湿法制粒工艺放大与终点判断
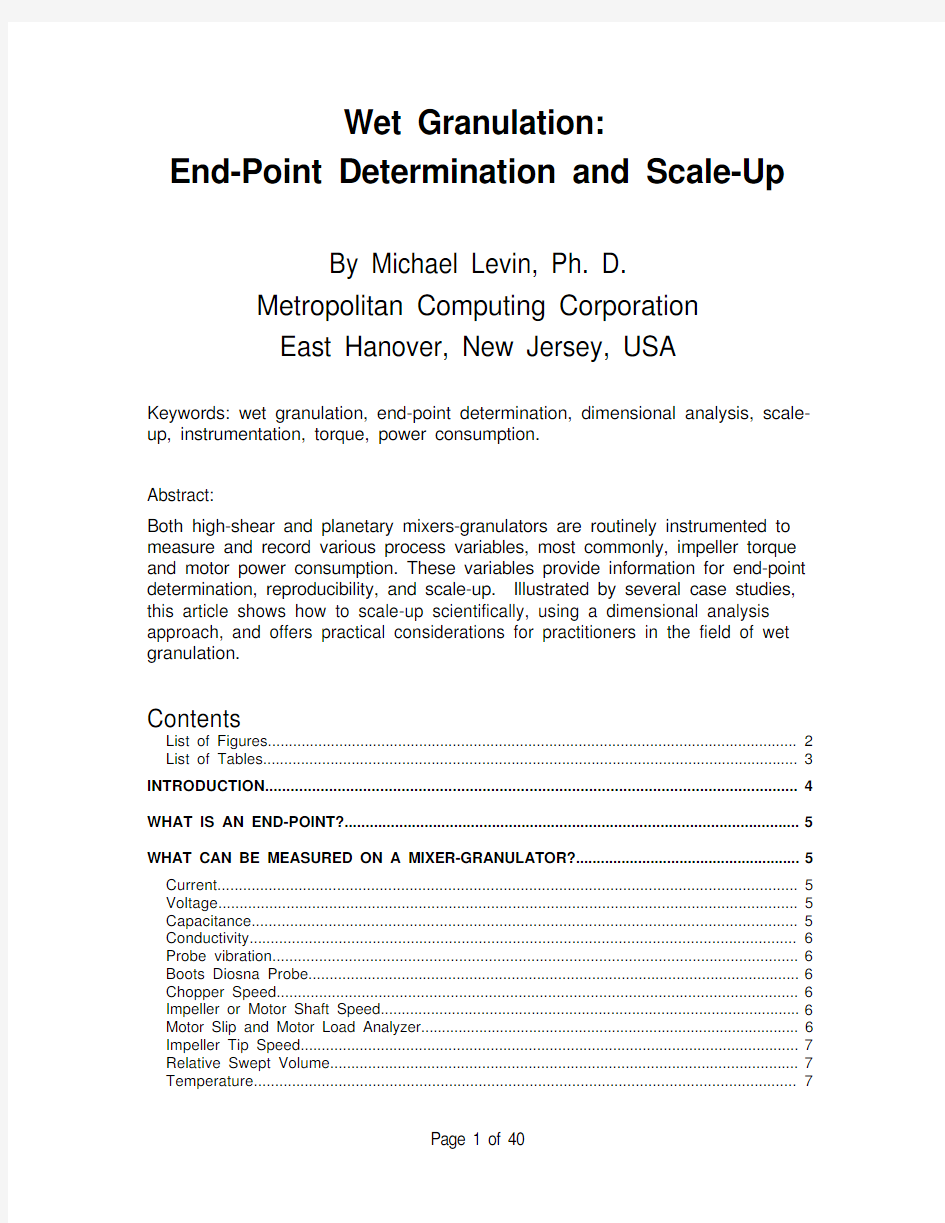
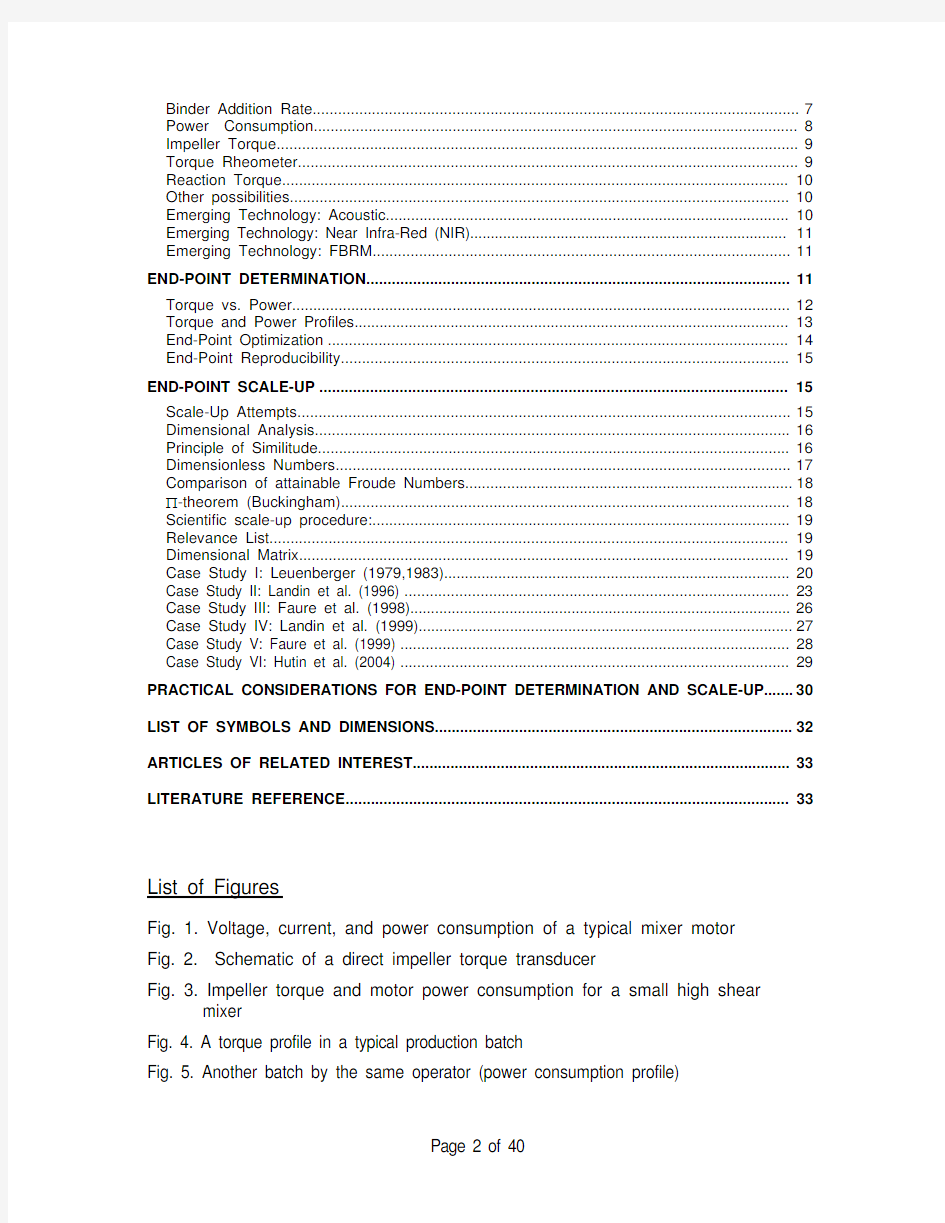
Wet Granulation:
End-Point Determination and Scale-Up
By Michael Levin, Ph. D.
Metropolitan Computing Corporation
East Hanover, New Jersey, USA
Keywords: wet granulation, end-point determination, dimensional analysis, scale-up, instrumentation, torque, power consumption.
Abstract:
Both high-shear and planetary mixers-granulators are routinely instrumented to measure and record various process variables, most commonly, impeller torque and motor power consumption. These variables provide information for end-point determination, reproducibility, and scale-up. Illustrated by several case studies, this article shows how to scale-up scientifically, using a dimensional analysis approach, and offers practical considerations for practitioners in the field of wet granulation.
Contents
List of Figures (2)
List of Tables (3)
INTRODUCTION (4)
WHAT IS AN END-POINT? (5)
WHAT CAN BE MEASURED ON A MIXER-GRANULATOR? (5)
Current (5)
Voltage (5)
Capacitance (5)
Conductivity (6)
Probe vibration (6)
Boots Diosna Probe (6)
Chopper Speed (6)
Impeller or Motor Shaft Speed (6)
Motor Slip and Motor Load Analyzer (6)
Impeller Tip Speed (7)
Relative Swept Volume (7)
Temperature (7)
Binder Addition Rate (7)
Power Consumption (8)
Impeller Torque (9)
Torque Rheometer (9)
Reaction Torque (10)
Other possibilities (10)
Emerging Technology: Acoustic (10)
Emerging Technology: Near Infra-Red (NIR) (11)
Emerging Technology: FBRM (11)
END-POINT DETERMINATION (11)
Torque vs. Power (12)
Torque and Power Profiles (13)
End-Point Optimization (14)
End-Point Reproducibility (15)
END-POINT SCALE-UP (15)
Scale-Up Attempts (15)
Dimensional Analysis (16)
Principle of Similitude (16)
Dimensionless Numbers (17)
Comparison of attainable Froude Numbers (18)
Π-theorem (Buckingham) (18)
Scientific scale-up procedure: (19)
Relevance List (19)
Dimensional Matrix (19)
Case Study I: Leuenberger (1979,1983) (20)
Case Study II: Landin et al. (1996) (23)
Case Study III: Faure et al. (1998) (26)
Case Study IV: Landin et al. (1999) (27)
Case Study V: Faure et al. (1999) (28)
Case Study VI: Hutin et al. (2004) (29)
PRACTICAL CONSIDERATIONS FOR END-POINT DETERMINATION AND SCALE-UP (30)
LIST OF SYMBOLS AND DIMENSIONS (32)
ARTICLES OF RELATED INTEREST (33)
LITERATURE REFERENCE (33)
List of Figures
Fig. 1. Voltage, current, and power consumption of a typical mixer motor
Fig. 2. Schematic of a direct impeller torque transducer
Fig. 3. Impeller torque and motor power consumption for a small high shear mixer
Fig. 4. A torque profile in a typical production batch
Fig. 5. Another batch by the same operator (power consumption profile)
Fig. 6. A batch by a novice operator (power consumption profile)
Fig. 7. Another batch by inexperienced operator (torque profile)
Fig. 8. Wet granulation end-point as a factor in tableting optimization
Fig. 9. The range of Froude numbers for Collette Gral high-shear mixers.
Fig. 10. The range of Froude numbers for Fielder PMA high-shear mixers.
Fig. 11. Newton Power Number as a function of the Specific Amount of Graqnulating Liquid (adapted from , Leuenberger and Sucker, 1979). Fig. 12. Regression lines of the Newton Power Number on the product of Reynolds number, Froude Number, and the length ratio for 3 different Fielder mixers
(from Landin et al., 1996).
Fig. 13. Regression graph of Case Study III.
Fig. 14. Regression graph of Case Study IV.
Fig. 15. Regression graph of Case Study V.
List of Tables
Table I. The Relevance List used by Leuenberger (1983)
Table II. The Dimensional Matrix for Case Study I
Table III. The Transformed Dimensional Matrix for Case Study I
Table IV: Dimensionless Π groups formed from the matrix in Table III
Table V. Relevance List for Case Study II (Landin et al., 1996)
Table VI. The Dimensional Matrix for Case Study II
Table VII. The Transformed Dimensional Matrix for Case Study II
Table VIII: Dimensionless Π groups formed from the matrix in Table VII
Table IX. Relevance list for Hutin et al. (2004)
Introduction
Wet granulation is used mainly to improve flow and compressibility of powders, and to prevent segregation of the blend components. Particle size of the granulate is affected by the quantity and feeding rate of granulating liquid.
Wet massing in a high-shear mixing is frequently compared to fluid bed mixing and to roller compaction technique (1), and the results seem to be formulation dependent. Compared to high shear granulation, low shear or fluid bed process requires less fluid binder, resulting in a shorter drying time, but also in a less cohesive material (see, for example, 2, 3, or 4).
For excellent classical review of the wet granulation process, equipment and variables, and measurement instruments available in the field, see papers by P. Holm and his group (5-12). These papers have become a standard reference for numerous subsequent publications.
Due to rapid densification and agglomeration that are caused by the shearing and compressing action of the impeller in a high-shear single pot system, mixing, granulation and wet massing can be done relatively quickly and efficiently. The dangers lie in a possibility of overgranulation due to excessive wetting and producing low porosity granules thus affecting the mechanical properties of the tablets. As the liquid bridges between the particles are formed, granules are subjected to coalescence alongside with some breakage of the bonds.
It stands to reason that mean granule size is strongly dependent on the specific surface area of the excipients, as well as the moisture content and liquid saturation of the agglomerate. During the wet massing stage, granules may increase in size to a certain degree while the intragranular porosity goes down. However, some heating and evaporation may also take place leading to a subsequent decrease in the mean granule size, especially in small scale mixers. Load on the main impeller is indicative of granule apparent viscosity and wet mass consistency. It can be seen as an interplay of acceleration (direct impact of the impeller), centrifugal, centripetal, and friction forces that act on the particles. According to Cliff (13-14), binder addition rate controls granule density, while impeller and chopper speed control granule size and granulation rate. The end-point controls the mix consistency and reproducibility. Other factors that affect the granule quality include spray position and spray nozzle type, and, of course, the product composition. Such variables as mixing time and bowl or product temperature are not independent factors in the process but rather are responses of the primary factors listed above.
What is an end-point?
End-point can be defined by the formulator as a target particle size mean or distribution. Alternatively, the end-point can be defined in rheological terms. It has been shown (15) that once you have reached the desired end-point, the granule properties and the subsequent tablet properties are very similar regardless of the granulation processing factors, such as impeller or chopper speed or binder addition rate. I would call this “the principle of equifinality”.
The ultimate goal of any measurement in a granulation process is to estimate viscosity and density of the granules, and, perhaps, to obtain an indication of the particle size mean and distribution. One of the ways to obtain this information is by measuring load on the main impeller.
Mixer instrumentation, in general, has numerous benefits. In addition to a possible end-point determination, it can be used to troubleshoot the machine performance (for example, help detect worn-out gears and pulleys or identify mixing and binder irregularities). Instrumentation can serve as a tool for formulation fingerprinting, assure batch reproducibility, aid in raw material evaluation, process optimization and scale-up.
What Can Be Measured on a Mixer-Granulator?
Current
Current in DC motors can be used as some indication of the load on the main impeller because impeller torque is proportional to current in some intervals (13) and therefore a current meter (ammeter) can be used for small scale direct current (DC) motors. However, for alternating current (AC) motors (most often used in modern mixers), there may be no significant change in current as motor load varies up to 50% of full scale. At larger loads, current draw may increase but this increase is not linearly related to load, and, consequently, current is completely ineffective as a measurement of load. Moreover, current baseline may shift with time.
Voltage
Voltage measurement generally has no relation to load.
Capacitance
Capacitive sensor responds to moisture distribution and granule formation (16-20). It provided similar end-points (based on the total voltage change) under varying rates of agitation and liquid addition. Capacitive sensor can be threaded into an existing thermocouple port for in-process monitoring.
Conductivity
Conductivity of the damp mass (21) makes it possible to quantify uniformity of liquid distribution and packing density during wet massing time.
Probe vibration
Probe vibration analysis (22-23) require a specially constructed probe that includes a target plate attached to an accelerometer (for in-process monitoring). This measurement is based on the theory that increasing granule size results in the increase of the acceleration of agglomerates striking the probe target. The method has a potential for granulation monitoring and end-point control.
Boots Diosna Probe
This probe (23) measured densification and increase of size of granules (changes in momentum of granules moving with constant velocity due to a mass change of the granules). The method did not gain popularity because of its invasive nature.
Chopper Speed
Chopper speed has no significant effect on the mean granule size (5,6). Impeller or Motor Shaft Speed
Rate of impeller rotation could be used as some indication of the work being done on the material (24). Since the motor or impeller power consumption is proportional to the product of torque and speed, the latter is an important factor in evaluating the corresponding load.
Motor Slip and Motor Load Analyzer
Motor slip is the difference between rotational speed of an idle motor and motor under load (25-26). Motor slip measurements, although relatively inexpensive, do not offer advantages over the power consumption measurements. The method did not gain popularity, probably because the slip is not linearly related to load (27) despite some claims to the contrary.
Impeller Tip Speed
Impeller tip speed corresponds to shear rate and has been used as a scale-up parameter in fluid mixing (28). For processing of lactose granulations in Gral mixers, however, it was shown by Horsthuis et al. (29) that the same tip speed did not result in the same end-point (in terms of particle size distribution). These findings were contradicted by other studies with Fielder mixers indicating that for a constant tip speed successful scale-up is possible when liquid volume is proportional to the batch size and wet massing time is related to the ratio of impeller speeds (30).
Relative Swept Volume
Relative swept volume, that is, the volume swept by the impeller (and chopper) per unit time, divided by the mixer volume, has been suggested as a scale-up factor (11, 12, 31). This parameter is related to work done on the material and was studied extensively at various blade angles (32). Higher swept volume leads to higher temperature and denser granules. However, it was shown by Horsthuis et al. (29) that the same relative swept volume did not result in the same end-point (in terms of particle size distribution).
Temperature
Product and jacket temperature are usually measured by thermocouples. These response variables are controlled by a variety of factors, notably, the speed of the main impeller and the rate of binder addition.
Binder Addition Rate
There are conflicting reports on the preferred method of adding the binder. For example, Holm (33) does not generally recommend adding dry binder to the mix (as commonly done in order to avoid preparation of a binder solution) because a homogeneity of binder distribution can not be assured. Others recommend just the opposite (34-36).
Slow continuous addition of water (in case the water-soluble binder is dry mixed) or a binder solution to the mix is a granulation method of choice (5, 6, 10-12, 37-40 and many others). The granulating fluid should be added at a slow rate to avoid local overwetting (34).
If the binder solution is added continuously, then the method of addition (pneumatic or binary nozzle, atomization by pressure nozzle) should be considered in any end-point determination and scale-up.
An alternative to a continuous binder liquid addition method is to add binder liquid all at once (29) to assure ease of processing and reproducibility, reduce processing time and to avoid wet mass densification that may occur during the liquid addition. This
latter phenomenon may obscure the scale-up effect of any parameter under investigation.
Power Consumption
One of the most popular and relatively inexpensive measurements is the power consumption of the main mixer motor. It is measured by a watt transducer or a power cell utilizing Hall effect (a measurable transversive voltage between the two radial sides of a current conductor in a magnetic field, an effect discovered by E.H. Hall in 1879).
Power consumption of the mixer motor for end-point determination and scale-up is widely used [Leuenberger (40) and subsequent work, Holm (5) and subsequent work; Landin et al. (41-44); Faure et al., (46-49); and many others (16, 17, 20, 34, 36-40, 50-51)] because the measurement is economical, does not require extensive mixer modifications and is well correlated with granule growth.
Power consumption correlates with mean granule size of a granulation (8), although the correlation is not always linear in the entire range. Intragranular porosity also shows some correlation with power consumption (52). Normalized work of granulation (power profile integrated over time) can accurately determine end-points and is correlated well with properties granulates (53).
The main problem with power consumption measurements is that this variable reflects load on the motor rather than load on the impeller. It relates to the overall mixer performance, depends on motor efficiency and can change with time regardless of the load.
Fig. 1. Voltage, current, and power consumption of a typical mixer motor Motor power consumption is a product of current, voltage, and the so-called power factor. In the range of interest, motor power consumption is generally proportional to load on the motor and, to some degree, can reflect the load on the impeller (Fig.1).
However, up to 30% of the power consumption of a motor can be attributed to
no-load losses due to windage (by cooling fan and air drag), friction in bearings, and core losses that comprise hysteresis and eddy current losses in the motor
magnetic circuit. Load losses include stator and rotor losses (resistance of materials used in the stator, rotor bars, magnetic steel circuit) and stray load losses such as current losses in the windings (54).
Attempts to use a no-load (empty bowl, or dry mix) values as a baseline may be confounded by a possible nonlinearity of friction losses with respect to load (55). As the load increases, so does the current draw of the motor. This results in heat generation that further impacts the power consumption (27). A simple test might be to run an empty mixer for several hours and see if there is any the shift in the baseline. Also, as the motor efficiency drops with age, the baseline most definitely shifts over time.
Motor power consumption is non-linearly related to the power transmitted to the shaft (56) and the degree of this non-linearity could only be “guestimated”. Impeller Torque
In a mixing process, changes in torque on the blades and power consumption of the impeller occur as a result of change in the cohesive force or the tensile strength of the agglomerates in the moistened powder bed.
Fig. 2. Schematic of a direct impeller torque transducer
Direct torque measurement requires installation of strain gages on the impeller shaft or on the coupling between the motor and impeller shaft (Fig.2). Since the shaft is rotating, a device called slip ring is used to transmit the signal to the stationary data acquisition system.
Planetary mixer instrumentation for direct torque measurement does not substantially differ from that of a high shear mixer. Engineering design should only take into account the planetary motion in addition to shaft rotation (57). Impeller torque is an excellent in-line measure of the load on the main impeller (52, 58).
Torque Rheometer
A torque rheometer is a device that provides an off-line measurement of torque required to rotate the blades of the device and this torque can be used to assess rheological properties of the granulation. It has been extensively used for end-point determination (41, 59-61). The torque values thus obtained were termed a “measure of wet mass consistency” (46, 47, 62).
One of the main concerns is that using the torque value that the unit is reporting instead of the dynamic viscosity for calculation of Reynolds numbers renders the latter to become dimensional. Therefore, the Reynolds number calculated from torque rheometer data is referred to as “pseudo-Reynolds” dimensional number. Due to the fact that torque was shown to be proportional to a kinematic (rather than dynamic) viscosity (63), it can have a conditional use in the dimensional analysis of the process, as will be shown below.
Reaction Torque
By the third law of Newton, for every force there is a counter-force, collinear, equal and opposite in direction. As the impeller shaft rotates, the motor tries to rotate in the opposite direction, but it does not because it is bolted in place. The tensions in the stationary motor base can be measured by a reaction torque transducer.
Reaction torque is a less expensive alternative to direct impeller torque and is recommended for mixers that have the motor and impeller shafts axially aligned (in this case, the reaction torque is equal to direct torque and is opposite in sign). Other possibilities
When the agglomeration process is progressing very rapidly, neither power consumption nor torque on the impeller may be sensitive enough to adequately reflect changes in the material. Some investigators feel that other measurements, such as torque or force on the impeller blades may be better suited to monitor such events.
There are other ideas floating around, for example, use of neural network to describe and predict the behavior of the wet granulation (64) or control the end-point by rapid image processing system (65).
A technique for measuring tensile strength of granules, in addition to power consumption measurement, to facilitate optimal end-point determination was recently described by Betz, Bürgin and Leuenberger (50).
Powder flow patterns in wet granulation can be studied using positron emission particle tracking (66). Eventually, this and similar techniques can be used to validate various mathematical and statistical models of the process. Emerging Technology: Acoustic
Applicability of piezo-electric acoustic emission sensors to end-point determination have been studied since the beginning of this century (67). The technique is very promising, especially since it is non-invasive, sensitive and relatively inexpensive. Granulation process signatures obtained with acoustic transducer can be used to monitor changes in particle size, flow and compression properties (68, 69).
Emerging Technology: Near Infra-Red (NIR)
Use of a refractive NIR moisture sensor for end-point determination of wet granulation was described by several authors (70, 71). There are technological challenges associated with this approach, as the sensor can only measure the amount of water at the powder surface.
Near infra-red monitoring of granulation process was attempted by researchers at many major pharmaceutical corporations with a modest success. In particular, yet unpublished work by David Rudd of GlaxoSmithKline in England should be mentioned as a part of the global effort in the field of Process Analytical Technology (PAT).
Emerging Technology: FBRM
Focused Beam Reflectance Measurement (FBRM) is a particle size determination technique based on a laser beam focusing in the vicinity of a sapphire window of a probe. The beam follows a circular path at speeds of up to 6 m/s. When it intersects with the edge of a particle passing by a window surface, an optical collector records a backscatter signal. The time interval of the signal multiplied by the beam speed represents a chord length between two points on the edge of a particle. The chord length distribution (CLD) can be recalculated to represent either a number or volume weighted particle size distribution.
in many cases, where precision is more important than accuracy, CLD measurements are adequate to monitor dynamic changes in process parameters related to particle size and shape, concentration, and rheology of fluid suspensions
Several attempts were made to evaluate the use of FBRM particle size analyzer as a potential tool for granulation end-point determination (72). Dilworth et al. (73) have compared power consumption, FBRM and acoustic signals in a study of a wet granulation process in Fielder PMA 200 mixer. It was found that these techniques were complimentary, with FBRM probe capable to follow median granule size growth even when the power consumption curve showed a plateau.
A major disadvantage of the FBRM method is that measured CLD does not directly represent a particle size distribution (PSD). Conversion of CLD to PSD
is not straightforward and requires sophisticated mathematical software that is not easy to validate. Moreover, CLD depends on optical properties and shape of the particles, as well as the focal point position. The total number of counts measured is a function both of solids concentration and probe location.
End-Point Determination
End-point detection in wet granulation has become a major scientific and technological challenge (74). Monitoring granulation is most commonly achieved by collecting either power or torque signals, or both. In what follows, we will compare both methods.
Torque vs. Power
When we say “power consumption”, we usually refer to the main motor. It reflects the load on the motor due to useful work, as well as the power needed to run the motor itself (losses due to eddy currents, friction in couplings, etc.).
It is quite possible (and, indeed, quite pertinent) to talk about the power consumption of the impeller, which is, obviously, quantitatively less than the power consumption of the motor and relates directly to the load on the impeller.
Power ~ Torque * Speed
Impeller power consumption can be calculated as a product of the direct torque, rotational impeller speed, and a coefficient (usually equal to 2π times a unit conversion factor, if required).
The power consumption of the mixer motor differs from that of the impeller by the variable amount of power draw imposed by various sources (mixer condition, transmission, gears, couplings, motor condition, etc.)
Compared to impeller torque, motor power consumption is easier to measure; watt meters are inexpensive and can be installed with almost no downtime. However, motor power signal may not be sensitive enough for specific products or processing conditions. Wear and tear of mixer and motor may cause power fluctuations. Moreover, power baseline may shift with load.
Impeller torque, on the other hand, is closer to where the action is, and is directly related to the load on the impeller. Torque is not affected by mixer condition. Although the motor power consumption is strongly correlated with the torque on the impeller (38), it is less sensitive to high frequency oscillations caused by direct impact of particles on the blades as evidenced by FFT technique (16).
Power consumption or torque fluctuations are influenced by granule properties (particle size distribution, shape index, apparent density) and the granulation time. Fluctuation of torque / power consumption and intensity of spectrum obtained by FFT analysis can be used for end-point determination (37).
It was observed that when the end-point region of a granulation is reached, the frequency distribution of a power consumption signal reaches a steady state (75). It should be repeated here that torque shows more sensitivity to high frequency oscillations.
Torque and Power Profiles
Fig. 3. Impeller torque and motor power consumption for a small high shear
mixer (Fielder PMA 10).
Fig. 3 illustrates the classical power and torque profiles that start with a dry mixing stage, rise steeply with binder solution addition, level off into a plateau, and then exhibit overgranulation stage. The power and torque signals have similar shape and are strongly correlated. The pattern shows a plateau region where power consumption or torque is relatively stable.
The peak of the derivative indicates the inflection point of the signal. Based on the theory by Leuenberger (1979 and subsequent work), useable granulates can be obtained in the region that starts from the peak of the signal derivative with respect to time and extends well into the plateau area (40). Prior to the inflection point, a continuous binder solution addition may require variable quantities of liquid. After that point, the process is well defined and the amount of binder solution required to reach a desired end-point may be more or less constant.
Torque or power consumption pattern of a mixer is a function of the viscosity of both the granulate and binder. With the increasing viscosity, the plateau is shortened and sometimes vanishes completely thereby increasing the need to stop the mixer at the exact end-point.
At low impeller speeds or high liquid addition rates, the classic S-shape of the power consumption curve may become distorted with a steep rise leading into overgranulation (9).
The area under the torque-time curve is related to the energy of mixing and can be used as an end-point parameter. Area under power consumption curve divided by the load gives the specific energy consumed by the granulation process. This quantity is well correlated with the relative swept volume (11, 12, 32).
The consumed energy is completely converted into heat of the wet mass (7), so that the temperature rise during mixing shows some correlation with relative swept volume and Froude number (29) that relates the inertial stress to the gravitational force per unit area acting on the material.
Fig. 4. A torque profile in a typical production batch
Fig. 4 represents a record of a typical granulation batch done by an experienced operator on large Hobart mixer. You can see that the batch was stopped on the downslope of the derivative.
Fig. 5. Another batch by the same operator (power consumption profile)
On a Fig. 5 you can see another batch made by the same operator. This time it is a power consumption trace, but again it extends beyond the peak of the derivative and the end-point thus can be deemed reproducible.
Fig.6. A batch by a novice operator (power consumption profile)
In the batch represented in Fig. 6, a novice operator trainee has stopped the batch well before the peak of the derivative. This required a major adjustment of the tableting operation (force and speed) to produce tablets in an acceptable range of material properties (hardness and friability).
Fig. 7. Another batch by inexperienced operator (torque profile)
In this batch (Fig. 7), the same novice operator has stopped the granulation process, opened the lid, took a sample, and decided to granulate for another 10 seconds. You can see that there is no indication that the peak of the derivative was reached at the end-point.
Thus, it seems that monitoring torque or power can fingerprint not only the product, but the process and the operators as well.
A number of publications relate to practical experience of operators on the production floor (34, 76-78).
End-Point Optimization
Fig. 8. Wet granulation end-point as a factor in tableting optimization Agglomeration of particles in wet granulation have been studied extensively (24, 79). The optimal end-point can be thought of as the factor affecting a number of agglomerate properties (Fig.8).
With so many variables involved in a granulation process, it is no wonder that more and more researchers throw in a number of factors together in an attempt to arrive at an optimum response (30, 35, 80-88).
The final goal of any granulation process is a solid dosage form, such as tablets. Therefore, when optimizing a granulation process, the list of factors affecting tablet properties may include both the granulation end-point and the tableting processing parameters, such as compression force or tablet press speed.
In one of the most interesting works based on the experimental design approach, an attempt was made to find a statistical relationship between the major factors affecting both granulation and compaction, namely, granulation end-point, press speed (dwell time), and compression force (88). The resulting equation allowed optimization of such standard response parameters as tablet hardness, friability and disintegration time. This study has also investigated the possibility of adjusting the tableting parameters in order to account for an inherent variability of a wet granulation process.
Multivariate optimization of wet granulation may include hardness, disintegration and ejection as response variables (89). Compressibility property of granulations is extremely sensitive to various processing parameters of wet granulation (90). Recently, the experimental design procedure was applied to low shear wet granulation (91) with a factorial design used to evaluate the influence of such factors as binder strength and agitator speed.
End-Point Reproducibility
As will be shown in the next section, for every blend and a fixed set of values for processing factors (such as mixer geometry, blade speed, powder volume, amount and method of addition of granulating liquid), a wet granulation process state (end-point) is completely characterized by rheological properties of the wet mass (density, viscosity), which are, in turn, a function of particle size, shape and other properties. The process can be quantified with the help of dimensionless Newton Power Number N p that will assume a certain numerical value for every state (condition) of the granulate. Under fixed processing conditions, N p will be proportional to Net Power Consumption ΔP for any end-point (defined, in part, by wet mass density). Thus, in order to reproduce an end-point, it is sometimes sufficient to monitor power of the impeller (or the motor) and stop when a predefined net level of the signal is reached. If, however, any of the processing variables or the rheological definition of the end-point has changed, a more sophisticated approach is required, as described below.
End-Point Scale-Up
Scale-Up Attempts
Numerous studies were undertaken in an attempt to determine empirically (and, lately, with a solid theoretical foundation) useful scale-up parameters of the wet granulation process (e.g., 46, 87, 92).
In a seminal and elegant work published in 1993, Horsthuis and his colleagues from Organon in The Netherlands have studied granulation process in Gral mixers of 10, 75, and 300 liter size (29). Comparing relative swept volume, blade tip speed and Froude numbers with respect to end-point determination (as expressed by the time
after which there is no detectable change in particle size), they have concluded that only constant Froude numbers result in a comparable end-point.
In another attempt to determine good scale-up parameters, the University of Maryland group under the direction of Dr. Larry Augsburger (30) has applied the ideas of Leuenberger and Horsthuis to show that, for a specific material, end-point can be expressed in terms of wet massing time. For a constant ratio of a binder volume to a batch size, this factor was found to be inversely proportional to impeller speed when the impeller tip speed was held constant for all batches. However, this result was not corroborated by other studies or other materials.
Yet another example of semi-empirical scale-up effort (93) was based on the fact that normalized power profiles are very similar and allow for direct comparison of different size granulators, at least for the equipment and materials used in the study. Normalized power curve rose at a relatively constant rate in the region where the ratio of water to dry mass is 0.1 - 0.2 (“slope plateau”). Despite a rapid increase in the slope of the power curve, the desired end-point was still detectable at a moment when the slope of power consumption signal exceeded the plateau level by a factor of 5 (empirical observation). Using this approach, an acceptable end-point (target particle size of 135 microns) was first established on a 10 liter Fielder and then scaled to 65 liter Fielder and 250 liter Diosna.
Dimensional Analysis
A rational approach to scale-up using dimensional analysis has been in use in chemical engineering for quite some time. This approach, based on the use of process similarities between different scales, was being applied to pharmaceutical granulation since the early work of Hans Leuenberger in 1979 (40).
Dimensional analysis is a method for producing dimensionless numbers that completely describe the process. The analysis should be carried out before the measurements are made because dimensionless numbers essentially condense the frame in which the measurements are performed and evaluated. The method can be applied even when the equations governing the process are not known. Dimensional analytical procedure was first proposed by Lord Rayleigh in 1915 (94).
Principle of Similitude
Imagine that you have successfully scaled up from a 10 liter batch to 300 liter batch. What exactly happened? You may say: “I got lucky”. Apart from luck, there had to be similarity in the processing and the end-point conditions of the wet mass of the two batches.
According to the modeling theory, two processes may be considered similar if there is a geometrical, kinematic and dynamic similarity (95).
Two systems are called geometrically similar if they have the same ratio of characteristic linear dimensions. For example, two cylindrical mixing vessels are geometrically similar if they have the same ratio of height to diameter.
Two geometrically similar systems are called kinematically similar if they have the same ratio of velocities between corresponding system points. Two kinematically similar systems are dynamically similar when they have the same ratio of forces between corresponding points. Dynamic similitude for wet granulation would imply that the wet mass flow patterns in the bowl are similar.
The gist of dimensionless analysis is as follows: For any two dynamically similar systems, all the dimensionless numbers necessary to describe the process have the same numerical value (96). Once a process is expressed in terms of dimensionless variables, we are magically transferred in a world where there is no space and no time. Therefore, there is no scale and, consequently, there are no scale-up problems. The process is characterized solely by numerical values of the dimensionless variables (numbers). In other words, dimensionless representation of the process is scale-invariant.
Lack of geometrical similarity often is the main obstacle in applying the Dimensional Analysis to solving the scale-up problems. It was shown, for example, that Collette Gral 10, 75 and 300 are not geometrically similar (29). In such cases, a proper correction to the resulting equations is required.
Dimensionless Numbers
Dimensionless numbers most commonly used to describe wet granulation process are Newton, Froude and Reynolds:
N p = ΔP / (ρ n3 d5) Newton (power)
Fr = n2 d / g Froude
Re = d2 n ρ / ηReynolds
(for list of symbols, notation and dimensions, see Appendix).
Newton (power) number, which relates the drag force acting on a unit area of the impeller and the inertial stress, represents a measure of power requirement to overcome friction in fluid flow in a stirred reactor. In mixer-granulation applications, this number can be calculated from the power consumption of the impeller or estimated from the power consumption of the motor.
Froude Number (96) has been described for powder blending and was suggested as a criterion for dynamic similarity and a scale-up parameter in wet granulation (29). The mechanics of the phenomenon was described as interplay of the centrifugal force (pushing the particles against the mixer wall) and the centripetal force produced by the wall, creating a “compaction zone”.
Reynolds numbers relate the inertial force to the viscous force (97). They are frequently used to describe mixing processes and viscous flow, especially in chemical engineering (98).
We have seen that there exists sort of a “principle of equifinality” that states: “An end-point is an end-point is and end-point, no matter how it was obtained”. Different processing pathways can lead to different end-points, each with its own set of granulation properties. However, once an end-point is reached, it is characterized by certain numerical values of the dimensionless variables describing the process, and these values will be independent of scale.
At the same end-point, no matter how defined, the rheological and dimensional properties of the granules are similar. As we will see from the examples described below, that means that the density and dynamic viscosity of the wet mass are constant, and the only variables that are left are the process variables, namely batch mass, impeller diameter and speed, and the geometry of the vessel. Comparison of attainable Froude Numbers
Horsthuis et al. (29) showed that an end-point can be reproduced and scaled up in Gral mixers by keeping the Froude numbers constant. For the same end-point, in dynamically similar mixers (same geometrical ratios, same flow patterns), all dimensionless numbers describing the system should have the same numerical value, but Froude numbers for any mixer are easiest to compute.
Fig. 9. The range of Froude numbers for Collette Gral high-shear mixers. Each mixer has a range of attainable Froude numbers, and an end-point transfer between mixers can only be achieved when such ranges overlap. Fig. 9 represents such a range for Collette Gral mixers. It can be seen that Gral 10 and Gral 150 has no overlap of Froude number ranges, and therefore a direct scale-up is not possible (in addition, Gral mixers are not exactly similar geometrically, as was stated elsewhere).
The range of Froude numbers for Fielder PMA series mixers is shown on Fig. 10. The 10 liter laboratory scale mixer at its lowest speed settings can reach the Froude numbers of all other mixers, except one. These considerations can be useful for planning a scale-up or technology transfer operation.
Fig. 10. The range of Froude numbers for Fielder PMA high-shear mixers.
Π-theorem (Buckingham)
The so-called π-theorem (or Buckingham theorem (99) states:
Every physical relationship between n dimensional variables and constants
?(x0, x1, x2, … , xn) =0
can be reduced to a relationship
?(Π0 ,Π1, … , Πm) = 0
between m = n - r mutually independent dimensionless groups,
where r = number of dimensional units, i.e. fundamental units (rank of the dimensional matrix).
Scientific scale-up procedure:
1. Describe the process using a complete set of dimensionless numbers, and
2. Match these numbers at different scales.
The dimensionless space in which the measurements are presented or measured will make the process “scale invariant”.
Relevance List
The dimensional analysis starts with a list of all variables thought to be important for the process being analyzed (the so-called “relevance list”).
To set up a relevance list for any process, one needs to compile a complete set of all relevant and mutually independent variables and constants that affect the process. The word “complete” is crucial here. All entries in the list can be subdivided into geometric, physical and operational. Each relevance list should include only one target (dependent “response”) variable.
Many pitfalls of dimensional analysis are associated with the selection of the reference list, target variable, or measurement errors (e.g. when friction losses are of the same order of magnitude as the power consumption of the motor). The larger the scale-up factor, the more precise the measurements of the smaller scale have to be (96).
Dimensional Matrix
Dimensional analysis can be simplified by arranging all relevant variables from the relevance list in a matrix form, with a subsequent transformation yielding the required dimensionless numbers. The dimensional matrix consists of a square core matrix and a residual matrix (you will see examples in the Case Studies below).
The rows of the matrix consist of the basic dimensions, while the columns represent the physical quantities from the relevance list. The most important physical properties and process-related parameters, as well as the “target” variable (that is, the one we would like to predict on the basis of other variables) are placed in one of the columns of the residual matrix.
The core matrix is then linearly transformed into a matrix of unity where the main diagonal consists only of ones and the remaining elements are all zero. The
dimensionless numbers are then created as a ratio of the residual matrix and the core matrix with the exponents indicated in the residual matrix. This rather simple process will be illustrated below in the examples.
Case Study I: Leuenberger (1979,1983)
This example is based on the ground-breaking studies conducted by Hans Leuenberger at the University of Basel and Sandoz AG (40, 100-103).
Table I. The Relevance List used by Leuenberger [1983]
Quantity Symbol Units
Dimensions
1 Power consumption P Watt M L
2 T-3
2 Specific
density ρkg / m3 M
L-3
3 Blade
diameter d m L
4 Blade velocity n rev / s T-1
5 Binder
amount s kg M
6 Bowl
volume V b m3L3
7 Gravitational
constant g m / s2 L
T-2
8 Bowl
height H m L
The Relevance List in Table I reflects certain assumptions used to simplify the model, namely, that there are short range interactions only and no viscosity factor (and therefore, no Reynolds number).
Why do we have to consider the gravitational constant? Well, imagine the same process to be done on the moon - would you expect any difference?
One target variable (Power consumption) and 7 process variables / constants thus represent the number n=8 of the Π-theorem. The number of basic dimensions r = 3 (M, L, and T). According to the theorem, the process can be reduced to relationship between m = n - r = 8 – 3 = 5 mutually independent dimensionless groups.
Table II. The Dimensional Matrix for Case Study I
Core matrix Residual Matrix
ρ d n P s V b g H
Mass [M] 1 0 0 1 1 0 0 0
Length [L] –3 1 0 2 0 3 1 1
极点及系统稳定性
极点对系统性能影响 一.控制系统与极点 自动控制系统根据控制作用可分为:连续控制系统和采样控制系统,采样系统又叫离散控制系统。通常把系统中的离散信号是脉冲序列形成的离散系统,称为采样控制系统。连续控制系统即指控制量为连续的模拟量如时变系统。 系统的数学模型一般由系统传递函数表达。传递函数为零初始条件下线性系统响应(即输出)量的拉普拉斯变换(或z 变换)与激励(即输入)量的拉普拉斯变换之比。记作Φ(s )=Xo (s )/Xi (s ),其中Xo (s )、Xi (s )分别为输出量和输入量的拉普拉斯变换。 特征方程的根称为极点。如试Φ﹙S ﹚= C [∏(S-Pi )/∏(S-Qi) ]中Q1 Q2 Q3 …… Qi ……即为系统的极点。 二.极点对系统的影响 极点--确定了系统的运动模态;决定了系统的稳定性。下面对连续系统与离散系统分别进行分析: ⑴连续系统 理论分析:连续系统的零极点分布有如下几种形式 设系统函数为: 将H(S)进行部分分式展开: 1n a s -+++
系统冲激响应H(S)的时域特性h(t)随时间衰减的信号分量完全由系统函数H(S)的极点位置决定。每一个极点将决定h(t)的一项时间函数。 稳定性:由上述得知Y(S)= C [∏(S-Pi )/(S-Qi) ]可分解为Y(S)=C1/(S-τ1)+ C2/(S-τ2)+ C3/(S-τ3)+……+ Ci/(S-τi)+…… 则时间响应为 …… 由于特征方程的根不止一个,这时,应把系统的运动看成是多个运动分量的合成。只要有一个运动分量是发散的,则系统是不稳定的。因此,特征方程所有根的实部都必须是负数,亦即所有的根都在复平面的左半平面。 通过复变函数幅角定理将S 由G 平面映射到GH 平面。 如果封闭曲线 F 内有Z 个F(s)的零点,有P 个F(s)的极点,则s 沿 F 顺时针转一圈时,在F(s)平面上,F(s)曲线绕原点顺时针转的圈数R 为z 和p 之差,即R =z -p 。 若R 为负,表示F(s)曲线绕原点逆时针转过的圈数。 F(s)的分母是G0(s)的分母,其极点是G0(s)的极点;其分子是?(s)的分母,即?(s)的特征多项式,其零点是?(s)的极点。 取D 形曲线(D 围线)如图所示,是整个右半复平面。 且设D 曲线不经过F(s)的任一极点或零点。 s 沿D 曲线顺时针变化一周,F(s)顺时针包围原点的周数为: n=z-p=F(s)在右半复平面的零点数(闭环传函在右半复平面极点数) -F(s)在右半复平面的极点数(开环传函在右半复平面极点数) 所以闭环系统稳定的充分必要条件是: n=- p =-开环传函在右半复平面的极点数 1212()n s t s t s t n y t C e C e C e =+++0()0()0()0()t s y t y t Ce y t y t t ααααα=<→?? ===??>→∞? →∞(1)只有一个实根:时,时,恒量时,()()121()0cos()00j t j t t s j y t C e C e C e t t αωαωααωαω?αα+-=±=+?=+==??>? →∞(2)有一对复根:时,收敛时,等幅振荡时,发散
详细的制粒技术及经验
药智网免费提供药品标准查询”https://www.360docs.net/doc/e13856465.html,/biaozhun.htm 一、制粒技术概念 制粒(granulation)技术:是把粉末、熔融液、水溶液等状态的物料加工制成一定形状与大小的粒状物的技术。 制粒的目的:①改善流动性,便于分装、压片;②防止各成分因粒度密度差异出现离析现象;③防止粉尘飞扬及器壁上的粘附;④调整堆密度,改善溶解性能;⑤改善片剂生产中压力传递的均匀性;⑥便于服用,方便携带,提高商品价值。 制粒方法:湿法制粒、干法制粒、一步制粒、喷雾制粒,其中湿法制粒应用最多。 制粒技术的应用:在固体制剂,特别在颗粒剂、片剂中应用最为广泛。 二、制粒方法 (一)、湿法制粒 湿法制粒:在药物粉末中加入粘合剂或润湿剂先制成软材,过筛而制成湿颗粒,湿颗粒干燥后再经过整粒而得。湿法制成的颗粒具用表面改性较好、外形美观、耐磨性较强、压缩成形性好等优点,在医药工业中应用最为广泛。 湿法制粒机理:首先是粘合剂中的液体将药物粉末表面润湿,使粉粒间产生粘着力,然后在液体架桥与外加机械力的作用下制成一定形状和大小的颗粒,经干燥后最终以固体桥的形式固结。 湿法制粒主要包括制软材、制湿颗粒、湿颗粒干燥及整粒等过程。 1、制软材:将按处方称量好的原辅料细粉混匀,加入适量的润湿剂或粘合剂混匀即成软材。 制软材应注意的问题 (1)粘合剂的种类与用量要根据物料的性质而定; (2)加入粘合剂的浓度与搅拌时间,要根椐不同品种灵活掌握; (3)软材质量。由于原辅料的差异,很难定出统一标准,一般凭经验掌握,用手捏紧能成团块,手指轻压又能散裂得开。 (4)湿搅时间的长短对颗粒的软材有很大关系,湿混合时间越长,则粘性越大,制成的颗粒就越硬。 2、制湿颗粒:使软材通过筛网而成颗粒。颗粒由筛孔落下如成长条状时,表明软材过湿,湿合剂或润湿剂过多。相反若软材通过筛孔后呈粉状,表明软材过干,应适当调整。 常用设备:摇摆式颗粒机、高速搅拌制粒机 筛网:有尼龙丝、镀锌铁丝、不锈钢、板块四种筛网。 3、湿颗粒干燥:过筛制得的湿颗粒应立即干燥,以免结块或受压变形(可采用不锈钢盘将制好的湿颗粒摊开放置并不时翻动以解决湿颗粒存放结块及变形问题)。 干燥温度:由原料必性质而定,一般为50-60℃;一些对湿、热稳定的药物,干燥温度
数学物理方法作业
数学物理方法简答题 1.复数有哪几种表达方式?在复数的开方运算和对数函数的计算中,应特别 注意复数的什么性质?(复习掌握复数运算和几种基本函数的定义和计算) 书上列出了三种:代数式,三角式,指数式。其实还可以用级数式表示复数。 注意角度是除以/乘以一个数。 2.复变函数可导的充分必要条件是什么?可导与解析这两个概念有什么联 系和区别?(复习掌握柯西-黎曼条件以及求解解析函数的实部或虚部的方法) 复变函数可导的充分必要条件:函数的偏导数存在且连续,并满足柯西-黎曼方程。 联系与区别:对于一个点,函数解析必定可导,反之不一定;对于一个区域,解析和可导等价。 3.解析函数的两条性质是什么? 一:函数的实部和虚部分别等于一个常数,这个两个曲线族在其区域B上是相互正交的。 二:函数的实部和虚部均为其区域B上的调和函数。 4.已知某函数在某一回路上的积分为零,可否据此对此函数的解析性质作出 判断?为什么?(复习掌握柯西定理、柯西公式) 可以,根据柯西定理,在闭单连通区域上的解析函数回路积分为零,可以据此对函数解析性质做出判断。 5.什么情况下某一积分回路的内部一定是复连通的区域? 在回路内有奇点,则该回路积分会等于对其内部奇点所有回路积分之和。 6.收敛的幂级数和双边幂级数的收敛区域分别是什么类型的区域?在收敛 区域的境界线和外部是否一定发散?(复习掌握计算泰勒展开和洛朗展开的基本方法,特别是有理分式的展开) 一个是圆一个是环。在幂级数的境界线上要具体分析,其余都发散。 7.奇点可分为哪几类?孤立奇点可分为哪几类?简要说明它们之间的区别。
奇点分为孤立奇点和非孤立奇点,。函数在点z不可导,若在z的任意小领域除点z外处处可导,则为孤立奇点,若在任意小领域内可以找到除z以外不可导的点,则z为非孤立奇点。 孤立奇点根据挖去该点而形成环域上的解析函数的洛朗展开级数中负幂项情况,可以分为可取奇点(没有负幂项)、极点(有限个负幂项)、本性奇点(无限个负幂项)。 8.如何判断极点的阶数? 函数在该极点上的洛朗展开,最低次幂项的次数的绝对值是极点的阶数。 9.试用文字说明什么是留数?(复习掌握留数计算相关公式) 被积函数在回路上的积分,将被积函数展开后逐项积分,除去留下来的项其他都为零,则这个不为零的项除以一常数后称之为留数/残数。 10.留数定理将回路积分归结成什么?对于回路积分的计算有什么意义?(复 习掌握留数定理计算回路积分和实函数积分的相关公式) 将回路积分归结成根据极点求留数,简化了对回路积分的计算。
中药湿法制粒的原理和小经验
[转贴]中药湿法制粒的原理和小经验 湿法制粒, 中药, 原理, 粒子, 经验 湿法制粒(wet granulation)原理 是在药物粉末中加入液体粘合剂,靠粘合剂的架桥或粘结作用使粉末聚结在一起而制备颗粒的方法。由于湿法制粒的产物具有外形美观、流动性好、耐磨性较强、压缩成形性好等优点,在医药工业中的应用最为广泛。而对于热敏性、湿敏性、极易溶性等特殊物料可采 用其它方法制粒。 (一)制粒机理 1.粒子间的结合力 制粒时多个粒子粘结而形成颗粒,Rumpf提出粒子间的结合力有五种不同方式(1)固体粒子间引力固体粒子间发生的引力来自范德华力(分子间引力)、静电力和磁力。这些作用力在多数情况下虽然很小,但粒径<50μm时,粉粒间的聚集现象非常显著。这些作用随着粒径的增大或颗粒间距离的增大而明显下降,在干法制粒中范德华力的作用 非常重要。 (2)自由可流动液体(freely movable liquid)产生的界面张力和毛细管力以可流动液体作为架桥剂进行制粒时,粒子间产生的结合力由液体的表面张力和毛细管力产生,因此液体的加入量对制粒产生较大影响。液体的加入量可用饱和度S表示:在颗粒的空隙中液体架桥剂所占体积(VL)与总空隙体积(VT)之比,液体在粒子间的充填方式由液体的加入量决定,参见图16-25。(A)干粉状态;(a)S≤0.3时,液体在粒子空隙间充填量很少,液体以分散的液桥连接颗粒,空气成连续相,称钟摆状(pendular state);(b)适当增加液体量0.3<S<0.8时,液体桥相连,液体成连续相,空隙变小,空气成分散相,称索带状(funicularstate);(c)液体量增加到充满颗粒内部空隙(颗粒表面还没有被液体润湿)S≥0.8时,称毛细管状(capillary state);(d)当液体充满颗粒内部与表面S≥1时,形成的状态叫泥浆状(slurry state)。毛细管的凹面变成液 滴的凸面。 一般,在颗粒内液体以悬摆状存在时,颗粒松散;以毛细管状存在时,颗粒发粘,以索带状存在时得到较好的颗粒。可见液体的加入量对湿法制粒起着决定性作用。 (3)不可流动液体(immobile liquid)产生的附着力与粘着力不可流动液体包括高粘度液体和吸附于颗粒表面的少量液体层(不能流动)。因为高粘度液体的表面张力很小,易涂布于固体表面,靠粘附性产生强大的结合力;吸附于颗粒表面的少量液体层能消除颗粒表面粗糙度,增加颗粒间接触面积或减小颗粒间距,从而增加颗粒间引力等,如图 16-26A[11]。淀粉糊制粒产生这种结合力。 (4)粒子间固体桥(solid bridges)固体桥(图16-26B)形成机理可由以下几方面论述。①结晶析出?架桥剂溶液中的溶剂蒸发后析出的结晶起架桥作用;②粘合剂固化?液体状态的粘合剂干燥固化而形成的固体架桥;③熔融?由加热熔融液形成的架桥经冷却固结
专升本药学选择题
专升本药学选择题 本题答案:c 第2题下列哪种片剂不宜用硬脂酸镁作润滑剂 本题答案:c 第3题专门治理的药品是指 本题答案:d 第4题湿法制粒工艺流程图为 本题答案:b 第5题用以补充体内水分及电解质的输液是 本题答案:e 第6题舌下片应符合以下哪一条要求 本题答案:D 第7题调配处方时,如发觉处方书写不符合要求或有差错,药剂人员的正确做法是 本题答案:A
第8题关于医院制剂叙述错误的是 本题答案:A 第9题药材含水量一样为多少,大量生产浸出制剂应结合生产体会,定出含水量的操纵标准()。 A.8%~lO% B.3%~5% C.9%~16% D.12%~20% E.30%~50% 本题答案:C 第10题欲称取0.1g药物,按规定其相对误差不超过±10%,选用天平的分度值为 本题答案:C 第11题某药物的组织结合率专门低,讲明 本题答案:D 第12题非处方药分为甲、乙两类的按照是 本题答案:D 第13题西药或中成药处方,每张处方不得超过 本题答案:B
第14题口服缓释制剂可采纳的制备方法是 本题答案:D 第15题PVP是指 本题答案:B 第16题我国的药品质量监督治理的原则不包括 本题答案:C 第17题OTC分为甲、乙两类的要紧依据是 本题答案:D 第18题《药品治理法》规定,医疗机构配制制剂必须取得 本题答案:E 第19题禁止公布广告的药品是 本题答案:C 第20题甲氧氯普胺增加对乙酰氨基酚的吸取速度,其机制是本题答案:A
第21题栓剂的吸取途径中通过肝脏的首过作用的是 本题答案:A 第22题仅供皮肤使用的液体剂型是 本题答案:E 第23题关于纳米载药系统叙述错误的是 本题答案:A 第24题《药品治理法实施条例》规定,个人设置的门诊部、诊所等医疗机构不得 本题答案:A 第25题乳剂中,若水为分散相,油为连续相,则会制成何类型的乳剂 本题答案:B 第26题《医疗机构制剂许可证》的有效期为 本题答案:D 第27题我国药品技术监督的最高机构是 本题答案:D
第五章 留数(答案)
复变函数练习题 第五章 留数 系 专业 班 姓名 学号 §1 孤立奇点 孤立奇点类型的判别法 1、洛朗展开法 f(z)在点a 处的洛朗展式中, 若无负幂项,则点a 为可去奇点; 若负幂项最高次数为m ,则点a 为m 阶极点; 若负幂项为无穷多个,则点a 为本性奇点。 2、极限法 lim ()z a f z → 存在且有限,则点a 为可去奇点; 等于无穷,则 a 为极点(无法判断阶数); 不存在且不等于无穷,则a 为本性奇点。 3、判断极点的方法 1 ()()()m f z g z z a = -,g(z)在点a 解析且g(a)不等于零; 1()()lim ()lim()()() m m z a z a f z g z g z z a f z z a →→= =--,存在且有限; 1 ()()() m z a h z f z =-, h(z)在点a 解析且h(a)不等于零 一、选择题 1.函数 cot 23 z z π-在||2z i -=内奇点的个数为 [ D ] (A )1 (B )2 (C )3 (D )4 cot cos 3 (23)sin 0,()23(23)sin 2 z z z z z k k z z z ππππ=-=?=∈--Z ,
2.设()f z 与()g z 分别以z a =为可去奇点和m 级极点,则z a =为()()f z g z +的 [ C ] (A )可去奇点 (B )本性奇点 (C )m 级极点 (D )小于m 级的极点 (对f(z)和g(z)分别进行洛朗展开并求和) 3.0z =为函数2 41sin z e z z -的m 级极点,那么m = [ C ] (A )5 (B )2 (C )3 (D ) 4 224 2 2455 32 01112!3.3=(1)sin sin sin sin 2!lim (1)1sin 2!z z z z z e z e z z z z z z z z z z z z z z →??++ ?--?=?=?++ ? ? ?++= ?? ? L L L 利用方法, 4.z =∞是函数3 2 32z z z ++的 [ B ] (A )可去奇点 (B )一级极点 (C )二级极点 (D )本性奇点 32 22 32321=32=0z z z z z z ζζζζ??++++=++ ??? 以为一阶极点 5.1z =是函数1 (1)sin 1 z z --的 [ D ] (A )可去奇点 (B )一级极点 (C )一级零点 (D )本性奇点 (将函数在z=1洛朗展开,含无穷多个负幂项) 二、填空题 1.设0z =为函数3 3 sin z z -的m 级零点,那么m = 9 。 () () 3 5 3391563 3 3 3 91sin ()()3!5!3!5!3!5! z z z z z z z z z z -=--++=-+=-+L L L 2.设0z =为函数3sin z z 的n 级极点,那么n = 2 。 三、解答题 1.下列函数在有限点处有些什么奇点如果是极点,指出它的级:
湿法制粒
湿法制粒 湿法制粒(wet granulation)是在药物粉末中加入液体粘合剂,靠粘合剂的架桥或粘结作用使粉末聚结在一起而制备颗粒的方法。由于湿法制粒的产物具有外形美观、流动性好、耐磨性较强、压缩成形性好等优点,在医药工业中的应用最为广泛。而对于热敏性、湿敏性、极易溶性等特殊物料可采用其它方法制粒。 (一)制粒机理 1.粒子间的结合力 制粒时多个粒子粘结而形成颗粒,Rumpf提出粒子间的结合力有五种不同方式[10]: (1)固体粒子间引力固体粒子间发生的引力来自范德华力(分子间引力)、静电力和磁力。这些作用力在多数情况下虽然很小,但粒径<50μm时,粉粒间的聚集现象非常显著。这些作用随着粒径的增大或颗粒间距离的增大而明显下降,在干法制粒中范德华力的作用非常重要。 (2)自由可流动液体(freely movable liquid)产生的界面张力和毛细管力以可流动液体作为架桥剂进行制粒时,粒子间产生的结合力由液体的表面张力和毛细管力产生,因此液体的加入量对制粒产生较大影响。液体的加入量可用饱和度S 表示:在颗粒的空隙中液体架桥剂所占体积(VL)与总空隙体积(VT)之比,即。 液体在粒子间的充填方式由液体的加入量决定,参见图16-25。(A)干粉状态; (a)S≤0.3时,液体在粒子空隙间充填量很少,液体以分散的液桥连接颗粒,空气成连续相,称钟摆状(pendular state);(b)适当增加液体量0.3<S<0.8时,液体桥相连,液体成连续相,空隙变小,空气成分散相,称索带状(funicular state); (c)液体量增加到充满颗粒内部空隙(颗粒表面还没有被液体润湿)S≥0.8时,称毛细管状(capillary state);(d)当液体充满颗粒内部与表面S≥1时,形成的状态叫泥浆状(slurry state)。毛细管的凹面变成液滴的凸面。 一般,在颗粒内液体以悬摆状存在时,颗粒松散;以毛细管状存在时,颗粒发粘,以索带状存在时得到较好的颗粒。可见液体的加入量对湿法制粒起着决定性作用。 (3)不可流动液体(immobile liquid)产生的附着力与粘着力不可流动液体包括高粘度液体和吸附于颗粒表面的少量液体层(不能流动)。因为高粘度液体的表面张力很小,易涂布于固体表面,靠粘附性产生强大的结合力;吸附于颗粒表面的少量液体层能消除颗粒表面粗糙度,增加颗粒间接触面积或减小颗粒间距,从而增加颗粒间引力等,如图16-26A[11]。淀粉糊制粒产生这种结合力。 (4)粒子间固体桥(solid bridges)固体桥(图16-26B)形成机理可由以下几方面论述。①结晶析出—架桥剂溶液中的溶剂蒸发后析出的结晶起架桥作用;②粘合剂固化—液体状态的粘合剂干燥固化而形成的固体架桥;③熔融—由加热熔融液形成的架桥经冷却固结成固体桥。④烧结和化学反应产生固体桥。制粒中常见的固体架桥发生在粘合剂固化或结晶析出后,而熔融—冷凝固化架桥发生在压片,挤压制粒或喷雾凝固等操作中。 (5)粒子间机械镶嵌(mechanical interlocking bonds) 机械镶嵌发生在块状颗粒的搅拌和压缩操作中。结合强度较大(如图16-26C),但一般制粒时所占比例不
判断极点阶数的方法
判断极点阶数的方法 已知0z 是()z f 的n 阶极点,是()z g 的m 阶极点。 (一)0z 是()()z g z f 的m+n 阶极点 () 的二级极点是则的一级极点的一级极点,是是例如:1 1 0;1110-=-=z z e z z e z z (二)如果n m ≠,则0z 是()()z g z f ±的),max(n m 阶极点 的二级极点是则级极点的级极点,是的是 例如:1 110;11121022-+=-=z z e z z e z z 如果n m =,则需要把()()z g z f +通分成 () () z g z f 11这种形式 () 判断。再用下面(三)的方法通分成需要把的一级极点 却不是则的一级极点的一级极点,是是例如:,1 111111 10;1110-------=-=z z z z z e z z e e z e z z e z z 已知0z 是()z f 1的n 阶零点,是()z g 1的m 阶零点。 (三)0z 是 () () z g z f 11的m-n 阶极点,其中0>-n m , ( ) ( ) 级极点的是 则级零点的级零点,是的是例如:21 sin 0;311sin 02 2 -=-=z z e z z z e z z z 如果0≤-n m ,则0z 是 () () z g z f 11的可去奇点。 ( ) () 的可去奇点 是则级零点的级零点,是的是例如:1 10;21210---=---=z z z z e z z e z e z z e z 判断零点阶数的方法 已知0z 是()z f 1的n 阶零点,是()z g 1的m 阶零点。 (四)0z 是()()z g z f 11的m+n 阶零点 ( ) 的二级零点 是则的一级零点的一级零点,是是例如:10;10-=-=z z e z z e z z (五)如果n m ≠,则0z 是()()z g z f ±的),min(n m 阶零点
【运行】HLSG系列湿法混合制粒机操作规程
【关键字】运行 一.目的 规范HLSG系列湿法混合制粒机的操作方法,确保设备及操作人员的安全,使设备在正常状态下运行。 二.适用范围 适用于HLSG220F、HLSG50A湿法混合制粒机操作全过程。 三.责任者 操作人员:负责设备的日常点检和操作。 车间管理人员:负责设备运行全过程的监控以及突发事件的处理。 设备管理人员:负责指导、监督操作人员的行为,本规程的培训以及监督本规程执行情况,确保生产正常。 四.相关定义 无。 五.工作程序 1.设备说明 1.1.设备简介 HLSG系列湿法混合制粒机是能一次完成混合、加湿、制粒、清洗工序,生产效率高,制粒效果好,无交叉感染,符合GMP标准,物料锅及物料锅盖采用了旋压及拉伸技术,几何形状好,整体强度高,另外该机设有多项安全联锁装置,使用
该机安全、可靠,该机由中航工业北京航空工程研究所制造。 1.2. HLSG系列湿法混合制粒机技术参数 1.3.原理说明 HLSG系列湿法混合制粒机由主机、搅拌部分、出料机构、切碎(辅助搅拌)部分、加浆/清洗部分(配选)、PLC控制部分、平衡支撑部分、操作箱和强电柜组成。药材细粉或提取物干浸膏细粉等粉状物料投入到物料锅,待物料锅盖关闭后在搅拌桨的搅拌下,粉料在容器内作上下翻滚的旋转运动,同时物料又被搅拌桨的离心力作用沿锥形壁方向作运动变化,形成半流动的高效混和状态,物料被碰撞分散达到充分的混和。制粒时利用加浆装置(配选)把粘合剂均匀注入,使粉料逐渐湿润,物料性状发生变化,加强了桨叶和筒壁对物料的挤压、
摩擦、捏合,在粘合剂的作用下,逐步生成液桥,物料逐步转变为疏松的软材。 2.开机前准备工作 -检查油雾器内应不缺油,并将分水过滤器内部积水和杂质清除。 -检查各控制仪表应完好,在有效期内。 -打开压缩空气阀门,将三通球阀旋至通气位置,压缩空气压力调至≥0.50MPa。 -打开物料锅盖,检查搅拌浆和切碎刀中心部的进气气流,如不理想,可调节气液操作板上的流量计,一般正常流量控制为0.80 m3/h。 -检查切碎刀刀片安装应正确,且和搅拌浆都已紧固,用手转动切碎刀和搅拌浆,转动应灵活,无卡滞现象,再关闭物料锅盖。 3.操作步骤 3.1.开机 HLSG220F湿法混合制粒机 -确认已打开压缩空气阀门,并打开总电源开关和控制台上钥匙开关,触摸屏加入启动界面如下图1: 图1 图2 -点击登陆按键,加入主菜单界面如上图2,点击手动控制按键,加入手动界面如下图3:图3 -点击“出料开”和“出料关”键,检查出料活塞的进退应灵活,速度适中,如不理想,可调节气缸下面的接头式单向节流阀。 -点击启动手动控制键,指示灯亮。 -打开观察口盖,点击搅拌开和切碎开按键,短暂开启两电机,确认搅拌浆和切碎刀的旋转方向(面向零件为逆时针方向)和两电机运行应正常。 -检查安全连锁装置应可靠。 -检查设备整机运行应平稳,无异常响声。
片剂的题目
片剂 一、名词解释 溶出度;泡腾崩解剂;湿法制粒;稀释剂;粘合剂;崩解剂;糖衣片;肠溶衣片; 口腔贴片;分散片;口含片;植入片; 二、判断题 1. 片剂加入表面活性剂后,可改善其润湿性,加快其崩解。 2.片重差异受压片时颗粒流动性的影响。 3. 咀嚼片常用甘露醇作为稀释剂,通常情况下不加崩解剂。 4. 片剂径向加压测得破碎所需之力称为表面硬度。 5. 片剂制备必须具备的三个条件是流动性、压缩成型性、良好的崩解 性 6.片剂包糖衣的步骤:包粉衣层、包糖衣层、包有色衣层、打光。 7. 片剂中最常用的润滑剂是轻质氧化镁 8. 片剂中包衣前后,均需进行片重差异的检查。 9. 片剂包糖衣过程中常用虫腊作打光层。 10. 环糊精是常用的片剂稀释剂。 三、填空题 1、根据辅料的作用特点,片剂的辅料可分为稀释剂、、润湿 剂、和润滑剂等 2、可供粉末直接压片的填充剂有、、等 3、制粒过程中常用的润湿剂主要有、。 4、淀粉浆的制备方法有和两种。
5、崩解剂的加入方法有法、法和法。 6、广义的润滑剂包括、、三种。 7、干法制粒有和法。 8、片剂崩解迟缓的原因主要有、、 和压力过大的原因等。 9、凡检查的制剂,不必检查片重差异,凡检查的制剂,不必检查崩解度。 10、CAP是指邻苯二甲酸醋酸纤维素、在片剂中用作__________材料。 11、片剂中根据崩解剂的种类不同其崩解的作用机理有__________、__________、 __________、酶解作用。 四、单选题 1、下列片剂的叙述错误的为( )。 A.片剂为药物与辅料均匀混合后压制而成的片状制剂 B.片剂的生产机械化,自动化程度较高 C.片剂的生产成本及售价较高 D.片剂运输、贮存、携带及应用方便 2、下列是片剂的特点的叙述,不包括 A.体积较小,其运输、贮存及携带、应用都比较方便
药剂学期末复习试题库附答案_(1)
药剂学期末复习试题库附答案_(1) 题目:药剂学概念正确的表述是 答案A 研究药物制剂的处方理论、制备工艺和合理应用的综合性技术科学 答案B 研究药物制剂的基本理论、处方设计、制备工艺和合理应用的综合性技术科学答案C 研究药物制剂的处方设计、基本理论和应用的技术科学 答案D 研究药物制剂的处方设计、基本理论和应用的科学 题目编号: 0102 第 1 章 1 节页码难度系数: A 题目:中国药典中关于筛号的叙述,哪一个是正确的()。 药剂学期末复习试题库附答案_(1) 答案B 一号筛孔最大,九号筛孔最小答案C 最大筛孔为十号筛 答案D 二号筛相当于工业200目筛 药剂学期末复习试题库附答案_(1)题目:下述中哪相不是影响粉体流动性的因素()。 药剂学期末复习试题库附答案_(1) 答案B 含湿量答案C 加入其他成分答案D 润湿剂 药剂学期末复习试题库附答案_(1) 题目:用包括粉体本身孔隙及粒子间孔隙在内的体积计算的密度为()。 药剂学期末复习试题库附答案_(1) 答案B 真密度答案C 粒密度答案D 高压密度 药剂学期末复习试题库附答案_(1)题目:下列关于胶囊剂的叙述哪一条不正确()。 药剂学期末复习试题库附答案_(1) 答案B 可提高药物的稳定性答案C 可避免肝的首过效应答案D 可掩盖药物的不良嗅味 药剂学期末复习试题库附答案_(1) 题目:配制含毒剧药物散剂时,为使其均匀混合应采用()。 药剂学期末复习试题库附答案_(1) 答案B 振摇法答案C 搅拌法 答案D 等量递增法 药剂学期末复习试题库附答案_(1)题目:药物剂量为0.01-0.1g时可配成()。 药剂学期末复习试题库附答案_(1)答案B.1:100倍散答案C.1:5倍散
极点与系统稳定性
极点对系统性能影响 一.控制系统与极点 自动控制系统根据控制作用可分为:连续控制系统和采样控制系统,采样系统又叫离散控制系统。通常把系统中的离散信号是脉冲序列形成的离散系统,称为采样控制系统。连续控制系统即指控制量为连续的模拟量如时变系统。 系统的数学模型一般由系统传递函数表达。传递函数为零初始条件下线性系统响应(即输出)量的拉普拉斯变换(或z变换)与激励(即输入)量的拉普拉斯变换之比。记作Φ(s)=Xo(s)/Xi(s),其中Xo(s)、Xi(s)分别为输出量和输入量的拉普拉斯变换。 特征方程的根称为极点。如试Φ﹙S﹚= C [∏(S-Pi)/∏(S-Qi) ]中Q1 Q2 Q3 ……Qi ……即为系统的极点。 二.极点对系统的影响 极点--确定了系统的运动模态;决定了系统的稳定性。下面对连续系统与离散系统分别进行分析: ⑴连续系统 理论分析:连续系统的零极点分布有如下几种形式 设系统函数为: 将H(S)进行部分分式展开:
系统冲激响应H(S)的时域特性h(t)随时间衰减的信号分量完全由系统函数H(S)的极点位置决定。每一个极点将决定h(t)的一项时间函数。 稳定性:由上述得知Y(S)= C [∏(S-Pi )/(S-Qi) ]可分解为Y(S)=C1/(S-τ1)+ C2/(S-τ2)+ C3/(S-τ3)+……+ Ci/(S-τi)+…… 则时间响应为 …… 由于特征方程的根不止一个,这时,应把系统的运动看成是多个运动分量的合成。只要有一个运动分量是发散的,则系统是不稳定的。因此,特征方程所有根的实部都必须是负数,亦即所有的根都在复平面的左半平面。 通过复变函数幅角定理将S 由G 平面映射到GH 平面。 如果封闭曲线 F 内有Z 个F(s)的零点,有P 个F(s)的极点,则s 沿 F 顺时针转一圈时,在F(s)平面上,F(s)曲线绕原点顺时针转的圈数R 为z 和p 之差,即R =z -p 。 若R 为负,表示F(s)曲线绕原点逆时针转过的圈数。 F(s)的分母是G0(s)的分母,其极点是G0(s)的极点;其分子是?(s)的分母,即?(s)的特征多项式,其零点是?(s)的极点。 取D 形曲线(D 围线)如图所示,是整个右半复平面。 且设D 曲线不经过F(s)的任一极点或零点。 s 沿D 曲线顺时针变化一周,F(s)顺时针包围原点的周数为: n=z-p=F(s)在右半复平面的零点数(闭环传函在右半复平面极点数) -F(s)在右半复平面的极点数(开环传函在右半复平面极点数) 所以闭环系统稳定的充分必要条件是: n=- p =-开环传函在右半复平面的极点数 1212()n s t s t s t n y t C e C e C e =+++ 0()0()0()0()t s y t y t Ce y t y t t ααααα=<→?? ===??>→∞?→∞(1)只有一个实根:时,时,恒量时,()()121 ()0cos()00j t j t t s j y t C e C e C e t t αωαωααωαω?αα+-=±=+?=+==??>? →∞ (2)有一对复根:时,收敛时,等幅振荡时,发散
制粒工艺操作规范
总的工序流程:领料→拆料→处理→投料→制软材(叫粘合剂)→制湿颗粒→干燥→整粒→称重混合(加草莓粉末香精)→储存 一原辅料粉碎过筛 1.相关流程:预算领取物料>上报统计员拿取领料单>仓库领料至物净间点数(检查外包装袋有无破损,如有破损应及时 与仓库人员说明情况)>拆外包装消毒放置原辅料存放间>过筛置备料间。(做好标识,并填写生产记录与管理记录) 2.头孢克肟颗粒使用原辅料过筛目数:头孢克肟过100目,甘露醇过60目,交联聚维酮过60目。 3.如何提前预算领取的物料数量。 确保足够的备料量,需根据周生产计划量和岗位原辅料使用记录来确定,提前领取的物料数量。 4.在仓库领料需做的工作? 核对物料的批号、品名、数量、进库批号,并且拿相应的物料检验报告单。 5.拆除处包装,需检查物料处包装是否破损,物料颜色是否异常有变化的情况并及时反馈给质检员或工艺员。 6.拆除外包装的物料需用3%的过氧化氢消毒物料外表面,并贴上物料卡,拉置原辅料存放间。 7.原辅料粉碎过筛,应核对对处理物料所需筛网目数,物料品名,批号,数量更换品种必须换筛网清洗摇摆机。 8.物料过筛后收率应不低于99.5%。 9.处理后的物料应将原始批号,进库批号,物料总重量报至工艺员处。 10.对于不合格的物料应放回到原包装或原物料桶内并做好密封与标识。 二.投料称量 11.(一)生产前的准备。 12.1.检查工作区已没有存在任何与本次生产无关的残留物或生产记录等,并已清洁消毒且在有效 期内。 13.2.检查生产区内的压差,温度,温度是否在规定范围内。 14.3.在工艺员领取本次需生产的指令单和所需记录。(记得复核指令单) 15.4.根据指令单,在生产门口挂上生产状态牌,并填写相应的产品名称,批号,规格,生产日期。 16.5.生产前,用标准砝码校准秘需的衡器。 17.6.在需用生产的设备,衡器上换挂上生产状态牌(正在运行) 18.7.领取所需的生产用具,工具,容器等,并确认清洁消毒在有效期内。 19.8.根据指令单,领取已处理的原辅料,并核对品名,批号。 20.(二)操作 21.1.启动称量罩5分钟,开启地秤,待显示为0.00时,将600LIBC推上地秤,并除皮(即显示 0.00),翻起过板桥并固定好。 22.2.根据指令单核对物料品名,批号,然后用提升机投料至600LIBC中,根据指令单的净重, 准确称取,即百分百投料,所有的物料以去皮方式依次投入。 23.3.所有的称量操作都必须双人复核(如核对物料品名,批号,核对投料中每种物料在地秤或电 子台秤上显示的数值,都必须与指令单上的数值一致) 24.4.称量过程中所用的容器具应每种物料一个,不得混用,以避免造成交叉污染。 25.5.批记录和管理记录必须及时填好,(不得写备忘录或回忆录) 26.6.每一锅次称量完成后,在600LIBC的状态牌上标示清楚信息,产品名称,规格,批号(次) 和数量,最后才移至下一工序。 27.7.称量结束后,剩余的物料应及时盘点,并更新物料卡信息,填写实际的物料信息。 28.8.将日落黄铝色淀、阿斯帕坦等过40目筛到600L料斗内备用。 29.9.电子称使用。
药剂学-第4章.doc
第四章固体制剂-1(散剂、颗粒剂、片剂、片剂的包衣) 固体制剂常用的固体制剂包括:散剂、颗粒剂、片剂、胶囊剂、滴丸剂、膜剂等 固体制剂的共性:(1)物理、化学稳定性比液体制剂好,生产制造成本较低,服用与携带方便;(2)制备过程前处理的单元操作经历相同;(3)药物在体内首先溶解后才能透过生理膜,被吸收入血。 固体剂型的制备工艺流程图 对于固体制剂来说 药物需溶解后才能被胃肠道所吸收,特别是对一些 口服制剂吸收的快慢顺序:溶液剂> 混悬剂> 散剂> 颗粒剂> 胶囊剂> 片剂> 丸剂 三、Noyes-Whitney方程 ●药物溶出速度可用Noyes-Whitney方程描述: dC/dt=KS (C S-C) K=D/Vδ 式中:K- 溶出速度常数S-溶出界面面积D-药物的扩散系数CS-药物的溶解度δ-扩散边界层厚C-药物的浓度V-溶出介质的量 改善药物溶出速度的措施: (1)增大药物的溶出面积(粉碎,崩解)(2)增大溶解速度常数(加强搅拌)(3)提高药物的溶解度(提高温度,改变晶型,制成固体分散物等) 粉碎技术、药物的固体分散技术、药物的包合技术等可以有效地提高药物的溶解度或溶出表面积。 对于难溶性药物提高溶出度的有效方法是: 第二节散剂 ●散剂(Powders)系指一种或数种药物均匀混合而制成的粉末状制剂,可外用也可内服。 特点:①粉碎程度大,比表面积大、易于分散、起效快;②外用覆盖面积大,可以同时发挥保护和收敛等作用;③贮存、运输、携带比较方便;④制备工艺简单,剂量易于控制,便于婴幼儿服用。此外还有飞散性、附着性、团聚性、吸湿性等特点 散剂的制备物料前处理→粉碎→过筛→混合→分剂量→质量检查→包装储存 ●固体药物的粉碎是将大块物料借助机械力破碎成适宜大小的颗粒或细粉的操作。 ●粉碎度或粉碎比(n)n = D1/D2 D1粉碎前的粒度D2粉碎后的粒度 粉碎操作的意义: ●有利于提高难溶性药物的溶出速度以及生物利用度; ●有利于各成分的混合均匀; ●有利于提高固体药物在液体、半固体、气体中的分散度; ●有助于从天然药物中提取有效成分等。 粉碎机有(1)研钵(2)球磨机(3)冲击式粉碎机(4)流能磨等 (三)筛分 筛分法(sieving method)是借助筛网孔径大小将物料进行分离的方法。筛分的目的是为了获得较均匀的粒子群(四)混合 ●混合(mixing)将两种以上组分的物质均匀混合的操作统称为混合。混合操作以含量的均匀一致为目的,是保证制剂产品质量的重要措施之一。混合度(degree of mixing)是表示物料混合均匀程度的指标。大小介于0~1之间。混合机理:对流混合、剪切混合、扩散混合。三种混合方式在实际的操作过程中并不是独立进行,而是相互联系的。 混合的影响因素
湿法制粒设备综述
湿法制粒设备综述 班级:学号:姓名: 摘要:所有的固体制剂的制备过程几乎都离不开制粒过程。而制粒方法大体分两大类,湿法制粒和干法制粒。传统的湿法制粒是目前制粒的主要方法。湿法制粒设备主要有这几种:挤压式制粒机、转动制粒机、高速搅拌制粒装置、流化床制粒机、喷雾制粒机、复合型制粒机等。 关键字:湿法制粒,湿法制粒设备,制粒机,复合型制粒设备 制粒是把粉末、熔融液、水溶液等状态的物料经加工制成具有一定形状与大小粒状物的操作。几乎所有的固体制剂的制备过程都离不开制粒过程。所制成的颗粒可能是最终产品,如颗粒剂;也可能是中间产品,如片剂。而制粒方法大体分两大类,湿法制粒和干法制粒。传统的湿法制粒是目前制粒的主要方法。 目前,常用的湿法制粒设备有挤压式制粒机、转动制粒机、高速搅拌制粒机、流化床制粒机、喷雾制粒机。此外,对制粒技术及产品的要求越来越高,为了发挥流化床制粒的优势,出现了一系列以流化床为母体的多功能的新型复合制粒设备。 1.挤压式制粒机 挤压式制粒机是将药物粉末与处方中的辅料混合均匀后加入黏合剂制软材,然后将软材用强制挤压的方式过筛制粒。这种制粒设备有螺旋挤压式、旋转式挤压式、摇摆挤压式等。 特点: 1)颗粒的大小由筛网的孔径大小调节,可制得粒径范围在0.3~30mm做左右,粒度分布较窄,粒子形状多为圆柱状、角柱状; 2)颗粒的松软程度可用不同黏合剂及其加入量调节,以适应压片的需要; 3)制粒前必须混合、制软材等,程序多、劳动强度大,不适合大批量和连续生产; 4)制备小粒径颗粒时筛网的寿命短。 在挤压制粒过程中,制软材(捏合)是关键步骤,黏合剂用量过多时软材被挤压成条状,并重新黏合在一起。黏合剂用量过少时不能制成完整的颗粒,而成粉状。因此,在制软材的过程中选择适宜黏合剂及适宜用量是非常重要的。 挤压式制粒机的工作原理以哈尔滨科海制药设备厂出售的这一款制粒机为例做介绍。 粉末物料经配料混合后,由专用加料机(气动上料或机动上料)从制粒机顶端加料口加入料仓内,送料仓上下端各配有一套料位计,根据物料加入量的变 化自动加料,粉末物料经一根横向螺旋送料浆将物料推送入另一根 枞向螺旋送料浆,将物料挤压入下部的压辊间挤压成型,当粉末物 料通过这两段横向纵向螺旋送料浆时,由于两根浆叶的直径和转速 不同,粉末与物料会形成预挤压,在物料挤压过程中,如果物料中 的空气没有经过有效的去除,那么压合成型的物料会出现开裂和破 碎,碎片和细粉量将会大大增加。故本机另配有真空脱气系统,真 空除气装置会去除物料中含有的空气量,从而有效的解决这一问题。 在某些案例中,可以使产量提高4-5倍,压合效率提高40%。挤压
零点与极点计算和分析
关于放大器极、零点与频率响应的初步实验 1.极零点的复杂性与必要性 一个简单单级共源差分对就包含四个极点和四个零点,如下图所示: 图1 简单单级共源全差分运放极零点及频率、相位响应示意图 上图为简单共源全差分运放的极零点以及频率响应的示意图,可以看到,运放共有四个极点,均为负实极点,共有四个零点,其中三个为负实零点,一个为正实零点。后面将要详细讨论各个极零点对运放的频率响应的影响。 正在设计中的折叠共源共栅运算放大器的整体极零点方针则包括了更多的极零点(有量级上的增长),如下图所示:
图2 folded-cascode with gain-boosting and bandgap all-poles details
图3 folded-cascode with gain-boosting and bandgap all-zeros details 从上述两张图可以看到,面对这样数量的极零点数量(各有46个),精确的计算是不可能的,只能依靠计算机仿真。但是手算可以估计几个主要极零点的大致位置,从而预期放大器的频率特性。同时从以上图中也可以看到,详细分析极零点情况也是很有必要的。可以看到46个极点中基本都为左半平面极点(负极
点)而仿真器特别标出有一个正极点(RHP )。由于一般放大器的极点均应为LHP ,于是可以预期这个右半平面极点可能是一个设计上的缺陷所在。(具体原因现在还不明,可能存在问题的方面:1。推测是主放大器的CMFB 的补偿或者频率响应不合适。 2。推测是两个辅助放大器的带宽或频率响应或补偿电容值不合适)其次可以从极零点的对应中看到存在众多的极零点对(一般是由电流镜产生),这些极零点对产生极零相消效应,减少了所需要考虑的极零点的个数。另外可以看到46个零点中45个为负零点,一个为正零点,这个正零点即是需要考虑的对放大器稳定性产生直接影响的零点。 以上只是根据仿真结果进行的一些粗略的分析,进一步的学习和研究还需要 进行一系列实验。 1. 单极点传输函数——RC 低通电路 首先看一个最简单的单极点系统——RC 低通电 路,其中阻值为1k ,电容为1p ,传输函数为: sRC s H +=11)( 则预计极点p0=1/(2πRC )=1.592e8 Hz ,仿真得 到结果与此相同。 而从输出点的频率响应图中可以得到以下几个结 论: 图4 一阶RC 积分电路 1)-3dB 带宽点(截止频率)就是传输函数极点,此极点对应相位约为-45°。 2)相位响应从0°移向高频时的90°,即单极点产生+90°相移。 3)在高于极点频率时,幅度响应呈现-20dB/十倍频程的特性。 图5 一阶RC 电路极点与频率响应(R=1k C=1p )
制药工艺流程
海蛤壳、厚壳贻贝抗炎清火口含片 制药工艺设计 专业:生物技术(海洋生物制药) 2015年 7 月 9 日
目录 1 工艺概述....................................................错误!未定义书签。 设计背景.................................................错误!未定义书签。 片剂特点.................................................错误!未定义书签。 海蛤壳、厚壳贻贝抗炎清火口含片特点.......................错误!未定义书签。 湿法制粒压片工艺特点.....................................错误!未定义书签。 2 处方设计及工艺流程..........................................错误!未定义书签。 处方设计.................................................错误!未定义书签。 工艺流程图...............................................错误!未定义书签。 工艺设计.................................................错误!未定义书签。 粉碎.................................................错误!未定义书签。 筛分.................................................错误!未定义书签。 混合.................................................错误!未定义书签。 制湿颗粒.............................................错误!未定义书签。 压片.................................................错误!未定义书签。 包衣.................................................错误!未定义书签。 包装.................................................错误!未定义书签。 储存.................................................错误!未定义书签。 3 物料衡算....................................................错误!未定义书签。 物料衡算概念.............................................错误!未定义书签。 基本程序.................................................错误!未定义书签。 损耗率计算...............................................错误!未定义书签。 实际年产量...............................................错误!未定义书签。 所需生产能力.............................................错误!未定义书签。 物料衡算的意义...........................................错误!未定义书签。