毕业设计冷凝器外文翻译
模具毕业设计外文翻译(英文+译文)
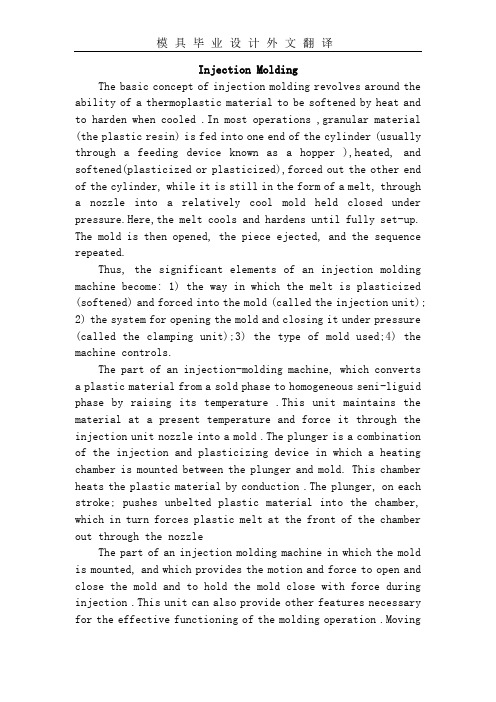
Injection MoldingThe basic concept of injection molding revolves around the ability of a thermoplastic material to be softened by heat and to harden when cooled .In most operations ,granular material (the plastic resin) is fed into one end of the cylinder (usually through a feeding device known as a hopper ),heated, and softened(plasticized or plasticized),forced out the other end of the cylinder, while it is still in the form of a melt, through a nozzle into a relatively cool mold held closed under pressure.Here,the melt cools and hardens until fully set-up. The mold is then opened, the piece ejected, and the sequence repeated.Thus, the significant elements of an injection molding machine become: 1) the way in which the melt is plasticized (softened) and forced into the mold (called the injection unit);2) the system for opening the mold and closing it under pressure (called the clamping unit);3) the type of mold used;4) the machine controls.The part of an injection-molding machine, which converts a plastic material from a sold phase to homogeneous seni-liguid phase by raising its temperature .This unit maintains the material at a present temperature and force it through the injection unit nozzle into a mold .The plunger is a combination of the injection and plasticizing device in which a heating chamber is mounted between the plunger and mold. This chamber heats the plastic material by conduction .The plunger, on each stroke; pushes unbelted plastic material into the chamber, which in turn forces plastic melt at the front of the chamber out through the nozzleThe part of an injection molding machine in which the mold is mounted, and which provides the motion and force to open and close the mold and to hold the mold close with force during injection .This unit can also provide other features necessary for the effective functioning of the molding operation .Movingplate is the member of the clamping unit, which is moved toward a stationary member. the moving section of the mold is bolted to this moving plate .This member usually includes the ejector holes and mold mounting pattern of blot holes or “T” slots .Stationary plate is the fixed member of the clamping unit on which the stationary section of the mold is bolted .This member usually includes a mold-mounting pattern of boles or “T” slots. Tie rods are member of the clamping force actuating mechanism that serve as the tension member of the clamp when it is holding the mold closed. They also serve as a gutted member for the movable plate .Ejector is a provision in the clamping unit that actuates a mechanism within the mold to eject the molded part(s) from the mold .The ejection actuating force may be applied hydraulically or pneumatically by a cylinder(s) attached to the moving plate, or mechanically by the opening stroke of the moving plate.Methods of melting and injecting the plastic differ from one machine to another and are constantly being implored .conventional machines use a cylinder and piston to do both jobs .This method simplifies machine construction but makes control of injection temperatures and pressures an inherently difficult problem .Other machines use a plasticizing extruder to melt the plastic and piston to inject it while some hare been designed to use a screw for both jobs :Nowadays, sixty percent of the machines use a reciprocating screw,35% a plunger (concentrated in the smaller machine size),and 5%a screw pot.Many of the problems connected with in ejection molding arise because the densities of polymers change so markedly with temperature and pressure. thigh temperatures, the density of a polymer is considerably cower than at room temperature, provided the pressure is the same.Therefore,if molds were filled at atmospheric pressure, “shrinkage” would make the molding deviate form the shape of the mold.To compensate for this poor effect, molds are filled at high pressure. The pressure compresses the polymer and allows more materials to flow into the mold, shrinkage is reduced and better quality moldings are produced.Cludes a mold-mounting pattern of bolt holes or “T” slots. Tie rods are members of the clamping force actuating mechanism that serve as the tension members of clamp when it is holding the mold closed. Ejector is a provision in the calming unit that actuates a mechanism within the mold to eject the molded part(s) form the mold. The ejection actuating force may be applied hydraulically or pneumatically by a cylinder(s) attached to the moving plate, or mechanically by the opening stroke of the moving plate.The function of a mold is twofold: imparting the desired shape to the plasticized polymer and cooling the injection molded part. It is basically made up of two sets of components: the cavities and cores and the base in which the cavities and cores are mounted. The mold ,which contains one or more cavities, consists of two basic parts :(1) a stationary molds half one the side where the plastic is injected,(2)Moving half on the closing or ejector side of the machine. The separation between the two mold halves is called the parting line. In some cases the cavity is partly in the stationary and partly in the moving section. The size and weight of the molded parts limit the number of cavities in the mold and also determine the machinery capacity required. The mold components and their functions are as following:(1)Mold Base-Hold cavity (cavities) in fixed, correctposition relative to machine nozzle.(2)Guide Pins-Maintain Proper alignment of entry into moldinterior.(3)Spree Bushing (spree)-Provide means of entry into moldinterior.(4)Runners-Conroy molten plastic from spree to cavities.(5)Gates-Control flow into cavities.(6)Cavity (female) and Force (male)-Control the size,shape and surface of mold article.(7)Water Channels-Control the temperature of mold surfacesto chill plastic to rigid state.(8)Side (actuated by came, gears or hydrauliccylinders)-Form side holes, slots, undercuts and threaded sections.(9)Vent-Allow the escape of trapped air and gas.(10)Ejector Mechanism (pins, blades, stripper plate)-Ejectrigid molded article form cavity or force.(11)Ejector Return Pins-Return ejector pins to retractedposition as mold closes for next cycle.The distance between the outer cavities and the primary spree must not be so long that the molten plastic loses too much heat in the runner to fill the outer cavities properly. The cavities should be so arranged around the primary spree that each receives its full and equal share of the total pressure available, through its own runner system (or the so-called balanced runner system).The requires the shortest possible distance between cavities and primary sprue, equal runner and gate dimension, and uniform culling.注射成型注射成型的基本概念是使热塑性材料在受热时熔融,冷却时硬化,在大部分加工中,粒状材料(即塑料树脂)从料筒的一端(通常通过一个叫做“料斗”的进料装置)送进,受热并熔融(即塑化或增塑),然后当材料还是溶体时,通过一个喷嘴从料筒的另一端挤到一个相对较冷的压和封闭的模子里。
电气自动化专业毕业设计外文翻译(中英文对照翻译)-制冷压缩机速度的模糊控制
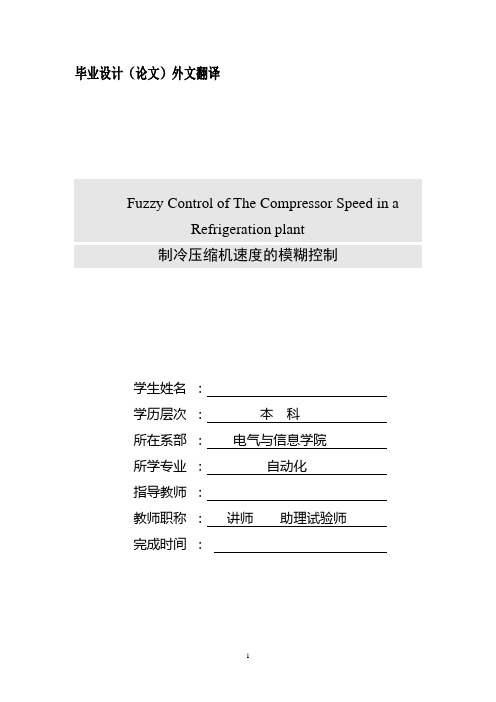
毕业设计(论文)外文翻译Fuzzy Control of The Compressor Speed in aRefrigeration plant制冷压缩机速度的模糊控制制冷压缩机速度的模糊控制摘要在这篇文章里,所提到的是在通常应用于商业上的蒸汽压缩制冷装之中,用模糊控制算法控制制冷压缩机的速度使之达到最有效的速度来控制冷气的温度。
它主要的目标是根据模糊控制算法,通过变换器对压缩机速度进行连续调控,并估算节能效果;不同于传统恒温控制,这里通过控制压缩机冷藏容量,施加给控制压缩机50Hz的开关运转频率。
通过控制压缩机的电动机的供电电流达到的速度变化范围是30-50Hz,由于转动频率过低会有因飞溅系统而出现的润滑问题,现今所提供的压缩机转动频率一般不考虑小于30Hz的。
在这个范围,在二个最适当的工作流体之中,可以代替R22有很多,例如R407C (R32/R125/R134a 23/25/52%组)和R507 (R125/R143A 50/50%组)比较好。
压缩机速度模糊控制与传统的温度控制相比,更多的用于冷藏和其他制冷系统。
实验结果表明,当R407C 作为工作流体时,可以达到显著的节能效果,( 13%)。
值得注意的是,从节能观点看,当压缩机速度变化时可以达到的最佳的效果。
另外,考虑到变换器费用问题,回收期要比可接受的产品型号更具有决定性。
关键词:压缩系统; 冷室; 活塞式压缩机; 易变的速度; 章程; 模糊逻辑;R407C; R5071引言蒸气压缩冷却装置,虽则被设计满足最大载荷,但为了延长寿命,通常在部分装载下工作,并通过开关周期调控,在50 Hz的频率下运作,这样就决定了高能消耗量的恒温控制。
而且,制冷时耗电量低被认为间接的释放了温室气体; 改进上述的系统的能量转换效率可以减少这种排放物。
各种各样的冷藏容量控制方法和部分装载理论表明压缩机速度变异是最高效率的技术。
[1,2]。
冷藏容量控制这个方法在最近3–10年已经被分析研究,包括提高压缩机的速度以不断的达到制冷效果。
暖通空调专业-毕业设计外文翻译

Refrigeration System Performance using Liquid-Suction Heat ExchangersS. A. Klein, D. T. Reindl, and K. BroWnellCollege of EngineeringUniversity of Wisconsin - MadisonAbstractHeat transfer devices are provided in many refrigeration systems to exchange energy betWeen the cool gaseous refrigerant leaving the evaporator and Warm liquid refrigerant exiting the condenser. These liquid-suction or suction-line heat exchangers can, in some cases, yield improved system performance While in other cases they degrade system performance. Although previous researchers have investigated performance of liquid-suction heat exchangers, this study can be distinguished from the previous studies in three Ways. First, this paper identifies a neW dimensionless group to correlate performance impacts attributable to liquid-suction heat exchangers. Second, the paper extends previous analyses to include neW refrigerants. Third, the analysis includes the impact of pressure drops through the liquid-suction heat exchanger on system performance. It is shoWn that reliance on simplified analysis techniques can lead to inaccurate conclusions regarding the impact of liquid-suction heat exchangers on refrigeration system performance. From detailed analyses, it can be concluded that liquid-suction heat exchangers that have a minimal pressure loss on the loW pressure side are useful for systems using R507A, R134a, R12, R404A, R290, R407C, R600, and R410A. The liquid-suction heat exchanger is detrimental to system performance in systems using R22, R32, and R717.IntroductionLiquid-suction heat exchangers are commonly installed in refrigeration systems With the intent of ensuring proper system operation and increasing system performance.Specifically, ASHRAE(1998) states that liquid-suction heat exchangers are effective in:1) increasing the system performance2) subcooling liquid refrigerant to prevent flash gas formation at inlets to expansion devices3) fully evaporating any residual liquid that may remain in the liquid-suction prior to reaching the compressor(s)Figure 1 illustrates a simple direct-expansion vapor compression refrigeration system utilizing a liquid-suction heat exchanger. In this configuration, high temperature liquid leaving the heat rejection device (an evaporative condenser in this case) is subcooled prior to being throttled to the evaporator pressure by an expansion device such as a thermostatic expansion valve. The sink for subcoolingthe liquid is loW temperature refrigerant vapor leaving the evaporator. Thus, the liquid-suction heat exchanger is an indirect liquid-to-vapor heat transfer device. The vapor-side of the heat exchanger (betWeen the evaporator outlet and the compressor suction) is often configured to serve as an accumulator thereby further minimizing the risk of liquid refrigerant carrying-over to the compressor suction. In cases Where the evaporator alloWs liquid carry-over, the accumulator portion of the heat exchanger Will trap and, over time, vaporize the liquid carryover by absorbing heat during the process of subcooling high-side liquid.BackgroundStoecker and Walukas (1981) focused on the influence of liquid-suction heat exchangers in both single temperature evaporator and dual temperature evaporator systems utilizing refrigerant mixtures. Their analysis indicated that liquid-suction heat exchangers yielded greater performance improvements When nonazeotropic mixtures Were used compared With systems utilizing single component refrigerants or azeoptropic mixtures. McLinden (1990) used the principle of corresponding states to evaluate the anticipated effects of neW refrigerants. He shoWed that the performance of a system using a liquid-suction heat exchanger increases as the ideal gas specific heat (related to the molecular complexity of the refrigerant) increases. Domanski and Didion (1993) evaluated the performance of nine alternatives to R22 including the impact of liquid-suction heat exchangers. Domanski et al. (1994) later extended the analysis by evaluating the influence of liquid-suction heat exchangers installed in vapor compression refrigeration systems considering 29 different refrigerants in a theoretical analysis. Bivens et al. (1994) evaluated a proposed mixture to substitute for R22 in air conditioners and heat pumps. Their analysis indicated a 6-7% improvement for the alternative refrigerant system When system modifications included a liquid-suction heat exchanger and counterfloW system heat exchangers (evaporator and condenser). Bittle et al. (1995a) conducted an experimental evaluation of a liquid-suction heat exchanger applied in a domestic refrigerator using R152a. The authors compared the system performance With that of a traditional R12-based system. Bittle et al. (1995b) also compared the ASHRAE method for predicting capillary tube performance (including the effects of liquid-suction heat exchangers) With experimental data. Predicted capillary tube mass floW rates Were Within 10% of predicted values and subcooling levels Were Within 1.7 C (3F) of actual measurements.This paper analyzes the liquid-suction heat exchanger to quantify its impact on system capacity and performance (expressed in terms of a system coefficient of performance, COP). The influence of liquid-suction heat exchanger size over a range of operating conditions (evaporating and condensing) is illustrated and quantified using a number of alternative refrigerants. Refrigerants included in the present analysis are R507A, R404A, R600, R290,R134a, R407C, R410A, R12, R22, R32, and R717. This paper extends the results presented in previous studies in that it considers neW refrigerants, it specifically considers the effects of the pressure drops,and it presents general relations for estimating the effect of liquid-suction heat exchangers for any refrigerant.Heat Exchanger EffectivenessThe ability of a liquid-suction heat exchanger to transfer energy from the Warm liquid to the cool vapor at steady-state conditions is dependent on the size and configuration of the heat transfer device. The liquid-suction heat exchanger performance, expressed in terms of an effectiveness, is a parameter in the analysis. The effectiveness of the liquid-suction heat exchanger is defined in equation (1):Where the numeric subscripted temperature (T) values correspond to locations depicted in Figure 1. The effectiveness is the ratio of the actual to maximum possible heat transfer rates. It is related to the surface area of the heat exchanger. A zero surface area represents a system Without a liquid-suction heat exchanger Whereas a system having an infinite heat exchanger area corresponds to an effectiveness of unity.The liquid-suction heat exchanger effects the performance of a refrigeration system by in fluencing both the high and loW pressure sides of a system. Figure 2 shoWs the key state points for a vapor compression cycle utilizing an idealized liquid-suction heat exchanger on a pressure-enthalpy diagram. The enthalpy of the refrigerant leaving the condenser (state 3) is decreased prior to entering the expansion device (state 4) by rejecting energy to the vapor refrigerant leaving the evaporator (state 1) prior to entering the compressor (state 2). Pressure losses are not shoWn. The cooling of the condensate that occurs on the high pressure side serves to increase the refrigeration capacity and reduce the likelihood of liquid refrigerant flashing prior to reaching the expansion device. On the loW pressure side, the liquid-suction heat exchanger increases the temperature of the vapor entering the compressor and reduces the refrigerant pressure, both of Which increase the specific volume of the refr igerant and thereby decrease the mass floW rate and capacity. A major benefit of the liquid-suction heat exchanger is that it reduces the possibility of liquid carry-over from the evaporator Which could harm the compressor. Liquid carryover can be readily caused by a number of factors that may include Wide fluctuations in evaporator load and poorly maintained expansiondevices (especially problematic for thermostatic expansion valves used in ammonia service).(翻译)冷却系统利用流体吸热交换器克来因教授,布兰顿教授, , 布朗教授威斯康辛州的大学–麦迪逊摘录加热装置在许多冷却系统中被用到,用以制冷时遗留在蒸发器中的冷却气体和离开冷凝器发热流体之间的能量的热交换.这些流体吸收或吸收热交换器,在一些情形中,他们降低了系统性能, 然而系统的某些地方却得到了改善. 虽然以前研究员已经调查了流体吸热交换器的性能, 但是这项研究可能从早先研究的三种方式被加以区别. 首先,这份研究开辟了一个无限的崭新的与流体吸热交换器有关联的群体.其次,这份研究拓宽了早先的分析包括新型制冷剂。
暖通空调专业 毕业设计外文翻译3
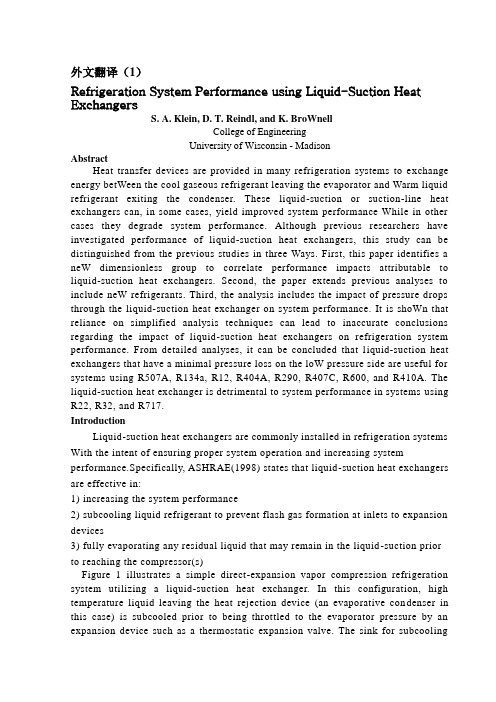
外文翻译(1)Refrigeration System Performance using Liquid-Suction Heat ExchangersS. A. Klein, D. T. Reindl, and K. BroWnellCollege of EngineeringUniversity of Wisconsin - MadisonAbstractHeat transfer devices are provided in many refrigeration systems to e xchange energy betWeen the cool gaseous refrigerant leaving the evaporator and Warm liquid refrigerant exiting the condenser. These liquid-suction or suction-line heat exchangers can, in some cases, yield improved system performance While in other cases they degrade system performance. Although previous researchers have investigated performance of liquid-suction heat exchangers, this study can be distinguished from the previous studies in three Ways. First, this paper identifies a neW dimensionless group to correlate performance impacts attributable to liquid-suction heat exchangers. Second, the paper extends previous analyses to include neW refrigerants. Third, the analysis includes the impact of pressure drops through the liquid-suction heat exchanger on system performance. It is shoWn that reliance on simplified analysis techniques can lead to inaccurate conclusions regarding the impact of liquid-suction heat exchangers on refrigeration system performance. From detailed analyses, it can be concluded that liquid-suction heat exchangers that have a minimal pressure loss on the loW pressure side are useful for systems using R507A, R134a, R12, R404A, R290, R407C, R600, and R410A. The liquid-suction heat exchanger is detrimental to system performance in systems using R22, R32, and R717.IntroductionLiquid-suction heat exchangers are commonly installed in refrigeration systems With the intent of ensuring proper system operation and increasing system performance.Specifically, ASHRAE(1998) states that liquid-suction heat exchangers are effective in:1) increasing the system performance2) subcooling liquid refrigerant to prevent flash gas formation at inlets to expansion devices3) fully evaporating any residual liquid that may remain in the liquid-suction prior to reaching the compressor(s)Figure 1 illustrates a simple direct-expansion vapor compression refrigeration system utilizing a liquid-suction heat exchanger. In this configuration, high temperature liquid leaving the heat rejection device (an evaporative con denser in this case) is subcooled prior to being throttled to the evaporator pressure by an expansion device such as a thermostatic expansion valve. The sink for subcoolingthe liquid is loW temperature refrigerant vapor leaving the evaporator. Thus, the liquid-suction heat exchanger is an indirect liquid-to-vapor heat transfer device. The vapor-side of the heat exchanger (betWeen the evaporator outlet and the compressor suction) is often configured to serve as an accumulator thereby further minimizing the risk of liquid refrigerant carrying-over to the compressor suction. In cases Where the evaporator alloWs liquid carry-over, the accumulator portion of the heat exchanger Will trap and, over time, vaporize the liquid carryover by absorbing heat during the process of subcooling high-side liquid.BackgroundStoecker and Walukas (1981) focused on the influence of liquid-suction heat exchangers in both single temperature evaporator and dual temperature evaporator systems utilizing refrigerant mixtures. Their analysis indicated that liquid-suction heat exchangers yielded greater performance improvements When nonazeotropic mixtures Were used compared With systems utilizing single component refrigerants or azeoptropic mixtures. McLinden (1990) used the principle of corresponding states to evaluate the anticipated effects of neW refrigerants. He shoWed that the performance of a system using a liquid-suction heat exchanger increases as the ideal gas specific heat (related to the molecular complexity of the refrigerant) increases. Domanski and Didion (1993) evaluated the performance of nine alternatives to R22 including the impact of liquid-suction heat exchangers. Domanski et al. (1994) later extended the analysis by evaluating the influence of liquid-suction heat exchangers installed in vapor compression refrigeration systems considering 29 different refrigerants in a theoretical analysis. Bivens et al. (1994) evaluated a proposed mixture to substitute for R22 in air conditioners and heat pumps. Their analysis indicated a 6-7% improvement for the alternative refrigerant system When system modifications included a liquid-suction heat exchanger and counterfloW system heat exchangers (evaporator and condenser). Bittle et al. (1995a) conducted an experimental evaluation of a liquid-suction heat exchanger applied in a domestic refrigerator using R152a. The authors compared the system performance With that of a traditional R12-based system. Bittle et al. (1995b) also compared the ASHRAE method for predicting capillary tube performance (including the effects of liquid-suction heat exchangers) With experimental data. Predicted capillary tube mass floW rates Were Within 10% of predicted values and subcooling levels Were Within 1.7 C (3F) of actual measurements.This paper analyzes the liquid-suction heat exchanger to quantify its impact on system capacity and performance (expressed in terms of a system coefficient of performance, COP). The influence of liquid-suction heat exchanger size over a range of operating conditions (evaporating and condensing) is illustrated and quantified using a number of alternative refrigerants. Refrigerants included in the present analysis are R507A, R404A, R600, R290,R134a, R407C, R410A, R12, R22, R32, and R717. This paper extends the results presented in previous studies in that it considers neW refrigerants, it specifically considers the effects of the pressure drops,and it presents general relations for estimating the effect of liquid-suction heat exchangers for any refrigerant.Heat Exchanger EffectivenessThe ability of a liquid-suction heat exchanger to transfer energy from the Warm liquid to the cool vapor at steady-state conditions is dependent on the size and configuration of the heat transfer device. The liquid-suction heat exchanger performance, expressed in terms of an effectiveness, is a parameter in the analysis. The effectiveness of the liquid-suction heat exchanger is defined in equation (1):Where the numeric subscripted temperature (T) values correspond to locations depicted in Figure 1. The effectiveness is the ratio of the actual to maximum possible heat transfer rates. It is related to the surface area of the heat exchanger. A zero surface area represents a system Without a liquid-suction heat exchanger Whereas a system having an infinite heat exchanger area corresponds to an effectiveness of unity.The liquid-suction heat exchanger effects the performance of a refrigeration system by in fluencing both the high and loW pressure sides of a system. Figure 2 shoWs the key state points for a vapor compression cycle utilizing an idealized liquid-suction heat exchanger on a pressure-enthalpy diagram. The enthalpy of the refrigerant leaving the condenser (state 3) is decreased prior to entering the expansion device (state 4) by rejecting energy to the vapor refrigerant leaving the evaporator (state 1) prior to entering the compressor (state 2). Pressure losses are not shoWn. The cooling of the condensate that occurs on the high pressure side serves to increase the refrigeration capacity and reduce the likelihood of liquid refrigerant flashing prior to reaching the expansion device. On the loW pressure side, the liquid-suction heat exchanger increases the temperature of the vapor entering the compressor and reduces the refrigerant pressure, both of Which increase the specific volume of the refr igerant and thereby decrease the mass floW rate and capacity. A major benefit of the liquid-suction heat exchanger is that it reduces the possibility of liquid carry-over from the evaporator Which could harm the compressor. Liquid carryover can be readily caused by a number of factors that may include Wide fluctuations in evaporator load and poorly maintained expansiondevices (especially problematic for thermostatic expansion valves used in ammonia service).(翻译)冷却系统利用流体吸热交换器克来因教授,布兰顿教授, , 布朗教授威斯康辛州的大学–麦迪逊摘录加热装置在许多冷却系统中被用到,用以制冷时遗留在蒸发器中的冷却气体和离开冷凝器发热流体之间的能量的热交换.这些流体吸收或吸收热交换器,在一些情形中,他们降低了系统性能, 然而系统的某些地方却得到了改善. 虽然以前研究员已经调查了流体吸热交换器的性能, 但是这项研究可能从早先研究的三种方式被加以区别. 首先,这份研究开辟了一个无限的崭新的与流体吸热交换器有关联的群体.其次,这份研究拓宽了早先的分析包括新型制冷剂。
冷水机组 毕业设计外文翻译

英文翻译Chilled Water Systems[1]Chilled water systems were used in less than 4% of commercial buildings in the U.S. in 1995. However, because chillers are usually installed in larger buildings, chillers cooled over 28% of the U.S. commercial building floor space that same year (DOE, 1998). Five types of chillers are commonly applied to commercial buildings: reciprocating, screw, scroll, centrifugal, and absorption. The first four utilize the vapor compression cycle to produce chilled water. They differ primarily in the type of compressor used. Absorption chillers utilize thermal energy (typically steam or combustion source) in an absorption cycle with either an ammonia-water or water-lithium bromide solution to produce chilled water.Overall SystemFigure 4.2.2 shows a simple representation of a dual chiller application with all the major auxiliary equipment. An estimated 86% of chillers are applied in multiple chiller arrangements like that shown in the figure (Bitondo and Tozzi, 1999). In chilled water systems, return water from the building is circulated through each chiller evaporator where it is cooled to an acceptable temperature (typically 4 to 7°C) (39 to 45°F). The chilled water is then distributed to water-to-air heat exchangers spread throughout the facility. In these heat exchangers, air is cooled and dehumidified by the cold water. During the process, the chilled water increases in temperature and must be returned to the chiller(s).The chillers shown in Figure 4.2.2 are water-cooled chillers. Water is circulated through the condenser of each chiller where it absorbs heat energy rejected from the high pressure refrigerant. The water is then pumped to a cooling tower where the water is cooled through an evaporation process. Cooling towers are described in a later section. Chillers can also be air cooled. In this configuration, the condenserwould be a refrigerant-to-air heat exchanger with air absorbing the heat energy rejected by the high pressure refrigerant.Chillers nominally range in capacities from 30 to 18,000 kW (8 to 5100 ton). Most chillers sold in the U.S. are electric and utilize vapor compression refrigeration to produce chilled water. Compressors for these systems are either reciprocating, screw, scroll, or centrifugal in design. A small number of centrifugal chillers are sold that use either an internal combustion engine or steam drive instead of an electric motor to drive the compressor.[1]节选自James B. Bradford et al. “HVAC Equipment and Systems”.Handbook of Heating, Ventilation, and Air-Conditioning.Ed. Jan F. Kreider.Boca Raton, CRC Press LLC. 2001FIGURE 4.2.2 A dual chiller application with major auxiliary systems (courtesy of Carrier Corporation).The type of chiller used in a building depends on the application. For large office buildings or in chiller plants serving multiple buildings, centrifugal compressors are often used. In applications under 1000 kW (280 tons) cooling capacities, reciprocating or screw chillers may be more appropriate. In smaller applications, below 100 kW (30 tons), reciprocating or scroll chillers are typically used.Vapor Compression ChillersTable 4.2.5 shows the nominal capacity ranges for the four types of electrically driven vapor compression chillers. Each chiller derives its name from the type of compressor used in the chiller. The systems range in capacities from the smallest scroll (30 kW; 8 tons) to the largest centrifugal (18,000 kW; 5000 tons).Chillers can utilize either an HCFC (R-22 andR-123) or HFC (R-134a) refrigerant. The steady state efficiency of chillers is often stated as a ratio of the power input (in kW) to the chilling capacity (in tons). A capacity rating of one ton is equal to 3.52 kW or 12,000 btu/h. With this measure of efficiency, the smaller number is better. As seen in Table 4.2.5, centrifugal chillers are the most efficient; whereas, reciprocating chillers have the worst efficiency of the four types. The efficiency numbers provided in the table are the steady state full-load efficiency determined in accordance to ASHRAE Standard 30 (ASHRAE, 1995). These efficiency numbers do not include the auxiliary equipment, such as pumps and cooling tower fans that can add from 0.06 to 0.31 kW/ton to the numbers shown (Smit et al., 1996).Chillers run at part load capacity most of the time. Only during the highest thermal loadsin the building will a chiller operate near its rated capacity. As a consequence, it is important to know how the efficiency of the chiller varies with part load capacity. Figure 4.2.3 shows a representative data for the efficiency (in kW/ton) as a function of percentage full load capacity for a reciprocating, screw, and scroll chiller plus a centrifugal chiller with inlet vane control and one with variable frequency drive (VFD) for the compressor. The reciprocating chiller increases in efficiency as it operates at a smaller percentage of full load. In contrast, the efficiency of a centrifugal with inlet vane control is relatively constant until theload falls to about 60% of its rated capacity and its kW/ton increases to almost twice its fully loaded value.FIGURE 4.2.3 Chiller efficiency as a function of percentage of full load capacity.In 1998, the Air Conditioning and Refrigeration Institute (ARI) developed a new standard that incorporates into their ratings part load performance of chillers (ARI 1998c). Part load efficiency is expressed by a single number called the integrated part load value (IPLV). The IPLV takes data similar to that in Figure 4.2.3 and weights it at the 25%, 50%,75%, and 100% loads to produce a single integrated efficiency number. The weighting factors at these loads are 0.12, 0.45, 0.42, and 0.01, respectively. The equation to determine IPLV is:Most of the IPLV is determined by the efficiency at the 50% and 75% part load values. Manufacturers will provide, on request, IPLVs as well as part load efficiencies such as those shown in Figure 4.2.3.FIGURE 4.2.4 Volume-pressure relationships for a reciprocating compressor.The four compressors used in vapor compression chillers are each briefly described below. While centrifugal and screw compressors are primarily used in chiller applications, reciprocating and scroll compressors are also used in smaller unitary packaged air conditioners and heat pumps.Reciprocating CompressorsThe reciprocating compressor is a positive displacement compressor. On the intake stroke of the piston, a fixed amount of gas is pulled into the cylinder. On the compressionstroke, the gas is compressed until the discharge valve opens. The quantity of gas compressed on each stroke is equal to the displacement of the cylinder. Compressors used in chillers have multiple cylinders, depending on the capacity of the compressor. Reciprocating compressors use refrigerants with low specific volumes and relatively high pressures. Most reciprocating chillers used in building applications currently employ R-22.Modern high-speed reciprocating compressors are generally limited to a pressure ratio of approximately nine. The reciprocating compressor is basically a constant-volumevariable-head machine. It handles variousdischarge pressures with relatively small changes in inlet-volume flow rate as shown by the heavy line (labeled 16 cylinders) in Figure 4.2.4. Condenser operation in many chillers is related to ambient conditions, for example, through cooling towers, so that on cooler days the condenser pressure can be reduced. When the air conditioning load is lowered, less refrigerant circulation is required. The resulting load characteristic is represented by the solid line that runs from the upper right to lower left of Figure 4.2.4.The compressor must be capable of matching the pressure and flow requirements imposed by the system. The reciprocating compressor matches the imposed discharge pressure at any level up to its limiting pressure ratio. Varying capacity requirements can be met by providing devices that unloadindividual or multiple cylinders. This unloading is accomplished by blocking the suction or discharge valves that open either manually or automatically. Capacity can also be controlled through the use of variable speed or multi-speed motors. When capacity control is implemented on a compressor, other factors at part-load conditions need to considered, such as (a) effect on compressor vibration and sound when unloaders are used, (b) the need for good oil return because of lower refrigerant velocities, and (c) proper functioning of expansion devices at the lower capacities.With most reciprocating compressors, oil is pumped into the refrigeration system from the compressor during normal operation. Systems must be designed carefully to return oil to the compressor crankcase to provide for continuous lubrication and also to avoid contaminating heat-exchanger surfaces.Reciprocating compressors usually are arranged to start unloaded so that normal torque motors are adequate for starting. When gas engines are used for reciprocating compressor drives, careful matching of the torque requirements of the compressor and engine must be considered.FIGURE 4.2.5 Illustration of a twin-screw compressor design (courtesy of CarrierCorporation).Screw CompressorsScrew compressors, first introduced in 1958 (Thevenot, 1979), are positive displacement compressors. They are available in the capacity ranges that overlap with reciprocating compressors and small centrifugal compressors. Both twin-screw and single-screw compressors are used in chillers. The twin-screw compressor is also called the helical rotary compressor. Figure 4.2.5 shows a cutaway of a twin-screw compressor design. There are two main rotors (screws). One is designated male (4 in the figure) and the other female (6 in the figure).The compression process is accomplished by reducing the volume of the refrigerant with the rotary motion of screws. At the low pressure side of the compressor, a void is created when the rotors begin to unmesh. Low pressure gas is drawn into the void between the rotors. As the rotors continue to turn, the gas is progressively compressed as it moves toward the discharge port. Once reaching a predetermined volume ratio, the discharge port is uncovered and the gas is discharged into the high pressure side of the system. At a rotation speed of 3600 rpm, a screw compressor has over 14,000 discharges per minute (ASHRAE, 1996).Fixed suction and discharge ports are used with screw compressors instead of valves, as used in reciprocating compressors. These set the built-in volume ratio — the ratio of the volume of fluid space in the meshing rotors at the beginning of the compression process to the volume in the rotors as the discharge port is first exposed. Associated with the built-in volume ratio is a pressure ratio that depends on the properties of the refrigerant being compressed. Screw compressors have the capability to operate at pressure ratios of above 20:1 (ASHRAE, 1996). Peak efficiency is obtained if the discharge pressure imposed by the system matchesthe pressure developed by the rotors when the discharge port is exposed. If the interlobe pressure in the screws is greater or less than discharge pressure, energy losses occur but no harm is done to the compressor.Capacity modulation is accomplished by slide valves that provide a variable suction bypass or delayed suction port closing, reducing the volume of refrigerant compressed. Continuously variable capacity control is most common, but stepped capacity control is offered in some manufacturers’ machines. Variable discharge porting is available on some machines to allow control of the built-in volume ratio during operation.Oil is used in screw compressors to seal the extensive clearance spaces between the rotors, to cool the machines, to provide lubrication, and to serve as hydraulic fluid for the capacity controls. An oil separator is required for the compressor discharge flow to remove the oil from the high-pressure refrigerant so that performance of system heat exchangers will not be penalized and the oil can be returned for reinjection in the compressor.Screw compressors can be direct driven at two-pole motor speeds (50 or 60 Hz). Their rotary motion makes these machines smooth running and quiet. Reliability is high when the machines are applied properly. Screw compressors are compact so they can be changed out readily for replacement or maintenance. The efficiency of the best screw compressors matches or exceeds that of the best reciprocating compressors at full load. High isentropic and volumetric efficiencies can be achieved with screw compressors because there are no suction or discharge valves and small clearance volumes. Screw compressors for building applications generally use either R-134a or R-22.译文冷水机组1995年,在美国,冷水机组应用在至少4%的商用建筑中。
空调专业毕业设计外文翻译--工程热力学和制冷循环
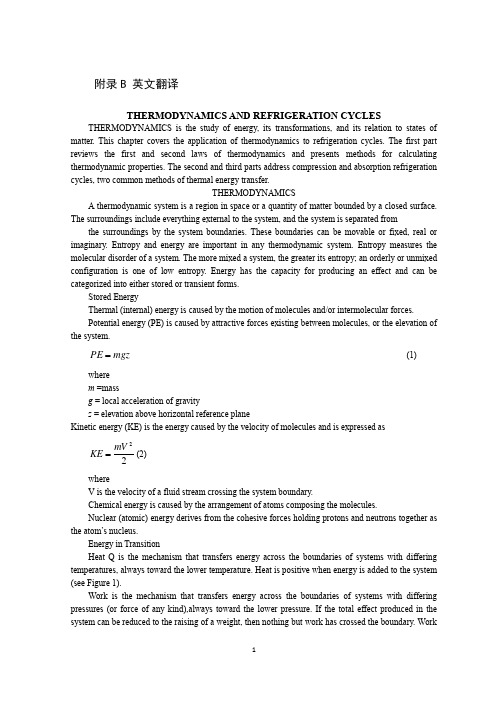
附录B 英文翻译THERMODYNAMICS AND REFRIGERATION CYCLES THERMODYNAMICS is the study of energy, its transformations, and its relation to states of matter. This chapter covers the application of thermodynamics to refrigeration cycles. The first part reviews the first and second laws of thermodynamics and presents methods for calculating thermodynamic properties. The second and third parts address compression and absorption refrigeration cycles, two common methods of thermal energy transfer.THERMODYNAMICSA thermodynamic system is a region in space or a quantity of matter bounded by a closed surface. The surroundings include everything external to the system, and the system is separated from the surroundings by the system boundaries. These boundaries can be movable or fixed, real or imaginary. Entropy and energy are important in any thermodynamic system. Entropy measures the molecular disorder of a system. The more mixed a system, the greater its entropy; an orderly or unmixed configuration is one of low entropy. Energy has the capacity for producing an effect and can be categorized into either stored or transient forms.Stored EnergyThermal (internal) energy is caused by the motion of molecules and/or intermolecular forces.Potential energy (PE) is caused by attractive forces existing between molecules, or the elevation of the system.mgzPE=(1)wherem =massg = local acceleration of gravityz = elevation above horizontal reference planeKinetic energy (KE) is the energy caused by the velocity of molecules and is expressed as22m VKE=(2)whereV is the velocity of a fluid stream crossing the system boundary.Chemical energy is caused by the arrangement of atoms composing the molecules.Nuclear (atomic) energy derives from the cohesive forces holding protons and neutrons together as the atom’s nucleus.Energy in TransitionHeat Q is the mechanism that transfers energy across the boundaries of systems with differing temperatures, always toward the lower temperature. Heat is positive when energy is added to the system (see Figure 1).Work is the mechanism that transfers energy across the boundaries of systems with differing pressures (or force of any kind),always toward the lower pressure. If the total effect produced in the system can be reduced to the raising of a weight, then nothing but work has crossed the boundary. Workis positive when energy is removed from the system (see Figure 1).Mechanical or shaft work W is the energy delivered or absorbed by a mechanism, such as a turbine, air compressor, or internal combustion engine.Flow work is energy carried into or transmitted across the system boundary because a pumping process occurs somewhere outside the system, causing fluid to enter the system. It can bemore easily understood as the work done by the fluid just outside the system on the adjacent fluid entering the system to force or push it into the system. Flow work also occurs as fluid leaves the system.Flow work =pv (3)where p is the pressure and v is the specific volume, or the volume displaced per unit mass evaluated at the inlet or exit.A property of a system is any observable characteristic of the system. The state of a system is defined by specifying the minimum set of independent properties. The most common thermodynamic properties are temperature T, pressure p, and specific volume v or density ρ. Additional thermodynamic properties include entropy, stored forms of energy, and enthalpy.Frequently, thermodynamic properties combine to form other properties. Enthalpy h is an important property that includes internal energy and flow work and is defined as≡(4) pvh+uwhere u is the internal energy per unit mass.Each property in a given state has only one definite value, and any property always has the same value for a given state, regardless of how the substance arrived at that state.A process is a change in state that can be defined as any change in the properties of a system. A process is described by specifying the initial and final equilibrium states, the path (if identifiable), and the interactions that take place across system boundaries during theprocess.A cycle is a process or a series of processes wherein the initial and final states of the system are identical. Therefore, at the conclusion of a cycle, all the properties have the same value they had at the beginning. Refrigerant circulating in a closed system undergoes acycle.A pure substance has a homogeneous and invariable chemical composition. It can exist in more than one phase, but the chemical composition is the same in all phases.If a substance is liquid at the saturation temperature and pressure,it is called a saturated liquid. If the temperature of the liquid is lower than the saturation temperature for the existing pressure, it is called either a subcooled liquid (the temperature is lower than the saturation temperature for the given pressure) or a compressed liquid (the pressure is greater than the saturation pressure for the given temperature).When a substance exists as part liquid and part vapor at the saturation temperature, its quality is defined as the ratio of the mass of vapor to the total mass. Quality has meaning only when the substance is saturated (i.e., at saturation pressure and temperature).Pressure and temperature of saturated substances are not independent properties.If a substance exists as a vapor at saturation temperature and pressure, it is called a saturated vapor. (Sometimes the term dry saturated vapor is used to emphasize that the quality is 100%.)When the vapor is at a temperature greater than the saturation temperature, it is a superheated vapor. Pressure and temperature of a superheated vapor are independent properties, because the temperature can increase while pressure remains constant. Gases such as air at room temperature and pressure are highly superheated vapors.FIRST LAW OF THERMODYNAMICSThe first law of thermodynamics is often called the law of conservation of energy. The following form of the first-law equation is valid only in the absence of a nuclear or chemical reaction.Based on the first law or the law of conservation of energy for any system, open or closed, there is an energy balance asNet amount of energy Net increase of stored=added to system energy in systemor[Energy in] – [Energy out] = [Increase of stored energy in system]Figure 1 illustrates energy flows into and out of a thermodynamic system. For the general case of multiple mass flows with uniform properties in and out of the system, the energy balance can be written=-++++-+++∑∑W Q gz V pv u m gz V pv u m out out in in )2()2(22 []system i i f f gz V pv u m gz V pv u m )2()2(22++-++ (5)where subscripts i and f refer to the initial and final states,respectively.Nearly all important engineering processes are commonly modeled as steady-flow processes. Steady flow signifies that all quantities associated with the system do not vary with time. Consequently,0)2()2(22=-+++-++∑∑W Q gz V h m gz V h m leavingstream all entering stream all (6)where h = u + pv as described in Equation (4).A second common application is the closed stationary system for which the first law equation reduces to[]system i f u u m W Q )(-=- (7)SECOND LAW OF THERMODYNAMICSThe second law of thermodynamics differentiates and quantifies processes that only proceed in a certain direction (irreversible) from those that are reversible. The second law may be described in several ways. One method uses the concept of entropy flow in an open system and the irreversibility associated with the process. The concept of irreversibility provides added insight into the operation of cycles. For example, the larger the irreversibility in a refrigeration cycle operating with a given refrigeration load between two fixed temperature levels, the larger the amount of work required tooperate the cycle. Irreversibilities include pressure drops in lines andheat exchangers, heat transfer between fluids of different temperature, and mechanical friction. Reducing total irreversibility in a cycle improves cycle performance. In the limit of no irreversibilities, a cycle attains its maximum ideal efficiency. In an open system, the second law of thermodynamics can be described in terms of entropy asdI s m s m dS e e i i T Q system +-+=δδδ(8)wheredS = total change within system in time dt during process systemδm s = entropy increase caused by mass entering (incoming)δm s = entropy decrease caused by mass leaving (exiting)δQ/T = entropy change caused by reversible heat transfer between system and surroundings at temperature TdI = entropy caused by irreversibilities (always positive)Equation (8) accounts for all entropy changes in the system. Rearranged, this equation becomes []I d dS s m s m T Q sys i i e e -+-=)(δδδ (9)In integrated form, if inlet and outlet properties, mass flow, and interactions with the surroundings do not vary with time, the general equation for the second law isI ms ms T Q S S out in revsystem i f +-+=-∑∑⎰)()(/)(δ (10)In many applications, the process can be considered to operate steadily with no change in time. The change in entropy of the system is therefore zero. The irreversibility rate, which is the rate of entropy production caused by irreversibilities in the process, can be determined by rearranging Equation (10):∑∑∑--=surrin out T Q ms ms I )()( (11) Equation (6) can be used to replace the heat transfer quantity.Note that the absolute temperature of the surroundings with which the system is exchanging heat is used in the last term. If the temper-ature of the surroundings is equal to the system temperature, heat istransferred reversibly and the last term in Equation (11) equals zero.Equation (11) is commonly applied to a system with one mass flow in, the same mass flow out, no work, and negligible kinetic or potential energy flows. Combining Equations (6) and (11) yields []surr inout in out T h h s s m I ---=)( (12)In a cycle, the reduction of work produced by a power cycle (or the increase in work required by a refrigeration cycle) equals the absolute ambient temperature multiplied by the sum of irreversibilities in all processes in the cycle. Thus, the difference in reversible and actual work for any refrigeration cycle, theoretical or real, operating under the same conditions, becomes∑+=I T W W reversible actual 0 (13)THERMODYNAMIC ANAL YSIS OFREFRIGERATION CYCLESRefrigeration cycles transfer thermal energy from a region of low temperature T to one of higher temperature. Usually the higher-T R temperature heat sink is the ambient air or cooling water, at temperature T 0, the temperature of the surroundings.The first and second laws of thermodynamics can be applied to individual components to determine mass and energy balances and the irreversibility of the components. This procedure is illustrated in later sections in this chapter.Performance of a refrigeration cycle is usually described by a coefficient of performance (COP), defined as the benefit of the cycle (amount of heat removed) divided by the required energy input to operate the cycle:Useful refrigerating effectCOP ≡Useful refrigeration effect/Net energy supplied from external sources (14)Net energy supplied from external sources For a mechanical vapor compression system, the net energy supplied is usually in the form of work, mechanical or electrical, and may include work to the compressor and fans or pumps. Thus,net evapW Q COP = (15)In an absorption refrigeration cycle, the net energy supplied is usually in the form of heat into the generator and work into the pumps and fans, ornet gen evapW Q Q COP += (16)In many cases, work supplied to an absorption system is very small compared to the amount of heat supplied to the generator, so the work term is often neglected.Applying the second law to an entire refrigeration cycle shows that a completely reversible cycle operating under the same conditions has the maximum possible COP. Departure of the actual cycle from an ideal reversible cycle is given by the refrigerating efficiency:tev R COP COP)(=η (17)The Carnot cycle usually serves as the ideal reversible refrigeration cycle. For multistage cycles, each stage is described by a reversible cycle.工程热力学和制冷循环工程热力学是研究能量及其转换和能量与物质状态之间的关系。
毕业设计蒸发式冷凝器

摘要本课程设计是关于蒸发式冷凝器的设计,针对蒸发式冷凝器的换热过程同时存在显热和潜热交换,计算过程比较复杂且方法较多的情况,采用一种简单的蒸发式冷凝器的设计计算方法,通过基本参数确定、盘管设计、水系统设计和风系统设计,进行系统设计计算,得出换热量、传热面积、淋水量、水泵功率和风机功率等设计参数,该方法适用于常规蒸发式冷凝器的设计计算。
关键词:蒸发式冷凝器;盘管;水系统;风系统。
AbstractThe evaporative condenser is designed. For the heat transfer process of evaporative condenser with latent heat exchange and sensible heat exchange, the calculation method is complex. It has a lot of method for evaporative condenser and a simple practical design calculation method of evaporative condenser is used for the design and calculations of the conventional evaporative condenser.Through the calculation of basic parameters, coil design, water system design and air system design, system design calculations were completed. The quantity of heat transfer, the area of heat transfer, the quantity of spray water, pump power and fan power were calculated. This method is applicable to the conventional design and calculation of the evaporative condenser.Keywords :evaporative condenser; coil ; water system ; air system目录绪论 (1)第1章冷凝器的种类 (2)1.1水冷式冷凝器 (2)1.1.1立式壳管式冷凝器 (2)1.1.2卧式壳管式冷凝器 (3)1.1.3套管式冷凝器 (3)1.2空气冷却式冷凝器 (4)1.3淋水式式冷凝器 ................. 错误!未定义书签。
毕业设计_BJS1200浮头式冷凝器设计
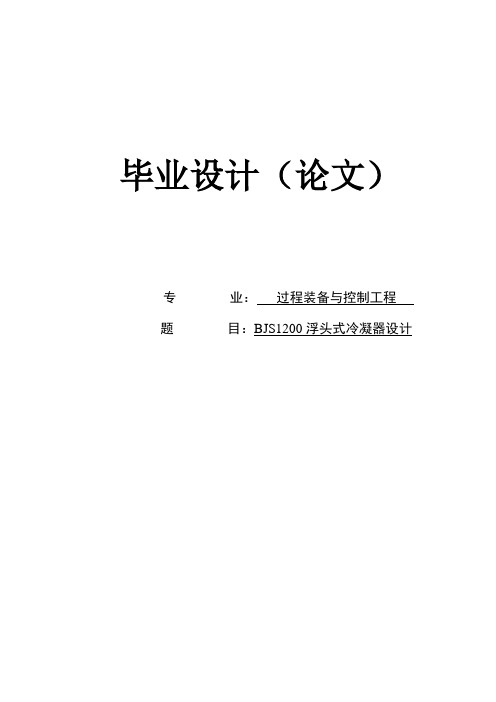
2、本课题研究的主要内容: (1)完成冷凝器(管程设计压力为 2.5MPa,壳程设计压力为 1.0MPa,壳程介质 R113 即三氯三氟乙烷进出口温度 65ºC/40ºC,管程介质自来水进出口温度 18ºC/34ºC,公称 换热面积 610m2)整体结构及零部件设计计算。 (2)进行冷凝器的热力过程分析,完成换热器受压元件的强度计算,完成传热面 积及换热量的计算。 3、提交的成果: (1)毕业设计(论文)正文; (2)A1 图纸 1 张,A2 图纸 1 张,A3 图纸 1 张; (3)至少一篇引用的外文文献及其译文; (4)附不少于 10 篇主要参考文献的题录及摘要。
指导教师(签字) 教研室主任(签字) 批准日期 接受任务书日期
完成日期 接受任务书学生(签字)
BJS1200 浮头式冷凝器设计
摘要
换热器是国民经济和工业生产领域中应用十分广泛的热量交换设备。 随着现 代新 工艺、新技术、新材料的不断开发和能源问题的日趋严重,世界各国已普遍 把石油化 工深度加工和能源综合利用摆到十分重要的位置。换热器因而面临着新 的挑战。换热 器的性能对产品质量、能量利用率以及系统运行的经济性和可靠性 起着重要的作用, 有时甚至是决定性的作用。目前在发达的工业国家热回收率已 达 96%。换热设备在现 代装置中约占设备总重的 30%左右,其中管壳式换热器仍然占绝对的优势,约 70%。其 余 30%为各类高效紧凑式换热器、新型热管热泵和蓄热器等设备,其中板式、螺旋板式、 板翅式以及各类高效传热元件的发展十分迅速。 在继续提高设备热效率的同时,促进 换热设备的结构紧凑性,产品系列化、标准化和专业化,并朝大型化的方向发展。本设 计旨在本课题研究的主要内容: (1)完成冷凝器整体结构及零部件设计计算。 (2)进行传热面积及 换热量的计算。
- 1、下载文档前请自行甄别文档内容的完整性,平台不提供额外的编辑、内容补充、找答案等附加服务。
- 2、"仅部分预览"的文档,不可在线预览部分如存在完整性等问题,可反馈申请退款(可完整预览的文档不适用该条件!)。
- 3、如文档侵犯您的权益,请联系客服反馈,我们会尽快为您处理(人工客服工作时间:9:00-18:30)。
氨制冷系统的节能设计,改造和蒸发式冷凝器的控制阿卜杜勒穆罕默德和凯利,工业评估中心,代顿大学摘要氨制冷系统通常提供了许多节能商机,因为他们的大动力消耗,运行时间长的和动态的操作。
氨制冷系统的能源使用高度依赖于冷凝头的压力,而这是一个函数的蒸发式冷凝器容量和控制功能。
本文研究系统能源利用中聚光能力和冷凝器的控制之间的关系。
它首先开发方法来确定冷凝器的性能,然后以仿真模型模拟压缩机和冷凝器风扇的年度能源利用。
,它使用工程基本面和经验两个数据,准确地捕捉压缩机,冷凝器和环境湿球温度之间的协同效应。
节约能源是三种情况:安装在冷凝器风机变频驱动器,采用湿球控制方法战略和提高聚光性能。
以说明气候的影响,这些模拟是两个不同的ASHRAE气候区,迈阿密,佛罗里达州和执行明尼阿波利斯,明尼苏达州,这是炎热和寒冷的气候分别。
结果表明,提高表现不佳的冷凝器的性能是最经济有效的节能测量。
但是节约能源从冷凝器安装变频驱动器球迷和利用湿球的方法策略取决于环境气候条件,与位置无关。
接下来,内部收益率的计算方法来安装额外的聚光能力超越在为相同的两个ASHRAE气候区新建筑应用的标准做法。
结果表明,安装两次基线聚光能力,内部收益率超过20 %。
综上所述,本文提出的设计,改造的综合方法在氨制冷系统蒸发式冷凝器的控制权。
节约能源衍生通过使用这种方法可以显著提高氨的能量效率制冷系统。
介绍约7.5 %的总生产能耗用于食品加工行业,其中约21%的能量是电能(二零零六年环评)。
在这些设备中,氨制冷系统是最大的能源消耗部分。
制冷与冷却工艺所用电量是食品加工行业(二零零六年EIA)的用电量的27%。
制冷系统使用的能量是高度依赖于冷凝压力,而这又是冷凝器容量和控制性能。
因此,提高聚光能力和控制可导致显著的节能效果。
本文首先确定使用的数据从实际的聚光性能制冷控制系统。
然后是开发仿真模型来计算每年的能源使用所研究的压缩机和冷凝器风扇。
该仿真模型,用来计算节能三个节能措施(ECMS):在冷凝器风扇安装变频器,采用湿球的方法策略,提高聚光性能。
以说明气候的影响,这些仿真用于执行迈阿密,佛罗里达州和明尼苏达州明尼阿波利斯,这是炎热和寒冷的气候分别。
文章最后决定回报的安装额外的容量超出标准规范的内部收益率在新的建筑应用。
系统说明分析系统是一个两阶段的氨制冷系统具有两个低压侧压缩机和两个高级压缩机。
所有的压缩机是螺杆式与滑阀控制和热虹吸油冷却。
一种蒸发式冷凝器以恒定的速度从系统散发热量。
对于本文的其余部分,术语系统将参考冷凝器风扇和压缩机。
从冷凝器泵的能源使用小,并且不评价了本文。
关键系统参数,包括电动机电流,氨的压力和温度从制冷控制系统获得。
氨性数据的计算使用参考流体热力学和输运性质数据(NIST ,2010)也被称为REFPROP 。
图中显示了制冷系统的替补的示意图。
图1。
电路图制冷系统的pH值图上计算排热到冷凝器冷凝压力是决定系统能源利用的一个关键变量。
为了准确地计算冷凝压力,冷凝器性能必须确定。
在第一步骤中确定冷凝器性能是计算从压缩机排出到总热量冷凝器。
在系统中的能量平衡显示了总的热拒绝了冷凝器是由低和高级压缩机加两个设置在制冷(QREF0)低和高级压缩机两者的压缩或轴功率(WS)的热量。
QCond.actual = ΣQrefLS +ΣQrefHS +ΣWSLS +ΣWsHS (1)所有的热拒绝从低温压缩机减去热虹吸拒绝的低级压缩机油冷却(TSOC ,LS)将被转移到高压侧制度。
因此,由高温压缩机提供(TRprovided ,HS)的制冷是:ΣTRprovided ,HS = ΣQrefLS +ΣQrefHS +ΣWLS - ΣTSOC ,LS ( 2 )冷凝器必须从高级的制冷和轴功率该热压缩机,加上从低级侧压缩机TSOC 。
因此,散热到冷凝器由三个主成分:QCond.actual = ΣTRprovided ,HS + ΣWsHS + ΣTSOC ,LS (3 )制冷高级阶段压缩机(TRprovided ,HS)提供制造商提供的额定制冷量的或额定吨(TR )压缩机的吸入范围和冷凝温度。
此数据可以被嵌入到一个二阶与交互项多项式方程(Manske 2000),以确定额定在给定的吸气能力和冷凝温度为:TR = C0 + C1 •Tcond + C2 •TSUC + C11 •Tcond ²+ C22 •TSUC ²+ C12 •Tcond •TSUC (4 )压缩机的百分制冷容量的滑阀百分比的函数打开的位置,但它们不相同。
在这种情况下,百分之制冷容量为函数滑阀位置的示于图2。
该数据被从制冷得到控制系统。
该曲线是相似的一个报告Stoecker (1998)。
图2。
部分容量与分数公开赛滑阀位置吸气和冷凝温度所使用的各自的压力测定和氨的属性数据。
压缩机的实际制冷容量可以使用等式4和来自控制系统的百分之制冷容量,计算如下:QREF = Qrrated •%容量(5 )压缩热由高温级压缩机(WsHS)生产来自控制系统的数据而获得的每个压缩机的电机电流。
至相关电机电流轴功率(WS),电机电流和输入之间的关系权力必须得到发展。
这种关系中,可以从点测量开发电机电流和输入功率在整个压缩机的工作范围。
通过使用的压缩机(ὴm )的两个铭牌效率和f (A ),轴功率或等价每个压缩机的压缩热量可以计算为:WsHS = F(A )* ὴm (6 )热虹吸油冷却(TSOC)考虑了两阶段的低温循环在图1中表示的氨制冷系统。
在状态1LS ,氨进入压缩机作为饱和蒸汽和离开压缩机的过热蒸汽在状态2LS 。
路径1LS - 2LS表示压缩热。
理想情况下,热量被排出到下一阶段将在点2LS和3LS的焓差。
然而,一些热量损失到压缩机油,并在压缩机的出口处的制冷剂的实际温度是在点2aLS而不是2LS 。
在点2aLS的温度和压力,从控制已知系统数据,因此焓点2aLS可以使用属性数据来确定氨的低级压缩机压缩的高温,WSLS ,也可以使用计算式(6)。
通过对压缩机施加能量平衡放电转移到热在热虹吸油冷却系统可以计算如下:TSOC ,LS = WSLS - mref.LS •(h2a.LS - h1.LS )(7 )制冷剂通过低压侧的压缩机质量流量可以计算如下:MREF -LS = Qref.LS / (h1.LS - h4.LS )(8)通常,制造商报告的体积流量的空气速率,标称容量,并且热抑制因子(HRF )。
体积流量是用于使用计算的质量流率空气的密度在标准条件。
该HRF ,这既是外部空气湿球温度计的功能温度(TWB)和饱和冷凝温度(Tcond ),用于确定在额定容量冷凝器对于一个给定TWB和Tcond为(Manske ,Reindl和2001年克莱因):额定电容容量=标称容量/ HRF ( TWB ,Tcond )( 10 )等式9b和10可以适用于制造商的规格为蒸发冷凝器,以确定对于一个给定的湿球Tcond和效力之间的关系范围。
有效性被发现是线性相关的Tcond为:effM = E0 - E1 · Tcond ( 11 )由于蒸发式冷凝器的运行期间的实际容量已计算的,实际效果可以适合于在等式11的形式的线。
测量效力与从所研究的系统Tcond数据被绘制时,无论是蒸发式冷凝器,风机和水泵是在图3满负荷生产。
额定制造商从式(11)效果也绘制在同一张图来比较的有效性上一个新的蒸发式冷凝器,以其中一个已经服役了几年。
图3表示该蒸发式冷凝器性能已劣化随着时间的推移。
实际容量比制造商的额定容量少约40%。
此信息可以被用作用于模拟程序的校准参数。
例如,在图3中,冷凝器容量为一个新的冷凝器将约为1.69倍,目前的实际能力。
图3。
实际和制造商有效性的蒸发式冷凝器模拟年能源消耗每年的能量使用的制冷系统的是压缩机和冷凝器的总和风机能耗。
冷凝压力是必须正确地计算一个关键的变量正确模拟压缩机和冷凝器风扇的能源使用。
以下步骤概述一方法计算压缩机功率,冷凝压力和冷凝器风扇电源。
计算压缩机输入功率一个给定的压缩机在一定范围抽吸的额定轴功率( bhprated )和冷凝温度可以从制造商处获得。
此数据可以被嵌入到一个二阶多项式方程的交互项来确定额定满载轴功率在给定的吸气和冷凝温度( Manske 2000),如:bhprated = P0 + P1 • Tcond + P2 • TSUC + P11 • Tcond ² + P22 • TSUC ² + P12 • Tcond • TSUC ( 12 )在该制冷系统中的压缩机,像许多制冷系统中,在操作碱/修剪方式,表示过去压缩机接通的每个阶段是修剪压缩机。
式(4),它类似于公式12中,示出的满负荷容量压缩机吸入的函数和冷凝温度下,该压缩机运行。
知道制冷负荷(参考负载)和碱的量被操作(Σ TRBase ),则该部分的容量修剪压缩机的压缩机定阶段( FCTrim )可以计算如下:FCTrim = (参考负载 - Σ TRBase ) / TRTrim ( 13 )如果基压缩机的容量大于或等于该致冷负荷,然后由基座压缩机提供的制冷为零。
通常情况下,压缩机的部分负荷功率变化的基础上部分的容量和压力比。
最好是确定采用现场每台压缩机的部分负荷电源测量。
上述压缩机在这种分析中,一个典型的部分负荷的部分负荷功率性能曲线是用来(1998 Stoecker )。
压缩机的部分功率是下面的表格:FP = A0 + A1 • FC + A2 • FC2 + A3 • FC3 + A4 •镨( 14 )要修剪压缩机,制动马力在部分负荷可以建模为:bhpact = bhprated ,修剪• FP ( 15 )使用电机的额定效率,每台压缩机的计算轴功率,总投入所有压缩机(ΣPCompressors )的功率,单位为千瓦,可以计算为:Σ PCompressors = Σ bhpact / ὴm • 0.746 (千瓦/马力)( 16 )计算的冷凝压力的蒸发冷凝器随着冷凝温度的容量的增加和环境湿球温度下降。
此外,电力的消耗压缩机的增加而增加,冷凝温度和制冷负荷。
能源利用两个冷凝器和压缩机的变化与冷凝温度显著的;准确地确定实际操作的冷凝温度是必不可少的量化能源利用系统。
图5示出了冷凝器的冷却能力高和低的湿球温度和从压缩机的高和低制冷负荷在一定范围内的散热冷凝温度。
矩形显示出所在的聚光能力和压缩机散热是相等的。
请注意,对于低的湿球温度和低的制冷负荷,冷凝器有足够的能力来拒绝压缩机的热量还要低冷凝压力。
然而,在实践中大多数制冷系统不能在冷凝压力下操作90 psig的或更少由于系统的限制。
因此,在冷凝压力设定点保持通过控制冷凝器风机和泵的最低冷凝压力。