19.2% efficient c-Si solar cells using ion implantation采用离子注入技术的晶硅太阳电池效率达到19.2%
二茂铁染料在敏化太阳能电池的研究进展
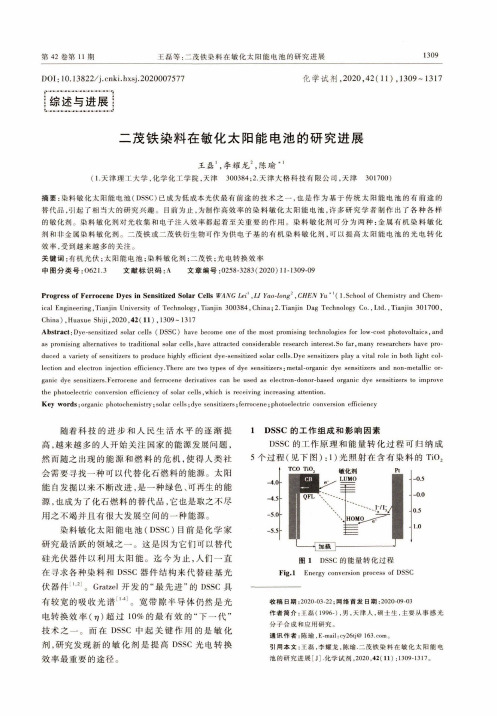
DOI: 10.13822/ki.hxsj.2020007577j综述与进展化学试剂,2020,42(11) ,1309〜1317二茂铁染料在敏化太阳能电池的研究进展王磊、李耀龙2,陈瑜“(1.天津理工大学,化学化工学院,天津300384;2.天津大格科技有限公司,天津301700)摘要:染料敏化太阳能电池(DSSC)已成为低成本光伏最有前途的技术之一,也是作为基于传统太阳能电池的有前途的替代品,引起了相当大的研究兴趣。
目前为止,为制作高效率的染料敏化太阳能电池,许多研究学者制作出了各种各样的敏化剂。
染料敏化剂对光收集和电子注入效率都起着至关重要的作用。
染料敏化剂可分为两种:金属有机染料敏化剂和非金属染料敏化剂。
二茂铁或二茂铁衍生物可作为供电子基的有机染料敏化剂,可以提高太阳能电池的光电转化效率,受到越来越多的关注。
关键词:有机光伏;太阳能电池;染料敏化剂;二茂铁;光电转换效率中图分类号:062丨.3 文献标识码:A文章编号:0258-3283( 2020) 11 -丨309-09Progress of Ferrocene Dyes in Sensitized Solar Cells WANG Lei' ,L I Yao-long1 ?CHEN Y u*\ 1.School of Chemistry a n d C h e mical Engineering,Tianjin University of Te c h n o l o g y,Tianjin 300384,C h i n a;2.Tianjin D a g Technology C o.,Ltd.,Tianjin 301700, C h i n a) ,H u a x u e Shiji,2020,42(11) , 1309 ~ 1317Abstract:Dye-sensitized solar cells (D S S C)have b e c o m e one of the most promising technologies for low-cost photovoltaics,and as promising alternatives to traditional solar cells,have attracted considerable research interest.So f a r,m a n y researchers have prod u c e d a variety of sensitizers to produce highly efficient dye-sensitized solar cells.Dye sensitizers play a vital role in both light collection and electron injection efficiency.There are two types of dye sensitizers :metal-organic dye sensitizers and non-metallic or- ganic dye sensitizers.Ferrocene a n d ferrocene derivatives can be used as electron-donor-based organic dye sensitizers to improve the photoelectric conversion efficiency of solar cells,which is receiving increasing attention.Key w ords:organic photochemistry;solar cells;dye sensitizers;ferrocene;photoelectric conversion efficiency随着科技的进步和人民生活水平的逐渐提高,越来越多的人开始关注国家的能源发展问题,然而随之出现的能源和燃料的危机,使得人类社会需要寻找一种可以代替化石燃料的能源。
Surface passivation of high-efficiency silicon solar cells by atomic-layer-deposited Al2O3

小学下册第十四次英语第6单元期末试卷

小学下册英语第6单元期末试卷考试时间:90分钟(总分:140)A卷一、综合题(共计100题共100分)1. 选择题:What is the smallest continent?A. AsiaB. AfricaC. AustraliaD. Europe答案:C2. 选择题:What is the term for a small rocky body that orbits the sun?A. CometB. AsteroidC. MeteorD. Planet3. 听力题:I want to ________ (create) something special.4. 选择题:What is the main purpose of a compass?A. To tell timeB. To find directionC. To measure distanceD. To calculate speed答案: B5. 填空题:The ______ (蚂蚁) works hard to gather food.6. 选择题:What is the name of the famous explorer who sailed the Pacific Ocean?A. Ferdinand MagellanB. Christopher ColumbusC. Vasco da GamaD. John Cabot答案: A7. 填空题:中国的________ (historical) 文化深深植根于传统和信仰中。
8. 选择题:What is the capital city of France?A. BerlinB. LondonC. ParisD. Madrid9. 选择题:What do we call a person who plays the piano?A. PianistB. MusicianC. ArtistD. All of the above10. 选择题:What is the name of the fairy tale character who has long hair?A. MulanB. RapunzelC. ArielD. Belle11. 填空题:The _______ (青蛙) likes to jump around.12. 选择题:What do you call a collection of books?A. LibraryB. ArchiveC. AnthologyD. Gallery答案:A13. 填空题:The _____ (小狗) is barking at the mailman.14. 听力题:The Ptolemaic model placed the Earth at the _______ of the universe.I enjoy making ______ (手工艺品) from recycled materials. It’s a fun way to be creative and eco-friendly.16. 填空题:The ancient Egyptians created vast ________ (陵墓) for their pharaohs.17. 填空题:I have a toy ______ (飞机) that can fly high in the sky. It is very ______ (酷).18. 选择题:What instrument has strings and is played with a bow?A. FluteB. PianoC. ViolinD. Drum答案: C19. 填空题:We have a ______ (特别的) day planned for school.20. 填空题:The __________ (历史的分析工具) aid in research.21. 填空题:My mom loves __________ (参加志愿活动).22. 听力题:A _______ is a reaction that releases heat.23. 选择题:What is 7 x 2?A. 12B. 14C. 16D. 18答案: B24. 听力题:The _____ (telescope) helps us see stars.25. 填空题:I enjoy watching the _______ (小动物) in the park.We are learning about _______ (动物) in school.27. 选择题:What is the name of the ocean between Africa and Australia?A. Atlantic OceanB. Indian OceanC. Arctic OceanD. Southern Ocean答案: B28. 选择题:What do you call a drink made from fermented grapes?A. BeerB. WhiskeyC. WineD. Cider答案:C29. 填空题:The ________ was a famous artist known for his paintings.30. 填空题:The __________ (历史的价值) is foundational.31. 填空题:The flamingo stands gracefully on one _________. (腿)32. 填空题:A ________ (植物景观规划) beautifies spaces.33. 填空题:The _______ (The 19th Amendment) granted women the right to vote in the US.34. 填空题:The discovery of ________ has had extensive implications for health.35. 听力题:I want to _____ (visit/see) my grandma.36. 听力题:When vinegar and baking soda mix, they produce ________.37. 填空题:The __________ (历史的讨论) can lead to greater understanding.What do you call the main character in a story?a. Antagonistb. Protagonistc. Narratord. Villain答案:B39. 填空题:My favorite subject to study is ______.40. 填空题:I want to learn how to ________ (骑车).41. 选择题:What instrument is known as the "king of instruments"?A. PianoB. OrganC. GuitarD. Violin42. 填空题:People often plant flowers for __________ (美观).43. 听力题:I like to ______ movies with my family. (watch)44. 选择题:What do we call a sweet food made from sugar and typically eaten after a meal?A. DessertB. SnackC. AppetizerD. Side dish答案:A45. 听力题:Planetary atmospheres can protect from harmful _______ radiation.46. 选择题:What do we call a story that is meant to teach a lesson?A. FableB. MythC. LegendD. Folktale答案: AThe chicken lays ______ (鸡蛋). They are a good source of ______ (蛋白质).48. 选择题:What do we call a collection of maps?A. AtlasB. DictionaryC. EncyclopediaD. Almanac答案:A49. 填空题:The __________ (历史的深度) enhances insight.50. 选择题:What do we call the person who designs buildings?A. EngineerB. ArchitectC. ContractorD. Carpenter答案: B51. 选择题:What is your name in English?A. NameB. TitleC. IdentityD. Label52. 听力题:The state of matter that fills its container is a _______.53. 选择题:Which planet is known as the Blue Planet?A. MarsB. EarthC. VenusD. Jupiter答案: B54. 听力题:The __________ can help reveal the effects of human activities on the environment.55. 听力题:The chemical formula for linoleic acid is ______.A __________ (溶胶) is a colloidal mixture with solid particles dispersed in a liquid.57. 听力题:The chemical formula for sodium acetate is _______.58. 选择题:What is the main ingredient in sushi?A. RiceB. NoodlesC. BreadD. Potatoes答案: A59. 填空题:My sister has a keen interest in __________ (天文学).60. 填空题:We saw a _______ (电影) last night.61. 选择题:What is the capital city of Nigeria?A. LagosB. AbujaC. Port HarcourtD. Kano62. 听力题:A _______ can symbolize friendship.63. 填空题:I can ______ (提升) my creativity through art.64. 选择题:What do bees make?A. MilkB. HoneyC. BreadD. Cheese答案:B65. 选择题:What do you call the act of putting something away in a safe place?A. StoringB. HidingC. KeepingD. Securing答案: A66. an Revolution led to the establishment of the ________ (苏维埃政权). 填空题:The Russ67. 填空题:I saw a _______ (小鹿) drinking water.68. 填空题:The capital of Greece is ________ (雅典).69. 填空题:The __________ (国际合作) is needed for global issues.70. 填空题:My dad enjoys helping me with ____.71. 填空题:The flamingo stands gracefully on _______ (一条腿).72. 听力题:Some birds build nests to protect their __________.73. 填空题:My brother is really _____ (幽默) and always makes me laugh.74. 选择题:How many continents are in the world?A. 5B. 6C. 7D. 875. 听力题:A __________ is a substance that cannot be broken down into simpler substances.76. 填空题:The __________ (历史的交织) creates understanding.77. 填空题:I love my _____ (毛绒玩具) that is soft.78. 听力题:The capital of Thailand is ________.79. 填空题:The __________ (历史的桥梁) connect past and present.80. 听力题:Soil is essential for ______ growth.81. 填空题:The _____ (紫罗兰) blooms in spring.82. 听力题:If you drop a feather and a rock, the rock will fall _______.83. 听力题:I want to be a ________.84. 填空题:I like to _______ new things every day.85. 选择题:How many legs does an octopus have?A. 6B. 8C. 10D. 12答案: B86. 填空题:A dolphin is a playful _______ that enjoys swimming in the sea.87. 听力题:The chemical formula for lithium hydroxide is _______.88. 填空题:I have a toy _______ that can change colors.89. 填空题:I am learning how to ________ (游泳) this summer.90. 听力题:The train is coming ___. (soon)91. 选择题:What do we call the holiday celebrated on January 1st?A. ChristmasB. New Year's DayC. Valentine's DayD. Thanksgiving92. 听力题:His favorite food is ________.93. 选择题:What do we call the force that pulls objects toward the Earth?A. MagnetismB. GravityC. FrictionD. Pressure答案:B94. 听力题:The ____ is often seen in gardens looking for food.95. 听力题:The soup is ___ (hot/cold) today.96. 填空题:__________ (植物) use water and sunlight for photosynthesis.97. 选择题:What is the main purpose of a compass?A. To measure weightB. To tell timeC. To find directionD. To measure temperature答案:C98. 填空题:A _____ (海豚) is very friendly.99. 填空题:The raccoon is known for its _______ (聪明) nature.100. 选择题:What is the capital of Estonia?a. Tallinnb. Tartuc. Narvad. Pärnu答案:a。
Solar cell--CIGS
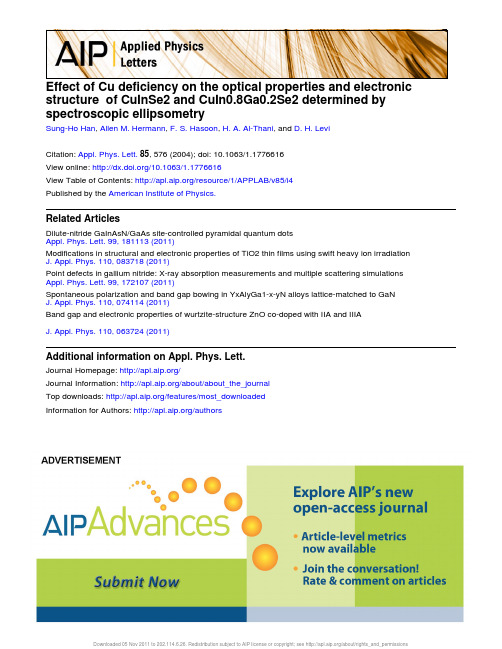
Effect of Cu deficiency on the optical properties and electronic structure of CuInSe2 and CuIn0.8Ga0.2Se2 determined by spectroscopic ellipsometrySung-Ho Han, Allen M. Hermann, F. S. Hasoon, H. A. Al-Thani, and D. H. LeviCitation: Appl. Phys. Lett. 85, 576 (2004); doi: 10.1063/1.1776616View online: /10.1063/1.1776616View Table of Contents: /resource/1/APPLAB/v85/i4Published by the American Institute of Physics.Related ArticlesDilute-nitride GaInAsN/GaAs site-controlled pyramidal quantum dotsAppl. Phys. Lett. 99, 181113 (2011)Modifications in structural and electronic properties of TiO2 thin films using swift heavy ion irradiation J. Appl. Phys. 110, 083718 (2011)Point defects in gallium nitride: X-ray absorption measurements and multiple scattering simulations Appl. Phys. Lett. 99, 172107 (2011)Spontaneous polarization and band gap bowing in YxAlyGa1-x-yN alloys lattice-matched to GaNJ. Appl. Phys. 110, 074114 (2011)Band gap and electronic properties of wurtzite-structure ZnO co-doped with IIA and IIIAJ. Appl. Phys. 110, 063724 (2011)Additional information on Appl. Phys. Lett.Journal Homepage: /Journal Information: /about/about_the_journalTop downloads: /features/most_downloadedInformation for Authors: /authorsEffect of Cu deficiency on the optical properties and electronic structure of CuInSe2and CuIn0.8Ga0.2Se2determined by spectroscopic ellipsometry Sung-Ho Han a)and Allen M.HermannDepartment of Physics,University of Colorado,Boulder,Colorado80303-0390F.S.Hasoon,H.A.Al-Thani,and D.H.LeviNational Renewable Energy Laboratory,1617Cole Boulevard,Golden,Colorado80401-3393(Received29March2004;accepted4June2004)Spectroscopic ellipsometry measurements of CuInSe2(CIS)and CuIn0.8Ga0.2Se2(CIGS)reveal thatthere are important differences in electronic properties between stoichiometric CIS(CIGS)andCu-poor CIS(CIGS).Wefind a reduction in the absorption strength in the spectral region of1–3eV.This reduction can be explained in terms of the Cu3d density of states.Cu-poor CIS(CIGS)materials show an increase in band gap due to the reduction in repulsion between Cu3d andSe4p states.The experimental results have important implications for the function ofpolycrystalline optoelectronic devices.©2004American Institute of Physics.[DOI:10.1063/1.1776616]Polycrystalline thin-film chalcopyrite CuIn1−x Ga x Se2 (CIGS)is currently used as an absorber layer for high-efficiency photovoltaic(PV)solar cells.The efficiency of record laboratory polycrystalline thin-film solar cells based on CIGS has reached nearly20%,1while single-crystalline CIGS solar cells have just reached13%.2Electronic struc-tures of CuB III X2VI materials have been thoroughly studied.3–5 High-efficiency polycrystalline solar cells are always slightly Cu deficient,with about23.5–24.5at.%Cu.There have been studies of the optical properties of CIGS materials with different Ga compositions,6–8but considering the fact that high-efficiency PV solar cells use Cu-poor CIGS,it is crucial to study the effect of content on CIGS electronic properties. In this study,through the analysis of the dielectric function, we compare the electronic structure of Cu-poor ͑21.7at.%Cu͒CuInSe2(CIS)films with stoichemetric ͑25.1at.%Cu͒CISfilms.We also compare the electronic structure of slightly Cu-poor͑23.3at.%Cu͒CIGSfilms withstoichiometric͑24.8at.%Cu͒bulk polycrystalline CIGS.CIGS surfaces are inclined to have Cu vacancies.9,10In con-trast to zinc-blende semiconductors,where the nonpolar (110)surface is more stable than all polar surfaces,the chal-copyrite semiconductor CuInSe2has the lowest energy when the surface has the(112)-cation and͑1¯1¯2¯͒-anion polar facets through defect-induced reconstructions.9Previous work has studied electronic and geometric structures of nearly sto-ichiometric bulk and Cu-poor surfaces.4,10,11In contrast,we focus on Cu-poor CIS and CIGS samples where both the surface and bulk regions are Cu poor to probe the properties of Cu-deficient CIS(CIGS)materials.Spectroscopic ellipsometry(SE)is a powerful technique for determining the optical functions of bulk and thin-film materials.Alonso et al.have reported SE measurements of the pseudodielectric functions of single-crystalline CIS and CuGaSe2(CGS),6as well as bulk polycrystalline CIGS alloys.7In those studies,they used a two-phase model to analyze the ellipsometric data.12Such a treatment is not ap-propriate for the analysis of thin-film polycrystalline materi-als used for real-world CIGS solar cells.The polycrystalline thin-film CIS and CIGS samples were deposited onto molybdenum-coated soda-lime glass.The molybdenum thickness was about1.0m.CIS and CIGS layer thick-nesses were about1.2m and2.0m,respectively.These films were grown by the single-stage coevaporation tech-nique,where thefluxes of Cu,In,Ga,and Se were constant during deposition.To accurately determine the optical prop-erties of these multilayer thin-film samples,one must analyze the SE data using a full multilayer model including the ef-fects of the surface roughness and the underlying molybde-num layer.8We have applied these techniques to determine the dielectric functions for several polycrystalline thinfilms of CIS and CIGS alloys.The ellipsometer used to make the measurements in this study is a J.A.Woollam M2000variable-angle spectroscopic ellipsometer,which uses a rotating compensator design.For this work,ellipsometric spectra were measured at angles of incidence of65°,70°,75°,and80°to ensure an accurate determination of the dielectric function of the material,the thicknesses of the material layer,and surface roughness layer.Auger electron spectroscopy(AES)depth profiles showed that the materials have uniform compositions throughout the entire thickness of thefilms.Thicknesses measured by profilometer are in quantitative agreement with those determined by SE.Inductively coupled plasma(ICP) analysis measures the compositions of thin-film CIS and CIGS.Table I provides at.%Cu of thesefilms as determineda)Also with:National Renewable Energy Laboratory,Golden,CO80401; electronic mail:sung-ho.han@ TABLE I.at.%Cu and critical points analyzed by the CPPB model.All samples are polycrytalline.Stoichiometricthin-film CISCu-poorthin-film CISStoichiometricbulk CIGS aSlightlyCu-poorthin-film CIGS at.%Cu25.121.724.823.3E0͑A,B͒ 1.03 1.08 1.11 1.12E0͑C͒ 1.22 1.29 1.33 1.34a Ref.7.APPLIED PHYSICS LETTERS VOLUME85,NUMBER426JULY2004 0003-6951/2004/85(4)/576/3/$20.00©2004American Institute of Physics576by ICP.X-ray diffraction revealed that these films are single phase for stoichemetric thin-film CIS ͑25.1at.%Cu ͒and slightly Cu-poor ͑23.3at.%Cu ͒thin-film CIGS,and mixed phase for Cu-poor ͑21.7at.%Cu ͒thin-film CIS.The CuIn 0.8Ga 0.2Se 2material studied by Alonso et al.also showed uniform chalcopyrite structure with no secondary phases found.7More detailed discussion on the experimental conditions can be found in Ref.8.Figure 1(a )compares the absorption coefficient spectra of stoichemetric and Cu-poor CIS.Figure 1(b )extends this comparison to CIGS materials to generalize the effect of Cu on CIGS materials.Both Figs.1(a )and 1(b )show similar trends.Relative to the stoichiometric samples,absorption de-creases in E 0,E ͑⌫X ͒,and E 1͑A ͒transitions,but increases in the E ͑⌬X ͒transition for Cu-poor materials.The optical tran-sitions in the spectral range of 1–5eV,can be found else-where .6,7Depression of the absorption coefficient is found in Fig.1(a )between stoichiometric ͑24.8at.%Cu ͒thin-film CIS and Cu-poor ͑21.7at.%Cu ͒thin-film CIS in the spec-tral region,1–3eV.Although the band-gap energies are slightly different due to different Ga compositions,Fig.1(b )also shows the depression of absorption coefficient between stoichiometric bulk polycrystalline CuIn 0.8Ga 0.2Se 2and slightly Cu-poor thin-film CIGS with x ϵGa/͑In+Ga ͒=0.18.According to the theoretical calculations of the elec-tronic band structure and density of state (DOS )of the ter-nary chalcopyrite materials by Jaffe and Zunger,3the upper valence band,within 3–4eV of the valence-band maximum (VBM ),is composed primarily of the Cu 3d orbitals,hybrid-ized with the Se-4p orbitals.Several authors have calculated the band structure and DOS of extremely Cu-poor ␥-phase CIS ͑CuIn 5Se 8͒.5Both of these calculations show a reduction of the DOS within 3–4eV of the VBM.Reduction in the DOS of hole states near the VBM should produce a decrease in absorption coefficient near the band edge.This theoretical result is consistent with our experimental observations.Theoretical calculations of CIS band structure predict another effect of Cu deficiency.As stated above,in ternary chalcopyrite CuB III X 2VI ,the upper valence band is composed of Cu 3d and VI 4p state electrons.This was observed ex-perimentally using synchrotron radiation photoemission spectroscopy.13The repulsive p –d interaction pushes the an-tibonding p –d state that constitutes the VBM to higher en-ergies.In the case of Cu-poor CIS and CIGS,the p –d repul-sion is expected to be less than that of stoichiometric materials.The net effect of the decrease in this repulsive interaction would then be a lowering of the VBM.Hence,we expect an increase of the band gap for Cu-poor CIGS.14We analyze the band gap using the critical-point para-bolic band (CPPB )model.14The fitting procedure is done on the calculated second derivative of dielectric function d 2͑͒/d 2,using the method of smoothing polynomials 15to enhance the structure present in the spectra.The structure of the fundamental absorption edge of CuInSe 2is well known.3Considering crystal-field splitting and spin–orbit in-teraction,the three-fold degenerate ⌫15VBM splits into three transitions E 0͑A ͒,E 0͑B ͒,and E 0͑C ͒.The measured critical points are compiled in Table I.In the case of CIS and CuIn 0.8Ga 0.2Se 2,the separation between E 0͑A ͒and E 0͑B ͒cannot be measured because it is below our resolution.7Thus,the structure is composed of the two degenerate peaks,E 0͑A ,B ͒and E 0͑C ͒.E 0͑A ,B ͒and E 0͑C ͒of stoichemetric thin-film CIS 1.03eV and 1.22eV and those of Cu-poor thin-film CIS are 1.08eV and 1.29eV,respectively.We can see that the band gap increases by 0.05eV for Cu-poor CIS.CPs of CIGS materials show trends analogous to those of CIS materials.We compare the dielectric function of bulk polycrystalline stoichiometric ͑24.8at.%Cu ͒CuIn 0.8Ga 0.2Se 2from Alonso et al.7with the dielectric func-tion of slightly Cu-poor ͑23.3at.%Cu ͒thin-film CIGS with x =0.18.E 0͑A ,B ͒,and E 0͑C ͒of bulk stoichiometric CIGS are extracted from the equation with x =0.18in Alonso et al.7According to that calculation,E 0͑A ,B ͒,and E 0͑C ͒of sto-ichiometric bulk CIGS are 1.11eV and 1.33eV,whereas E 0͑A ,B ͒and E 0͑C ͒of slightly Cu-poor thin-film CIGS are 1.12eV and 1.34eV,respectively.We can see that the band gap increases by 0.01eV.This value is smaller than that of CIS due to the smaller difference in the quantities of at.%Cu.Considering our experimental results in the context of the theoretical calculations of the band structure of stoichio-metric and Cu-poor CIS (CIGS ),3–5we have shown that the reduction of the near band-edge absorption coefficient ob-served in Cu-poor CIS (CIGS )is related to a decrease in the density of states near the VBM.This result has important implications for the functioning of high-efficiencypolycrys-FIG.1.(a )Comparison of absorption coefficients between stoichiometric ͑25.1at.%Cu ͒thin-film CIS and Cu-poor thin-film CIS ͑21.7at.%Cu ͒(b ).Comparison of absorption coefficients between stoichiometric ͑24.8at.%Cu ͒bulk polycrystalline CIGS with x =0.2by Alonso et al.(Ref.7)and slightly Cu poor ͑23.3at.%Cu ͒with x =0.18.talline CIGS thin-film the highest.As stated previously,high-est efficiency CIGS PV devices are slightly Cu poor,with23.5–24.5at.%Cu.Theoretical calculations have shown thatthe most energetically favorable surfaces for CIS are the (112)-cation and͑1¯1¯2¯͒-anion polar facets with defect-induced reconstructions producing a layer of Cu vacancies atthe surface.9Numerous experimental measurements haveconfirmed that CIS(CIGS)surfaces are Cu poor.10Becausegrain boundaries(GBs)can be considered as interior sur-faces,it is reasonable to postulate that in the slightly Cu-poorCIGS material used in high-efficiency solar cells,the mate-rial near the GBs is Cu poor,while the grain interiors(GIs)are nearly stoichiometric.4As shown in Ref.4,the reductionof the DOS near the VBM at the GBs effectively produces acharge-neutral barrier to holes.This effectively passivatesthe GBs by only allowing minority-carrier electrons to pen-etrate the GB region.It is known that the GBs act to getterthe defects and impurities in these materials;hence,passiva-tion of the GBs is exceptionally effective in reducing nonra-diative recombination in CIS(CIGS)thin-film solar cells.The Cu-poor materials studied in this letter are significantly more Cu deficient than the materials used in solar cells.It is reasonable to assume that both GIs and GBs are Cu poor in thesefilms.Hence,our measurements of the optical proper-ties and electronic structure reveal the properties of the GB material in an actual solar cell.Hence,these experimental measurements serve as a confirmation of the theoretical cal-culations put forth in Ref.4.The authors thank H.Moutinho for assistance in atomic force microscopy measurements,R.Bhattacharya for assis-tance in ICP measurements,and J.Pankow for assistance in AES profile measurements.The authors acknowledge valu-able discussions with R.Noufiand C.Persson.This work was supported by the U.S.Department of Energy under Con-tract No.DE-AC36-99GO10337.1K.Ramanathan,M.A.Contreras,C.L.Perkins,S.Asher,F.S.Hasoon,J. Keane,D.Young,M.Romero,W.Metzger,R.Noufi,J.Ward,and A. Duda,Prog.Photovoltaics11,225(2003).2C.H.Champness,Proceedings of the29th IEEE Conference(IEEE,Pis-cataway,NJ,2002),p.732;L.S.Yip and I.Shih,Proceedings of the First World Conference on Photovoltaic Energy Conversion(IEEE,Piscataway, NJ,1994),p.210.3J.E.Jaffe and A.Zunger,Phys.Rev.B27,5176(1983);ibid.28,5822 (1983);ibid.29,1882(1984).4C.Persson and A.Zunger,Phys.Rev.Lett.91,266401(2003).5S.B.Zhang,S.-H.Wei,and A.Zunger,Phys.Rev.B57,9642(1998);C. Domain,ribi,S.Taunier,and J.F.Guillemoles,J.Phys.Chem.Solids 64,1657(2003).6M.I.Alonso,K.Wakita,J.Pascual,M.Garriga,and N.Yamamoto,Phys. Rev.B63,075203(2001).7M.I.Alonso,M.Garriga,C.A.Durante Rincön,E.Hernández,and M. León,Appl.Phys.A:Mater.Sci.Process.74,659(2002).8S.-H.Han,D.H.Levi,H.A.Al-Thani,F.S.Hasoon,R.N.Bhattacharya, and A.M.Hermann,Mater.Res.Soc.Symp.Proc.763,B1.8(2003).9J.E.Jaffe and A.Zunger,Phys.Rev.B64,241304(2001);S.B.Zhang and S.-H.Wei,ibid.65,081402(2002);J.E.Jaffe and A.Zunger,J.Phys. Chem.Solids64,1547(2003).10R.Herberholz,U.Rau,H.W.Schock,T.Haalboom,T.Gödecke,F.Ernst, C.Beilharz,K.W.Benz,and D.Cahen,Eur.Phys.J.:Appl.Phys.6,131 (1999);D.Liao and A.Rockett,Appl.Phys.Lett.82,2829(2003).11A.Meeder,L.Weinhardt,R.Stresing,D.Fuertes Marrón,R.Würz,S.M. Babu,T.Schedel-Niedring,M.C.Lux-Steiner,C.Heske,and E.Umbach, J.Phys.Chem.Solids64,1553(2003);I.M.Kötschau and H.W.Schock, ibid.64,1559(2003);J.M.Merino,M.Di Michiel,and M.León,ibid. 64,1649(2003).12R.M.A.Azzam and N.M.Bashara,Ellipsometry and Polarized Light (North-Holland,Amsterdam,1977).13M.Turowski,G.Magaritondo,M.K.Kelly,and R.D.Tomlinson,Phys. Rev.B31,1022(1985).utenschlager,M.Garriga,S.Logothetidis,and M.Cardona,Phys. Rev.B35,9174(1987).15A.Savitzky and M.J.E.Golay,Anal.Chem.36,1627(1964);J.Steinier, Y.Termonia,and J.Deltour,Anal.Chem.44,1906(1972).。
小学上册第十四次英语上册试卷(含答案)
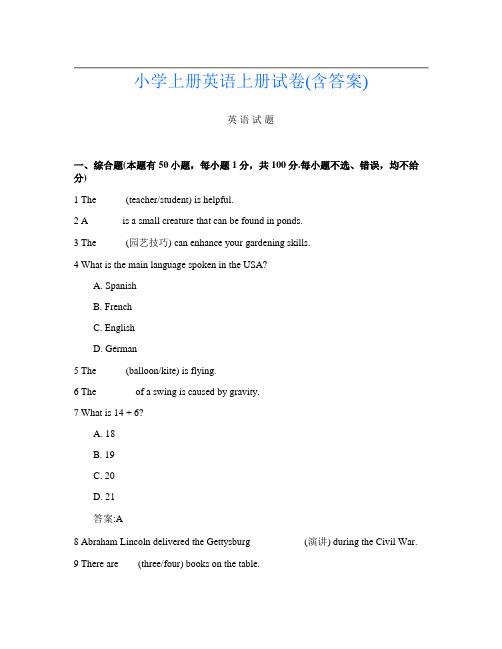
小学上册英语上册试卷(含答案)英语试题一、综合题(本题有50小题,每小题1分,共100分.每小题不选、错误,均不给分)1 The _____ (teacher/student) is helpful.2 A ______ is a small creature that can be found in ponds.3 The _____ (园艺技巧) can enhance your gardening skills.4 What is the main language spoken in the USA?A. SpanishB. FrenchC. EnglishD. German5 The _____ (balloon/kite) is flying.6 The _______ of a swing is caused by gravity.7 What is 14 + 6?A. 18B. 19C. 20D. 21答案:A8 Abraham Lincoln delivered the Gettysburg __________ (演讲) during the Civil War.9 There are ___ (three/four) books on the table.10 How many strings does a violin have?A. 4B. 5C. 6D. 711 What is the name of the famous ancient structure in England?A. StonehengeB. ColosseumC. Great WallD. Pyramids答案: A. Stonehenge12 What do you call a journey to space?a. Explorationb. Expeditionc. Flightd. Mission答案:D13 This ________ (玩具) is full of possibilities.14 In summer, I enjoy _______ (游泳) in the pool.15 What do we call a large body of saltwater?A. OceanB. SeaC. GulfD. Bay答案: A16 What do we call the tool we use to measure temperature?A. ThermometerB. BarometerC. HydrometerD. Anemometer17 I can see a __________ in the sky.18 I like swimming in the _____ (pool/desk).19 We will go to the ______ (museum) on Saturday.20 We have a ______ (愉快的) celebration for achievements.21 What is the name of the famous mouse created by Walt Disney?A. Mickey MouseB. Donald DuckC. GoofyD. Pluto22 I want to _____ (see/watch) a movie tonight.23 My favorite animal is a ______ (狗) because they are loyal.24 _____ (农田) are areas where crops are grown.25 Salt dissolves in _____.26 How many colors are in a rainbow?A. FiveB. SixC. SevenD. Eight27 The chemical formula for glucose is ______.28 What does a light-year measure?B. DistanceC. SpeedD. Mass29 Which season comes after spring?a. Winterb. Summerc. Falld. Autumn答案:b30 The _____ (冬季) can be harsh for some plants.31 The peacock has beautiful _________. (羽毛)32 The chemical industry produces a wide range of ________ for various applications.33 The soup is ______ (warm) and delicious.34 The dog is _______ (running) after the ball.35 The __________ (历史的呼唤) resonates deeply.36 What is the name of the famous river in Egypt?A. NileB. AmazonC. MississippiD. Yangtze答案: A37 What do we call a place where we can see art?A. MuseumB. GalleryD. Workshop38 Pulsars are rotating neutron _______ that emit beams of radiation.39 The main component of starch is ______.40 The ancient Greeks constructed ________ for their religious ceremonies.41 What is the opposite of "happy"?A. JoyfulB. SadC. ExcitedD. Cheerful42 The ________ (农业可持续发展目标) guide practices.43 The _______ of sound can be amplified with a speaker.44 The ________ (feedback) helps us improve.45 What is the name of the first satellite sent into space?A. Apollo 11B. SputnikC. Voyager 1D. Hubble46 What is the name of the famous American landmark in New York City?A. Golden Gate BridgeB. Statue of LibertyC. Empire State BuildingD. Mount Rushmore47 A dwarf planet is similar to a planet but does not clear its orbit of ______.48 What is the term for an animal that eats both plants and meat?A. HerbivoreB. CarnivoreC. OmnivoreD. Insectivore答案:C. Omnivore49 My brother is my silly _______ who always knows how to make me smile.50 The __________ is a large area of sandy soil.51 What is the name of the famous bear in the cartoon?A. PaddingtonB. Winnie the PoohC. YogiD. Baloo答案:B52 What is the capital of Portugal?A. LisbonB. PortoC. FaroD. Braga53 The study of rocks is known as ______.54 What is the name of the device used to measure pressure?a. Barometerb. Hygrometerc. Thermometerd. Anemometer答案:a55 The __________ (历史的抉择) shapes destiny.56 The __________ is known for its unique wildlife and natural beauty. (新西兰)57 The chemical formula for acetylene is ______.58 Which of these is a cold-blooded animal?A. HumanB. SharkC. SnakeD. Bird答案:C59 I like to _____ (collect/throw) stamps.60 I want to grow a ________ to make my room bright.61 Which animal is known as "man's best friend"?A. CatB. DogC. RabbitD. Fish答案:B62 The _______ (小驴) brays loudly in the pasture.63 My favorite animal at the zoo is a ________.64 The __________ (历史的探索者) uncover stories long forgotten.65 What is the main ingredient in a smoothie?A. IceB. MilkC. FruitD. Sugar66 What is the name of the fairy tale character with long hair?A. CinderellaB. RapunzelC. Snow WhiteD. Belle67 What do we call the solid part of the Earth?A. AtmosphereB. HydrosphereC. LithosphereD. Biosphere答案:C68 I need to _____ (finish/start) my homework.69 What is the name of the large body of saltwater that covers most of the Earth?A. LakeB. SeaC. OceanD. River答案:C70 A cheetah is the fastest _______ on land, running swiftly to catch its prey.71 What do we call a scientist who studies the effects of pollution?a. Environmental scientistb. Ecologistc. Biologistd. Chemist答案:a72 What is the name of the ocean located between Africa and Australia?A. Indian OceanB. Atlantic OceanC. Pacific OceanD. Arctic Ocean答案:A73 The ______ (植物分类) helps identify species.74 The basic unit of a protein is an ________.75 What do you call a young ant?A. LarvaB. PupaC. WorkerD. Queen76 The chemical properties of a substance are observed during a _____ change.77 The first man on the moon was ________ (尼尔·阿姆斯特朗).78 My favorite subject is __________. (数学)79 A garden can attract various ______ (昆虫).80 What is the capital of Cyprus?A. NicosiaB. LimassolC. LarnacaD. Famagusta答案:A81 What do we call the sound a sheep makes?A. MooB. QuackC. BaaD. Neigh答案:C82 I can ________ (jump) very high.83 I like to draw _____ in my notebook.84 My brother has a great sense of __________ (幽默感).85 What do we call the traditional game played with a ball and net?A. CricketB. BaseballC. TennisD. Soccer86 The ____ is known for its agility and speed.87 What do you call a place where animals are kept for public display?A. ParkB. ZooC. FarmD. Aquarium答案: B88 My cousin is very __________ (活泼的) and energetic.89 What is the name of the famous artist known for his paintings of water lilies?A. Claude MonetB. Vincent van GoghC. Pablo PicassoD. Salvador Dalí90 What do we call a young female horse?A. FillyB. ColtC. FoalD. Mare91 What do you call a person who swims?A. DiverB. SurferC. SwimmerD. Sailor答案:C92 What do you call the liquid that comes from trees?A. GumB. SyrupC. SapD. Juice答案:C93 What is the capital of Australia?A. SydneyB. CanberraC. MelbourneD. Brisbane94 A fuel is a substance that can be burned to produce _____.95 The __________ is heavy with clouds. (天空)96 What is the smallest unit of life?A. TissueB. OrganC. CellD. Organism97 The study of compounds containing carbon is called ______ chemistry.98 The process of making charcoal involves _______.99 The __________ is known for its diverse ecosystems.100 We enjoy _____ to the library. (going)。
高一英语生物词汇单选题30题
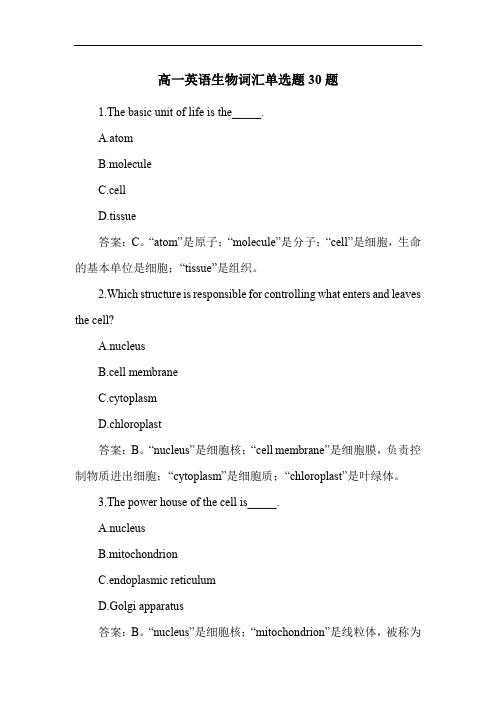
高一英语生物词汇单选题30题1.The basic unit of life is the_____.A.atomB.moleculeC.cellD.tissue答案:C。
“atom”是原子;“molecule”是分子;“cell”是细胞,生命的基本单位是细胞;“tissue”是组织。
2.Which structure is responsible for controlling what enters and leaves the cell?A.nucleusB.cell membraneC.cytoplasmD.chloroplast答案:B。
“nucleus”是细胞核;“cell membrane”是细胞膜,负责控制物质进出细胞;“cytoplasm”是细胞质;“chloroplast”是叶绿体。
3.The power house of the cell is_____.A.nucleusB.mitochondrionC.endoplasmic reticulumD.Golgi apparatus答案:B。
“nucleus”是细胞核;“mitochondrion”是线粒体,被称为细胞的动力工厂;“endoplasmic reticulum”是内质网;“Golgi apparatus”是高尔基体。
4.Which organelle is involved in protein synthesis?A.ribosomeB.lysosomeC.vacuoleD.peroxisome答案:A。
“ribosome”是核糖体,参与蛋白质合成;“lysosome”是溶酶体;“vacuole”是液泡;“peroxisome”是过氧化物酶体。
5.The storage site of genetic information is the_____.A.nucleusB.mitochondrionC.chloroplastD.cytoplasm答案:A。
基质血管组分(SVF)临床分离技术研究
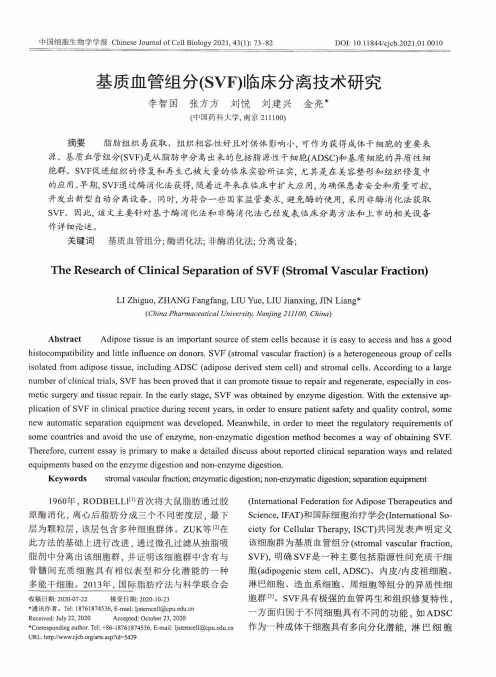
中国细胞生物学学报 Chinese Journal of Cell Biology2021,43( 1):73-82 DOI: 10.11844/cjcb.2021.01.0010基质血管组分(SVF)临床分离技术研究李智国张方方刘悦刘建兴金亮*(中国药科大学,南京211100)摘要 脂肪组织易获取、组织相容性好且对供体影响小,可作为获得成体干细胞的重要来源。
基质血管组分(S V F)是从脂肪中分离出来的包括脂源性干细胞(A D S C)和基质细胞的异质性细 胞群。
S V F促进组织的修复和再生已被大量的临床实验所证实,尤其是在美容整形和组织修复中的应用。
早期,S V F通过酶消化法获得,随着近年来在临床中扩大应用,为确保患者安全和质量可控,开发出新型自动分离设备。
同时,为符合一些国家监管要求,避免酶的使用,采用非酶消化法获取 S V F。
因此,该文主要针对基于酶消化法和非酶消化法已经发表临床分离方法和上市的相关设备 作详细论述。
关键词基质血管组分;酶消化法;非酶消化法;分离设备;The Research of Clinical Separation of SVF (Stromal Vascular Fraction)LI Zhiguo,Z H A N G Fangfang,L I U Y u e,L I U Jianxing,JIN Liang*{China Pharmaceutical University, Nanjing 211100, China)Abstract Adipose tissue is an important source of stem cells because i t is easy to access and has a g o o d histocompatibility and l i t t l e influence on donors.S V F(stromal vascular fraction)is a heterogeneous group of cells isolated from adipose tissue,including A D S C(adipose derived stem cell)and stromal cells.According to a large n u m b e r of clinical trials,S V F has been proved that i t can promote tissue to repair and regenerate,especially in cosmetic surgery and tissue repair.In the early stage,S V F w a s obtained by e n z y m e digestion.With the extensive application of S V F in clinical practice during recent years,in order to ensure patient safety and quality control,s o m e n e w automatic separation equipment w a s developed.M e a n w h i l e,in order to meet the regulatory requirements of s o m e countries and avoid the use of e n z y m e,non-enzymatic digestion m e t h o d b e c o m e s a w a y of obtaining S V F. Therefore,current essay i s primary to m a k e a detailed discuss about reported clinical separation w a y s a nd related equipments based on the e n z y m e digestion and non-enzyme digestion.K e y w o r d s stromal vascular fraction;enzymatic digestion;non-enzymatic digestion;separation equipmentI960年,R O D B E L L l[11首次将大鼠脂肪通过胶 原酶消化,离心后脂肪分成三个不同密度层,最下 层为颗粒层,该层包含多种细胞群体。
perovskite solar cells
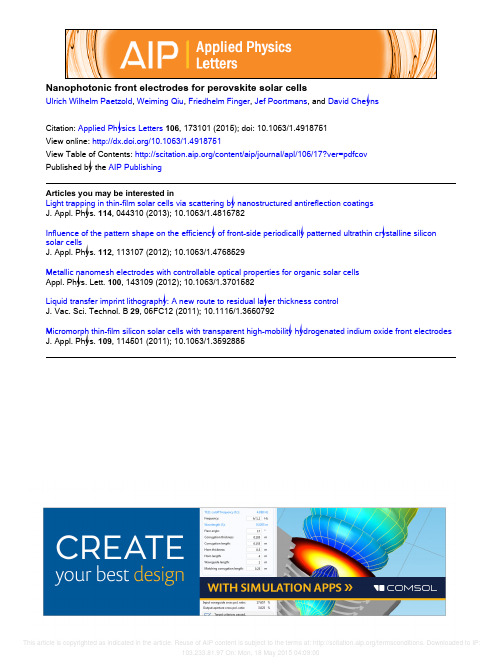
Nanophotonic front electrodes for perovskite solar cellsUlrich Wilhelm Paetzold, Weiming Qiu, Friedhelm Finger, Jef Poortmans, and David CheynsCitation: Applied Physics Letters 106, 173101 (2015); doi: 10.1063/1.4918751View online: /10.1063/1.4918751View Table of Contents: /content/aip/journal/apl/106/17?ver=pdfcovPublished by the AIP PublishingArticles you may be interested inLight trapping in thin-film solar cells via scattering by nanostructured antireflection coatingsJ. Appl. Phys. 114, 044310 (2013); 10.1063/1.4816782Influence of the pattern shape on the efficiency of front-side periodically patterned ultrathin crystalline silicon solar cellsJ. Appl. Phys. 112, 113107 (2012); 10.1063/1.4768529Metallic nanomesh electrodes with controllable optical properties for organic solar cellsAppl. Phys. Lett. 100, 143109 (2012); 10.1063/1.3701582Liquid transfer imprint lithography: A new route to residual layer thickness controlJ. Vac. Sci. Technol. B 29, 06FC12 (2011); 10.1116/1.3660792Micromorph thin-film silicon solar cells with transparent high-mobility hydrogenated indium oxide front electrodes J. Appl. Phys. 109, 114501 (2011); 10.1063/1.3592885Nanophotonic front electrodes for perovskite solar cellsUlrich Wilhelm Paetzold,1,2,a)Weiming Qiu,1Friedhelm Finger,2Jef Poortmans,1,3,4and David Cheyns 11IMEC v.z.w.,Kapeldreef 75,3001Leuven,Belgium2IEK5-Photovoltaik,Forschungszentrum Juelich GmbH,D-52425Juelich,Germany 3Katholieke Universiteit Leuven,ESAT-Electa,Kardinaal Mercierlaan,3001Leuven,Belgium 4Hasselt University,Wetenschapspark 1,3590Diepenbeek,Belgium(Received 5February 2015;accepted 10April 2015;published online 27April 2015)In less than 3years’time,a vast progress in power conversion efficiencies of organometal halide perovskite solar cells has been achieved by optimization of the device architecture,charge transport layers,and interfaces.A further increase in these efficiencies is expected from an improvement in the optical properties via anti-reflection coatings and nanophotonic light management concepts.In this contribution,we report on the development and implementation of a nanophotonic front electrode for perovskite solar cells.The nanostructures were replicated via the versatile and large-area compatible UV-nanoimprint lithography.The shallow design of the used transparent and conductive nanostructures enabled easy integration into our solution-based baseline process.Prototype methylammonium lead iodide perovskite solar cells show an improvement of 5%in short-circuit current density and an improvement from 9.6%to 9.9%in power conversionefficiency compared to the flat reference device.VC 2015AIP Publishing LLC .[/10.1063/1.4918751]The enormous potential and recent vast development of organometal halide perovskite solar cells has triggered an unprecedented fast progress of its power conversion efficien-cies (g ).Today,less than 3years after the first reported solid state perovskite solar cell 1a record efficiency of 20.1%2has been certified and several groups have reported efficiencies above 17%.3,4The current key challenges of perovskite solar cells are the apparent uncertainties about the stability of the devices,5–7the discrepancies in measuring routines due to hysteresis,8,9and the difficulties in replacing the toxic heavy metal lead.10,11The promise of perovskite solar cells is founded in their close to optimal combination of electrical and optical material properties.Free carrier diffusion lengths from 100nm (Refs.12and 13)up to 1l m (Ref.13)have been reported for the current work-horse materials,methyl-ammonium lead iodide perovskite (CH 3NH 3PbI 3)and the partially chlorine-substituted mixed halide perovskite (CH 3NH 3PbI 3ÀX Cl X ).In addition,the material shows a sharp optical absorption edge at wavelengths of 800nm (i.e.,1.55eV)with absorption depths below 350nm (for k <750nm).14Since the combination of these material properties allows for optically thick layers (!350nm)in combination with close to optimal charge carrier collection,the first attention in the development of perovskite solar cells was directed towards the optimization 3,15–18of the charge carriers dynamics in the device architecture,3,7,13,15–23in the charge transport layers,12,24,25and in the interfaces.4,26However,in order to further improve the efficiencies,the optical aspects of organometal halide perovskite solar cells need optimization.The reflection at the air/substrate inter-face is around 4%and additional reflection and parasitic absorption losses are apparent at the transparent frontcontact.While a broad range of anti-reflection coatings for the front side of transparent glass and plastic substrates have been explored,27research on nanophotonic transparent front electrodes for perovskite solar cells for improved light incou-pling is lacking.Such nanostructured electrodes areintendedFIG.1.Nanophotonic ITO front electrodes for application in perovskite thin-film solar cells.(a)A photograph of the ITO front electrode on the glass substrate.The colored haze depicts the light diffraction of the nanopatterned regions of the electrode.Scanning electron microscopy (SEM)image of the surface topography of the nanopatterned ITO front electrode with a period of (b)500nm and (c)1000nm.a)Author to whom correspondence should be addressed.Electronic mail:ulrich.w.paetzold.ext@imec.be0003-6951/2015/106(17)/173101/5/$30.00VC 2015AIP Publishing LLC 106,173101-1APPLIED PHYSICS LETTERS 106,173101(2015)to reduce reflection at both interfaces of the front electrode. Moreover,they can be used to improve the light-trapping, i.e.,increase the optical path length in the photo-active material.Such nanophotonic transparent front electrodes have already been researched and prototyped for a number of other solar cells,such as organic based PV,28–30 thin-film silicon,31,32GaAs(Ref.33),and crystalline silicon.34,35In this contribution,we report on the prototyp-ing and application of transparent nanophotonic front elec-trodes for improved light incoupling in perovskite solar cells.The nanophotonic front electrode was prepared by nano-patterning a transparent glass-like resist on a plane glass sub-strate(3Â3cm2).With a soft-polymer mold and a UV-nanoimprint process,periodic nanopatterns were transferred into the transparent resist that hardens at intense UV expo-sure(details on the nanoimprint process are described in the supplemental material36well as the literature37,38).A125nm thick indium-tin-oxide(ITO)layer was then sputtered onto the resist,forming the front electrode.The nanopatterned regions exhibit areas of0.5Â0.5cm2and periods ranging from1200nm to500nm.In contrast to theflat reference, light diffraction at periodic nanopatterns causes spectral selective redirection of light that can be observed by eye (shown in Figure1(a)).Due to the moderate conformal growth of the sputtered ITO,the rectangular nanostructures at the surface of the UV-nanoimprinted resist(80nm height and300nm width)reveal a semi-ellipsoidal shape(around 80nm height and320nm width)at the ITO surface(see Figures1(b)and1(c)).A methylammonium lead iodide perovskite solar cell was fabricated on top of the ITO front electrode.Since our substrate holds both nanopatterned andflat regions,it was possible to process both nanopatterned and reference devices in parallel and on the same substrate,i.e.,using the same sputtered ITO material quality and layer thickness.36 Moreover,our experiments ensure optimal comparability of the device architecture and material compositions,which is otherwise difficult to achieve for solution-processed layers. In Figure2(a),a photograph of the entire device is shown. Five areas of0.5Â0.5cm2with decreasing brightness indi-cate the regions of the nanopatterns at the ITO front elec-trode.Some of the nanopatterns show a colored haze due to light diffraction at the two-dimensional nanopattern of the front electrode.The solar cells layer sequence was deposited onto these electrodes in the conventional device architecture, with the hole transport layer at the front electrode and the electron transport layer at the Al back electrode(layer sequence in Figure2(b)).The device architecture presented here has been proposed before in literature by Bai et al.39A FIG.2.Prototype methylammonium lead iodide solar cell.(a)A photography of the substrate from the front side.Thefive areas with decreased brightness indicate the regions of the nanopatterns of the ITO front electrode.For the500,600,and800nm patterns,light diffraction is apparent by a colored haze.(b)A schematic illustration of the nanopatterned perovskite solar cell.(c)Images of atomic force microscopy measurements of the nanopatterned resist,the nanopat-terned resist covered with ITO,and the rear side of the perovskite solar cell.(d)The absorptance A and(e)the external quantum efficiency EQE of the methyl-ammonium lead iodide solar cells with nanophotonic electrodes compared to solar cells deposited on theflat reference electrode.The period of the nanopattern is varied from500nm to1200nm.spin-coated poly(3,4-ethylenedioxythiophene):poly(4-styre-nesulfonate)(PEDOT:PSS)layer acts as hole transport layer in contact with the ITO front electrode.The photoactive methylammonium lead iodide perovskite CH3NH3PbI3Cl layer was prepared by annealing a“pristine”spin-coated precursor solution(CH3NH3PbI3ÀX Cl X).A spin-coated [6,6]-phenyl C60-butyric acid methyl ester(PC60BM)and a spin-coated zinc oxide establish the bilayer-structured elec-tron transporting layer.Finally,the reflective Al back elec-trode was deposited through a shadow mask defining the cell area(approximately15mm2).More details on the processing conditions and composition of the applied solutions are pre-sented in the supplemental material.36The thickness of the perovskite absorber layer was determined by profilometer measurements to320nm.The conformity of the ITO deposi-tion on the nanopatterned substrates was investigated with atomic force microscopy measurements(Figure2(c)).It is shown that the front electrode is nanostructured but the back electrode isflat.Since the photoactive perovskite layer is significantly thicker than the nanostructure at the front elec-trode,the nanopattern is not apparent at the back electrode. The combination of PC60BM and ZnO layers is of particular importance to our device architecture.This double layered electron transport structurefills the cavities existing in the methylammonium lead iodide perovskite layer after forma-tion of the crystallites,while a low resistive contact with the cathode is attained with the ZnO.The photograph in Figure2(a)reveals that absorptance of incident light is increased for the solar cells deposited on the nanopatterned front electrodes compared to theflat reference.In order to quantify this observation we show the spectral resolved absorptance of the solar cells deposited on the nanophotonic front electrodes with periods ranging from 1200nm to500nm in Figure2(d).With decreasing period, the absorptance in the entire wavelength range is enhanced. This enhancement is attributed to a decreased light reflection and increased light transmission at the nanostructured ITO front electrode and the adjacent nanostructured layers.The cause of this decrease reflection and increased transmission lies in the complex nanophotonic properties of the nanostruc-tured layer stack of the ITO front electrode and the adjacent layers.We can picture these nanophotonic properties in two macroscopic effects:(i)an effective match of the refractive index due to the dimensions of the nanostructures below the wavelength of incident light and(ii)parts of the light reflected at the nanopatterned electrode are diffracted at the two-dimensional grating texture beyond the angle of total internal refraction of the front glass/air interface.The com-bined effects ensure that incident light is transmitted with reduced parasitic reflection losses into the photo-active per-ovskite layer.Having shown that the absorptance of perovskite solar cells with nanophotonic front electrodes is enhanced,the arising question is to which extent this enhancement leads to enhanced photocurrent generation,i.e.,power conversion efficiency g.To answer this question and spectrally resolve the effect,we compare the external quantum efficiency EQE of the solar cells deposited on the nanophotonic front electrode(with periods of500nm and800nm)to the solar cell prepared on theflat reference front electrode(cf.Figure 2(e)).At very short wavelengths below400nm,the parasitic losses in the ITO front electrode are known to dominate the absorptance of the device such that the EQE is reduced.For wavelengths longer than800nm,the EQE vanishes since no light can be absorbed in the perovskite layer above its band gap of1.55eV.For wavelength between400nm and750nm, the EQE shows a similar trend compared to the absorptance, i.e.,the EQE is enhanced in this wavelength region for the solar cells prepared on the nanophotonic front electrodes. Moreover,the enhancement in EQE is maximum for the nanophotonic front electrodes with500nm period of the nanopatterns,which also showed the strongest enhancement in absorptance(cf.Figure2(d)).From the EQE,the short-circuit current density J SC of the solar cells is derived under consideration of the AM1.5spectrum.Wefind that the best nanophotonic ITO front electrode shows5%improvement in J SC compared to theflat reference due to reduced reflection of incident light at the front interfaces of the solar cell.Importantly,the enhancement in J SC also leads to an improved initial power conversion efficiency g of9.9%com-pared to9.6%for the solar cells prepared on the nanopho-tonic front electrode and theflat electrode,respectively.This relative improvement of3%in g is less than the relative improvement of5%in J SC,since a slight decrease infill fac-tor FF is observed,while comparable open-circuit voltages V OC are obtained(see Table I).Our prototype irrevocably demonstrates that solution-processed methylammonium lead iodide perovskite solar cells of very comparable electrical properties can be fabricated onflat ITO electrodes and nano-patterned ITO front electrodes with moderate aspect ratio of the nanostructures.The shallow design of the used transpar-ent and conductive nanostructures enabled easy integration into our solution-based processing of the solar cell.It shall be noted that power conversion efficiencies of up to14.2% have been realized onflat commercialized ITO substrates for the given device architecture.However,due to reduced transmittance,reduced conductivity,and increased rough-ness of our in-house ITO,the efficiency of theflat reference ITO decreased significantly.To validate our interpretation of the improved light incoupling at the nanophotonic ITO front electrode in meth-ylammonium lead iodide perovskite solar cells,we performTABLE I.Solar cell parameters.Fill factor FF,open-circuit voltage V OC,short-circuit current density J SC,and the power conversion efficiency g of the meth-ylammonium lead iodide solar cells fabricated on aflat electrode and nanophotonic electrodes with a period,p,of500nm and1000nm.ITO electrode FF V OC(V)J SC(mA/cm2)(calc.from EQE)g(%)Flat0.590.8918.39.6 Nanophotonic(p¼500nm)0.590.8719.49.9 Nanophotonic(p¼1000nm)0.570.8818.59.3three-dimensional electromagnetic simulations of solar cells with flat and with nanophotonic ITO front electrodes.From the simulated electromagnetic field distribution,we derive the total absorptance of the solar cell and the absorptance in the photo-active perovskite layer.The thicknesses of the layers are taken from profilometer measurements and the ge-ometry of the nanopatterns are taken from AFM images (Figure 2(c)).To simplify the geometry,we assumed a cubic nanostructure of the nanopatterns on the resist (height 80nm,width 300nm)and semi-ellipsoidal nanostructures (height 80nm,width 320nm)at the surface of the ITO.The optical data of the perovskite material is taken from ellipsometry measurements of bare perovskite films (details in the supple-mentary material 36).Our simulations show a convincing agreement between simulated and measured absorptance with flat and with nanophotonic ITO front electrodes (see Figure 3(a)).Moreover,assuming charge carrier collection efficiency (viz.,internal quantum efficiency)of 90%in the photoactive perovskite layer,we obtain a simulated EQE that matches well the measured EQE of the solar cells with flat and nanophotonic electrode (see Figure 3(b)).These agreements demonstrate the relevance and predictive power of our simulations.Most importantly,our simulations prove that the nanopatterns of the front electrodes induce improved light incoupling that accounts for the reduced parasitic reflection.Having proven that the simulated reflectance and EQE are in good agreement with the measured data of the prototype so-lar cells,we numerically investigate the dependence of the light management of the nanophotonic ITO front electrode on the geometry of the nanopattern.In Figure 3(c),the simulated average reflectance h R i 450–700(for 400nm <k <700nm)is shown for periods of the nanopattern from 400nm to 1200nm and height of the nanostructures of 20nm,40nm,80nm,and 120nm.Two fundamental relations are validated:(i)the broad band reflectance decreases with increasing geometrical fill factor of the nanostructures (i.e.,decreasing period)until a minimum reflectance at periods around 350–400nm and (ii)the broad band reflectance decreases with increasing height of nanostructures.These fundamental relations have been thor-oughly studied for multiple applications of anti-reflection coat-ings.27The simulations show that improved geometries of the nanopatterns of ITO electrodes bear the potential to further decrease the reflectance losses.For example,half-ellipsoidal nanostructures with a base-diameter of 320nm (as applied in this contribution)with periods of 400nm and a height of 120nm leads to broad band reflectance of 5.7%.This broad band reflectance is already very close to the minimum broad band reflectance of around 4.3%given by the planar air/glass interface.In future studies,the shape,unit cell,and dielectric layer stack of the nanophotonic front electrode will be optimized to further reduce the reflectance losses.Foreseen strategies to further decrease the reflection are nanopatterns with increasing geometrical fill factor of the nanostructures (decreasing period,hexagonal unit cell),an increasing height,and a more tapered shape of the nanostructures.These strat-egies will enable a further improved transmission at the interfa-ces of perovskite solar cells.The applied UV-nanoimprint process shown here is capable of replication at precision down to a few nanometers,including random textures and high as-pect ratios periodic nanopatters.40–43The presented nanophotonic front electrodes for improved broad band light transmittance into the solar cell can also be applied for improved light transmittance at the rear side of the perovskite solar cell.Such functionality is highly desired in the long wavelength range for tandem device concepts with perovskite solar cell.15,44,45Similar nanopat-terns to those investigated in this contribution have already been replicated into transparent substrates on large scale for photovoltaic applications at low costs.46Thus,the industrial realization of our nanophotonic front electrode for improved light incoupling in perovskite solar cells is inreach.FIG.3.Numerical simulations.(a)Comparison of simulated absorptance A sim and measured absorptance A exp ,as well as simulated external quantum efficiency EQE sim and measured EQE exp of methylammonium lead iodide solar cell with flat ITO front electrode.(b)The same comparison but for a nanophotonic ITO front electrode (period 500nm).(c)Simulated average reflectance (for 350<k <750nm)for nanophotonic electrodes with various heights of the nanopattern and various periods.In conclusion,we present a versatile,large-area applica-ble nanophotonic front electrode for application in perov-skite solar cells.The nanopatterns of the nanophotonic ITO electrode reduce the parasitic reflectance losses by effec-tively matching the refractive indices of the layers at the front side of the solar cell.Our prototype methylammonium lead iodide perovskite solar cell shows an relative improve-ment of5%in short-circuit current density and a power con-version efficiency improvement from9.6%to9.9% compared to theflat reference device.The authors thank Erwin Vandenplas,M.Smeets, Manuela Meier,and A.Schmalen for the technical support. The fruitful discussions with M.Meier,T.Kirchartz,U.Rau, J.Tait,J.Bastos,E.Voroshazi,and A.Hadipour are highly appreciated.This research has received(partial)funding from the Flemish Government—Department of Economics, Science and Innovation as well as the European Commission’s Seventh Framework Program(Mujulima 604148).The work of Ulrich W.Paetzold wasfinanced by the PostDoc Program of the German Academic Exchange Program(DAAD).1H.-S.Kim,C.-R.Lee,J.-H.Im,K.-B.Lee,T.Moehl,A.Marchioro,S.-J. Moon,R.Humphry-Baker,J.-H.Yum,J.E.Moser,M.Gr€a tzel,and N.-G. Park,Sci.Rep.2,591(2012).2Research Cell Efficiency Records see /ncpv/.3N.-G.Park,Mater.Today18(2),65(2014).4H.Zhou,Q.Chen,G.Li,S.Luo,T.-b.Song,H.-S.Duan,Z.Hong,J. You,Y.Liu,and Y.Yang,Science345,542(2014).5A.Mei,X.Li,L.Liu,Z.Ku,T.Liu,Y.Rong,M.Xu,M.Hu,J.Chen,Y. Yang,M.Gratzel,and H.Han,Science345,295(2014).6G.Niu,W.Li,F.Meng,L.Wang,H.Dong,and Y.Qiu,J.Mater.Chem.A 2,705(2014).7J.Burschka,N.Pellet,S.-J.Moon,R.Humphry-Baker,P.Gao,M.K. Nazeeruddin,and M.Gr€a tzel,Nature499,316(2013).8S.A.Bretschneider,J.Weickert,J.A.Dorman,and L.Schmidt-Mende, APL Mater.2,040701(2014).9H.J.Snaith,A.Abate,J.M.Ball,G.E.Eperon,T.Leijtens,N.K.Noel,S.D.Stranks,J.T.Wang,K.Wojciechowski,and W.Zhang,J.Phys.Chem. Lett.5,1511(2014).10N.K.Noel,S.D.Stranks,A.Abate,C.Wehrenfennig,S.Guarnera,A.-A. Haghighirad,A.Sadhanala,G.E.Eperon,S.K.Pathak,M.B.Johnston,A. Petrozza,L.M.Herz,and H.J.Snaith,Energy Environ.Sci.7,3061(2014). 11F.Hao,C.C.Stoumpos,D.H.Cao,R.P.H.Chang,and M.G.Kanatzidis, Nature Photon.8,489(2014).12G.Xing,N.Mathews,S.Sun,S.S.Lim,m,M.Gr€a tzel,S. Mhaisalkar,and T.C.Sum,Science342,344(2013).13S.D.Stranks,G.E.Eperon,G.Grancini,C.Menelaou,M.J.P.Alcocer, T.Leijtens,L.M.Herz,A.Petrozza,and H.J.Snaith,Science342,341 (2013).14S.De Wolf,J.Holovsky,S.-J.Moon,P.L€o per,B.Niesen,M.Ledinsky, F.-J.Haug,J.-H.Yum,and C.Ballif,J.Phys.Chem.Lett.5,1035(2014). 15H.J.Snaith,J.Phys.Chem.Lett.4,3623(2013).16O.Malinkiewicz,A.Yella,Y.H.Lee,G.M.Espallargas,M.Graetzel,M. K.Nazeeruddin,and H.J.Bolink,Nature Photon.8,128(2013).17M.Gr€a tzel,Nat.Mater.13,838(2014).18M.A.Green,A.Ho-Baillie,and H.J.Snaith,Nature Photon.8,506 (2014).19C.Wehrenfennig,G.E.Eperon,M.B.Johnston,H.J.Snaith,and L.M. Herz,Adv.Mater.26,1584(2014).20Y.Takahashi,R.Obara,Z.-Z.Lin,Y.Takahashi,T.Naito,T.Inabe,S. Ishibashi,and K.Terakura,Dalton Trans.40,5563(2011).21M.Liu,M.B.Johnston,and H.J.Snaith,Nature501,395(2013).22M.M.Lee,J.Teuscher,T.Miyasaka,T.N.Murakami,and H.J.Snaith, Science338,643(2012).23Q.Chen,H.Zhou,Z.Hong,S.Luo,H.Duan,H.Wang,Y.Liu,G.Li,and Y.Yang,J.Am.Chem.Soc.136,622(2014).24L.Zhu,J.Xiao,J.Shi,J.Wang,S.Lv,Y.Xu,Y.Luo,Y.Xiao,S.Wang, Q.Meng,X.Li,and D.Li,“Efficient CH3NH3PbI3perovskite solar cells with2TPA-n-DP hole-transporting layers,”Nano Res.(published online). 25J.H.Heo,S.H.Im,J.H.Noh,T.N.Mandal,C.Lim,J.A.Chang,Y.H. Lee,H.Kim,A.Sarkar,M.K.Nazeeruddin,M.Gr€a tzel,and S.Il Seok, Nature Photon.7,486(2013).26W.Jaegermann,A.Klein,and T.Mayer,Adv.Mater.21,4196(2009).27H.K.Raut,V.A.Ganesh,A.S.Nair,and S.Ramakrishna,Energy Environ.Sci.4,3779(2011).28S.Y.Chou and W.Ding,Opt.Express21(Suppl1),A60(2013).29D.-H.Ko,J.R.Tumbleston,A.Gadisa,M.Aryal,Y.Liu,R.Lopez,and E.T.Samulski,J.Mater.Chem.21,16293(2011).30D.H.Wang,D.-G.Choi,K.-J.Lee,J.-H.Jeong,S.H.Jeon,O.O.Park, and J.H.Park,Org.Electron.11,285(2010).31C.Battaglia, C.-M.Hsu,K.S€o derstr€o m,J.Escarr e, F.-J.Haug,M. Charrie`re,M.Boccard,M.Despeisse,D.T.L.Alexander,M.Cantoni,Y. Cui,and C.Ballif,ACS Nano6,2790(2012).32U.W.Paetzold,M.Smeets,M.Meier,K.Bittkau,T.Merdzhanova,V. Smirnov,D.Michaelis,C.Waechter,R.Carius,and U.Rau,Appl.Phys. Lett.104,131102(2014).33K.S.Cho,P.Mandal,K.Kim,I.H.Baek,S.Lee,H.Lim,D.J.Cho,S. Kim,J.Lee,and F.Rotermund,mun.284,2608(2011).34P.Bermel,C.Luo,L.Zeng,L.C.Kimerling,and J.D.Joannopoulos,Opt. Express15,16986(2007).35C.Trompoukis,O.El Daif,V.Depauw,I.Gordon,and J.Poortmans, Appl.Phys.Lett.101,103901(2012).36See supplementary material at /10.1063/1.4918751for details on the fabrication of nanophotonic front electrodes,fabrication of the perovskite solar cells,and characterization techniques.37M.Meier,U.W.Paetzold,M.Pr€o mpers,T.Merdzhanova,R.Carius,and A.Gordijn,Prog.Photovoltaics:Res.Appl.22,1226(2013).38U.W.Paetzold,W.Zhang,M.Pr€o mpers,J.Kirchhoff,T.Merdzhanova, S.Michard,R.Carius,A.Gordijn,and M.Meier,Mater.Sci.Eng.B178, 617(2013).39S.Bai,Z.Wu,X.Wu,Y.Jin,N.Zhao,Z.Chen,Q.Mei,X.Wang,Z.Ye, T.Song,R.Liu,S.Lee,and B.Sun,Nano Res.7,1749(2014).40H.Schift,J.Vac.Sci.Technol.B:Microelectron.Nanometer Struct.26, 458(2008).41S.Y.Chou,P.R.Krauss,and P.J.Renstrom,Science272,85(1996).42J.Escarr e,K.S€o derstr€o m,C.Battaglia,F.Haug,and C.Ballif,Sol. Energy Mater.Sol.Cells95,881(2011).43E.A.Costner,M.W.Lin,W.Jen,and C.G.Willson,Annu.Rev.Mater. Res.39,155(2009).44B.W.Schneider,l,S.Baker-Finch,and T.P.White,Opt.Express 22,A1422(2014).45P.Loper,B.Niesen,S.-J.Moon,S.Martin de Nicolas,J.Holovsky,Z. Remes,M.Ledinsky,F.-J.Haug,J.-H.Yum,S.De Wolf,and C.Ballif, IEEE J.Photovolt.4,1545(2014).46A.J.M.van Erven,M.Steltenpool,M.Bos,J.Rutten,G.van der Hofstad, J.Muller,H.de Groot,J.de Ruijter,A.Tavakoliyaraki,B.Titulaer,and G. Rajeswaran,in201238th IEEE Photovoltaic Specialists Conference (IEEE,2012),pp.000690–000693.。
- 1、下载文档前请自行甄别文档内容的完整性,平台不提供额外的编辑、内容补充、找答案等附加服务。
- 2、"仅部分预览"的文档,不可在线预览部分如存在完整性等问题,可反馈申请退款(可完整预览的文档不适用该条件!)。
- 3、如文档侵犯您的权益,请联系客服反馈,我们会尽快为您处理(人工客服工作时间:9:00-18:30)。
19.2% efficient c-Si solar cells using ion implantationJian Wu, Yumei Li, Xusheng Wang, Linjun Zhang Canadian Solar Inc., 199 Lushan Road, SND, Suzhou, Jiangsu, ChinaABSTRACTIon-implantation offers numerous advantages for solar cell manufacturing. Canadian Solar Inc. (CSI) has developed an average efficiency 19.23%, champion efficiency 19.39% solar cell (156mm) process using a high throughput Varian (Applied Materials) Solion ion-implant tool based on a beam line design. The path to commercialization has included module process development, achieving 265W with 6×10 cells. With the installation of the high-throughput ion implanter for PV, optimized unit processes were transferred from the lab to the production floor at CSI.1. INTRODUCTIONIon implantation has been reported as a breakthrough technology in PV industry to drive down $/W cost. Ion implantation is a process of precisely introducing a known amount of energetic particles into any substrate to alter its material properties. Emitters formed by ion implantation show improved cell efficiency due to the benefit in blue wavelength region. The quality of the emitters formed by ion implantation followed by activation has clear advantages over emitters made by conventional POCl3diffusion, in terms of lattice defect recovery as well as dopant activation (no dead layer), as depicted by TEM micrographs and SIMS profiles. By tailoring the activation temperature and ambient, a layer of oxide can be formed during activation [1]. J0e data show surface passivation is better with SiO2/SiN x stack than single SiN x layer. We prove ion implantation technology is mass production ready for PV cell manufacturing, and it is an essential enabler of lower $/W.2. EXPERIMENTSFig. 1Process simplification for fabrication of ion implantation emitter cells: (a) standard POCl3 process;(b) implanted process.Ion implanted process is described in Fig.1 (a). Fig.1 (b) presents traditional POCl3diffusion solar cell process as reference. Ion implantation is a single side process, so edge isolation is not necessary. SIMS and TEM are conducted on both POCl3diffused and ion implanted samples, to understand and characterize the emitter quality. Lower surface concentration from ion implantation is observed. The precise dose control of ion implanter provides excellent uniformity and repeatability within wafer and wafer-to-wafer on 156mm pseudo sq. wafers. Metallization was optimized to account for the modified doping profile and SiO2/SiN x stack passivation layer. The internal quantum efficiency (IQE) and dopantprofile are compared between cells made by ion implantation and incumbent POCl 3 diffusion process. Modules are fabricated and characterized to ensure that the efficiency gain is fully transferred into increased module power output.3. RESULTS AND DISCUSSIONS3.1 Emitter uniformity on Si-wafersFig. 2 Sheet resistance comparisons between ion implanted (top) and POCl 3-diffused (bottom) wafers.Fig.2 shows the sheet resistance mapping with 49 points by ion implantation (top), and POCl 3-diffusion (bottom). For a fair comparison, the target sheet resistance (R sq ) for both cases was set ~60Ω/sq. The R sq standard deviation of diffused wafer is 5.47Ω/sq, larger than 2.38Ω/sq of implanted wafer. It indicates the emitter fabricated by ion implantation is relatively more uniform and acceptable for mass productionprocess.3.2 Superior Junction QualityOne concern about the implantation process is if the implanted regions can be perfectly re-grown without any residual damage within the layer. Defects in the re-grown lattice may cause increased carrier recombination in the emitter.(a)(b)(c)Fig. 3 Cross section images of: (a) as implanted; (b) furnace annealed; (c) POCl 3 diffused wafer samples, illustrating no residual defects in the crystal lattice inthe implanted emitter.Fig.3 shows a TEM comparison of implanted and diffused emitters with similar R sq values of 60Ω/sq. Image (a) shows as-implanted sample and reveals a high uniform and fully amorphized ~30nm region. After a furnace annealing, the amorphous region completely recrystallized without any defect, as shown in Fig.3 (b). The cross-section comparisons illustrate the perfectly re-grown Si crystal lattice with no dead regions in the near surface region of the implanted emitter. On contrary, POCl 3diffused image (c) presents ~10nm dark region, correlated with the junction defects, or called dead region. Image (b) also illustrates the presence of a thin layer of thermally grown silicon dioxide during the post-implantation annealing. The smoother interface between the oxide layer and the Si sample for the implanted emitters (reduced surface recombination area) combined with the high quality thermal oxide (with very few interface states) is also evident in the picture.3.3 Comparison of dopant junction profileIon implantation provides a powerful method to precisely control the amount of dopant in the emitter layer. The dopant profile may use an appropriate annealing step to maximize cell performance. For the same sheet resistance, the implanted emitter has a lower surface concentration than diffused one, thus enabling lowerrecombination in the emitter region.Fig. 4Comparison of dopant junction profiles between POCl3-diffused and ion implanted emitter. The implanted emitter with and without furnace annealed both had been measured.Figure 4 shows the SIMS profile of the emitters of POCl3-diffusion and ion-implantation before and after annealing. All test samples have similar R sq values of 60Ω/sq. POCl3emitter profile shows a thin layer with non-activated peak concentration up to 1022cm-3, relating to the dead layer. For the dopant activation profiles, the as-implanted sample presents surface concentration of 7×1020cm-3. After high temperature annealing, phosphorus atoms are activated and driven deeper into Si substrate, to form an even lower (2×1020cm-3) concentration dopant emitter.3.4 Improved Surface Passivation with thermal oxideOxide passivation has been used successfully on very high efficiency solar cells [3]. Since ion implantation does not require any parasitic dopant containing layers (such as the phosphor-silicate glass in POCl3 diffusion), it is possible to grow and retain a high quality thermal oxide for implanted emitters without the removal/ additional process. As seen in the Table 1, implied V oc@1 Sun value over 650mV of ion implanted solar cell is indicative of very low recombination in the emitters due to the excellent surface passivation after the anneal. After SiN x and firing, the value increases even more, due to H atoms passivation in Si bulk. Table 1. Implied V oc data after activation/diffusion, SiN x and firing of the ion implanted and POCl3 emitter.As further evidence of superior emitter quality, we refer to Fig. 5 that shows the internal quantum efficiencies(IQE) of POCl 3-diffused and ion implanted solar cells. The IQE of the ion implanted solar cell was higher than that of POCl 3-diffused in the short wavelength region, the so-called blue response, as shown in Fig 5. The excellent blue response of the cell is indicative of very low recombination in the emitter and excellent surface passivation, as well as the absence of dead layer of the emitter.Fig. 5 Comparison of the internal quantum efficiency (IQE) for POCl 3-diffused and ion implanted solar cell.3.6 Performance of the cell efficiency in productionIon implantation provides a powerful method to precisely control the amount of dopant in the emitter layer. The uniformity and precision doping via implant enables a repeatable process for fabrication of lightly doped emitter regions [4-6]. The efficiency distribution of over 2000pcs cells produced recently is shown in Fig. 6 with an average efficiency of 19.23% and champion efficiency of 19.39%. The absolute efficiency gain reaches 0.5%, accordingto Table 2.Fig. 6 Histogram of cell efficiency for selected cells in production.Table 2. Comparison of the average I-V parameters between ion-implanted and POCl 3-diffused solar cells of more than 2000 pcs.3.7 Module powerFull size modules (60cells) with conventional layout have been made with 19.2% ion implanted solar cells. They can achieve over 265W module power, with the cell to module (CTM) loss ~ 3%. It is proved the ion implanted process repeatability and stability for manufacturing process. The EL image of the modules reveals the good current response in Fig. 7.Fig. 7 The EL image of the module made with ion implanted cells.4. DISCUSSION & CONCLUSIONSIn this study, we have reported the approach to boost the cell performance on standard p-Cz cells with an ion implantation process flow. The key benefits for improving cell efficiency are a) Process Simplification through elimination of PSG strip and junction isolation steps b) improved quality of junction doping (lower surface dopant concentration and elimination of dead layer), c) improved surface passivation through the integration of thermal oxidation in the process flow. A large number of cells efficiency over 19.2% is achieved by using ion implantation technology. Improved cell efficiency is transferred to higher module power output over 265W. REFERENCES[1] M. B. Spitzer, C. J. Keavney, Proceedings 18th IEEE Photovoltaic Specialist Conference (1985) 43.[2] J. Ben ick, et al., “Very Low Emitter Saturation Current Densities on Ion Implanted Boron Emitters”, 25th EU- PVSEC, Valencia, Spain, 2010.[3] J. Zhao et al., ”24.5% Efficiency Silicon PERT Cells on MCZ Substrates and 24.7% Efficiency PERL Cells on Fz Substrates”, Prog. Photovolt: Res. Appl. 7, 1999, pp. 471-474.[4] R. Low, A. Gupta et al., “High Efficiency Selective Emitter Enabled through Patterned Ion Imp lantation”, 35th IEEE PVSC, Honolulu, 2010. [5] J. Benick, et al., “Very Low Emitter Saturation Current Densities on Ion Implanted Boron Emitters”, 25th EU- PVSEC, Valencia, Spain, 2010.[6] T. Janssens, et al., “Implantation for an Excellent Definition of Doping Profiles in Si Solar Cells”, 25th EUPVSEC, Valencia, Spain, 2010.。