生物陶瓷英文文献
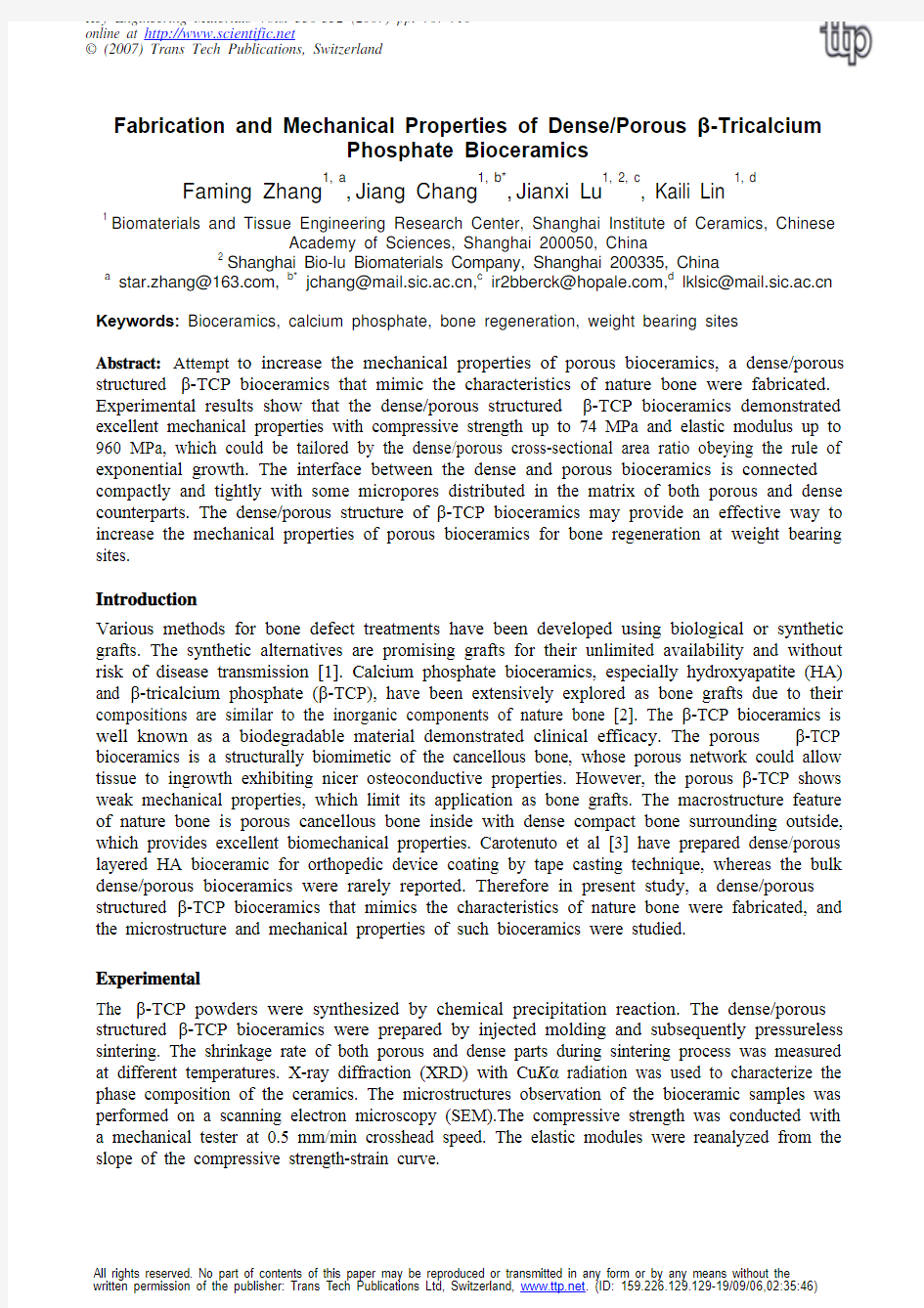
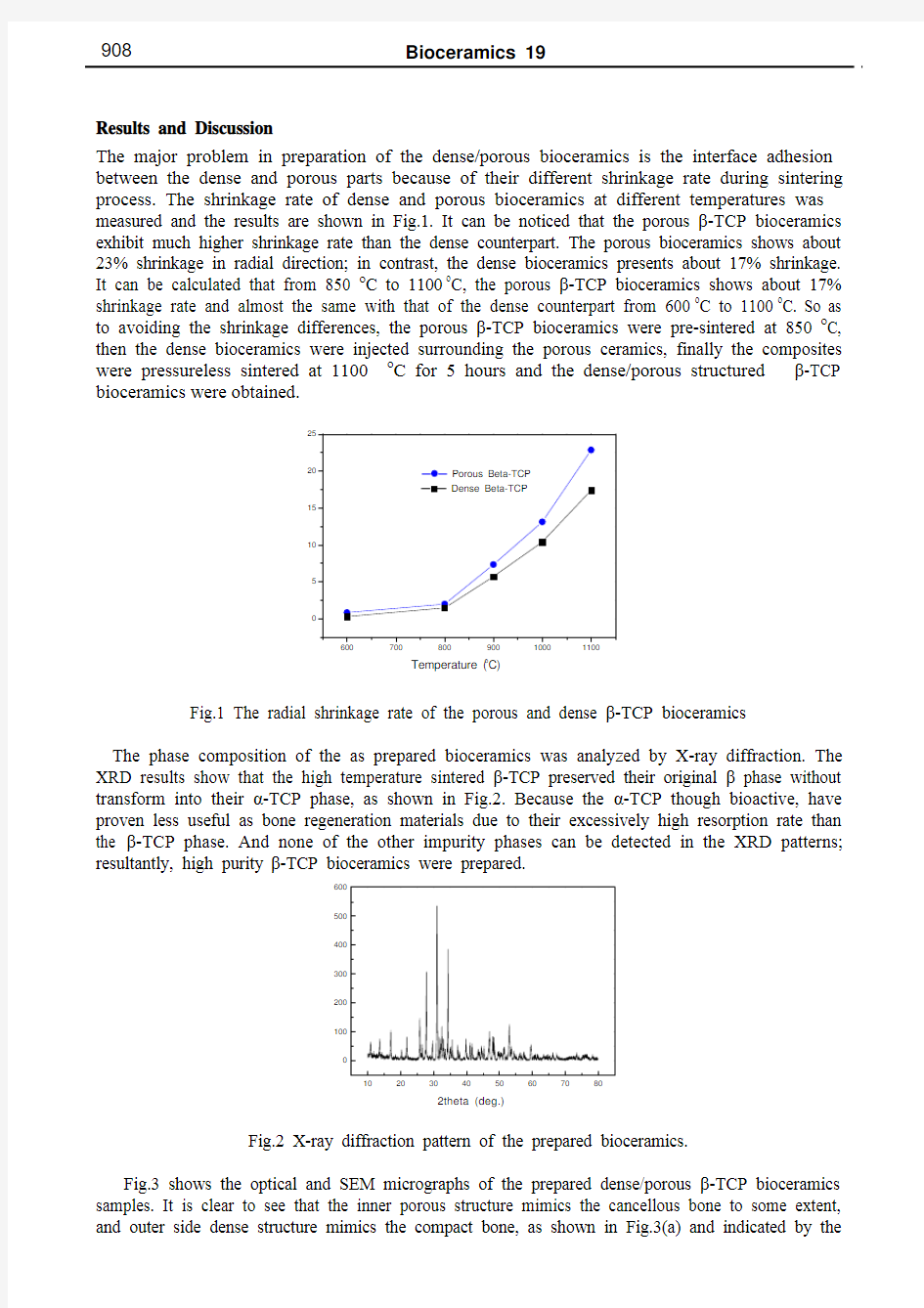
Fabrication and Mechanical Properties of Dense/Porous β-Tricalcium
Phosphate Bioceramics
Faming Zhang
1, a , Jiang Chang 1, b*, Jianxi Lu 1, 2, c , Kaili Lin 1, d 1 Biomaterials and Tissue Engineering Research Center, Shanghai Institute of Ceramics, Chinese
Academy of Sciences, Shanghai 200050, China 2 Shanghai Bio-lu Biomaterials Company, Shanghai 200335, China a star.zhang@https://www.360docs.net/doc/6f11265183.html,, b* jchang@https://www.360docs.net/doc/6f11265183.html,,c ir2bberck@https://www.360docs.net/doc/6f11265183.html,,d lklsic@https://www.360docs.net/doc/6f11265183.html,
Keywords: Bioceramics, calcium phosphate, bone regeneration, weight bearing sites
Abstract: Attempt t o increase the mechanical properties of porous bioceramics, a dense/porous structured β-TCP bioceramics that mimic the characteristics of nature bone were fabricated. Experimental results show that the dense/porous structured β-TCP bioceramics demonstrated excellent mechanical properties with compressive strength up to 74 MPa and elastic modulus up to 960 MPa, which could be tailored by the dense/porous cross-sectional area ratio obeying the rule of exponential growth. The interface between the dense and porous bioceramics is connected compactly and tightly with some micropores distributed in the matrix of both porous and dense counterparts. The dense/porous structure of β-TCP bioceramics may provide an effective way to increase the mechanical properties of porous bioceramics for bone regeneration at weight bearing sites.
Introduction
Various methods for bone defect treatments have been developed using biological or synthetic grafts. The synthetic alternatives are promising grafts for their unlimited availability and without risk of disease transmission [1]. Calcium phosphate bioceramics, especially hydroxyapatite (HA) and β-tricalcium phosphate (β-TCP), have been extensively explored as bone grafts due to their compositions are similar to the inorganic components of nature bone [2]. The β-TCP bioceramics is well known as a biodegradable material demonstrated clinical efficacy. The porous β-TCP bioceramics is a structurally biomimetic of the cancellous bone, whose porous network could allow tissue to ingrowth exhibiting nicer osteoconductive properties. However, the porous β-TCP shows weak mechanical properties, which limit its application as bone grafts. The macrostructure feature of nature bone is porous cancellous bone inside with dense compact bone surrounding outside, which provides excellent biomechanical properties. Carotenuto et al [3] have prepared dense/porous layered HA bioceramic for orthopedic device coating by tape casting technique, whereas the bulk dense/porous bioceramics were rarely reported. Therefore in present study, a dense/porous structured β-TCP bioceramics that mimics the characteristics of nature bone were fabricated, and the microstructure and mechanical properties of such bioceramics were studied.
Experimental
The β-TCP powders were synthesized by chemical precipitation reaction. The dense/porous structured β-TCP bioceramics were prepared by injected molding and subsequently pressureless sintering. The shrinkage rate of both porous and dense parts during sintering process was measured at different temperatures. X-ray diffraction (XRD) with Cu K α radiation was used to characterize the phase composition of the ceramics. The microstructures observation of the bioceramic samples was performed on a scanning electron microscopy (SEM).The compressive strength was conducted with a mechanical tester at 0.5 mm/min crosshead speed. The elastic modules were reanalyzed from the slope of the compressive strength-strain curve.
All rights reserved. No part of contents of this paper may be reproduced or transmitted in any form or by any means without the written permission of the publisher: Trans Tech Publications Ltd, Switzerland, https://www.360docs.net/doc/6f11265183.html, . (ID: 159.226.129.129-19/09/06,02:35:46)
Results and Discussion
The major problem in preparation of the dense/porous bioceramics is the interface adhesion between the dense and porous parts because of their different shrinkage rate during sintering process. The shrinkage rate of dense and porous bioceramics at different temperatures was measured and the results are shown in Fig.1. It can be noticed that the porous β-TCP bioceramics exhibit much higher shrinkage rate than the dense counterpart. The porous bioceramics shows about 23% shrinkage in radial direction; in contrast, the dense bioceramics presents about 17% shrinkage. It can be calculated that from 850 o C to 1100 o C, the porous β-TCP bioceramics shows about 17% shrinkage rate and almost the same with that of the dense counterpart from 600 o C to 1100 o C. So as to avoiding the shrinkage differences, the porous β-TCP bioceramics were pre-sintered at 850 o C, then the dense bioceramics were injected surrounding the porous ceramics, finally the composites were pressureless sintered at 1100 o C for 5 hours and the dense/porous structured β-TCP bioceramics were obtained.
Fig.1 The radial shrinkage rate of the porous and dense β-TCP bioceramics
The phase composition of the as prepared bioceramics was analyzed by X-ray diffraction. The XRD results show that the high temperature sintered β-TCP preserved their original β phase without transform into their α-TCP phase, as shown in Fig.2. Because the α-TCP though bioactive, have proven less useful as bone regeneration materials due to their excessively high resorption rate than the β-TCP phase. And none of the other impurity phases can be detected in the XRD patterns; resultantly, high purity β-TCP bioceramics were prepared.
Fig.2 X-ray diffraction pattern of the prepared bioceramics.
Fig.3 shows the optical and SEM micrographs of the prepared dense/porous β-TCP bioceramics samples. It is clear to see that the inner porous structure mimics the cancellous bone to some extent, and outer side dense structure mimics the compact bone, as shown in Fig.3(a) and indicated by the
S h i n k a g e (%)Temperature (o C)1020304050607080
100200300
400500600 2theta (deg.)
I n t e n s i t y (c p s )
arrows. Fig.3 (b) shows the interface of the dense/porous β-TCP bioceramic, it can be found that the interface between the dense and porous bioceramics is connected compactly and tightly. In the porous part, the macropore size is about 500 μm in diameter; the diameter of the interconnected pores is about 100 μm. Additionally, the porosity of the porous parts is about 72%, and the interconnectivity is more than 95%. The microstructure of the macroporous wall was shown in Fig.3(c); it is obvious that there are some micropores with diameter of 1 μm distributed uniformly in the porous wall. As the results, the microstructure of porous part of the bioceramics is a combination of macroporous and microporous. Contrastively, the microstructure of the dense bioceramics shows refined particle size and few micropores, as exhibited in Fig.3(d). The dense compact part is much denser than the porous cancellous part.
Fig.3 The dense/porous β-TCP bioceramic sample (a), the microstructure of dense/porous interface
(b), the macroporous wall (c) and dense compact bone (d).
The variation of the compressive strength and Elastic modulus of the bioceramics with different dense/porous cross-sectional area ratio (S dense /S porous ) was illustrated in Fig 4. It is exhibited that the compressive strength increases from 10 MPa to 74 MPa with the dense/porous ratio from 0.1 to 4.7 obeying rule of exponential growth. And the elastic modulus has been increased form 180 MPa to 960 MPa with the dense/porous ratio increment, also following exponential growth. Evidently, the value of the porous bioceramics is only about 2.0 MPa and the elastic modulus is about 20 MPa, indicated by the square in Fig.4. It has been achieved about 5 to 37 times increment in the mechanical properties by the dense/porous structure design. The mechanical properties of the dense/porous bioceramics could be tailored by the dense/porous cross-sectional area ratio.
Porous materials always have poor mechanical properties. Applications of calcium phosphates in the body have been limited by their low strength and numerous techniques have been investigated in attempts to retain their useful bioactive properties whilst providing more suitable mechanical properties for particular applications. These include the reinforcement of β-TCP using HA fiber or
bioglass additives [4, 5]; however these techniques are limited for the porous calcium phosphate Compact bone Cancellous
bone (b)(c) (d)
using in the load bearing sites’ bone regeneration. In this study, excellent mechanical properties of the porous β-TCP bioceramics have been achieved by the dense/porous structured design. The compressive strength of human femoral cancellous bone, weight bearing sites, is in the range of 25~90 MPa, so the dense/porous structured β-TCP is comparable to the strength of human femoral cancellous bone. The high interconnective porous structure of the dense/porous β-TCP bioceramics could allow the tissue ingrowths, and the dense structure could bear the load to some extent. The dense/porous structure of β-TCP bioceramics may provide a simple but effective way to increase the mechanical properties of porous bioceramics for the bone regeneration applications at weight bearing sites.
Fig.4 The variation of the compressive strength and elastic modulus of the bioceramics with
different dense/porous cross-sectional area ratio. Conclusions
The dense/porous structured β-TCP bioceramics were prepared and revealed excellent mechanical properties with compressive strength from 10 to 74 MPa and elastic modulus from 180 to 960 MPa, which is 5 to 37 times higher than that of the pure porous β-TCP and comparable to the strength of human femoral cancellous bone. The interface between the dense and porous bioceramics is connected compactly and tightly. The dense/porous structure of β-TCP bioceramics may provide a simple but effective way to increase the mechanical properties of porous bioceramics for weight bearing site’s bone regeneration.
Acknowledgement
Financial supports from the Shanghai Postdoctoral Scientific Key Program and the Science & Technology Commission of Shanghai Municipality of China (No.04DZ52043) are greatly acknowledged.
References:
[1] Niedhart C, Maus U, Redmann E, Schmidt-Rohlfing B, Niethard FU, Siebert CH: J Biomed
Mater Res Vol. 65A (2003), p.17
[2] Hench Larry L: Journal of the American Ceramic Society Vol. 81(1998), p.1705
[3] Carotenuto G: Advanced Performance Materials Vol. 5(1998), p.171
[4] Hassna R. R. Ramay, Zhang M.: Biomaterials Vol. 25(2004), p.5171
[5] Ashizuka M, Nakatsu M, Ishida E: Journal of the Ceramic Society of Japan, v 98(1990), p.204. 010203040506070800123450
200400600800
10001200
E l a s
t i c M o d u l u s (M P a ) C o m p r e s s i v e S t r e n g h (M P a )S dense /S porous
生物英文文献.doc
Application of α-amylase and Research α-amylase to be widely distributed throughout microorganisms to higher plants. The International Enzyme classification number is EC. 3.2.1.1, acting on the starch from the starch molecules within the random cut α a 1,4 glycosidic bond to produce dextrin and reducing sugar, because the end product of carbon residues as Α configuration configuration, it is called α-amylase. Now refers to α-amylase were cut from the starch molecules within the α-1,4 glycosidic bond from the liquefaction of a class of enzymes. α-amylase is an important enzyme, a large number of used food processing, food industry, brewing, fermentation, textile industry and pharmaceutical industries, which account for the enzyme about 25% market share. Currently, both industrial production to large-scale production by fermentation α-amylase. α-amylase in industrial applications 1.1 The bread baking industry, as a preservative enzymes used in baking industry, production of high quality products have been hundreds of years old. In recent decades, malt and microbial α-amylase, α-amylase is widely used in baking industry. The enzymes used for making bread, so that these products are much larger, better colors, more soft particles. Even today, baking industry have been α-amylase from barley malt and bacterial, fungal leaf extract. Since 1955 and after 1963 in the UK GRAS level validation, fungal amylase, has served as a bread additive. Now, they are used in different areas. Modern continuous baking process, add in f lour α-amylase can not only increase the fermentation rate and reduce dough viscosity (improving product volume and texture) to increase the sugar content in the dough, improved bread texture, skin color and baking quality, but also to extend the preservation time for baked goods. In the storage process, the bread particles become dry, hard, not crisp skin, resulting in deterioration of the taste of bread. These changes collectively referred to as degenerate. Each year simply because the losses caused by deterioration of bread more than 100 million U.S. dollars. A variety of traditional food additives are used to prevent deterioration and improve the texture and taste of baked goods. Recently, people started to pay attention enzyme as a preservative, preservative agent in improving the role of the dough, as amylopectin, amylase enzyme and a match can be effectively used as a preservative. However, excessive amylase causes a sticky bread too. Therefore, the recent trend is the use of temperature stability (ITS) α a amylase activity are high in starch liquefaction, but the baking process is completed before the inactivation. Despite the large number of microbes have been found to produce α-amylase, but with the temperature stability of the nature of the α-amylase only been found in several microorganisms. 1.2 starch liquefaction and saccharification of the main α-amylase starch hydrolysis product market, such as glucose and fructose. Starch is converted into high fructose corn syrup (HFCS). Because of their high sweetness, are used in the soft drink beverage industry sweeteners. The liquefaction process is used in thermal stability at high temperature α-amylase. α-amylase in starch liquefaction of
生物科学文献综述
纳米光催化颗粒对病原菌的杀灭效果研究
【文献综述】 纳米光催化颗粒对病原菌的杀灭效果研究 摘要:纳米光催化颗粒在可见光下对病原菌微生物的繁殖具有很好的杀灭效果,本文对光催化抗菌材料的现状和前景,优点和不足,损伤机理分析进行综述。 关键词:纳米光催化颗粒;病原菌;杀灭效果;损伤机理 引言 纳米光催化颗粒是具有杀灭或抑制病原微生物繁殖能力的一类光催化剂,当用可见光照射纳米颗粒时,通过一系列的作用,可产生具有强氧化能力的氧负离子(.O2-)和氢氧根负离子(.OH)。由于.O2-,.OH具有强氧化能力,可以氧化分解构成细菌微生物的主要成分的各种有机物质,干扰细菌蛋白质的合成[1],从而有效的的抑制细菌的繁殖生长,可以引发绝大多数有机物分子发生氧化还原反应,因此具有很好的消毒杀菌功能[2]。 1光催化抗菌材料的现状和前景 光催化抗菌材料是近些年来专家研究的热门领域之一,近年来,以二氧化钛为代表的光催化抗菌材料因其稳定性好、成本低、催化效率高等突出优点而备受人们的关注[3,4]。但是 ,二氧化钛光催化抗菌剂对太阳能的利用率低相对比较低 ,且对紫外线的要求比较严格,,从而无法有效的利用廉价的太阳能源,以致于对太阳能的应用受到了很大的限制 ,因此是否能够开发出能在可见光照射下而具有高效抗菌性能的新型光催化抗菌剂越来越受到人们的关心和重视。 纳米( nm )为长度单位, 1 nm相当于十亿分之一米。而光催化抗菌材料的纳米微粒的直径在1 nm ~ 100 nm之间。微小的颗粒能使纳米材料拥有量子尺寸的表面效应和量子隧道效应, 从而展现出多种其独特的性质,,所以光催化抗菌材料在滤光、催化、光吸收以及抗菌消毒等方面都有很高的科技价值以及广泛的应用前景[5]。 2光催化抗菌材料的优点和不足 因为半导体光催化剂具有良好的禁带宽度、催活性、氧化能力、无毒以及稳定性高等诸
微生物英文文献及翻译—翻译
A/O法活性污泥中氨氧化菌群落的动态与分布 摘要: 我们研究了在厌氧—好氧序批式反应器(SBR)中氨氧化菌群落(AOB)和亚硝酸盐氧化菌群落(NOB)的结构活性和分布。在研究过程中,分子生物技术和微型技术被用于识别和鉴定这些微生物。污泥微粒中的氨氧化菌群落结构大体上与初始的接种污泥中的结构不同。与颗粒形成一起,由于过程条件中生物选择的压力,AOB的多样性下降了。DGGE测序表明,亚硝化菌依然存在,这是因为它们能迅速的适应固定以对抗洗涤行为。DGGE更进一步的分析揭露了较大的微粒对更多的AOB种类在反应器中的生存有好处。在SBR反应器中有很多大小不一的微粒共存,颗粒的直径影响这AOB和NOB的分布。中小微粒(直径<0.6mm)不能限制氧在所有污泥空间的传输。大颗粒(直径>0.9mm)可以使含氧量降低从而限制NOB的生长。所有这些研究提供了未来对AOB微粒系统机制可能性研究的支持。 关键词:氨氧化菌(AOB),污泥微粒,菌落发展,微粒大小,硝化菌分布,发育多样性 ?简介 在浓度足够高的条件下,氨在水环境中对水生生物有毒,并且对富营养化有贡献。因此,废水中氨的生物降解和去除是废水处理工程的基本功能。硝化反应,将氨通过硝化转化为硝酸盐,是去除氨的一个重要途径。这是分两步组成的,由氨氧化和亚硝酸盐氧化细菌完成。好氧氨氧化一般是第一步,硝化反应的限制步骤:然而,这是废水中氨去除的本质。对16S rRNA的对比分析显示,大多数活性污泥里的氨氧化菌系统的跟?-变形菌有关联。然而,一系列的研究表明,在氨氧化菌的不同代和不同系有生理和生态区别,而且环境因素例如处理常量,溶解氧,盐度,pH,自由氨例子浓度会影响氨氧化菌的种类。因此,废水处理中氨氧化菌的生理活动和平衡对废水处理系统的设计和运行是至关重要的。由于这个原因,对氨氧化菌生态和微生物学更深一层的了解对加强处理效果是必须的。当今,有几个进阶技术在废水生物处理系统中被用作鉴别、刻画微生物种类的有价值的工具。目前,分子生物技术的应用能提供氨氧化菌群落的详细分类说明。
生物陶瓷材料的研究及应用
生物陶瓷材料的研究及应用 张波化工07-3班 120073304069 摘要介绍了生物陶瓷的定义,对羟基磷灰石生物陶瓷材料、磷酸钙生物陶瓷材料、复合生物陶瓷材料、涂层生物陶瓷材料和氧化铝生物陶瓷的特性和制备方法进行了较为深入的分析,在现代医学中的应用及发展前景。 关键词生物陶瓷,磷酸钙,复合生物陶瓷材料,涂层生物陶瓷材料,氧化铝陶瓷,生物陶瓷应用。 Bioceramic Materials Research and Application Zhangbo Chemical Engineering and Technology 073 class 120073304069 Abstract This paper introduces the definition of bio-ceramics, bio-ceramic material of hydroxyapatite, calcium phosphate bio-ceramic materials, composite bio-ceramic materials, coating materials, bio-ceramics and alumina ceramics of biological characteristics and preparation methods for a more in-depth analysis In modern medicine the application and development prospects. Key words bio-ceramics, calcium phosphate, composite bio-ceramic materials, coating materials, bio-ceramic, alumina ceramic, bio-ceramic applications. 1 引言 生物陶瓷是指用作特定的生物或生理功能的一类陶瓷材料,即直接用于人体或与人体相关的生物、医用、生物化学等的陶瓷材料。做为生物陶瓷材料,需具备如下条件:生物相容性;力学相容性;与生物组织有优异的亲和性;抗血栓;灭菌性并具有很好的 物理、化学稳定性。生物陶瓷材料可分为生物惰性陶瓷(如Al 2O 3 、ZrO 2 等)、生物活性 陶瓷(如致密羟基磷灰石、生物活性微晶玻璃等)和生物复合材料三类。生物陶瓷材料因其与人的生活密切相关,故一直倍受材料科学工作者的重视。 2 生物陶瓷材料的发展 目前世界各国相继发展了生物陶瓷材料,它不仅具有不锈钢塑料所具有的特性,而且具有亲水性、能与细胞等生物组织表现出良好的亲和性。因此生物陶瓷具有广阔的发展前景。生物陶瓷的应用范围也正在逐步扩大,现可应用于人工骨、人
生物医学工程中英文对照外文翻译文献
(文档含英文原文和中文翻译) 中英文对照外文翻译文献 Biological effects of the Magnetic Stimulation on the T oad Heart Abstract-W e stimulated the exposed toad heart by a low frequency and high energy magnetic. By analyze the data of this experiment, it shows that the pulsating of the weak toad heart would make change after stimulated by magnetic. W eak heartbeat strengthened, the single peak curve would become the two peaks curve with atria wave and ventricle wave after the magnetic stimulation. But the cycling of rhythmic pulsatile curve of toad doesn't change. I. INTRODUTION All life forms have magnetism. All kinds of magnetic field would have some effects on the configuration and activities of life forms that whichever environmental magnetic, additional magnetic or inside magnetic of organism. The biologic effects are related to the characteristics and
生物科技文献综述
生物科技文献综述江西师范大学生命科学学院
生物化学文献综述 引言: 生物化学是研究生命过程中化学基础的科学。疾病的发生发展是致病因子对生命过程的干扰和破坏;药物的防治是对病理过程的干预。生物化学通过用化学的理论和方法研究生命现象、生命过程的化学基础,通过探索干预和调整疾病发生发展的途径和机理,为新药发现中提供必不可少的理论依据。 生物化学是自90年代中期以来的新兴研究领域。哈佛大学的Schreiber博士和Scripps研究所的Schultz博士分别在东西海岸引领这个领域,他们的所在地所形成的重心地位甚至在加强。从源头来讲,化学是研究分子的科学,生物化学,分子生物学,还有生物学化学都是一样的。但是由于科学家们长期以来的习惯称谓,我们通常使用生物化学指蛋白质结构和活性的研究,用分子生物学指基因表达和控制的研究,用生物学化学指分子水平上的生物现象的研究。 三、关键词 化学生物学与分子生物学;临床医学;多学科融合;科研创新;虚拟实验;多方向研究;综合性实验四、主题综述: 化学生物学使用小分子作为工具解决生物学的问题或通过干扰、调节正常过程了解蛋白质的功能。在某种意义上,使用小分子调节目标蛋白质与制药公司发展新药类似。但是,当所有公司的目标蛋白质到目前为止仅是约450种的时候,人类基因组计划为我们带来了至少几万个目标蛋白质。最终的目标是寻找特异性调节素或寻找解开所有蛋白质之谜的钥匙,但这需要更系统和整体的方法而并非传统方法。化学生物学看起来是有希望的答案。系统的化学生物学仅仅诞生于90年代中期,部份是由于基础条件到那时才刚刚完备。代表性的技术进步包括机器人工程,高通量及高灵敏度的生物筛选,信息生物学,数据采集工具,组合化学和芯片技术例如DNA芯片。化学生物学更普遍的被叫做化学遗传学,而且它正在扩展到化学基因组学。和经典遗传学相比较,小分子并不是取代或超越基因表达,而是被用于抑制或活化翻译过程。 化学生物学、计算生物学与合成生物学,在生物芯片技术、计算模型方法与基因网络设计等方面构成了现代系统生物学与系统遗传学的重要技术基础。 五、研究法方向及方法 在进行研究的过程中,分为了正向研究和逆向研究。在正向法中,目标生物学现象第一次被定义,然后引起被寻找现象的分子选择自许多被应用的分子。被选择的分8子能被附到某些蛋白质上而且抑制/活化它们,引发重要的修饰,然后与分子相连的蛋白质被检查并研究。下面是使用正向法发现和发展肌基质蛋白的例子Nat。 首先,为了获得足量得化合物以引发要得到的现象,通过组合化学的合成方法制得嘌呤文库。多种化合物可与放射性研究引起的不同变异相比较。已经分化的神经原细胞和肌肉细胞很少被增殖。因此,一旦受伤,细胞长不好,恢复很难。这项研究的最初目的是为了找到一种化合物来引起改变肌肉细胞分化,达到再生目的。 分化的肌肉组织构成交织的管状结构。几百个嘌呤类化合物被在96孔圆片上植入潜伏肌肉组织中,找到了能够分离相连接的组织的化合物。这种化合物自肌管隔断嘌呤命名为肌基质
生物陶瓷材料的分类
惰性生物陶瓷材料 生物惰性陶瓷主要是指化学性能稳定,生物相容性好的陶瓷材料。这类陶瓷材料的结构都比较稳定,分子中的键力较强,而且都具有较高的机械强度、耐磨性以及化学稳定性。主要由氧化物陶瓷、非氧化物陶瓷以及陶材组成。其中,以Al、Mg、Ti、Zr 的氧化物应用最为广泛。 早在1969 年,Talbert[2]就将不同孔隙率的颗粒状Al2O3 陶瓷作为永久性可移植骨假体,植入成年杂种狗的股骨中进行实验,发现多晶氧化铝陶瓷对包括生物环境在内的任何环境都呈现惰性及其优越的耐磨损性和高的抗压强度。使氧化铝陶瓷材料成为最早获得临床应用的生物惰性陶瓷材料。目前氧化铝陶瓷材料已经应用于人造骨、人工关节及人造齿根的制作方面。 氧化铝陶瓷植入人体后,体内软组织在其表面生成极薄的纤维组织包膜,在体内可见纤维细胞增生,界面无化学反应,多用于全臀复位修复术及股骨和髋骨部连接[3]。单晶氧化铝陶瓷的机械性能更优于多晶氧化铝,适用于负重大、耐磨要求高的部位。但是由于Al2O3 属脆性材料,冲击韧性较低,且弹性模量和人骨相差较大,可能引起骨组织的应力,从而引起骨组织的萎缩和关节松动,在使用过程中,常出现脆性破坏和骨损伤,且不能直接与骨结合。 目前,国外有关学者通过各种方法,使Al2O3 陶瓷在韧性和相容性方面取得了显著提高[4],如在陶瓷表面涂上骨亲和性高的陶瓷,特别是能和骨发生化学结合的磷灰石,已经制造出更加先进的人工关
节。通过相变或微裂等方法,使材料内部产生微裂纹,只要微裂纹的尺寸足够小,则均匀分布的微裂纹会起到应力分散的作用。也可以提高材料的韧性[5]。 近年,氧化锆陶瓷由于其优良的力学性能,尤其是其远高于氧化铝瓷的断裂韧性,使其作为增强增韧第二相材料在人体硬组织修复体方面取得了较大研究的进展。Hench[6]报道,部分稳定氧化锆陶瓷的抗弯强度可达100 MPa,断裂韧性可达15MPa·m- 1/2。 但惰性生物陶瓷在体内被纤维组织包裹或与骨组织之间形成纤维组织界面的特性影响了该材料在骨缺损修复中的应用,因为骨与材料之间存在纤维组织界面,阻碍了材料与骨的结合,也影响材料的骨传导性,长期滞留体内产生结构上的缺陷,使骨组织产生力学上的薄弱。 2 生物活性陶瓷材料 生物活性陶瓷包括表面生物活性陶瓷和生物吸收性陶瓷,又叫生物降解陶瓷。生物表面活性陶瓷通常含有羟基,还可做成多孔性,生物组织可长入并同其表面发生牢固的键合;生物吸收性陶瓷的特点是能部分吸收或者全部吸收,在生物体内能诱发新生骨的生长。生物活性陶瓷有生物活性玻璃(磷酸钙系),羟基磷灰石陶瓷,磷酸三钙陶瓷等几种。 2.1 羟基磷灰石陶瓷 羟基磷灰石(hydroxyapatite),简称HAp,化学式为Ca10(PO4)6(OH)2,属表面活性材料,由于生物体硬组织(牙齿、骨)
生物陶瓷英文文献
Fabrication and Mechanical Properties of Dense/Porous β-Tricalcium Phosphate Bioceramics Faming Zhang 1, a , Jiang Chang 1, b*, Jianxi Lu 1, 2, c , Kaili Lin 1, d 1 Biomaterials and Tissue Engineering Research Center, Shanghai Institute of Ceramics, Chinese Academy of Sciences, Shanghai 200050, China 2 Shanghai Bio-lu Biomaterials Company, Shanghai 200335, China a star.zhang@https://www.360docs.net/doc/6f11265183.html,, b* jchang@https://www.360docs.net/doc/6f11265183.html,,c ir2bberck@https://www.360docs.net/doc/6f11265183.html,,d lklsic@https://www.360docs.net/doc/6f11265183.html, Keywords: Bioceramics, calcium phosphate, bone regeneration, weight bearing sites Abstract: Attempt t o increase the mechanical properties of porous bioceramics, a dense/porous structured β-TCP bioceramics that mimic the characteristics of nature bone were fabricated. Experimental results show that the dense/porous structured β-TCP bioceramics demonstrated excellent mechanical properties with compressive strength up to 74 MPa and elastic modulus up to 960 MPa, which could be tailored by the dense/porous cross-sectional area ratio obeying the rule of exponential growth. The interface between the dense and porous bioceramics is connected compactly and tightly with some micropores distributed in the matrix of both porous and dense counterparts. The dense/porous structure of β-TCP bioceramics may provide an effective way to increase the mechanical properties of porous bioceramics for bone regeneration at weight bearing sites. Introduction Various methods for bone defect treatments have been developed using biological or synthetic grafts. The synthetic alternatives are promising grafts for their unlimited availability and without risk of disease transmission [1]. Calcium phosphate bioceramics, especially hydroxyapatite (HA) and β-tricalcium phosphate (β-TCP), have been extensively explored as bone grafts due to their compositions are similar to the inorganic components of nature bone [2]. The β-TCP bioceramics is well known as a biodegradable material demonstrated clinical efficacy. The porous β-TCP bioceramics is a structurally biomimetic of the cancellous bone, whose porous network could allow tissue to ingrowth exhibiting nicer osteoconductive properties. However, the porous β-TCP shows weak mechanical properties, which limit its application as bone grafts. The macrostructure feature of nature bone is porous cancellous bone inside with dense compact bone surrounding outside, which provides excellent biomechanical properties. Carotenuto et al [3] have prepared dense/porous layered HA bioceramic for orthopedic device coating by tape casting technique, whereas the bulk dense/porous bioceramics were rarely reported. Therefore in present study, a dense/porous structured β-TCP bioceramics that mimics the characteristics of nature bone were fabricated, and the microstructure and mechanical properties of such bioceramics were studied. Experimental The β-TCP powders were synthesized by chemical precipitation reaction. The dense/porous structured β-TCP bioceramics were prepared by injected molding and subsequently pressureless sintering. The shrinkage rate of both porous and dense parts during sintering process was measured at different temperatures. X-ray diffraction (XRD) with Cu K α radiation was used to characterize the phase composition of the ceramics. The microstructures observation of the bioceramic samples was performed on a scanning electron microscopy (SEM).The compressive strength was conducted with a mechanical tester at 0.5 mm/min crosshead speed. The elastic modules were reanalyzed from the slope of the compressive strength-strain curve. All rights reserved. No part of contents of this paper may be reproduced or transmitted in any form or by any means without the written permission of the publisher: Trans Tech Publications Ltd, Switzerland, https://www.360docs.net/doc/6f11265183.html, . (ID: 159.226.129.129-19/09/06,02:35:46)
微生物技术分子生物技术中英文资料外文翻译文献
微生物技术分子生物技术 中英文资料外文翻译文献 A/O法活性污泥中氨氧化菌群落的动态与分布 摘要: 我们研究了在厌氧—好氧序批式反应器(SBR)中氨氧化菌群落(AOB)和亚硝酸盐氧化菌群落(NOB)的结构活性和分布。在研究过程中,分子生物技术和微型技术被用于识别和鉴定这些微生物。污泥微粒中的氨氧化菌群落结构大体上与初始的接种污泥中的结构不同。与颗粒形成一起,由于过程条件中生物选择的压力,AOB的多样性下降了。DGGE测序表明,亚硝化菌依然存在,这是因为它们能迅速的适应固定以对抗洗涤行为。DGGE更进一步的分析揭露了较大的微粒对更多的AOB种类在反应器中的生存有好处。在SBR反应器中有很多大小不一的微粒共存,颗粒的直径影响这AOB和NOB的分布。中小微粒(直径<0.6mm)不能限制氧在所有污泥空间的传输。大颗粒(直径>0.9mm)可以使含氧量降低从而限制NOB的生长。所有这些研究提供了未来对AOB微粒系统机制可能性研究的支持。 关键词:氨氧化菌(AOB),污泥微粒,菌落发展,微粒大小,硝化菌分布,发育多样性 1.简介 在浓度足够高的条件下,氨在水环境中对水生生物有毒,并且对富营养化有贡献。因此,废水中氨的生物降解和去除是废水处理工程的基本功能。硝化反应,将氨通过硝化转化为硝酸盐,是去除氨的一个重要途径。这是分两步组成的,由氨氧化和亚硝酸盐氧化细菌完成。好氧氨氧化一般是第一步,硝化反应的限制步骤:然而,这是废水中氨去除的本质。对16S rRNA的对比分析显示,大多数活
性污泥里的氨氧化菌系统的跟?-变形菌有关联。然而,一系列的研究表明,在氨氧化菌的不同代和不同系有生理和生态区别,而且环境因素例如处理常量,溶解氧,盐度,pH,自由氨例子浓度会影响氨氧化菌的种类。因此,废水处理中氨氧化菌的生理活动和平衡对废水处理系统的设计和运行是至关重要的。由于这个原因,对氨氧化菌生态和微生物学更深一层的了解对加强处理效果是必须的。当今,有几个进阶技术在废水生物处理系统中被用作鉴别、刻画微生物种类的有价值的工具。目前,分子生物技术的应用能提供氨氧化菌群落的详细分类说明。 如今,主要由于其细胞固定策略,好氧污泥颗粒处理已经成为传统废水处理的替代工艺。颗粒有更加彻底的紧密结构和快速适应速率。因此,颗粒污泥系统比传统活性污泥法有更高的混合悬浮固体浓度浓度(MLSS)和更长的污泥龄(SRT)。更长的污泥龄能提供足够长的时间让时代时间长的微生物生长(例如氨氧化菌)。有些研究表示,硝化颗粒可以在富铵离子废水中培养出来,并且颗粒的直径很小。其他研究报告说,大直径颗粒已经在序批式反应器(SBR)中人工合成的有机废水里培育出来了。污泥颗粒里的大量不同微生物共存,并去除COD和氮磷。然而,对于直径大于0.6mm的大颗粒来说,由于氧传递被限制不能到达颗粒核心,外部好氧壳和内部厌氧地带共存。这些特性表明,大颗粒污泥内部环境不适合氨氧化菌的生长。有些研究表明,颗粒大小和密度导致了氨氧化菌、亚硝酸氧化菌和反硝化菌的分布和优势种群。虽然不少研究力求评估废水处理系统中氨氧化菌的生态生理,但是至今仍然被污泥颗粒化过程的水力学、分布、氨氧化菌群落的数量化限制着。 2.原理和方法 2.1反应器设置和操作 污泥颗粒被接种在有效体积为4L的实验室规模的SBR里。反应器有效直径和高度分别为10cm和51cm。水力停留时间设为8h。来自全尺寸污泥处理设置(中国天津污水处理厂)的活性污泥被作为反应器的种污泥,其MLSS初始浓度为3876mg/L。反应器操作6小时为一循环,由2分钟的进水时间,90分钟厌氧混合,240反正抛弃阶段和5分钟出水阶段组成。在20天80个SBR循环后,污泥沉降时间逐渐从10分钟降到5分钟,并且只有沉降速度大禹4.5m/h的颗粒才能在反应器中停留。入流中的主要化合物包括NaAc(450mg/L),NH4Cl(100mg/L),
生物陶瓷在骨组织中的应用与展望
生物陶瓷在骨组织中的应用与展望 (南京工业大学材料学院材物0801 刘发付冯岩张文斌仇月东左辉) 摘要:综述了生物陶瓷材料在骨科中的应用研究进展.人体骨骼中有机物占干质量约33%,而无机物占干骨质量的65%~75%,其中又有质量分数95%为固体钙及磷。在此基础上,人们研究了生物陶瓷,并在骨组织方面得到了应用,且取得了一定成就,但是并没有达到真正的相容活性陶瓷.展望了生物陶瓷在骨科中的前景. 关键词: 无机材料;生物陶瓷;综述;骨科;活陶瓷 生物医用材料的研究与开发,对人类有重要意义。人们对健康和长寿的追求将推动生物医用材料的发展。它在世界经济中的地位己经可以和信息及汽车产业相比,目前以每年超过20%的速度增长,其材料及制品市场会达到药品市场规模,将成为21世纪的支柱产业。2004 年,美国在卫生与人类服务部(Department ofHealth & Human Services)建立了医学创新特别组[1],其任务是促进新医学技术的创新及发展。中国的骨质疏松病人近亿,美国每年约有15 万例的髋 关节及约30 万例膝关节的置换,但人工关节多采用金属或陶瓷构成,会引起炎症,甚至几年后还需再通过手术进行矫正,给病人带来痛苦。人们希望有耐能参与生命组织活动的人工骨。 1 生物陶瓷材料在骨科中的应用研究进展 目前, 对羟基磷灰石材料的研究重点是克服羟基磷灰石生物陶瓷材料的脆性和在生理环境中的疲劳破坏, 使其能用作承力的骨替换材料, 因此研究人员正试图利用纳米的微尺寸效应来研究纳米羟基磷灰石对提高材料强韧性以及对生物相容性的影响。有资料报道[13-15],羟基磷灰石材料近十年来受到临床重视, 它的种植体模仿了骨基质的结构, 具有骨诱导性, 能为新生骨组织的长入提供支架和通道, 孔径、孔率和孔内部的连通行是骨长入方式和数量的决定性因素。研究表明[16], 当种植体内部连通气孔的孔径为5~40 μm 时,允许纤维组织长入; 当孔径为 40~100 μm 时, 允许非矿化的骨样组织长入; 当孔径在150~200 μm 时, 能为骨组织的长入提供理想的场所; 当孔径超过200 μm时, 是骨传导的基本要求; 当孔径在200~400 μm 时,最有利于新骨生长。 陶瓷基复合材料是以陶瓷、玻璃或玻璃陶瓷基体, 通过不同方式引入颗粒、晶须或纤维等增强体而获得的一类复合材料。目前生物陶瓷基复合材料尚未达到大规模临床应用阶段, 其研究还主要集中于生物材料的活性和骨结合性能研究以及材料增强研究等。
生物质能源外文翻译外文文献英文文献中国的生物质能源
China’s Biomass Energy China leads the world in its energy reservation and is the second largest energy producer and consumer in the world. It is estimated that China has 4000 billion tons of potential primary energy reservation. However, per capita energy resource quantity and consuming quantity is far smaller than the world average level. The main characteristics of China’s energy exploration and utilization are as follows. 1. Coal is the primary energy; exploration and utilization of renewable resources is supplementary. China’s explored reserves of coal resource accounts for over 90% of the primary energy total, such as coal, oil, natural gas, water energy and nuclear energy. Coal is dominant id China’s energy production and consumption. on. 2. Energy consumption volume is increasing while energy utilization efficiency is comparatively low. As the economic scale expands, China’s energy consumption is on the constant increase. Under the influences of capital, technology, energy price, etc, China’s energy utilization efficiency is far lower than that of the developed countries. Energy comprehensive utilization efficiency is 32% and the overall energy systematic efficiency 9.3%. These numbers are only half of the developed country level. 3. With the sustained increase of energy consumption, the coal-dominant energy structure has caused urban air pollution while with the excessive consumption of bio-mass energy giving rise to ecological destruction, the pressure on ecological environment is more and more severe. According to the World Bank, the economic loss caused by air and water pollution in China, approximately accounts for 3%--8% of GNP. 4. The energy structure is getting more and more pluralized. By the middle of the 1990’s, energy self-sufficiency rate was 98%, while at present China is the second largest crude oil importer only after USA. The rapid development of China’s economy is based on the sufficient supply of energy, however, the higher-than-normal oil price on the international market together wit h China’s ever-increasing energy demand will be a serious subject faced with
生物与健康---文献综述
生物与健康 学院:生命科学学院班级:药学1班姓名:吴瑞鼎 摘要:生物与人类关系密切,在人类生活中占有的非常重要的地位,在我们生活的每一天都与之相接触,它既能引起人类疾病也能造福于人类。目前,生物大致分类为动物,植物,微生物。在现今科学如此发展的时代,我们要正确认识和使用生物,让生物给我们带来健康。 关键词:疾病、微生物、抗生素、浙八味、银杏、红豆杉、纳豆、杂交瘤技术、克隆器官、心理归宿 ——————————微生物 我们今天已经进入了21世纪,虽然我们能够制服引起一般传染病的微生物,但是,艾滋病和新出现的疾病依然严重威胁着人类,还有些一度被控制的传染病又开始死灰复燃。人类每天都和这些恐怖的传染病,如狂犬病,禽流感作斗争,在精神和资源方面深受侵害,人力和物力财力不断投入。世界卫生组织的资料,全世界每年因疾病死亡的人中,有1/3是由传染病造成的。 鼠疫,艾滋病(AIDS),癌症,肺结核、疟疾、霍乱“卷土重来”,埃博拉病毒、疯牛病、SARS、禽流感等都是由一些极少部分的微生物所致,而且这些病毒极易突变,难以研制疫苗。拿鼠疫来说吧,1347年的一场由鼠疫杆菌引起的瘟疫几乎摧毁了整个欧洲,有1/3 的人(约2500万人)死于这场灾难,在此后的80年间,这种疾病一再肆虐,实际上消灭了大约75%的欧洲人口,一些历史学家认为这场灾难甚至改变了欧洲文化。我国在解放前也曾多次流行鼠疫,死亡率极高。而且还证实,这些病毒还在变异,这就更加增加了对这些疾病研究的困难。全世界虽然已经花费了无法统计的经费,但有些疾病的危害力不减小,甚至艾滋病的患者和感染者还在每年成倍增长。人类和病原微生物的斗争也许是一场永远看不