挤压态ZK60镁合金的高温力学性能及其超塑性
铸态、挤压态和快速凝固态ZK60镁合金微观组织及压缩性能

铸态、挤压态和快速凝固态ZK60镁合金微观组织及压缩性能王敬丰;魏怡芸;吴夏;潘复生;汤爱涛;丁培道【摘要】Rapid solidified ZK60 magnesium alloy was prepared by copper mold casting. The micro-structure of the as-cast, as-extruded and rapid solidified ZK60 magnesium alloys were analyzed by X-ray diffractometer(XRD) and scanning electron microscope(SEM). The results show that the micro-structure of rapid solidified ZK60 magnesium alloys are refined and MgZn phases in dispersed particles are uniformly distributed in the matrix. In addition, the eutectic microstructure with high Zr content can be found in the matrix. The compression strength of rapid solidified ZK60 magnesium alloys were higher than those of as-cast and as-extruded conditions,e. g. an ultimate strength of 444MPa in rapid solidified ZK60 magnesium alloys.%采用铜模喷铸的方法制备得到快速凝固态ZK60镁合金,并通过X射线衍射仪,扫描显微镜和能谱分析仪对铸态、挤压态和快速凝固态ZK60镁合金的组织结构及相的组成进行表征.结果发现:快速凝固态ZK60镁合金的组织均匀细小,并且样品中弥散分布着颗粒状的MgZn相,除此之外,该合金中还存在尺寸较大的富Zr共晶团.通过压缩实验发现,快速凝固态ZK60镁合金具有高强度,其压缩断裂强度达到444MPa,远高于铸态和挤压态.【期刊名称】《材料工程》【年(卷),期】2011(000)008【总页数】5页(P32-35,41)【关键词】快速凝固;镁合金;压缩性能【作者】王敬丰;魏怡芸;吴夏;潘复生;汤爱涛;丁培道【作者单位】重庆大学国家镁合金材料工程技术研究中心,重庆400044;燕山大学亚稳材料制备技术与科学国家重点实验室,河北秦皇岛066004;重庆大学国家镁合金材料工程技术研究中心,重庆400044;重庆大学国家镁合金材料工程技术研究中心,重庆400044;重庆大学国家镁合金材料工程技术研究中心,重庆400044;重庆大学国家镁合金材料工程技术研究中心,重庆400044;重庆大学国家镁合金材料工程技术研究中心,重庆400044【正文语种】中文【中图分类】TG146.2近年来,随着航空航天及汽车等领域的快速发展,对材料的轻质高强性能提出了越来越高的要求。
板材镁合金牌号
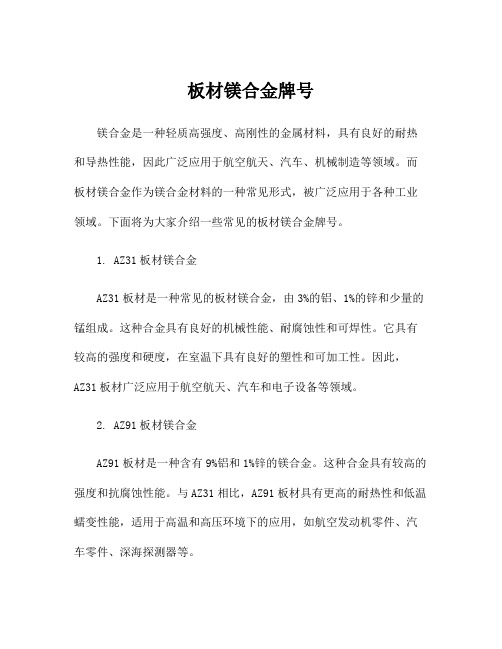
板材镁合金牌号镁合金是一种轻质高强度、高刚性的金属材料,具有良好的耐热和导热性能,因此广泛应用于航空航天、汽车、机械制造等领域。
而板材镁合金作为镁合金材料的一种常见形式,被广泛应用于各种工业领域。
下面将为大家介绍一些常见的板材镁合金牌号。
1. AZ31板材镁合金AZ31板材是一种常见的板材镁合金,由3%的铝、1%的锌和少量的锰组成。
这种合金具有良好的机械性能、耐腐蚀性和可焊性。
它具有较高的强度和硬度,在室温下具有良好的塑性和可加工性。
因此,AZ31板材广泛应用于航空航天、汽车和电子设备等领域。
2. AZ91板材镁合金AZ91板材是一种含有9%铝和1%锌的镁合金。
这种合金具有较高的强度和抗腐蚀性能。
与AZ31相比,AZ91板材具有更高的耐热性和低温蠕变性能,适用于高温和高压环境下的应用,如航空发动机零件、汽车零件、深海探测器等。
3. AZ61板材镁合金AZ61板材是一种含有6%铝和1%锌的镁合金。
这种合金具有良好的机械性能和抗蠕变性。
AZ61合金比AZ31合金具有更高的强度和硬度,且耐腐蚀性能更好。
AZ61板材广泛应用于汽车零件、航空电子设备和船舶构件等领域。
4. ZK60板材镁合金ZK60板材是一种含有6%锌和0.5%锆的镁合金。
这种合金具有良好的强度和硬度,同时具有较高的塑性和抗腐蚀性能。
ZK60板材常用于航空航天和军工领域,如飞机结构件、导弹部件和武器发射系统。
除了上述常见的板材镁合金牌号外,还有许多其他种类的板材镁合金。
每种合金都有其特定的性能和应用领域。
在选择和应用板材镁合金时,需根据具体的需求和要求,选择合适的牌号和合金组分。
总结一下,板材镁合金是一种重要的金属材料,在航空航天、汽车、机械制造等领域有广泛的应用。
常见的板材镁合金牌号包括AZ31、AZ91、AZ61和ZK60等。
每种合金都有其独特的性能和特点,适用于不同的应用领域。
选择适合的牌号和合金组分对于提高产品质量和性能至关重要。
ZK60镁合金

镁合金材料简介随着航空航天、交通运输、信息产业的发展,新型轻合金材料的研发逐渐受到各国的高度重视。
在许多领域,传统钢铁材料已逐渐被各种综合性能更为优良的新型材料所替代。
镁合金是以镁为基加入其他元素组成的合金,是目前实际应用中最轻的金属结构材料,具有密度小、强度高、阻尼性、切削加工性和铸造性能好的优点。
一、镁合金的分类一般来说,镁合金按以下三种方式进行分类:合金化学成分、合金是否含锆和成形工艺。
按镁合金的合金化学成分分为:二元系镁合金 Mg-A1、Mg-Zn、Mg-Mn、Mg —RE、Mg-Zr和Mg-Li等,三元系及多组元系镁合金Mg-A1-Zn、Mg-A1-Mn、Mg-Mn-Ce、Mg-RE—Zr、Mg-Zn—Zr。
根据加工工艺的不同可分为两大类,即铸造镁合金和变形镁合金。
两者在成分、组织和性能上存在的差异,使其在应用上也有明显区别。
大量镁合金产品采用铸造工艺进行生产,其产品主要应用于汽车零件、机件壳罩和电气构件等。
铸造工艺最常用的是压铸工艺,其主要特点是生产效率高、铸件质量好、组织优良、可生产薄壁及形状复杂的构件等。
最常见的压铸镁合金是Mg-A1系合金。
通过控制杂质含量,可通过压铸工艺生产出力学、耐腐蚀等综合性能优良的镁合金铸件,如最常见、使用量最大的AZ31合金。
但压铸成形的镁合金件难免内部会存在显微气孔等缺陷而且力学性能较差。
变形镁合金一般是指可用挤压、锻造等塑性成形方法加工成形的镁合金。
与铸造镁合金相比,镁合金热变形后微观组织结构得到细化,消除了铸造产生的缺陷,产品具有更高的强度、更好的延展性,其大大提高的综合性能能够满足更多样的结构件的需求。
变形镁合金由于具有这些优点,这些年得到广泛的关注并取得了长足的发展,形成了一些系列(如变形镁合金可以根据是否能进行热处理强化,分成可热处理强化变形镁合金和不可热处理强化变形镁合金)。
二、镁合金的特点与其他金属相比,镁合金具有以下特点:(1)镁合金的比重小,是目前最轻的结构材料,密度在1.75—1.85g/cm3之间,是钢密度的23%,铝密度的67%,塑料密度的170%。
激光焊接ZK60变形镁合金的组织和力学性能
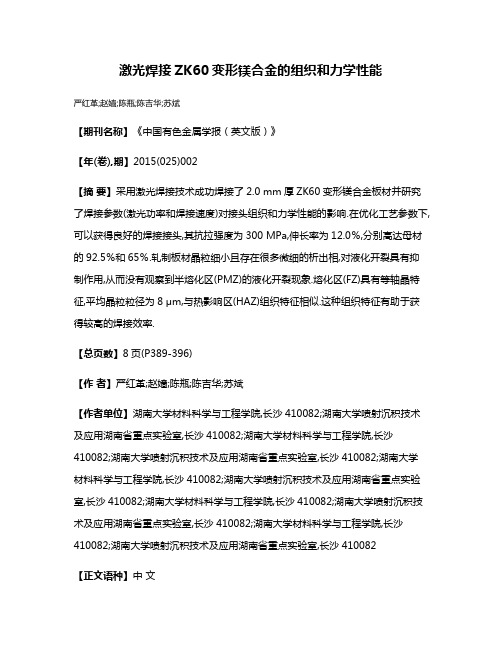
激光焊接ZK60变形镁合金的组织和力学性能
严红革;赵嫱;陈瓶;陈吉华;苏斌
【期刊名称】《中国有色金属学报(英文版)》
【年(卷),期】2015(025)002
【摘要】采用激光焊接技术成功焊接了2.0 mm厚ZK60变形镁合金板材并研究了焊接参数(激光功率和焊接速度)对接头组织和力学性能的影响.在优化工艺参数下,可以获得良好的焊接接头,其抗拉强度为300 MPa,伸长率为12.0%,分别高达母材的92.5%和65%.轧制板材晶粒细小且存在很多微细的析出相,对液化开裂具有抑制作用,从而没有观察到半熔化区(PMZ)的液化开裂现象.熔化区(FZ)具有等轴晶特征,平均晶粒粒径为8 μm,与热影响区(HAZ)组织特征相似.这种组织特征有助于获得较高的焊接效率.
【总页数】8页(P389-396)
【作者】严红革;赵嫱;陈瓶;陈吉华;苏斌
【作者单位】湖南大学材料科学与工程学院,长沙410082;湖南大学喷射沉积技术及应用湖南省重点实验室,长沙410082;湖南大学材料科学与工程学院,长沙410082;湖南大学喷射沉积技术及应用湖南省重点实验室,长沙410082;湖南大学材料科学与工程学院,长沙410082;湖南大学喷射沉积技术及应用湖南省重点实验室,长沙410082;湖南大学材料科学与工程学院,长沙410082;湖南大学喷射沉积技术及应用湖南省重点实验室,长沙410082;湖南大学材料科学与工程学院,长沙410082;湖南大学喷射沉积技术及应用湖南省重点实验室,长沙410082
【正文语种】中文
因版权原因,仅展示原文概要,查看原文内容请购买。
铸态、挤压态和快速凝固态ZK60镁合金微观组织及压缩性能
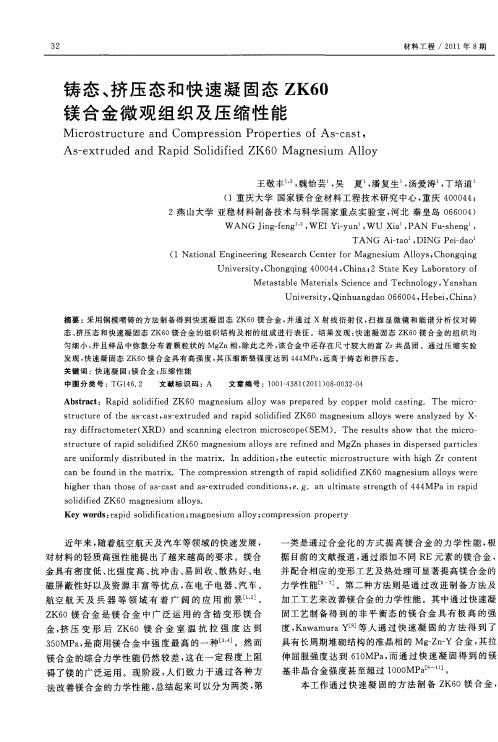
As e t u e n p d S l i e K6 a n su Al y — x r d d a d Ra i o i f d Z M g e i m l d i 0 o
王敬 丰 。魏 怡芸 吴 , ,
夏 潘 复生 汤爱 涛 丁 培道 , , ,
( 1重庆 大学 国家镁 合金 材料 工程技 术研 究 中心 , 重庆 4 0 4 ; 0 0 4
2燕 山大学 亚稳 材料 制备技 术与 科学 国家重 点实验 室 , 北 秦 皇 岛 0 6 0 ) 河 6 0 4
W ANG ig fn , E — u W U a PAN —h n Jn —e g W IYiy n , Xi , Fu s e g ,
T A N G it o DI G ida A — a , N Pe— o
3 2
材 料 工 程 /2 1 年 8期 01
铸 态 、 压 态 和 快 速 凝 固态 Z 0 挤 K6 镁 合 金微 观 组 织及 压 缩性 能
M ir s r c ur n m p e so o e te fA s c s , c o t u t ea d Co r s i n Pr p ri so — a t
Un v r iy Qih a g a 6 0 4, b iCh n ) ie st , n u n d o 0 6 0 He e, ia
摘 要 : 用 铜 模 喷 铸 的方 法 制 备 得 到 快 速 凝 固态 Z 0镁 合 金 , 通 过 x 射 线 衍 射 仪 , 描 显 微 镜 和 能 谱 分 析 仪 对 铸 采 K6 并 扫
s r c ur fr p d s ldiid ZK6 a e i t u t e o a i o i fe m gn sum lo r e i e nd M g ha e n d s r e r il s 0 a l ysa e r fn d a Zn p s si ipe s d pa tc e a e u f r l it i t d i he m a rx. I d to t u e tc mi r s r t e wih hi h Zr c nt nt r nio m y d s rbu e n t t i n a dii n, he e t c i c o t uc ur t g o e c n b o d i he ma rx The c m p e so t e gt fr p d s i fe a e f un n t t i . o r s i n s r n h o a i ol id ZK6 a e i di 0 m gn sum lo r a l yswe e h g rt n t os fa — a t a s e r de o dii ns, . i he ha h e o s c s nd a — xt u d c n to e g. a li t t e gt 4 M Pa i a d n u tma e s r n h of4 4 n r pi
基于管材挤压实验的ZK60镁合金本构关系模型
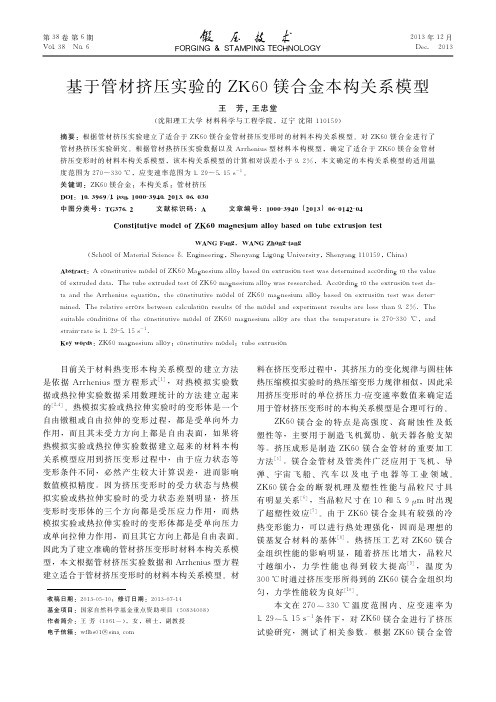
自由镦粗或自由 拉 伸 的 变 形 过 程 , 都 是 受 单 向 外 力 作用 , 而且其未 受 力 方 向 上 都 是 自 由 表 面 , 如 果 将 热模拟实验或热拉 伸 实 验 数 据 建 立 起 来 的 材 料 本 构 关系模型应用到 挤 压 变 形 过 程 中 , 由 于 应 力 状 态 等 变形条件不同 , 必 然 产 生 较 大 计 算 误 差 , 进 而 影 响 数值模拟精度 。 因为 挤 压 变 形 时 的 受 力 状 态 与 热 模 拟实验或热拉伸 实 验 时 的 受 力 状 态 差 别 明 显 , 挤 压 变形时变形体的 三 个 方 向 都 是 受 压 应 力 作 用 , 而 热 模拟实验或热拉伸 实 验 时 的 变 形 体 都 是 受 单 向 压 力 或单向拉伸力作用 , 而且其它方向上都是自由表面 。 因此为了建立准确的管材挤压变形时材料本构关系模 型 , 本文根据管材挤压实验数据和 A r r h e n i u s型方程 建立适合于管材挤压变形时的材料本构关系模型 。 材
3 0 0℃ 时通过挤压变形所得到的 Z K 6 0 镁合金组织均 [ 1 0] 匀 , 力学性能较为良好 。 本文在 2 7 0~3 3 0 ℃ 温 度 范 围 内、 应 变 速 率 为 -1 1 . 2 9 . 1 5s 条件下 , 对 Z K 6 0 镁合金进行了挤压 ~5 试验研究 , 测 试 了 相 关 参 数 。 根 据 Z K 6 0镁合金管
2
(
1 l n ε ( ) 3 n σ 在温度变化的条件下 ,Q 随温度的变化而变化 ,
α=
( ) 1
n
R, n, A 均 是 常 数, 根 据 式 ( 1) 可 以 得 到 Q 的 α, ) 。 计算式 ( 4
镁合金热挤压型材
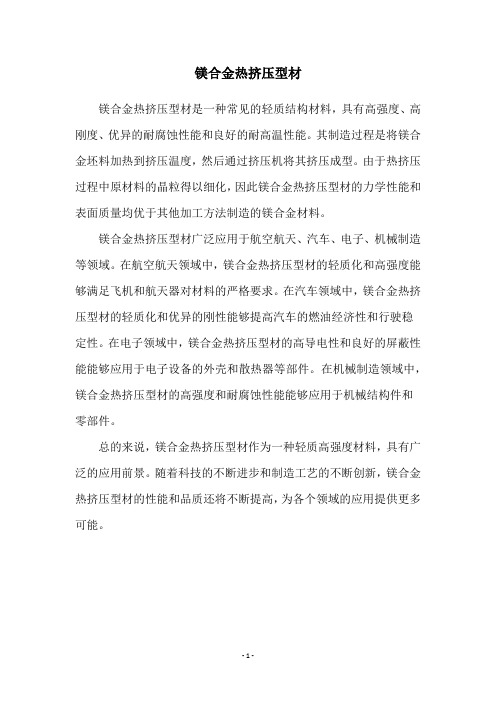
镁合金热挤压型材
镁合金热挤压型材是一种常见的轻质结构材料,具有高强度、高刚度、优异的耐腐蚀性能和良好的耐高温性能。
其制造过程是将镁合金坯料加热到挤压温度,然后通过挤压机将其挤压成型。
由于热挤压过程中原材料的晶粒得以细化,因此镁合金热挤压型材的力学性能和表面质量均优于其他加工方法制造的镁合金材料。
镁合金热挤压型材广泛应用于航空航天、汽车、电子、机械制造等领域。
在航空航天领域中,镁合金热挤压型材的轻质化和高强度能够满足飞机和航天器对材料的严格要求。
在汽车领域中,镁合金热挤压型材的轻质化和优异的刚性能够提高汽车的燃油经济性和行驶稳
定性。
在电子领域中,镁合金热挤压型材的高导电性和良好的屏蔽性能能够应用于电子设备的外壳和散热器等部件。
在机械制造领域中,镁合金热挤压型材的高强度和耐腐蚀性能能够应用于机械结构件和
零部件。
总的来说,镁合金热挤压型材作为一种轻质高强度材料,具有广泛的应用前景。
随着科技的不断进步和制造工艺的不断创新,镁合金热挤压型材的性能和品质还将不断提高,为各个领域的应用提供更多可能。
- 1 -。
材料学院-热处理对ZK60镁合金组织与力学性能的影响

Effects of heat treatment on microstructure andmechanical properties of ZK60 Mg alloyCHEN Xian-hua1, 2, HUANG Xiao-wang1, PAN Fu-sheng1, 2, TANG Ai-tao1, 2, WANG Jing-feng1, 2, ZHANG Ding-fei1, 21. College of Materials Science and Engineering, Chongqing University, Chongqing 400044, China;2. National Engineering Research Center for Magnesium Alloys, Chongqing University, Chongqing 400044, ChinaReceived 23 September 2010; accepted 25 December 2010Abstract: The microstructure and mechanical properties of ZK60 Mg alloy were investigated under different solution treatments and artificial aging conditions. When as-cast ZK60 alloy was solution treated at 400 °C for 10 h and artificially aged at 150 °C, the volume fraction of precipitates increased with the aging time up to 30 h. When the as-cast ZK60 alloy was solution treated at 400 °C for 10 h and artificially aged at 200 °C for 15−20 h, the volume fraction of precipitates reached a peak value. Tensile test at room temperature showed that a high density of the second phase precipitates was beneficial to improving the strength and elongation. Solution treatment at 400 °C for 10 h and artificial aging at 150 °C for 30 h is considered the optimum heat treatment condition to obtain a good combination of strength and ductility.Key words: Mg alloy; heat treatment; precipitate; strength; ductility1 IntroductionThere has been a renaissance in magnesium alloy research in the last few years driven by increasing global awareness of the link between energy usage and climate change[1]. Magnesium alloys are potentially suitable candidates for replacing heavier materials in some automobile and aerospace parts[2−4]. The mechanical limitations of Mg alloys reside basically in their limited strength, plasticity and formability[5−6]. In order to improve the mechanical properties, some investigations focused on optimizing heat treatment processing routes[7−9].In general, solution treatment and/or aging process is employed to enhance the mechanical properties of Mg alloys[8]. Solution treatment causes the precipitates dissolving into the Mg matrix and forms supersaturated solid solution during quenching. The aging process results in the transformation of supersaturated solid solution to fine precipitates. The precipitation of the second phases is dependent on aging temperature, time and chemical composition of the alloys. Among these variables, the temperature and time are controlled to elucidate the heat treatment process. Moreover, the aging time for these alloys must be carefully chosen to allow sufficient precipitation of the second phases. Depending upon their volume fraction and morphology, the second phase constituents strengthen and embrittle the matrix to varying degree.ZK60 Mg alloy is one of the most typical high-strength wrought Mg alloys. Considerable attention was concentrated lately on the study of the influence of heat treatment on the mechanical properties of ZK60 alloy subjected to plastic deformation, including extrusion, rolling, forging and so on[10−12]. However, quite limited studies reported on the heat treatment procedures of as-cast ZK60 alloy[13]. In the present work, the effects of solution treatment and artificial aging on the microstructure and mechanical properties of ZK60 alloy in cast state are examined. This study also aims to determine the structure transformation of ZK60 alloy after various heat treatments, and hence optimize the heat treatment parameters.Foundation item: Project (2009BB4215) supported by the Natural Science Foundation Project of CQ CSTC, China; Project (20090191120013) supported by the PhD Programs Foundation of Ministry of Education of China; Project (50725413) supported by the National Natural ScienceFoundation of China; Project (2007CB613704) supported by the National Basic Research Program of China; Project (CDJZR10 13 00 01)supported by the Fundamental Research Funds for the Central Universities, ChinaCorresponding author: CHEN Xian-hua; Tel: +86-23-65102821; E-mail: xhchen@DOI:10.1016/S1003-6326(11)60776-0CHEN Xian-hua, et al/Trans. Nonferrous Met. Soc. China 21(2011) 754−760 7552 ExperimentalAlloy ingots of ZK60 were prepared from high purity Mg (99.98%), Zn (99.99%) and Mg-27.85Zr (mass fraction, %) master alloy in an electric resistance furnace. When the temperature reached 780 °C, molten alloy was stirred for 8 min and subsequently held for 30 and 45 min, respectively. Then semi-continuous casting was used to prepare cylinder ingots of ZK60 magnesium alloy with a diameter of 90 mm. The actual chemical composition of the alloy is listed in Table 1. The ingots were solution treated at 400 °C for 10 h and subsequently water quenched. Artificial aging treatment was then performed at 150 and 200 °C for 5 , 10 , 15 , 20 , 25 and 30 h, respectively.Table 1 Chemical composition of ZK60 alloy (mass fraction, %)Si Fe Cu Mn Be 0.003 2 0.002 0 0.000 61 0.003 9 0.000 34Ni Zn Zr Mg<0.000 56.370.53Bal.The microstructural characterization was performed by using optical microscopy (OM) and scanning electron microscopy (SEM, TESCAN VEGA Ⅱ LMU) with an accelerating voltage of 20 kV and conventional filament source. Specimens for OM and SEM observations were mechanically polished and chemically etched in a solution of 1.5 g picric acid + 25 mL ethanol + 5 mL acetic acid + 10 mL water. Phase analyses were performed with a Rigaku D/MAX-2500PC X-ray diffractometer (XRD).The mechanical properties of the as-cast and heat-treated ZK60 alloys were measured by tensile testing and microhardness measurement at room temperature. Tensile specimens with size ofd5 mm ×50 mm were prepared through machining the alloy rods.Tensile tests were carried out on a CMT5105 material test machine at a constant strain rate of 10−3 s −1. The reported Vickers hardness (HV) represented the average value of ten separate measurements taken at randomly selected points under a load of 0.49 N for 10 s.3 Results and discussionThe typical microstructure of as-cast alloy is presented in Fig.1. The OM image (Fig.1(a)) shows the bright contrast of α-Mg matrix and the dark contrast of the second phase. The eutectic is a mixture of Mg-Zn phase and supersaturated solid solution. Fig.1(b) shows the fine structure of Mg-Zn phase.Fig.1 OM (a) and SEM (b) images of as-cast ZK60 alloyThe as-cast ZK60 alloy was held at 400 °C for 10 h, which could significantly reduce the volume fraction of the second Mg-Zn phase, resulting in a relatively high Zn solid solution concentration. As shown in Fig.2(a), it is clear that solution treatment also induces an obvious grain growth in ZK60 alloy. The mean grain size is almost doubled after solution treatment. Figures 2(b) and (c) show the optical microstructures of ZK60 alloy specimens subjected to solution treatment followed by aging at 150 °C for 15 h and 200 °C for 15 h, respectively. The sequential artificial aging treatment did not change much the grain size and distribution, while the precipitation of the second phase occurred inside the grains.SEM observation at higher magnification in Fig.3 clearly shows these precipitates in the specimens after artificial aging at 150 and 200 °C for different time. Most of the precipitates with a size of less than 1 µm are very fine. It is found that the distribution of the precipitates is not homogeneous in the grains, and more precipitate particles are presented in the vicinity of grain boundaries. This may be attributed to that the grain boundary region can act as effective nucleation sites for precipitating of the second phase, since the grain boundaries possess high excess energy and the impurities tend to exist in the region. The volume fraction of precipitates varies with aging time at any temperature. From Figs.3(a −c), it can be seen that aging treatment at 200 °C for 15 h leads to a higher density of precipitates compared with that aging for 5 and 20 h. For aging at 200 °C, the precipitate particles increase gradually with holding time as shown in Figs.3(d −f).CHEN Xian-hua, et al/Trans. Nonferrous Met. Soc. China 21(2011) 754−760756Fig.2 Optical images of ZK60 alloy in varying heat treatment conditions: (a) Solution treated; (b) Solution treated, and aged at 150 °C for 15 h; (c) Solution treated, and aged at 200 °C for 15 hFig.3 SEM images of alloy after solution treatment followed by different aging parameters: (a) 150 °C, 10 h; (b) 150 °C, 15 h; (c) 150 °C, 30 h; (d) 200 °C, 5 h; (e) 200 °C, 15 h; (f) 200 °C, 20 hIn order to identify the second phase precipitates in ZK60 alloy, XRD analysis was carried out on the specimens. The XRD patterns of as-cast, solution treated and solution treated plus aged at 150 °C are illustrated in Fig.4, which indicates that the precipitates of the alloys at various heat-treatment states mainly consist of MgZn2 and MgZn phases. From the intensity of diffraction peaks, it is concluded that the solution treatment gives rise to an obvious decrease in the volume fraction of the second phase, while lots of MgZn2 and MgZn phases precipitate again during the subsequent aging, especially during the aging treatment at 150 °C for 30 h. This is consistent with the result from OM and SEM observations.Typical EDS mapping of the precipitate particles in the alloy after solution treatment and aging at 150 °C for30 h is shown in Fig.5. It is noted that many precipitates Fig.4 XRD patterns of alloy in various heat treatment states: (a) solution treated, aged at 150 °C for 30 h; (b) Solution treated, aged at 150 °C for 15 h; (c) Solution treated, aged at 150 °C for 10 h; (d) Solution treated; (e) As castCHEN Xian-hua, et al/Trans. Nonferrous Met. Soc. China 21(2011) 754−760 757Fig.5 EDS mapping analysis of ZK60 alloy subjected to solution treatment and aging at 150 °C for 30 h: (a) SEM image; (b−d) Distribution of Mg, Zn and Zrare found in the Mg and Zn EDS maps, which is in agreement with the XRD analysis. However, Zr element is found in some second phase particles, which may be attributed to the following two reasons: 1) Zr-rich particles can act as the nucleation sites during Mg solidification by the peritectic reaction[14−15]; 2) Zn2Zr3 phase is presented in the grains[15]. It is possible that XRD analysis is not able to identify the Zr and Zn2Zr3 phases due to the low concentration of Zr.Figure 6 shows the aging hardening curves of ZK60 specimens at constant aging temperatures of 150 and 200 °C for different time, respectively. The specimens for aging treatment were solution treated at 400 °C for 10 h and obtained a microhardness of HV 67. The hardness increased to the peak value of HV 79 after aging at 200 °C for 15−20 h, then tended to decrease. The variation tendency of hardness value with aging time at 150 °C is different from that at 200 °C. For the specimen subjected to artificial aging at 150 °C, no peak value was found and the hardness was enhanced with the increase of holding time, when the aging time is up to 30 h, the hardness is about HV 77.According to the aging curves, uniaxial tensile testing was performed on seven ZK60 specimens in different heat-treatment states. The obtained tensile engineering stress—strain curves are shown in Fig.7. All the stress—strain curves can be divided into three regions. Region I corresponds to the elastic deformation.In region II, obvious strain hardening is present and the Fig.6 Aging hardening curves of alloy under different aging conditionsplastic deformation is uniform across the gauge length. In region III, localized necking occurs and the stress decreases gradually.A quantitative summary of the tensile results for each sample, including ultimate tensile strength (σb), yield strength (σ0.2) as well as elongation-to-failure (δ), is listed in Table 2. Table 2 shows the effect of heat treatment condition on the tensile properties of as-cast ZK60 Mg alloy. The as-cast alloy was solution treated at 400 °C for 10 h, and then artificially aged at varying temperature and time conditions. Solution treated alloys exhibit a decreased yield strength and increased tensile elongation compared with the as-cast ZK60 specimens. After subsequent aging treatment at 150 °C for 10 h, theCHEN Xian-hua, et al/Trans. Nonferrous Met. Soc. China 21(2011) 754−760758 Table 2 Tensile properties of ZK60 alloy in different heat treatment statesHeat treatment σb /MPa σ0.2 /MPaδ/% As-cast 261 128 16.3Solution treated 27310821.5Solution treated, aged at 150 °C for 10 h 274 123 19.4 Solution treated, aged at 150 °C for 15 h 277 132 19.8 Solution treated, aged at 150 °C for 30 h 285 145 21.7 Solution treated, aged at 200 °C for 5 h 266 133 13.0 Solution treated, aged at 200 °C for 15 h 280 146 17.3 Solution treated, aged at 200 °C for 20 h277 148 14.5specimen possesses higher strength and lower tensile elongation in comparison with the solution treated one. It is interesting to note that both the strength and elongation increase with increasing aging time. After aging treatment at 150 °C for 30 h, σb and σ0.2 are up to 285 and 145 MPa, respectively, which are obviously higher than those of as-cast and solution treated samples. In addition, the elongation of the specimen under this condition under 21.7%, which is comparable to that of the solution treated one and greater than that of the as-cast one. For aging treatment at 200 °C, the tensile strength and elongation reach peak values with the aging time of 15 h, and then exhibit a decrease with increasing aging time.The strength of Mg alloys is generally influenced by the contributions from intrinsic lattice, grain boundaries, solid solution, second phases and dislocations[16−17]. A possible expression of the yield strength of as-cast specimen can be approximated linearly as[6]:disp gb nss 02.0σσσσσ+++= (1)where σ0 is the intrinsic lattice resistance to basal slip; σnss is the contribution of non-uniform solid solution which is associated mostly with effects of Zn and Zr; σgb =Mk s d −1/2 is the grain boundary contribution through the Hall-Petch relation, in which d is the grain size, M isthe Taylor factor for basal ship, ()2/1crss*ps MC k τ=,p crss τ is related to more difficult prismatic slip in a single crystal for Mg based alloys and C * is a constant; σdisp =KV ε is the dispersion strengthening due to the second phase, where K is a constant, V is the volume fraction of Mg-Zn phases and ε is the applied strain.The microstructure of the solution treated specimen is more simple compared to that of the as-cast specimen. The yield strength of the solution treated specimen in that case can be expressed as[6]:Fig.7 Tensile engineering stress —strain curves of alloy in different heat treatment statesgb uss 02.0σσσσ++= (2)where σuss is the contribution of uniform solid solution.The microstructure change induced by artificial aging is mainly the precipitation of Mg-Zn phases. The yield strength of the specimens subjected to solution treatment plus aging can be written as:prec gb uss 0σσσσσ+++=y (3)where σprec =KV ε is the precipitation strengthening due to the second phase, where K is a constant, V is the volumeCHEN Xian-hua, et al/Trans. Nonferrous Met. Soc. China 21(2011) 754−760 759fraction of Mg-Zn precipitates and ε is the applied strain.Solution treatment increases the grain size greatly, which results in the fact that the contribution of σgb to the strength of solutionized specimen is significantly weaker compared to that of as-cast specimen. At the same time, the contribution of σprec to the strength is almost negligible for the solutionized specimen. Therefore, it is reasonable that the yield strength of the solution treated specimen is comparatively lower than that of the as-cast specimen.Numerous MgZn and MgZn2 precipitates form when the specimens are subjected to the treatment of solution treatment plus aging. Additionally, the grains grow quite slightly during aging treatment. The combined contribution of σgb and σprec to the yield strength of aged specimens is stronger compared to the contribution of σgb to the strength of the solutionized specimen, which is responsible for the fact that the yield strength of aged specimen is higher than that of solution treated specimen. According to the SEM observations, the volume fraction of precipitates increases with the holding time increasing for these specimens aged at 150 °C. Consequently, the yield strength is the highest when the aging time is 30 h. For the specimens aged at 200 °C, the highest volume fraction of precipitates and yield strength are obtained when the aging time is 15−20 h.After solution treatment and aging at 150 °C for 30 h, the specimen exhibits the highest tensile strength and elongation among these experimental specimens. This indicates that the precipitation of the second phase is beneficial to the enhancement of both strength and ductility, which is different from some results reported in Refs.[10−11]. It is considered that the formation of some second phase compounds containing impurities (such as Mg2Si and Mg2Ni) during aging could purify the Mg matrix, which might improve the plasticity of the alloy. Further systematic investigation is needed to explain the phenomenon.4 Conclusions1) When the as-cast ZK60 alloy is subjected to solution treatment at 400 °C for 10 h and artificial aging treatment at 150 °C for different times, the volume fraction of precipitates increases with the aging time up to 30 h.2) When the as-cast ZK60 alloy is subjected to solution treatment at 400 °C for 10 h and artificial aging treatment at 200 °C, the volume fraction of precipitates reaches the peak value with aging time of 15−20 h.3) Solution treatment at 400 °C for 10 h plus artificial aging at 150 °C for 30 h can be considered the optimum heat treatment. A good combination of tensile strength and ductility is achieved under this treatment condition.References[1]BOHLEN J, LETZIG D, KAINER K U. New perspectives forwrought magnesium alloys [J]. Materials Science Forum, 2007, 546−549: 1−10.[2]MORDIKE B L, EBERT T. Magnesium: properties—Applications—potential [J]. Materials Science and Engineering A, 2001, 302(5): 37−45.[3]KAINER K U, von BUCH F. Magnesium alloys and technologies[M]. Weinheim: WILEY-VCH Verlag GmbH, 2003: 1−3.[4]SUZUKI A, SADDOCK N D, JONES J W, POLLOCK T M.Solidification paths and eutectic intermetallic phases in Mg-Al-Ca ternary alloys [J]. Acta Materialia, 2005, 53(9): 2823−2834.[5]del V ALLE J A, CARRENO F, RUANO O A. Influence of textureand grain size on work hardening and ductility in magnesium-based alloys processed by ECAP and rolling [J]. Acta Materialia, 2006, 54:4247−4259.[6]YAKUBTSOV I A, DIAKA B J, SAGER C A, BHATTACHARYAB, MACDONALD W D, NIEWCZAS M. Effects of heat treatment on microstructure and tensile deformation of Mg AZ80 alloy at roomtemperature [J]. Materials Science and Engineering A, 2008, 496: 247−255.[7]BEER A G, BARNETT M R. Microstructure evolution in hotworked and annealed magnesium alloy AZ31 [J]. Materials Science and Engineering A, 2008, 485: 318−324.[8]BALASUBRAMANI N, PILLAI U T S, PAI B C. Optimization ofheat treatment parameters in ZA84 magnesium alloy [J]. Journal of Alloys and Compounds, 2008, 457: 118−123.[9]XU D K, LIU L, XU Y B, HAN E H. The effect of precipitates onthe mechanical properties of ZK60-Y alloy [J]. Materials Science andEngineering A, 2006, 420: 322−332.[10]CHEN H M, YU H S, KANG S B, CHO J, MIN G H. Optimizationof annealing treatment parameters in a twin roll cast and warm rolledZK60 alloy sheet [J]. Materials Science and Engineering A, 2010, 527: 1236−1242.[11]CHEN H M, KANG S B, YU H S, CHO J, KIN H W, MIN G H.Effect of heat treatment on microstructure and mechanical propertiesof twin roll cast and sequential warm rolled ZK60 alloy sheets [J].Journal of Alloys and Compounds, 2009, 476: 324−328.[12]YU Kun, LI Wen-xian, WANG Ri-chu. Effects of heat treatment onmicrostructures and mechanical properties of ZK60 magnesium alloy[J]. The Chinese Journal of Nonferrous Metals, 2007, 17(2): 188−192.(in Chinese)[13]YUAN Wu-hua, ZHANG Zhao-chun, GUO Qiang, XIA Wei-jun, JIZhe, CHEN Zhen-hua. Influence of solution treatment on structures and properties of ZK60 alloy [J]. Heat Working Technology, 2006, 35(2): 22−24. (in Chinese)[14]ROBERTS C S. Magnesium and its alloys [M]. New York: Wiley &Sons, 1960: 206−207.[15]SHAHZAD M, WAGNER L. Microstructure development duringextrusion in a wrought Mg-Zn-Z0r alloy [J]. Scripta Materialia, 2009,60: 536−538.[16]MEYERS M A, CHAWLA K K. Mechanical behavior of materials[M]. New Jersey: Prentice Hall, Inc, 1999: 375.[17]COURTNEY T H. Mechanical behavior of materials [M]. Michigan:McGraw-Hill Companies, Inc, 2000: 181−182.CHEN Xian-hua, et al/Trans. Nonferrous Met. Soc. China 21(2011) 754−760760热处理对ZK60镁合金组织与力学性能的影响陈先华1, 2, 黄小旺1, 潘复生1, 2, 汤爱涛1, 2, 王敬丰1, 2, 张丁非1, 21. 重庆大学材料科学与工程学院,重庆 400044;2. 重庆大学国家镁合金材料工程技术研究中心,重庆 400044摘 要:研究固溶和时效热处理工艺对铸态ZK60镁合金显微组织与力学性能的影响。
- 1、下载文档前请自行甄别文档内容的完整性,平台不提供额外的编辑、内容补充、找答案等附加服务。
- 2、"仅部分预览"的文档,不可在线预览部分如存在完整性等问题,可反馈申请退款(可完整预览的文档不适用该条件!)。
- 3、如文档侵犯您的权益,请联系客服反馈,我们会尽快为您处理(人工客服工作时间:9:00-18:30)。
收稿日期:2003-12-12
作者简介:郝艳君(1979-),女,辽宁葫芦岛人,助教.
文章编号:1000-1646(2004)03-0261-04
挤压态ZK60镁合金的高温力学性能及其超塑性
郝艳君1,陈立佳2,吴 伟2(11沈阳工业大学信息科学与工程学院,辽宁沈阳110023;21沈阳工业大学材料科学与工程学院,辽宁沈阳110023)
摘 要:针对挤压态ZK60镁合金的高温力学性能及其超塑性行为进行了研究.结果表明,挤压态ZK60镁合金的高温力学性能与试验温度、应变速率密切相关.通常,屈服强度和抗拉强度随试验温度的降低和应变速率的增加而提高,而延伸率则随试验温度的升高和应变速率的降低而增大.
塑性变形流变应力与温度的倒数之间呈线性关系,而且在应变速率为5×10
-4
/s下的激活能为
9314kJ/mol.关 键 词:镁合金;超塑性;高温力学性能中图分类号:TG113125 文献标识码:A
镁合金作为结构材料得到了越来越广泛的重视,并将在今后发挥越来越重要的作用.这是由于镁合金具有一些优良性能,如密度小、具有抗震性,易于机械加工等[1~4].而镁合金的常温塑性变形能力较差,并常常用于精加工,所以研究其高温力学性能具有较大意义.本文研究了挤压态的镁合金的高温性能,其中主要对其超塑性进行研究.通过对其高温力学性能的研究,并对其在不同条件下所得数据进行对比分析,从而得到挤压态镁合金超塑变形特征及其影响因素.1 试验材料及试验方法111 试验材料本试验所用材料是ZK60镁合金,其中Zn含量为6%,Zr含量≤017%,其余为Mg及Si、Cu等杂质.112 试样制备将ZK60镁合金进行热挤压,具体工艺流程是:挤压锭→去皮→预热→热挤压→冷却→矫直→截成规定尺寸.挤压比是35∶1.挤压态ZK60镁合金的拉伸试样用线切割机床切取,试样的标距长度为5mm.为了减少应力集中、降低缺口敏感性,需将试样上的切割痕迹用砂纸打磨掉.113 试验方法拉伸试验温度范围为200~300℃,应变速率范围为(1~10)×10-4/s.试样在电阻炉中进行加热,加热到预定温度后保温10~20min,再进行拉伸试验,记录高温拉伸试验期间的工程应力及应变数据,据此确定试样在不同拉伸实验条件下的抗拉强度和屈服强度;量取断裂试样的最终长度,
计算出延伸率.
2 试验结果及分析211 挤压态ZK60镁合金的高温塑性图1为挤压态ZK60镁合金在300℃温度下进行拉伸试验后所测得的延伸率与应变速率的关系曲线.由图可知,当应变速率为1×10
-4
/s时,
挤压态ZK60镁合金延伸率达到195%左右;而在(1×10-4~1×10
-3)/s的应变速率范围内,延
伸率变化不大;其后,随初始应变速率的进一步增加,延伸率降低.
图2为挤压态ZK60镁合金在5×10
-4
/s的
应变速率下拉伸试验后所测得的延伸率与温度之间的关系曲线.由图可知,随着试验温度由300℃降低至250℃时,延伸率由195%左右降低至12312%,当试验温度由250℃降至200℃时,挤压态ZK60镁合金的延伸率也有所降低,但降低幅度不大.
212 挤压态ZK60合金的强度图3为300℃的试验温度下对挤压态ZK60
镁合金进行拉伸试验时所获得的抗拉强度—初始应变速率曲线.由图中可以看出,同一试验温度
第26卷第3期2004年6月沈 阳 工 业 大 学 学 报JournalofShenyangUniversityofTechnologyVol126No13Jun.2004下,合金的抗拉强度随应变速率的增加而提高. 图4为300℃温度下进行拉伸试验时挤压态ZK60镁合金的屈服强度—应变速率曲线.从中可以观察到,同一试验温度下,合金的屈服强度随应变速率的增加而增加,而且表现出与抗拉强度随应变速率的变化相同的趋势.
图5所示为5×10
-4
/s的应变速率下对挤压
态ZK60合金进行拉伸试验时所获得的抗拉强度与试验温度之间的关系曲线.可以观察到,随着温度的提高,合金的抗拉强度明显减小,但减少幅度成减缓趋势.
图6为挤压态ZK60合金在5×10
-4
/s的应
变速率下拉伸时所获得的屈服强度与温度之间的关系曲线.可以看出,随着试验温度的提高,屈服强度明显的减小,但减少幅度也呈减缓趋势.
262 沈 阳 工 业 大 学 学 报第26卷213 超塑性变形特征图7所示为挤压态ZK60镁合金在300℃下的流变应力与应变速率间的关系曲线.其中.取ε=10%时的真应力作为流变应力.可以看出,logσ与logε之间呈现双线性关系.通常将logσ2logε曲线上每一点的斜率定义为应变速率敏感系数m即m=d(logσ)d(logε)(1)式中 m表示合金在变形过程中能够抑制颈缩的能力大小.m值愈大,表示合金越有可能获得较好的超塑性. 对图7中的数据进行线性回归,可以确定出,
当试验温度为300℃时,在(1~50)×10
-4
/s的
应变速率范围,相应的m值为01307.由此可知,
挤压态ZK60镁合金在较低的应变速率下可以实现超塑性变形.
图8为挤压态ZK60镁合金在应变速率为5×10-4/s时的流变应力与温度之间的关系曲线.可以看出,流变应力的对数与温度的倒数之间也呈很好的线性关系.
文献[5]提出了应变速率、流变应力与试验温度之间的定量表达式ε=C1σnexp(-Q/RT)(2)式中 C1,R为常数;Q为激活能;n为应力指数;σ为流变应力;ε为应变速率.根据这一公式,可以推导出如下形式lnσ=Q/nRT(3)显然,通过求出曲线斜率k,即可求得Q值Q=nRk(4) 对于本试验条件,可求得激活能Q的值为9314kJ/mol.3 结 论通过研究挤压态ZK60镁合金的高温力学性能及超塑性变形特征,可以得出以下结论:1)挤压态ZK60镁合金在试验温度为300℃、较高的应变速率下,合金的断裂延伸率可以达到190%左右.并且在相同的初始应变速率下,随着试验温度的升高,延伸率将有所增大.2)挤压态ZK60镁合金的高温抗拉强度与屈服强度均表现出较强的应变速率及温度依赖性.通常,随着试验温度的降低和应变速率的增加,合金的强度增加.
3)挤压态ZK60镁合金在300℃试验温度下的logσ2logε曲线呈双线性关系,相应的应变速率敏感系数为01307.
4)挤压态ZK60镁合金的流变应力与温度倒数之间呈线性关系,据此可计算出在应变速率为5×10
-4
/s时相应的激活能为9314kJ/mol.
参考文献:
[1]WinandyCD.Magnesiumdie2castinginmotorvehicles[J].Magnesium2theLightweightSolution,1998(5):8-9.[2]陈昌奇.轻合金[M].北京:国防工业出版社,1985.(ChenCQ.Lightalloys[M].Beijing:NationalDefense
IndustrialPress,1985.)[3]RudenTJ,AlbrightDL.Highductilitymagnesiumal2loysinautomotiveapplication[J].AdvancedMater&Process,1999,145(6):28-32.[4]BeckA.Thetechnologyofmagnesiumanditsalloys[M].London:F.A.HughesCo.Limited,1940.[5]JonasJJ,SellarsCM,TegartWJM.Strengthandstructureunderhot2workingconditions[J].Metallurgical
362第3期郝艳君等:挤压态ZK60镁合金的高温力学性能及其超塑性 Review,1969,130(14):1-24.Superplasticityandhigh2temperaturemechamicalpropertiesofas2extrudedZK60alloys
HAOYan2jun1,CHENLi2jia2,WUWei2(11SchoolofInformationScienceandEngineering,ShenyangUniversityofTechnrvology,Shenyang110023,China;21
SchoolofMaterialScienceandEngineering,ShenyengUnversityofTechnology,Shanyang,110023,China)
Abstract:Thesuperplasticityandhigh2temperaturemechanicalpropertiesofas2extrudedZK60alloysareinvestigated.Theresultsshowsthatthehigh2temperaturemechanicalpropertiesofas2extrudedZK60alloysareassociatedwithtestingtemperatureandstrainrates.Itisevidentfromtheexperimentsthatthe0.2%offsetyieldstrengthandultimatetensilestrengthofthealloyareincreaseswithdecreasingtemperatureandincreasingstrainrate.Buttheelongationtofailureincreaseswhenthetemperatureincreasedandthestrainratedecreased.Theflowingstressofas2extrudedZK60alloysduringplasticdeformationisproportionedtothereciprocaloftemperature.Attheinitialstrain2rateof5×10-4/s,thecalculatedactiveenergyat300℃is9314kJ/mol.
Keywords:magnesiumalloys;superplasticity;high2temperaturemechanicalproperties
(上接第242页)[2]Lozano2PerezT.Asimplemotion2planningalgorithmforgeneralrobotmanipulatiors[J].IEEEJofRoboticsandAutomation,1987,RA23(3):156.[3]刘成良.RV12L6R焊接机器人运动学及计算机仿真系统研究[J].机器人,1998(9):3332334.(LiuCL.StudyonkinematicsandcomputersimulationofRV12L6Rweldingrobot[J].Robot,1998(9):3332334.)[4]王伟,杨杨.机器人C2空间障碍边界建模与无碰撞路径规划[J].机器人,1998(7):282.(WangW,YangY.RobotC2Obstaclebounarymodelingandcollision2freepathplanning[J].1998(7);282.)[5]熊有伦.机器人学[M].北京机械业出版社,1993.(XiongYL.Robotics[M].Beijing:ChinaMachine