其中一个扩展部分的第二层。
图.3为对本建筑的实施计划,即瞬间爆除一层相邻的柱A2和A3,以评价其影响。
左图:图.1 圣地亚哥旅馆的南端视角,本论文研究其中心结构右图:图.2 扩展结构的第二层(南端视角)下图:图.3 拟对旅馆南扩展部分实施的柱的移除计划,第一层要被移除的柱用叉号标出如图.3所示楼盖系统纵向(南北向)有一个托梁。
根据两个混凝土构件受压的实验结果,对一个标准的混凝土柱,受压承载力为31MPa。
混凝土的弹性模量大概为26300MPa左右。
同样,通过横截面12.7mm的钢筋受拉实验,其屈服和极限抗拉强度分别为427和600MPa。
钢筋的极限变形为0.17。
钢筋的弹性模量近似为200000MPa。
这个建筑按计划将被爆破摧毁。
作为摧毁的一个步骤,第一层和第三层的填充墙被移除。
移除时上面没有活荷载。
所有的非结构部件包括隔墙、管道设备、家具都被事先搬走了,只有梁、柱、楼板梁和在边梁上的填充墙被留下。
3. 传感器布置混凝土和钢筋的应变传感器是用来测量梁和柱的应变变化的。
线性电位计用来测量整体和局部变形。
混凝土应变测量仪常900mm,最大应变为±0.02.钢筋应变测量仪应变极限为±0.2。
应变测量仪可以带到几百千赫兹。
电位计用来测量建筑中梁沿一端的转动和整体位移,这些以后将讲到。
电位计的分辨率为0.01mm,最大速度为1.0m/s,实验中最大记录速度为0.35m/s。
4. 有限元模型通过有限单元法,在软件SAP2000【8】中生成一个建筑模型。
梁和柱都被抽象成Bernoulli单元。
T和L型梁的翼缘计算宽度为四倍的较厚板的厚度【5】。
塑性铰可以发生在任何钢筋可能发生屈服的地方,包括单元的端点、加筋肋分离点和弯矩的屈服点。
在分析中,塑性铰的范围是构件高度的一半。
现行版本的SAP2000不能计算出单元斜裂缝的构成。
为了得出正确的构件挠曲刚度,反复做以下步骤:首先假设建筑的所有单元都是没有裂缝的;然后,需要弯矩同构件的出现裂缝的弯矩相比较。
分别降低板厚和梁的惯性矩35%,使需求弯矩大于裂缝出现弯矩。
梁外部出现裂缝的正负弯矩分别为58.2knM和37.9knM。
需要注意的是柱子没有裂缝出现。
再后,再按以上方法重新分析建筑和弯矩简图。
重复这些步骤直到所有的裂缝区域被鉴定和用模型表示出来。
除了两端区域建筑结构里的梁上部不配筋(图.4)。
例如,梁A1-A2在距A1点305mm以后,其上部不配筋(如图.4和5)。
为了确定出可能丧失挠曲强度的截面位置,将裂纹铰布置在上部没有配筋的可能的弯曲破坏点上。
塑性铰的挠曲强度设为于Mcr相等,当所受的弯矩达到Mcr时,该截面即发生破坏。
图.4 二层的梁A3-B3和梁A1-A2详细配筋情况楼盖系统有沿纵向(南北向)的次梁。
图.6所示为一典型的楼盖的横截面。
为了计算出次梁和板的可能的非线性响应,用梁单元为楼盖建立模型。
次梁按T型梁计算,翼缘的计算宽度为各自板厚的四倍【5】。
选取轴2和轴3的纵梁和其之间的一个宽20英寸的梁间的格栅为板的计算模型。
为了给出板沿横向的计算模型,同样用一个宽20英寸于横梁平行的梁。
在方形的板中其剪力流和梁单元的中的不一样。
所以其扭转刚度取为整个截面刚度的一半【9】。
图.5 梁的上部配筋弯曲位置(于梁A1-A2相似,在邻近建筑靠近柱A1的地方)图.6 典型的楼盖的次梁系统图.7 实验和分析的第二层柱A3的竖向位移建筑的2、4、5、6层有填充墙,并在门窗等开口位置有过梁,如前面提到的第1、三层的填充墙,在爆除前已经拆掉。
填充墙是用良好的空隙砖砌成的,空心砖的净空是其总大小的一半。
填充墙的平面效应增强了建筑的刚度和强度,并且影响建筑的对荷载反应即变形。
如果忽略墙的影响将得不到准确的建筑的刚度和强度。
在SAP2000中考虑了两种填充墙的形式:一种是用平面框架模型(模型A),另一种是FEMA365【10】中建议的受压杆件模型(模型B)。
4.1模型A是平面框架模型,但是,现行版本的SAP2000只能计算线性框架模型,不能计算裂缝的发展情况。
填充墙的抗拉强度大概为26psi,弹性模量为644ksi【10】。
由于裂缝的发展对填充墙的刚度影响很大,重复以下步骤来计算裂缝的形成:(1)假设填充墙是线性的而且没有开裂,运行非线性历史分析。
由于梁中的塑性铰的存在,梁中弯矩大于裂缝出现弯矩时候,对截面惯性矩有一个折减。
(2)判定填充墙出现的依据是看其应力于墙的抗拉强度大小关系。
(3)节点在拉应力大于抗拉强度的地方分离。
重复上面的步骤直到裂缝区域被确定。
4.2.模型B(受压杆件模型)如FEMA356【10】所述用受压杆件来代替填充墙,杆件的方向根据移除柱后的结构变形形式和开口位置确定。
4.3.柱的移除按以下步骤模拟柱的移除。
结构是在只受永久荷载下分析的,内力在柱端测定,将随着柱的移除而卸荷。
模型的建立是在移除第一层的柱A2、A3的情况下进行的。
结构同样是在永久荷载下进行静态分析的。
在此情况下,测得的柱端内力被当成永久外部荷载施加在结构上。
注意此分析结果跟第一步的分析是等价的。
第二步中大小相等方向相反的柱端力,被瞬间施加在原柱的位置上,然后进行动态分析。
4.4.实验和分析结果的比较结构计算最大竖向位移在第二层的柱A3上,图7所示为按模型A的实验和分析的梁A3竖向位移的比较。
实验数据是用三个粘在A3两端的传感器记录的。
实验和分析得到的最大位移分别是6.1mm和6.4mm,相差尽为4%。
实验和分析的位移产生所用时间分别为0.069S和0.066S。
分析结果显示永久位移为5.3mm,比实验结果小14%,实验结果为6.1mm。
图.8.第二层的柱A3在模型A和B下分别沿时间的竖向位移图.8.比较了第二层的柱A3分别在模型A和B下分析的沿时间的竖向位移。
由图中可以看出,按受压杆件模型(模型B)得出的最大竖向位移为11.4mm,比用模型A得出的结果高出约80%。
在图.7.可以看出按模型A得出的结果与实验结果是想接近的,B模型得出的结构变形过高。
如果最大竖向位移偏大的话,填充墙开裂情况会更加严重,更偏向于受压杆件形成,模型A和模型B得出结果差异将减小。
图.9.比较了用模型A时第二层的柱A2的分析和实验的位移值。
同样,第一次达到最大位移值的实验和分析值非常接近,分析的永久位移值比实验的位移值略微低些。
图.10.所示为根据模型A得出的最大竖向位移的结构变形放大200倍后的情况。
图.9.第二层的柱A2竖向位移实验和分析结果比较图.10.按模型A,FEM分析的结构变形形式(第二层的实验得出变形形式也给出)通过实测得的变形形式在图中也用实线标出了。
在二层的梁A1-A2、A3-B3的上下端部应力重分配复杂的地方共用了14个电位计。
梁上部和对应的下部电位计接在一起用来测量梁的扭转变形。
用上下端部电位计的差值除以电位计的距离(沿梁高)。
分析推算的二层梁端部变形曲线如图中的曲线所示。
由图可以看出,分析的变形梁的变形曲线跟实验所得结果非常吻合。
根据模型A分析结果表明预示钢筋屈服的塑性铰只有两个,四个没有上部配筋的截面,到达屈服极限而开裂。
图.10.给出了所有的塑性铰及开裂位置。
Response of a reinforced concrete infilled-frame structure to removal of two adjacent columnsMehrdad Sasani_Northeastern University, 400 Snell Engineering Center, Boston, MA 02115, UnitedStatesReceived 27 June 2007; received in revised form 26 December 2007; accepted 24January 2008Available online 19 March 2008AbstractThe response of Hotel San Diego, a six-story reinforced concrete infilled-frame structure, is evaluated following the simultaneous removal of two adjacent exterior columns. Analytical models of the structure using the Finite Element Method as well as the Applied Element Method are used to calculate global and local deformations. The analytical results show good agreement with experimental data. The structure resisted progressive collapse with a measured maximum vertical displacement of only one quarter of an inch (6.4 mm). Deformation propagation over the height of the structure and the dynamic load redistribution following the column removal are experimentally and analytically evaluated and described. The difference between axial and flexural wave propagations is discussed. Three-dimensional Vierendeel (frame) action of the transverse and longitudinal frames with the participation of infill walls is identified as the major mechanism for redistribution of loads in the structure. The effects of two potential brittle modes of failure (fracture of beam sections without tensile reinforcement and reinforcing bar pull out) are described. The response of the structure due to additional gravity loads and in the absence of infill walls is analytically evaluated.c 2008 Elsevier Ltd. All rights reserved.Keywords: Progressive collapse; Load redistribution; Load resistance; Dynamicresponse; Nonlinear analysis; Brittle failure1. IntroductionAs part of mitigation programs to reduce the likelihood of mass casualties following local damage in structures, the General Services Administration [1] and the Department of Defense [2] developed regulations to evaluate progressive collapse resistance of structures. ASCE/SEI 7 [3] defines progressive collapse as the spread of an initial local failure from element to element eventually resulting in collapse of an entire structure or a disproportionately large part of it. Following the approaches proposed by Ellingwood and Leyendecker [4], ASCE/SEI 7 [3] defines two general methods for structural design of buildings to mitigate damage due to progressive collapse: indirect and direct design methods. General building codes and standards [3,5] use indirect design by increasing overall integrity of structures. Indirect design is also used in DOD [2]. Although the indirect design method can reduce the risk of progressive collapse [6,7] estimation ofpost-failure performance of structures designed based on such a method is not readily possible. One approach based on direct design methods to evaluate progressive collapse of structures is to study the effects of instantaneous removal of load-bearing elements, such as columns. GSA [1] and DOD [2] regulations require removal of one load bearing element. These regulations are meant to evaluate general integrity of structures and their capacity of redistributing the loads following severe damage to only one element. While such an approach provides insight as to the extent to which the structures are susceptible to progressive collapse, in reality, the initial damage can affect more than just one column. In this study, using analytical results that are verified against experimental data, the progressive collapse resistance of the Hotel San Diego is evaluated, following the simultaneous explosion (sudden removal) of two adjacent columns, one of which was a corner column. In order to explode the columns, explosives were inserted into predrilled holes in the columns. The columns were then well wrapped with a few layers of protective materials. Therefore, neither air blast nor flying fragments affected the structure.2. Building characteristicsHotel San Diego was constructed in 1914 with a south annex added in 1924. The annex included two separate buildings. Fig. 1 shows a south view of the hotel. Note that in the picture, the first and third stories of the hotel are covered with black fabric. The six story hotel had a non-ductile reinforced concrete (RC) frame structure with hollow clay tile exterior infill walls. The infills in the annex consisted of two wythes (layers) of clay tiles with a total thickness of about 8 in (203 mm). The height of the first floor was about 190–800 (6.00 m). The height of other floors and that of the top floor were 100–600 (3.20 m) and 160–1000 (5.13 m), respectively. Fig. 2 shows the second floor of one of the annex buildings. Fig. 3 shows a typical plan of this building, whose response following the simultaneous removal (explosion) of columns A2 and A3 in the first (ground) floor is evaluated in this paper. The floor system consisted of one-way joists running in the longitudinal direction (North–South), as shown in Fig. 3. Based on compression tests of two concrete samples, the average concrete compressive strength was estimated atabout 4500 psi (31 MPa) for a standard concrete cylinder. The modulus of elasticity of concrete was estimated at 3820 ksi (26 300 MPa) [5]. Also, based on tension tests of two steel samples having 1/2 in (12.7 mm) square sections, the yield and ultimate tensile strengths were found to be 62 ksi (427 MPa) and 87 ksi (600 MPa), respectively. The steel ultimate tensile strain was measured at 0.17. The modulus of elasticity of steel was set equal to 29 000 ksi (200 000 MPa). The building was scheduled to be demolished by implosion. As part of the demolition process, the infill walls were removed from the first and third floors. There was no live load in the building. All nonstructural elements including partitions, plumbing, and furniture were removed prior to implosion. Only beams, columns, joist floor and infill walls on the peripheral beams were present.3. SensorsConcrete and steel strain gages were used to measure changes in strains of beams and columns. Linear potentiometers were used to measure global and local deformations. The concrete strain gages were 3.5 in (90 mm) long having a maximum strain limit of ±0.02. The steel strain gages could measure up to a strain of ±0.20. The strain gages could operate up to a several hundred kHz sampling rate. The sampling rate used in the experiment was 1000 Hz. Potentiometers were used to capture rotation (integral of curvature over a length) of the beam end regions and global displacement in the building, as described later. The potentiometers had a resolution of about 0.0004 in (0.01 mm) and a maximum operational speed of about 40 in/s (1.0 m/s), while the maximum recorded speed in the experiment was about 14 in/s (0.35 m/s).4. Finite element modelUsing the finite element method (FEM), a model of the building was developed in the SAP2000 [8] computer program. The beams and columns are modeled with Bernoulli beam elements. Beams have T or L sections with effective flange width on each side of the web equal to four times the slab thickness [5]. Plastic hinges are assigned to all possible locations where steel bar yielding can occur, including the ends of elements as well as the reinforcing bar cut-off and bend locations. The characteristics of the plastic hinges are obtained using section analyses of the beams and columns and assuming a plastic hinge length equal to half of the section depth. The current version of SAP2000 [8] is not able to track formation of cracks in the elements. In order to find the proper flexural stiffness of sections, an iterative procedure is used as follows. First, the building is analyzed assuming all elements are uncracked. Then, moment demands in the elements are compared with their cracking bending moments, Mcr . The moment of inertia of beam and slab segments are reduced by a coefficient of 0.35 [5], where the demand exceeds the Mcr. The exterior beam cracking bending moments under negative and positive moments, are 516 k in (58.2 kN m) and 336 k in (37.9 kN m), respectively. Note that no cracks were formed in the columns. Then the building is reanalyzed and moment diagrams are re-evaluated. This procedure is repeated until all of the cracked regions are properly identified and modeled.The beams in the building did not have top reinforcing bars except at the end regions (see Fig. 4). For instance, no top reinforcement was provided beyond the bend in beam A1–A2, 12 inches away from the face of column A1 (see Figs. 4 and 5). To model the potential loss of flexural strength in those sections, localized crack hinges were assigned at the critical locations where no top rebar was present. Flexural strengths of the hinges were set equal to Mcr. Such sections were assumed to lose their flexural strength when the imposed bending moments reached Mcr.The floor system consisted of joists in the longitudinal direction (North–South). Fig. 6 shows the cross section of a typical floor. In order to account for potential nonlinear response of slabs and joists, floors are molded by beam elements. Joists are modeled with T-sections, having effective flange width on each side of the web equal to four times the slab thickness [5]. Given the large joist spacing between axes 2 and 3, two rectangular beam elements with 20-inch wide sections are used between the joist and the longitudinal beams of axes 2 and 3 to model the slab in the longitudinal direction. To model the behavior of the slab in the transverse direction, equally spaced parallel beams with 20-inch wide rectangular sections are used. There is a difference between the shear flow in the slab and that in the beam elements with rectangular sections modeling the slab. Because of this, the torsional stiffness is setequal to one-half of that of the gross sections [9].The building had infill walls on 2nd, 4th, 5th and 6th floors on the spandrel beams with some openings (i.e. windows and doors). As mentioned before and as part of the demolition procedure, the infill walls in the 1st and 3rd floors were removed before the test. The infill walls were made of hollow clay tiles, which were in good condition. The net area of the clay tiles was about 1/2 of the gross area. The in-plane action of the infill walls contributes to the building stiffness and strength and affects the building response. Ignoring the effects of the infill wallsand excluding them in the model would result in underestimating the building stiffness and strength.Using the SAP2000 computer program [8], two types of modeling for the infills are considered in this study: one uses two dimensional shell elements (Model A) and the other uses compressive struts (Model B) as suggested in FEMA356 [10] guidelines.4.1. Model A (infills modeled by shell elements)Infill walls are modeled with shell elements. However, the current version of the SAP2000 computer program includes only linear shell elements and cannot account for cracking. The tensile strength of the infill walls is set equal to 26 psi, with a modulus of elasticity of 644 ksi [10]. Because the formation ofcracks has a significant effect on the stiffness of the infill walls, the following iterative procedure is used to account for crack formation:(1) Assuming the infill walls are linear and uncracked, a nonlinear time history analysis is run. Note that plastic hinges exist in the beam elements and the segments of the beam elements where moment demand exceeds the cracking moment have a reduced moment of inertia.(2) The cracking pattern in the infill wall is determined by comparing stresses in the shells developed during the analysis with the tensile strength of infills.(3) Nodes are separated at the locations where tensile stress exceeds tensile strength. These steps are continued until the crack regions are properly modeled.4.2. Model B (infills modeled by struts)Infill walls are replaced with compressive struts as described in FEMA 356 [10] guidelines. Orientations of the struts are determined from the deformed shape of the structure after column removal and the location of openings.4.3. Column removalRemoval of the columns is simulated with the following procedure.(1) The structure is analyzed under the permanent loads and the internal forces are determined at the ends of the columns, which will be removed.(2) The model is modified by removing columns A2 and A3 on the first floor. Again the structure is statically analyzed under permanent loads. In this case, the internal forces at the ends of removed columns found in the first step are applied externally to the structure along with permanent loads. Note that the results of this analysis are identical to those of step 1.(3) The equal and opposite column end forces that were applied in the secondstep are dynamically imposed on the ends of the removed column within one millisecond [11] to simulate the removal of the columns, and dynamic analysis is conducted.4.4. Comparison of analytical and experimental resultsThe maximum calculated vertical displacement of the building occurs at joint A3 in the second floor. Fig. 7 shows the experimental and analytical (Model A) vertical displacements of this joint (the AEM results will be discussed in the next section). Experimental data is obtained using the recordings of three potentiometers attached to joint A3 on one of their ends, and to the ground on the other ends. The peak displacements obtained experimentally and analytically (Model A) are 0.242 in (6.1 mm) and 0.252 in (6.4 mm), respectively, which differ only by about 4%. The experimental and analytical times corresponding to peak displacement are 0.069 s and 0.066 s, respectively. The analytical results show a permanent displacement of about 0.208 in (5.3 mm), which is about 14% smaller than the corresponding experimental value of 0.242 in (6.1 mm).Fig. 8 compares vertical displacement histories of joint A3 in the second floor estimated analytically based on Models A and B. As can be seen, modeling infills with struts (Model B) results in a maximum vertical displacement of joint A3 equal to about 0.45 in (11.4 mm), which is approximately 80% larger than the value obtained from Model A. Note that the results obtained from Model A are in close agreement with experimental results (see Fig. 7), while Model B significantly overestimates the deformation of the structure. If the maximum vertical displacement were larger, the infill walls were more severely cracked and the struts were more completely formed, the difference between the results of the two models (Models A and B) would be smaller.Fig. 9 compares the experimental and analytical (Model A) displacement of joint A2 in the second floor. Again, while the first peak vertical displacement obtained experimentally and analytically are in good agreement, the analytical permanent displacement under estimates the experimental value.Analytically estimated deformed shapes of the structure at the maximum vertical displacement based on Model A are shown in Fig. 10 with a magnification factor of 200. The experimentally measured deformed shape over the end regions of beams A1–A2 and A3–B3 in the second floorare represented in the figure by solid lines. A total of 14 potentiometers were located at the top and bottom of the end regions of the second floor beams A1–A2 and A3–B3, which were the most critical elements in load redistribution. The beam top and corresponding bottom potentiometer recordings were used to calculate rotation between the sections where the potentiometer ends were connected. This was done by first finding the difference between the recorded deformations at the top and bottom of the beam, and then dividing the value by the distance (along the height of the beam section) between the two potentiometers. The expected deformed shapes between the measured end regions of the second floor beams areshown by dashed lines. As can be seen in the figures, analytically estimated deformed shapes of the beams are in good agreement with experimentally obtained deformed shapes.Analytical results of Model A show that only two plastic hinges are formed indicating rebar yielding. Also, four sections that did not have negative (top) reinforcement, reached cracking moment capacities and therefore cracked. Fig.10 shows the locations of all the formed plastic hinges and cracks.。