Thermal Width Broadening of the 0++ Glueball Spectrum near Tc
broad temperature range 温度
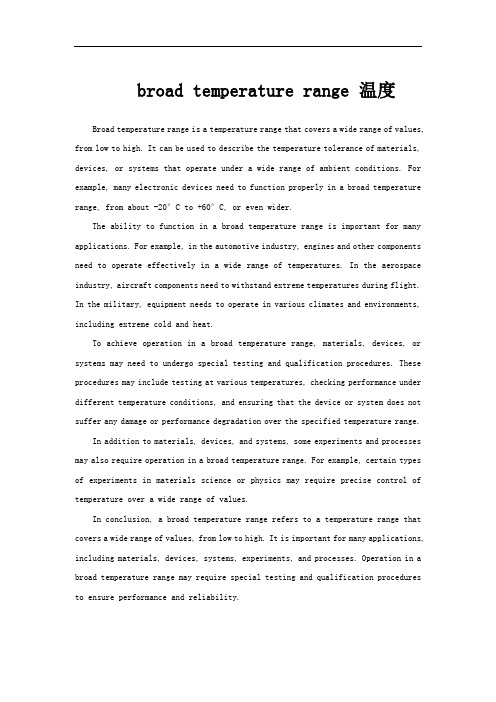
broad temperature range 温度Broad temperature range is a temperature range that covers a wide range of values, from low to high. It can be used to describe the temperature tolerance of materials, devices, or systems that operate under a wide range of ambient conditions. For example, many electronic devices need to function properly in a broad temperature range, from about -20°C to +60°C, or even wider.The ability to function in a broad temperature range is important for many applications. For example, in the automotive industry, engines and other components need to operate effectively in a wide range of temperatures. In the aerospace industry, aircraft components need to withstand extreme temperatures during flight. In the military, equipment needs to operate in various climates and environments, including extreme cold and heat.To achieve operation in a broad temperature range, materials, devices, or systems may need to undergo special testing and qualification procedures. These procedures may include testing at various temperatures, checking performance under different temperature conditions, and ensuring that the device or system does not suffer any damage or performance degradation over the specified temperature range.In addition to materials, devices, and systems, some experiments and processes may also require operation in a broad temperature range. For example, certain types of experiments in materials science or physics may require precise control of temperature over a wide range of values.In conclusion, a broad temperature range refers to a temperature range that covers a wide range of values, from low to high. It is important for many applications, including materials, devices, systems, experiments, and processes. Operation in a broad temperature range may require special testing and qualification procedures to ensure performance and reliability.。
辐射强度与波长与温度的关系 英文解释
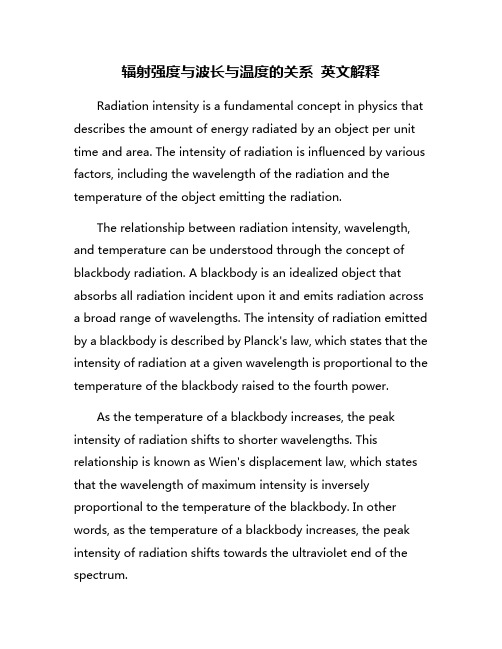
辐射强度与波长与温度的关系英文解释Radiation intensity is a fundamental concept in physics that describes the amount of energy radiated by an object per unit time and area. The intensity of radiation is influenced by various factors, including the wavelength of the radiation and the temperature of the object emitting the radiation.The relationship between radiation intensity, wavelength, and temperature can be understood through the concept of blackbody radiation. A blackbody is an idealized object that absorbs all radiation incident upon it and emits radiation across a broad range of wavelengths. The intensity of radiation emitted by a blackbody is described by Planck's law, which states that the intensity of radiation at a given wavelength is proportional to the temperature of the blackbody raised to the fourth power.As the temperature of a blackbody increases, the peak intensity of radiation shifts to shorter wavelengths. This relationship is known as Wien's displacement law, which states that the wavelength of maximum intensity is inversely proportional to the temperature of the blackbody. In other words, as the temperature of a blackbody increases, the peak intensity of radiation shifts towards the ultraviolet end of the spectrum.The Stefan-Boltzmann law describes the total power radiated by a blackbody as a function of its temperature. According to this law, the total power radiated by a blackbody is proportional to the fourth power of its temperature. This means that as the temperature of a blackbody increases, the total power radiated by the object also increases exponentially.In addition to temperature, the wavelength of radiation also plays a crucial role in determining the intensity of radiation. The relationship between wavelength and intensity is described by the inverse square law, which states that the intensity of radiation is inversely proportional to the square of the wavelength.In conclusion, the intensity of radiation is influenced by both the temperature of the object emitting the radiation and the wavelength of the radiation. As the temperature of a blackbody increases, the peak intensity of radiation shifts to shorter wavelengths, while the total power radiated by the object increases exponentially. Additionally, the intensity of radiation is inversely proportional to the square of the wavelength. These relationships between radiation intensity, wavelength, and temperature are fundamental principles in the study ofblackbody radiation and play a crucial role in various areas of physics and engineering.。
光纤通信英文版常见中英对照单词表教学内容
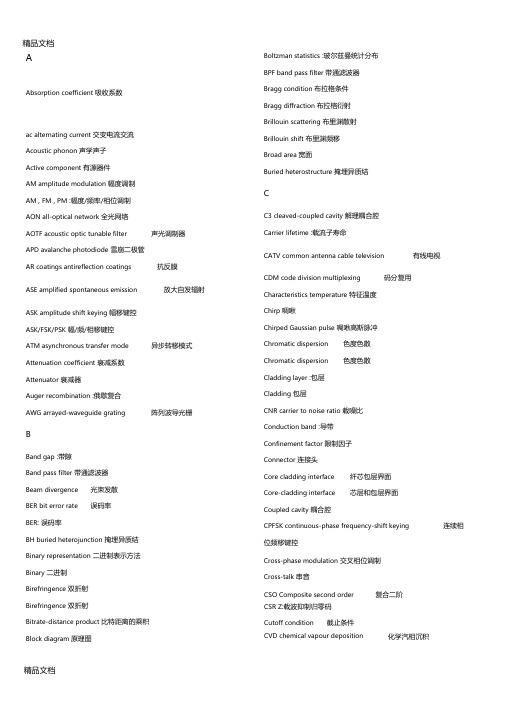
精品文档AAbsorption coefficient 吸收系数ac alternating current 交变电流交流Acoustic phonon 声学声子Active component 有源器件AM amplitude modulation 幅度调制AM , FM , PM :幅度/频率/相位调制AON all-optical network 全光网络AOTF acoustic optic tunable filter 声光调制器APD avalanche photodiode 雪崩二极管AR coatings antireflection coatings 抗反膜ASE amplified spontaneous emission 放大自发辐射ASK amplitude shift keying 幅移键控ASK/FSK/PSK 幅/频/相移键控ATM asynchronous transfer mode 异步转移模式Attenuation coefficient 衰减系数Attenuator 衰减器Auger recombination :俄歇复合AWG arrayed-waveguide grating 阵列波导光栅BBand gap :带隙Band pass filter 带通滤波器Beam divergence 光束发散BER bit error rate 误码率BER: 误码率BH buried heterojunction 掩埋异质结Binary representation 二进制表示方法Binary 二进制Birefringence 双折射Birefringence 双折射Bitrate-distance product 比特距离的乘积Block diagram 原理图Boltzman statistics :玻尔兹曼统计分布BPF band pass filter 带通滤波器Bragg condition 布拉格条件Bragg diffraction 布拉格衍射Brillouin scattering 布里渊散射Brillouin shift 布里渊频移Broad area 宽面Buried heterostructure 掩埋异质结CC3 cleaved-coupled cavity 解理耦合腔Carrier lifetime :载流子寿命CATV common antenna cable television 有线电视CDM code division multiplexing 码分复用Characteristics temperature 特征温度Chirp 啁啾Chirped Gaussian pulse 啁啾高斯脉冲Chromatic dispersion 色度色散Chromatic dispersion 色度色散Cladding layer :包层Cladding 包层CNR carrier to noise ratio 载噪比Conduction band :导带Confinement factor 限制因子Connector 连接头Core cladding interface 纤芯包层界面Core-cladding interface 芯层和包层界面Coupled cavity 耦合腔CPFSK continuous-phase frequency-shift keying 连续相位频移键控Cross-phase modulation 交叉相位调制Cross-talk 串音CSO Composite second order 复合二阶CSR Z:载波抑制归零码Cutoff condition 截止条件CVD chemical vapour deposition 化学汽相沉积CW continuous wave 连续波Cylindrical preform :预制棒DDBR distributed Bragg reflector 分布布拉格反射DBR: distributed Bragg reflector 分布式布拉格反射器精品文档dc direct current 直流DCF dispersion compensating fiber色散补偿光纤Depressed-cladding fiber: DFB distributed feedback DFB: Distributed Feedback Differential gain 微分增益 Differential quantum efficiency Diffusion 扩散Digital hierarchy 数字体系 DIP dual in line package 双列直插 Direct bandgap :直接带隙 Directional coupler定向耦合器Dispersion compensation fiber :色散补偿光纤 Dispersion decreasing fiber:色散渐减光纤Dispersion parameter :色散参数 Dispersion shifted fiber 色散位移光纤Dispersion slope 色散斜率 Dispersion slope :色散斜率 Dispersion-flatten fiber :色散平坦光纤 Dispersion-shifted fiber :色散位移光纤 Double heterojunction 双异质结 Double heterostructure :双异质结Doubly clad :双包层DPSK differential phase-shift keying 差分相移键控Driving circuit 驱动电路 Dry fiber 无水光纤 DSF dispersion shift fiber色散位移光纤DWDM dense wavelength division multiplexing/multiplexer 密集波分复用 / 器DWDM: dense wavelength division multiplexing密集波分 复用E~GEDFA erbium doped fiber amplifier掺铒光纤激光器Edge emitting LED 边发射 LED Edge-emitting 边发射 Effective index 有效折射率 Eigenvalue equation 本征值方程 Elastic scattering 弹性散射 Electron-hole pairs 电子空穴对 Electron-hole recombination 电子空穴复合 Electron-hole recombination :电子空穴复合Electrostriction 电致伸缩效应 Ethernet 以太网 External cavity 外腔External quantum efficiency 外量子效率 Extinction ratio 消光比 Eye diagram 眼图 FBG fiber-bragg grating光纤布拉格光栅FDDI fiber distributed data interface 光纤数据分配接口 FDM frequency division multiplexing 频分复用FDM :频分复用 Fermi level 费米能级 Fermi level :费米能级Fermi-Dirac distribution :费米狄拉克分布 FET field effect transistor场效应管Fiber Manufacturing :光纤制作 Field radius 模场半径Filter 滤波器Flame hydrolysis 火焰裂解FM frequency modulation 频率调制Forward-biased :正向偏置 FP Fabry Perot 法布里 - 珀落 Free spectral range 自由光谱范围 Free - space communication自由空间光通信系统Fresnel transmissivity 菲涅耳透射率 Front end 前端Furnace 熔炉FWHM full width at half maximum 半高全宽Differential-dispersion parameter 凹陷包层光纤 分布反馈 分布式反馈微分量子效率FWHM: 半高全宽FWM four-wave mixing 四波混频Gain coefficient 增益系数Gain coupled 增益耦合Gain-guided semiconductor laser 增益波导半导体激光器Germania 锗GIOF graded index optical fiber 渐变折射率分布Graded-index fiber 渐变折射率光纤Group index 群折射率精品文档 GVD group-velocity dispersion GVD: 群速度色散 H~LHBT heterojunction-bipolar transistor 异质结双极晶体管HDTV high definition television高清晰度电视Heavy doping :重掺杂 Heavy-duty cable 重型光缆Heterodyne 外差 Heterojunction :异质结 HFC hybrid fiber-coaxial混合光纤 / 电缆Higher-order dispersion 高阶色散 Highpass filter 高通滤波器 Homodyne 零差Homojunction :同质结 IC integrated circuit 集成电路 IM/DD intensity modulation with direct detection强度调制直接探测IM/DD: 强度调制 / 直接探测 IMD intermodulation distortion 交互调制失真 Impulse 冲激Impurity 杂质 Index-guided 折射率导引 Indirect bandgap :非直接带隙 Inelastic scattering 非弹性散射 Inhomogeneous 非均匀的Inline amplifier 在线放大器 Intensity noise 强度噪声 Intermodal dispersion : 模间色散 Intermode dispersion 模间色散 Internal quantum efficiency :内量子效率 Intramodal dispersion: 模内色散 Intramode dispersion 模内色散 Intrinsic absorption 本征吸收 ISDN integrated services digital network 综合业务数字网ISI intersymbol interference码间干扰Isotropic 各向同性 Jacket 涂层 Jitter 抖动 Junction :结 Kinetic energy :动能精品文档群速度色散 Lambertian source 朗伯光源LAN local-area network 局域网Large effective-area fiber 大有效面积发光Laser threshold 激光阈值 Laser 激光器 Lateral mode 侧模 Lateral 侧向Lattice constant :晶格常数 Launched power 发射功率 LD laser diode 激光二极管 LD :激光二极管LED light emitting diode 发光二极管LED: 发光二极管 L-I light current 光电关系 Light-duty cable 轻型光缆 Linewidth enhancement factor 线宽加强因子 Linewidth enhancement factor 线宽增强因子Linewidth 线宽Longitudinal mode 纵模 Longitudinal model 纵模 Lowpass filter 低通滤波器 LPE liquid phase epitaxy 液相外延 LPE :液相外延M~NMacrobending 宏弯MAN metropolitan-area network 城域网 Material dispersion 材料色散 Material dispersion:材料色散Maxwell 's equations 麦克斯韦方程组 MBE molecular beam epitaxy 分子束外延 MBE :分子束外延MCVD Modified chemical vapor deposition相沉积MCVD :改进的化学汽相沉积 Meridional rays 子午光线 Microbending 微弯 Mie scattering 米氏散射MOCVD metal-organic chemical vapor deposition机物化学汽相沉积MOCVD :改进的化学汽相沉积 Modal dispersion 模式色散改进的化学汽金属有精品文档Mode index 模式折射率Modulation format 调制格式Modulator 调制器MONET Multiwavelength optical networkMPEG motion-picture entertainment group 小组MQW: 多量子阱MSR mode-suppression ratioMSR: Mode suppression ratioMultimode fiber 多模光纤MZ mach-Zehnder 马赫泽德NA numerical aperture 数值孔径Near infrared 近红外NEP noise-equivalent powerNF noise figure 噪声指数Nonradiative recombinationNonradiative recombinationNormalized frequency 归一化频率NRZ :非归零码NSE nonlinear Schrodinger equationNumerical aperture 数值孔径Nyquist criterion 奈奎斯特准则O P QOC optical carrier 光载波OEIC opto-electronic integrated circuit 光电集成电路OOK on-off keying 开关键控OOK :通断键控OPC optical phase conjugation 光相位共轭Optical mode 光模式Optical phase conjugation 光相位共轭Optical soliton 光孤子Optical switch 光开关Optical transmitter 光发射机Optical transmitter :光发射机OTDM optical time-division multiplexing 光时分复用OVD outside-vapor deposition 轴外汽相沉积OVD :轴外汽相沉积OXC optical cross-connect 光交叉连接Packaging 封装Packet switch 分组交换Parabolic-index fiber 抛物线折射率分布光纤Passive component 无源器件PCM pulse-code modulation 脉冲编码调制PCM :脉冲编码调制PCVD :等离子体化学汽相沉积PDF probability density function 概率密度函数PDM polarization-division multiplexing 偏振复用PDM :脉冲宽度调制Phase-matching condition 相位匹配条件Phase-shifted DFB laser 相移DFB 激光器Photon lifetime 光子寿命PMD 偏振模色散Polarization controller 偏振控制器Polarization mode dispersion :偏振模色散Polarization 偏振PON passive optical network 无源接入网Population inversion :粒子数反转Power amplifier 功率放大器Power-conversion efficiency 功率转换效率PPM :脉冲位置调制Preamplifer 前置放大器PSK phase-shift keying 相移键控Pulse broadening 脉冲展宽Quantization noise 量化噪声Quantum efficiency 量子效率Quantum limit 量子极限Quantum limited 量子极限Quantum noise 量子噪声RRA raman amplifier 喇曼放大器Raman scattering 喇曼散射Rate equation 速率方程Rayleigh scattering 瑞丽散射Rayleigh scattering 瑞利散射精品文档Receiver sensitivity 接收机灵敏度Receiver 接收机Refractive index 折射率Regenerator 再生器Repeater spacing 中继距离Resonant cavity 谐振腔ResponsibilityMPN mode-partition noise 模式分配噪声MQW multiquantum well 多量子阱MSK minimum-shift keying 最小频偏键控模式分配噪声模式抑制比等效噪声功率非辐射复合:非辐射复合NRZ non-return to zero 非归零多波长光网络视频动画专家非线性薛定额方程响应度Responsivity 响应度Ridge waveguide laser 脊波导激光器Ridge waveguide 脊波导RIN relative intensity noise 相对强度噪声RMS root-mean-square 均方根RZ return-to-zero 归零RZ: 归零码SSAGCM separate absorption, grading, charge, and multiplication 吸收渐变电荷倍增区分离APD 的一种SAGM separate absorption and multiplication 吸收渐变倍增区分离APD 的一种SAM separate absorption and multiplication 吸收倍增区分离APD 的一种Sampling theorem 抽样定理SBS 受激布里渊散射SBS stimulated Brillouin scattering 受激布里渊散射SCM subcarrier multiplexing 副载波复用SDH synchronous digital hierarchy 同步数字体系SDH :同步数字体系Self-phase modulation 自相位调制Sellmeier equation :塞米尔方程Sensitivity degradation 灵敏度劣化Sensitivity 灵敏度Shot noise 散粒噪声Shot noise 散粒噪声Single-mode condition 单模条件Sintering :烧结SIOF step index optical fiber 阶跃折射率分布SLA/SOA semiconductor laser/optical amplifier 半导体光放大器SLM single longitudinal mode 单纵模SLM: Single Longitudinal mode 单纵模Slope efficiency 斜率效率SNR signal-to-noise ratio 信噪比Soliton 孤子SONET synchronized optical network 同步光网络SONET :同步光网络Spectral density :光谱密度Spontaneous emission :自发辐射Spontaneous-emission factor 自发辐射因子SRS 受激喇曼散射SRS stimulated Raman scattering 受激喇曼散射Step-index fiber 阶跃折射率光纤Stimulated absorption :受激吸收Stimulated emission :受激发射STM synchronous transport module 同步转移模块STM :同步转移模块Stripe geometry semiconductor laser 条形激光器Stripe geometry 条形STS synchronous transport signal 同步转移信号Submarine transmission system 海底传输系统Substrate: 衬底Superstructure grating 超结构光栅Surface emitting LED 表面发射LED Surface recombination :表面复合Surface-emitting 表面发射TCP/IP transmission control protocol/internet protocol 传输控制协议/ 互联网协议TDM time-division multiplexing 时分复用TDM :时分复用TE transverse electric 横电模Ternary and quaternary compound :三元系和四元系化合物Thermal equilibrium :热平衡Thermal noise 热噪声Thermal noise 热噪声Threshold current 阈值电流Timing jitter 时间抖动TM transverse magnetic 横磁Total internal reflection 全内反射Transceiver module 收发模块精品文档Transmitter 发射机Transverse 横向Transverse mode 横模TW traveling wave 行波U ~ ZVAD vapor-axial epitaxy 轴向汽相沉积VAD :轴向沉积Valence band :价带VCSEL vertical-cavity surface-emitting laser 垂直腔表面发射激光器VCSEL: vertical cavity surface-emitting lasers 垂直腔表面发射激光器VPE vapor-phase epitaxy 汽相沉积VPE :汽相外延VSB vestigial sideband 残留边带Wall-plug efficiency 电光转换效率WAN wide-area network 广域网Waveguide dispersion 波导色散Waveguide dispersion :波导色散Waveguide imperfection 波导不完善WDMA wavelength-division multiple access 波分复用接入系统WGA waveguide-grating router 波导光栅路由器White noise 白噪声XPM cross-phase modulation 交叉相位调制YIG yttrium iron garnet 钇铁石榴石晶体Zero-dispersion wavelength 零色散波长Zero-dispersion wavelength :零色散波长。
TA Instruments Thermal Diffusivity Systems安装要求说明书
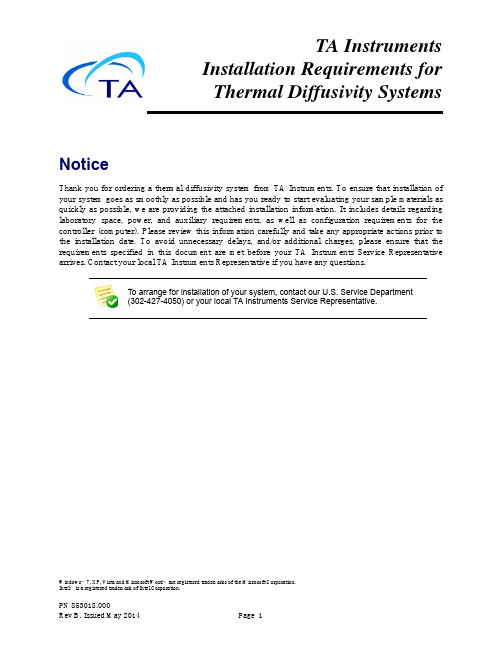
Windows® 7, XP, Vista and Microsoft Word® are registered trademarks of the Microsoft Corporation.Intel® is a registered trademark of Intel Corporation.PN 853018.000TA InstrumentsInstallation Requirements forThermal Diffusivity SystemsNoticeThank you for ordering a thermal diffusivity system from TA Instruments. To ensure that installation of your system goes as smoothly as possible and has you ready to start evaluating your sample materials as quickly as possible, we are providing the attached installation information. It includes details regarding laboratory space, power, and auxiliary requirements, as well as configuration requirements for the controller (computer). Please review this information carefully and take any appropriate actions prior to the installation date. To avoid unnecessary delays, and/or additional charges, please ensure that the requirements specified in this document are met before your TA Instruments Service Representativearrives. Contact your local TA Instruments Representative if you have any questions.To arrange for installation of your system, contact our U.S. Service Department (302-427-4050) or your local TA Instruments Service Representative.Important: TA Instruments Manual Supplement Please refer to the TA Manual Supplement to access the following important information supplemental to this document:•TA Instruments Trademarks•TA Instruments Patents•Other Trademarks•TA Instruments End-User License Agreement•TA Instruments OfficesTable of Contents Notice (1)Important: TA Instruments Manual Supplement (2)Table of Contents (3)Requirements for the Controller (Computer) (4)Hardware Considerations (4)Software Considerations (4)Requirements for the Thermal Diffusivity System Instrument (5)Instrument & Accessory Placement (5)DXF and DLF-1 Pulse Source (5)DLF-2 Pulse Source (6)EM-200 (7)EM-500 (8)EM-900 (9)EM-1200 (10)EM-1600 (11)EM-2800 (12)TA Instruments Offices (13)Requirements for the Controller (Computer)A working thermal diffusivity system consists of one or more measurement instruments (e.g., DLF-1600, DLF-2800, etc.) and a computer configured with appropriate TA Instruments software (this latter combination is subsequently referred to as a controller). The controller is included with the instrument and requires the following:Hardware Considerations•The computer should not be attached to other analytical instruments or any LAN.•The PC requires an unused RS232 serial port and an unused USB for connection to the instrument.•The PC is not permitted to run other programs or any power saving features while a test is running.•Network cards and/or certain network operation frequently interfere with the operation of the instru-ment control programs.•The PC requires a Windows 32-bit operating system.Software Considerations•Peripherals (e.g., printer) must be from the known Windows compatible list. (See Microsoft's web site at /hwtest for the most current list.)•TA Instruments is not responsible for resolving issues associated with connections to your corporate network. [See further information in the next section.]•TA Instruments is not responsible for resolving hardware/software conflicts created by the addition of third party hardware or software to the computer.Requirements for the Thermal Diffusivity System InstrumentA Thermal Diffusivity system consists of an instrument and a computer for instrument control. To obtain installation requirements for the instrument and controller, refer to the appropriate sections of this document.Instrument & Accessory PlacementSelect a location for the instrument with adequate floor space and a rigid laboratory bench that is level.Unless otherwise specified in the instrument requirements, the work space must allow 0.6 m (24 in) in front of the instrument, 0.3 m (12 in) on each side, and 0.3 m (12 in) behind the instrument. The PC must be located within 1.5 m (60 in) of the instrument, and the vacuum pump must be located within 1.2 m (48in) of the Environmental Module.DXF and DLF-1 Pulse Source Dimensions:Depth 102 cm (40 in), Width 61 cm (24 in), Height 46 cm (18 in)Weight:69 kg (155 lbs)Power requirements:Pulse Source supply voltage: 208–240 V AC 1 (rated for 10 A), 50/60 Hz 2, 3PC and peripherals: 208–240 V AC outlet (separate). Surge protection suggested.1.Supply voltages lower than indicated limit may result in a degradation of performance.2.This instrument is supplied with a power cord ~2 meters long. Ensure that the mains assigned do not also supply power to noise generating equipment nearby, such as motors, welders, transformers, etc.3.An independent heavy GROUND wire must be provided through the power hook up. Improper ground-ing may cause severe damage for which the supplier will not accept responsibility. All power strips must be fully grounded and carry the ground through to the sockets into which the computer is plugged.Laboratory conditions:Temperature: 15–35°CRelative Humidity: 5–80% (non-condensing at 15°C)The instrument should be located in a dust-free, vibration-free environment, away from exposure to direct sunlight and direct air drafts. Locate the instrument in a ventilated space (hood, etc.) if noxious gases or vapors are generated during the heating of samples.The PC must be located no more than 150 cm (60 in) from the right side of the instrument (as viewed from the front); Required space surrounding the instrument: 30 cm (12 in.) on all sides of equipmentDLF-2 Pulse Source Dimensions:Depth 25 cm (10 in), Width 30 cm (12 in), Height 114 cm (45 in)Weight:45 kg (100 lbs)Power requirements:Laser supply voltage: 208–240 V AC 1(rated for 5 A), 50/60 Hz 2, 3PC and peripherals: 208–240 V AC outlet (separate). Surge protection suggested.1.Supply voltages lower than indicated limit may result in a degradation of performance.2.This instrument is supplied with a power cord ~2 meters long. Ensure that the mains assigned do not also supply power to noise generating equipment nearby, such as motors, welders, transformers, etc.3.An independent heavy GROUND wire must be provided through the power hook up. Improper ground-ing may cause severe damage for which the supplier will not accept responsibility. All power strips must be fully grounded and carry the ground through to the sockets into which the computer is plugged.Laboratory conditions:Temperature: 15–35°CRelative Humidity: 5–80% (non-condensing at 15°C)The instrument should be located in a dust-free, vibration-free environment, away from exposure to direct sunlight and direct air drafts. Locate the instrument in a ventilated space (hood, etc.) if noxious gases or vapors are generated during the heating of samples.The PC must be located no more than 150 cm (60 in) from the right side of the instrument (as viewed from the front); Required space surrounded the instrument: 30 cm (12 in.) on all sides of equipmentDimensions:Depth 102 cm (40 in), Width 61 cm (24 in), Height 46 cm (18 in)Weight:10 kg (20 lbs)Laboratory requirements:Liquid Nitrogen: Maximum 22 psi (1.5 bar) supply pressure. Pressure exceeding22 psi will damage the instrument.Purge gas1: Nitrogen or argon (supplied by customer); preferred source: gascylinder. Minimum inlet pressure: 0.5 psi (0.03 bar); Maximum inlet pressure: 1psi (0.06 bar). Do NOT pressurize the furnace cavity.1.Improperly regulated, pulsating, or poor quality purge gas may cause irregular or erratic equipment oper-ation. Containment of exhaust is recommended if noxious or poisonous gases are released by samplewhen heated. Venting inert gases into small rooms may reduce the oxygen content of the air and become hazardous to personnel.Dimensions:Depth 102 cm (40 in), Width 61 cm (24 in), Height 77 cm (30 in)Weight:34 kg (75 lbs)Additional power requirements:Vacuum: 208–240 V AC outlet (rated for 10 A) (separate). Laboratory requirements:Liquid Nitrogen: 1 L/day in a small hand-held dewar flask, manually poured into the LN 2 receptacle.Purge gas 1: Nitrogen or argon (supplied by customer); preferred source: gas cylinder. Purge gas inlet must be filtered and dry. Minimum inlet pressure: 40 psi; Maximum inlet pressure 50 psi. Do NOT pressurize the furnace cavity.1.Improperly regulated, pulsating, or poor quality purge gas may cause irregular or erratic equipment oper-ation. Containment of exhaust is recommended if noxious or poisonous gases are released by sample when heated. Venting inert gases into small rooms may reduce the oxygen content of the air and become hazardous to personnel.Cooling water and drain:Minimum inlet pressure to the instrument: 40 psi 2; Maximum inlet pressure 80psi 3Nominal flow rate: 1–2 L/min. Varies with inlet pressurePermissible water temperature 15°C to 30°C, optimum 20°C 4, 5Air temperature/relative humidity: non-condensing at 15°C.The instrument is supplied with two hoses to connect to the Coolant Inlet and Outlet ports on the back of the furnace. The other end of each hose has a 1/4” male fitting. The coolant source must be connected to the 1/4” male fittings of each hose. The customer is required to make all hose connections.If plant-wide recirculation is used, a minimum inlet/outlet differential pressure of 50 psi is required.2.Operating close to or below minimum pressure will result in erratic operation.3.A chiller/circulator may be supplied. Consult the chiller/circulator manual for specifications concerning appropriate fluid types. The instrument is supplied with a hose to be connected between the instrument and the chiller/circulator. The customer is required to make all hose connections.4.Excessively cold water will result in “sweating” and corrosion of cooled metal surfaces. Warm water may not allow starting a test from below 25°C.5.Wall mounted supply shutoff, open drain, and city water are required if no chiller/circulator was ordered.Dimensions:Depth 102 cm (40 in), Width 61 cm (24 in), Height 77 cm (30 in)Weight:31 kg (75 lbs)Additional power requirements:Vacuum: 208–240 V AC outlet (rated for 10 A) (separate). Laboratory requirements:Liquid Nitrogen: 1 L/day in a small hand-held dewar flask, manually poured into the LN 2 receptacle.Purge gas 1: Nitrogen or argon (supplied by customer); preferred source: gas cylinder. Purge gas inlet must be filtered and dry. Minimum inlet pressure: 40 psi; Maximum inlet pressure 50 psi. Do NOT pressurize the furnace cavity.1.Improperly regulated, pulsating, or poor quality purge gas may cause irregular or erratic equipment oper-ation. Containment of exhaust is recommended if noxious or poisonous gases are released by sample when heated. Venting inert gases into small rooms may reduce the oxygen content of the air and become hazardous to personnel.Cooling water and drain:Minimum inlet pressure to the instrument: 40 psi 2; Maximum inlet pressure 80psi 3Nominal flow rate: 1–2 L/min. Varies with inlet pressurePermissible water temperature 15°C to 30°C, optimum 20°C 4, 5Air temperature/relative humidity: non-condensing at 15°C.The instrument is supplied with two hoses to connect to the Coolant Inlet and Outlet ports on the back of the furnace. The other end of each hose has a 1/4” male fitting. The coolant source must be connected to the 1/4” male fittings of each hose. The customer is required to make all hose connections.If plant-wide recirculation is used, a minimum inlet/outlet differential pressure of 50 psi is required.2.Operating close to or below minimum pressure will result in erratic operation.3.A chiller/circulator may be supplied. Consult the chiller/circulator manual for specifications concerning appropriate fluid types. The instrument is supplied with a hose to be connected between the instrument and the chiller/circulator. The customer is required to make all hose connections.4.Excessively cold water will result in “sweating” and corrosion of cooled metal surfaces. Warm water may not allow starting a test from below 25°C.5.Wall mounted supply shutoff, open drain, and city water required if no chiller/circulator was ordered.Dimensions:Depth 102 cm (40 in), Width 61 cm (24 in), Height 77 cm (30 in)Weight:34 kg (75 lbs)Laboratory requirements:Liquid Nitrogen cooling (static): 1 L/day; use a small hand held dewar flask and manually pour into the LN 2 receptacle.Purge gas 1: Nitrogen or argon (supplied by the customer); preferred source: gas cylinder. Purge gas inlet must be filtered and dry. Minimum inlet pressure: 40 psi; Maximum inlet pressure 50 psi. Do NOT pressurize the furnace cavity.The instrument is supplied with hose barbs to be connected to rubber or Tygon tubing, minimum inside tubing diameter of 3.175 mm (or equivalent), minimum pressure rating of 100 psi (7 bar). The customer is required to make all hose connections.1.Improperly regulated, pulsating, or poor quality purge gas may cause irregular or erratic instrument operation. Containment of exhaust is recommended if noxious or poisonous gases are released by sam-ple when heated. Venting inert gases into small rooms may reduce the oxygen content of the air andbecome hazardous to personnel.Cooling water and drain:Minimum inlet pressure to the instrument: 40 psi 2; Maximum inlet pressure 80psi 3Nominal flow rate: 1–2 L/min. Varies with inlet pressurePermissible water temperature 15°C to 30°C, optimum 20°C 4, 5Air temperature/relative humidity: non-condensing at 15°C.The instrument is supplied with two hoses to connect to the Coolant Inlet and Outlet ports on the back of the furnace. The other end of each hose has a 1/4” male fitting. The coolant source must be connected to the 1/4” male fittings of each hose. The customer is required to make all hose connections.If plant-wide recirculation is used, a minimum inlet/outlet differential pressure of 50 psi is required.2.Operating close to or below minimum pressure will result in erratic operation.3.A chiller/circulator may be supplied. Consult the chiller/circulator manual for specifications concerning appropriate fluid types. The instrument is supplied with a hose to be connected between the instrument and the chiller/circulator. The customer is required to make all hose connections.4.Excessively cold water will result in “sweating” and corrosion of cooled metal surfaces. Warm water may not allow starting a test from below 25°C.5.Wall mounted supply shutoff, open drain, and city water required if no chiller/circulator was ordered.Dimensions:Depth 72 cm (28 in), Width 107 cm (42 in), Height 153 cm (60 in)Weight:137 kg (300 lbs)Additional Power requirements:Main furnace supply voltage: 208–240 V AC outlet (rated for 25A), 50/60 Hz Pump: 220–240 V AC outlet (rated for 15A) (separate)Laboratory requirements:Liquid Nitrogen cooling (static): 1 L/day; use a small hand held dewar flask and manually pour into the LN 2 receptacle.Purge gas 1: Dry nitrogen or dry argon (supplied by the customer); preferred source: gas cylinder. Purge gas inlet must be filtered and dry. Minimum inlet pressure: 40 psi; Maximum inlet pressure 50 psi. Do NOT pressurize the furnace cavity.The instrument is supplied with hose barbs to be connected to rubber or Tygon tubing, minimum inside tubing diameter of 3.175 mm (or equivalent), minimum pressure rating of 100 psi (7 bar). The customer is required to make all hose connections.1.Improperly regulated, pulsating, or poor quality purge gas may cause irregular or erratic instrument operation. Containment of exhaust is recommended if noxious or poisonous gases are released by sam-ple when heated. Venting inert gases into small rooms may reduce the oxygen content of the air andbecome hazardous to personnel.Cooling water and drain:Minimum inlet pressure: 40 psi 2, 3; Maximum inlet pressure: 80 psiNominal flow rate: 1–2 L/min (varies with inlet pressure)Minimum inlet/outlet differential pressure (if plant-wide recirculation is used): 50 psiWater temperature: 15°C–30°C 4, optimum 20°CAir temperature/relative humidity: non-condensing at 15°CThe instrument is supplied with two hoses to connect to the Coolant Inlet and Outlet ports on the back of the furnace. The other end of each hose has a 1/4” male fitting. The coolant source must be connected to the 1/4” male fittings of each hose. The customer is required to make all hose connections.2.Operating close to or below minimum pressure will result in erratic operation3.Wall mounted supply shutoff, open drain, and city water required; 2 meters feed and drain hose supplied that may be used in place of hose barbs, if applicable.4.Excessively cold water may result in “sweating” and possible corrosion of cooled metal surfaces. Warm water may not permit beginning a test at 25°C or lower.Dimensions:Depth 183 cm (60 in), Width 76 cm (30 in), Height 153 cm (60 in)Weight:160 kg (350 lbs)Additional Power requirements:Main Furnace power supply voltage: 208-240 V AC (rated for 100A), 50/60 HzV oltage taps may be changed to 208V or 240V , if necessaryPump: 220–240 V AC outlet (rated for 15A) (separate)Laboratory requirements:Liquid Nitrogen: 1 L/day in a small hand-held dewar flask, manually poured into the LN 2 receptacle.Purge gas 1, 2: Argon is required (supplied by customer); preferred source: gas cylinder. Two stage regulator with second stage: 40–50 psi maximum rating required. Maximum delivery pressure: 45 psi. Operation in air or moisture bearing atmosphere is not permitted.Hose (supplied by customer): Rubber/Tygon tubing, minimum inside tubing diameter of 6 mm (or equivalent), minimum pressure rating of 100 psi (7 bar). The customer is required to make all hose connections.1.The instrument has provision for the collection of the effluent purge gas, which may be collected and piped away at the customer's option. Containment of the exhaust is recommended if noxious or poison-ous gases are released by the specimen when heated.2.Improperly regulated, pulsating, or poor quality purge gas may cause irregular or erratic equipment oper-ation. Containment of exhaust is recommended if noxious or poisonous gases are released by sample when heated. Venting inert gases into small rooms may reduce the oxygen content of the air and become hazardous to personnel.Cooling water and drain:Minimum inlet pressure to the instrument: 40 psi; Maximum inlet pressure 80 psiNominal flow rate: 4–5 L/min. Varies with inlet pressurePermissible water temperature 15°C to 30°C, optimum 20°C.Air temperature/relative humidity: non-condensing at 15°C.Vacuum (supplied by customer; required)Mechanical vacuum pump: Minimum 100 lpm, 10-3 torr (port blanked off)TA Instruments OfficesFor information on our latest products, contact information, and more, see our web site at: TA Instruments — Waters LLCCorporate Headquarters159 Lukens DriveNew Castle, DE 19720USATelephone: 302-427-4000Fax: 302-427-4001Email:**********************。
热影响区宽度的英文
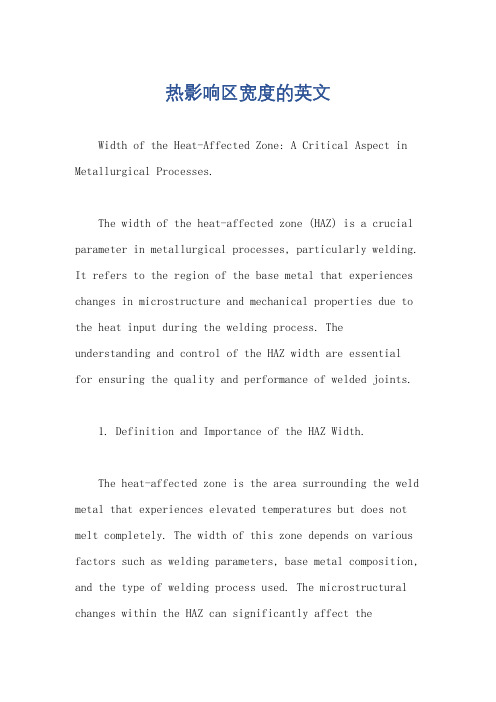
热影响区宽度的英文Width of the Heat-Affected Zone: A Critical Aspect in Metallurgical Processes.The width of the heat-affected zone (HAZ) is a crucial parameter in metallurgical processes, particularly welding. It refers to the region of the base metal that experiences changes in microstructure and mechanical properties due to the heat input during the welding process. The understanding and control of the HAZ width are essentialfor ensuring the quality and performance of welded joints.1. Definition and Importance of the HAZ Width.The heat-affected zone is the area surrounding the weld metal that experiences elevated temperatures but does not melt completely. The width of this zone depends on various factors such as welding parameters, base metal composition, and the type of welding process used. The microstructural changes within the HAZ can significantly affect themechanical properties of the welded joint, including its strength, toughness, and corrosion resistance.2. Factors Influencing the HAZ Width.Welding Parameters: The welding current, voltage, and welding speed significantly affect the heat input and, consequently, the width of the HAZ. Higher heat input results in a wider HAZ.Base Metal Composition: The chemical composition of the base metal determines its thermal conductivity, heat capacity, and phase transformation temperatures. These properties influence how the metal responds to the heat input and, therefore, the width of the HAZ.Welding Process: Different welding processes, such as gas metal arc welding (GMAW), gas tungsten arc welding (GTAW), and submerged arc welding (SAW), have different heat distributions and, thus, affect the HAZ width.3. Impact of the HAZ Width on Welded Joint Properties.Mechanical Properties: The microstructural changes within the HAZ can lead to variations in hardness, ductility, and strength. If the HAZ width is too wide, it can result in a decrease in joint strength and an increase in the risk of cracks.Corrosion Resistance: Changes in the microstructure of the HAZ can affect the corrosion resistance of the welded joint. Wider HAZs may exhibit increased susceptibility to corrosion.Fatigue Resistance: The HAZ width can influence the fatigue life of welded structures. Wider HAZs may introduce stress concentrations and reduce fatigue resistance.4. Controlling the HAZ Width.Selection of Welding Parameters: Careful selection of welding parameters can help limit the heat input and, thus, the width of the HAZ. Adjusting the welding current, voltage, and speed according to the base metal and desiredjoint properties is crucial.Preheat and Postweld Heat Treatment: The application of preheat and postweld heat treatment can control the cooling rate and minimize the risk of harmful microstructural changes within the HAZ.Use of Filler Materials: The selection of appropriate filler materials can influence the microstructural development within the HAZ and, thus, the width.5. Conclusion.The width of the heat-affected zone is a critical aspect in welding and other metallurgical processes. Understanding and controlling this parameter is essential for ensuring the quality and performance of welded joints. By considering factors such as welding parameters, base metal composition, and welding process, it is possible to optimize the HAZ width and achieve optimal welded joint properties.。
Thermal_Forming
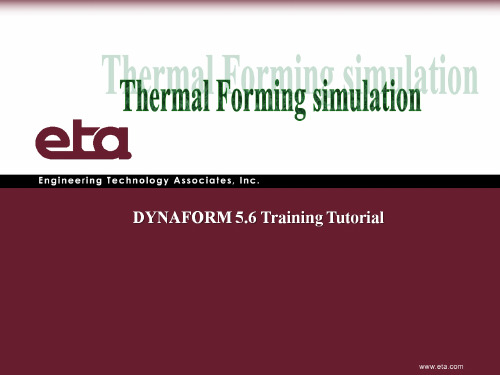
Figure 22
13
IV. Define Blank Structural Material
6. The stress/strain curve at 0 degree is imported. The result is shown in Figure 23. 7. Then Click Ok to accept the result. The first curve definition is displayed with valid. See Figure 24.
Figure 16 Figure 17
10
IV. Define Blank Structural Material
9. Repeat the above procedures (step 4-step 8) – Select LCPR-Poisson.cur as Poisson’s Ratio; – Select LCALPHA-Expansion.cur as Thermal Exp (ALPHA); – Select LCC-C.cur as the Viscous Para.C; – Select LCC-P.cur as the Viscous Para.P. – The result is shown in Figure 18.
Figure 4 Figure 6 Figure 5
4
III. Define Element Formulation
1. Click the button below Property (Figure 7) to define the element formulation as 16.Fully Integrated (Figure 8).
The interstellar medium in the edge-on galaxy NGC 5907
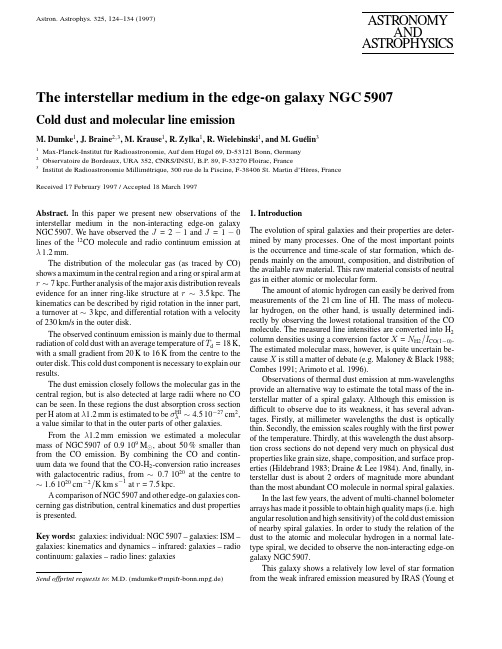
Astron.Astrophys.325,124–134(1997)ASTRONOMYANDASTROPHYSICSThe interstellar medium in the edge-on galaxy NGC5907Cold dust and molecular line emissionM.Dumke1,J.Braine2,3,M.Krause1,R.Zylka1,R.Wielebinski1,and M.Gu´e lin31Max-Planck-Institut f¨u r Radioastronomie,Auf dem H¨u gel69,D-53121Bonn,Germany2Observatoire de Bordeaux,URA352,CNRS/INSU,B.P.89,F-33270Floirac,France3Institut de Radioastronomie Millim´e trique,300rue de la Piscine,F-38406St.Martin d’H`e res,FranceReceived17February1997/Accepted18March1997Abstract.In this paper we present new observations of the interstellar medium in the non-interacting edge-on galaxyNGC5907.We have observed the J=2−1and J=1−0lines of the12CO molecule and radio continuum emission atλ1.2mm.The distribution of the molecular gas(as traced by CO)shows a maximum in the central region and a ring or spiral arm atr∼7kpc.Further analysis of the major axis distribution reveals evidence for an inner ring-like structure at r∼3.5kpc.Thekinematics can be described by rigid rotation in the inner part,a turnover at∼3kpc,and differential rotation with a velocityof230km/s in the outer disk.The observed continuum emission is mainly due to thermalradiation of cold dust with an average temperature of T d=18K,with a small gradient from20K to16K from the centre to theouter disk.This cold dust component is necessary to explain ourresults.The dust emission closely follows the molecular gas in thecentral region,but is also detected at large radii where no COcan be seen.In these regions the dust absorption cross sectionper H atom atλ1.2mm is estimated to beσHIλ∼4.510−27cm2, a value similar to that in the outer parts of other galaxies.From theλ1.2mm emission we estimated a molecularmass of NGC5907of0.9109M ,about50%smaller thanfrom the CO emission.By combining the CO and contin-uum data we found that the CO-H2-conversion ratio increaseswith galactocentric radius,from∼0.71020at the centre to ∼1.61020cm−2/K km s−1at r=7.5kpc.A comparison of NGC5907and other edge-on galaxies con-cerning gas distribution,central kinematics and dust propertiesis presented.Key words:galaxies:individual:NGC5907–galaxies:ISM–galaxies:kinematics and dynamics–infrared:galaxies–radio continuum:galaxies–radio lines:galaxiesSend offprint requests to:M.D.(mdumke@mpifr-bonn.mpg.de)1.IntroductionThe evolution of spiral galaxies and their properties are deter-mined by many processes.One of the most important pointsis the occurrence and time-scale of star formation,which de-pends mainly on the amount,composition,and distribution ofthe available raw material.This raw material consists of neutralgas in either atomic or molecular form.The amount of atomic hydrogen can easily be derived frommeasurements of the21cm line of HI.The mass of molecu-lar hydrogen,on the other hand,is usually determined indi-rectly by observing the lowest rotational transition of the COmolecule.The measured line intensities are converted into H2column densities using a conversion factor X=N H2/I CO(1−0). The estimated molecular mass,however,is quite uncertain be-cause X is still a matter of debate(e.g.Maloney&Black1988;Combes1991;Arimoto et al.1996).Observations of thermal dust emission at mm-wavelengthsprovide an alternative way to estimate the total mass of the in-terstellar matter of a spiral galaxy.Although this emission isdifficult to observe due to its weakness,it has several advan-tages.Firstly,at millimeter wavelengths the dust is opticallythin.Secondly,the emission scales roughly with thefirst powerof the temperature.Thirdly,at this wavelength the dust absorp-tion cross sections do not depend very much on physical dustproperties like grain size,shape,composition,and surface prop-erties(Hildebrand1983;Draine&Lee1984).And,finally,in-terstellar dust is about2orders of magnitude more abundantthan the most abundant CO molecule in normal spiral galaxies.In the last few years,the advent of multi-channel bolometerarrays has made it possible to obtain high quality maps(i.e.highangular resolution and high sensitivity)of the cold dust emissionof nearby spiral galaxies.In order to study the relation of thedust to the atomic and molecular hydrogen in a normal late-type spiral,we decided to observe the non-interacting edge-ongalaxy NGC5907.This galaxy shows a relatively low level of star formationfrom the weak infrared emission measured by IRAS(Young etM.Dumke et al.:The interstellar medium in the edge-on galaxy NGC5907125al.1989).Therefore it is–together with NGC4565observedby Neininger et al.(1996)–some kind of counterpart to themore active galaxies already observed in the radio continuumat mm-wavelengths:NGC891(Gu´e lin et al.1993),NGC3079(Braine et al.1997),NGC3627(Sievers et al.1994),NGC4631(Braine et al.1995),and M51(Gu´e lin et al.1995).NGC5907was observed in the HI-line twenty years ago andwas thefirst non-interacting galaxy where a galactic warp couldbe detected(Gu´e lin et al.1974;Sancisi1976).Former CO mea-surements(Sofue1994;Garc´ıa-Burillo&Gu´e lin1995)haveshown that this galaxy is relatively weak in CO.Although itsnearly edge-on orientation of i∼86◦.5does not allow us to ob-serve the emission of individual star forming regions or spiralarms in the disk,this inclination increases the column densityalong the line of sight to a significant and easier measurablevalue.Therefore NGC5907is a good candidate to study the ra-dial distribution of the dust and its correlation with the molecularand atomic hydrogen distributions.This will be done especiallyin comparison with the other non-interacting edge-on galaxiesmentioned above,namely NGC891and NGC4565.The latterone shows an even lower star formation activity than NGC5907and is also CO-weak,whereas NGC891contains a considerableamount of molecular gas and shows a relatively high level ofstar formation.These two galaxies are classified as Sb and Sbc,respectively,in contrast to the Sc-galaxy NGC5907.Furthermore edge-on galaxies are suitable to investigate thethickness of the gas and dust layer.Since NGC5907is lackingstrong star formation,one does not expect to detect a thick diskor halo in this galaxy because star formation and the existenceand structure of a gaseous halo seem to be directly connected(e.g.Dahlem et al.1995).In this paper we present our molecular line and bolometerobservations of NGC5907.The following section describes theobservations and the data reduction.Sect.3presents the resultswe obtained from the CO observations.In Sect.4we discussthe thermal dust emission.We estimate dust temperatures andabsorption cross sections,and compare the distribution of thecold dust with that of the atomic and molecular gas.From this weget some hints on how the CO-H2-conversion factor may vary ingalactic disks.In Sect.5the results for NGC5907are comparedwith those obtained for other edge-on galaxies,and thefinalsection gives a summary of our results and some concludingremarks.Table1lists some basic parameters of NGC5907which willbe used throughout this paper.2.Observations and data reductionThe observations presented here were all made with the30mtelescope of the Institut de Radio Astronomie Millim´e trique(IRAM),located on Pico Veleta(Spain).2.1.Molecular line observationsThe observations of the12CO(1−0)and the12CO(2−1)lineswere done in May1995and July1996.We used one3mm(two Table1.Some basic parameters of NGC5907Type Sc(de Vaucouleurs et al.1991)λ1.2mm centre:(this work)R.A.[1950]15h14m35s.7Dec.[1950]56◦30 37 .0Dynamical centre:(Garc´ıa-Burillo et al.1997)R.A.[1950]15h14m35s.5Dec.[1950]56◦30 43 .3v hel677km/s(Garc´ıa-Burillo et al.1997) Distance11Mpc(Sasaki1987)(1 corresponds to53pc)Pos.Angle155◦(Barnaby&Thronson1992)Incl.86.5◦(Garc´ıa-Burillo et al.1997)during the second observing period)and two1mm SIS receivers available at the30m telescope simultaneously.The receivers were tuned for image sideband rejections≥10dB(≥30dB at115GHz).The system temperatures were300-400K at 115GHz and500-700K at230GHz(in the T∗A scale).In the following we use main-beam line brightness temperatures T mb. These are converted from the antenna temperatures,corrected for atmospheric attenuation and rear spillover,T∗A,through T mb=T∗A/ηmb.The beam efficienciesηmb=B eff/F eff are0.73 and0.45for115and230GHz,respectively.The beamwidth was measured on Mars to be21 for the12CO(1−0)line and 11 for the12CO(2−1)line.The backends used consisted of two512×1MHz channelfilter banks,connected to one3mm and one1mm receiver,and an autocorrelator unit,connected to the other1mm receiver(and the other3mm receiver in July 1996).The observations were centered on the major axis of the galaxy.Adopting the central position from the bolometer ob-servations(given in Table1),we observed several points out toa projected radius of240 with12 spacings near the centre and24 spacings further out.Additionally we observed a few points above and below the major axis at distances of12 and24 from the plane.The observations were made by wobbling the subre-flector at a rate of0.5Hz between the source and a reference position located between±2 and±4 in azimuth(depending on the observing position and the orientation of the source).Some scans at larger radii were observed in the position-switching mode with on-and off-position located symmetrically around the center.Cold load calibrations were made every4-8minutes.During the CO observations we checked the pointing ac-curacy in two different ways.Firstly,we made pointing scans towards1641+399and1418+546every1-2hours.Sec-ondly,we measured(every∼2hours)small cuts perpendic-ular to the major axis at the center,consisting of three points at z=0 ,+12 ,and−12 ,and checked their symmetry,since the central point is expected to be strongest and the intensity of both off-axis points to be roughly equal.From these cuts and the pointing corrections made after each pointing scan we conclude that the mean pointing uncertainty is∼1 .5.The data reduction was done in a standard manner using the GILDAS software package.126M.Dumke et al.:The interstellar medium in the edge-on galaxy NGC59072.2.Bolometer observationsTheλ1.2mm observations were carried out in March1995withthe19-channel bolometer array developed at the Max-Planck-Institut f¨u r Radioastronomie,Bonn.The19channels are lo-cated in the centre and on the sides of two concentric regu-lar hexagons,with a spacing between two adjacent channels(beams)of20 .The central frequency and bandwidth of thebolometer are estimated to be245GHz and70GHz respec-tively(Gu´e lin et al.1995).For calibration purposes we haveobserved maps of Mars and Uranus during the bolometer obser-vations.These maps yielded a conversion factor from observedcounts to mJy/beam area of0.32mJy(beam area)−1count−1.The beamwidth at this frequency is∼10 .7.The continuum maps of NGC5907were observed in the Az-El coordinate system,with a scanning speed of4 /s in Azimuthwith data-acquisition every2 ,and a subscan separation of4in elevation.During the observations,the subreflector was wob-bled at2Hz in azimuth,with a beam throw of1 .The startingpoint of each subscan was shifted a few arcseconds in azimuthwith respect to the preceding one,which leads to a skewed shapeof each single coverage in the Az-El space,with two edges ofthe maps parallel to the major axis of the galaxy.This as wellas the use of different map sizes(between330 ×100 and 250 ×180 )was done in order to ensure that each subscan covers the galaxy and at least90 of blank sky on either side.We observed a total offifteen single maps of NGC5907,fivecentered on the optical centre(Barnaby&Thronson1992),theothers shifted164 along the major axis to the northwest andsoutheast,respectively.Since the optical centre and the centre oftheλ1.2mm emission(as found by our observations)differ bya few arcseconds,all offsets throughout this paper are relativeto the latter position which is given in Table1.During the bolometer observing session the pointing accu-racy was checked every1-2hours on1418+546.The pointingcorrections were always smaller than3 .The atmosphere wasrelatively stable and the sky opacity was∼0.2most of the time(always smaller than0.3).NGC5907was observed at relativelyhigh elevations(55◦-70◦)what reduces possible calibration er-rors,which are typically of the order15%.The data reduction was done with the MOPS software.Asecond order baseline wasfitted to each individual scan in az-imuth direction.Thefinal restoration was done applying the“mask-and-shift”restoring method,as outlined in the“PocketCookbook”(Zylka1996).3.Molecular gas in NGC59073.1.Observational results and kinematicsThe spectra along the major axis of the galaxy obtained duringthe molecular line observations are shown in Fig.1.The max-imum peak temperatures of∼0.33K and∼0.36K for theCO(1−0)and the(2−1)line respectively are reached near thecenter.CO is detected up to radii of more than200 (∼11kpc).A striking feature of many of the spectra(r≤80 )is thatthe observed lines contain at least two components,and thatthe Fig.1.Maps of the observed12CO(1−0)(left)and12CO(2−1)(right) major axis spectra of NGC5907,smoothed to a velocity resolution of 10.4km/s.The scale of the spectra is indicated by the small box at the bottom.Offsets are along the major axis,north is negativecentral spectrum shows a clear asymmetry.A natural explana-tion for the latter is that the location of the dynamical centre of the galaxy is not at x=0 (the centre of theλ1.2mm emission), but shifted by a few arcseconds to the northwest.This idea is also supported by the small asymmetry visible in the position-velocity diagrams(Fig.2).Garc´ıa-Burillo et al.(1997),who observed the central region of NGC5907using the Plateau de Bure interferometer,found the dynamical centre of the galaxy atα1950=15h14m35.s5,δ1950=56◦30 43. 3.This corresponds to(x,z)=(−6. 4,+1. 2)in our coordinates.They also found a small offset between the dynamical centre of the galaxy and theM.Dumke et al.:The interstellar medium in the edge-on galaxy NGC 5907127Fig. 2.Position-velocity diagram of the 12CO(1−0)(left)and the 12CO(2−1)(right)observations parallel to the major axis at z =0.Contour levels are -0.04(dashed),0.04,0.08,0.12,...,0.4K for both tran-sitions.The velocity resolution is 20km/s.The rms noise is variable along the major axis with a typical value of about 30mK for both transitions.The thick lines indicate the rotation curve as described in the text-200-100100200radius [arcsec]0204060d e p r o j e c t e d C O i n t e n s i t y [a .u .]-200-100100200x offset [arcsec]10203040I C O [K k m /s]Fig.3.a Observed (dashed line and filled black circles)and modelled (solid line)12CO(1−0)intensity distribution along the major axis of NGC 5907.The modelled distribution is obtained by a least-squares-fit and results from the line-of-sight-integrated radial distribution shown in b .b Adopted radial CO profile which leads to the major axis distribution as shown in aposition of the maximum CO intensity,which is in agreement with our results.The kinematics of NGC 5907can basically be described by rigid rotation up to a radius of ∼55 (which corresponds to ∼3kpc),followed by differential rotation with a rotational ve-locity of 230km /s.These values are relative to the systemic ve-locity of 677km /s and the dynamical centre given in Tab.1.The rotation curve follows from this work and the HI data (Caser-tano 1983)and is plotted as a thick line on the position-velocity diagrams (Fig.2).There are,however,a few deviations from this simple behaviour.A second line component is visible in the spectra near the central region (at |x |<30 ).Here the rigid (“normal”)rotation of the inner disk is accompanied by a high-velocity wing,with a much larger velocity gradient.This component is visible in the individual spectra as well as in the p -v -diagrams,and leads to a total line width of about 350km/s atthe assumed central position.It most probably results from non-circular motions due to a bar,as already suggested by Garc´ıa-Burillo &Gu´e lin (1995)and Garc´ıa-Burillo et al.(1997).3.2.Radial gas distributionThe observed major axis distribution of the CO intensity is the sum of the radial distribution and a projection effect (by which the emission at several radii is projected onto a certain position on the major axis).In order to deproject this distribution,we as-sumed a radial model function,consisting of a central Gaussian peak and two Gaussian rings,and fitted the resulting major axis distribution to the data.The existence of two ring-like struc-tures is suggested by two intensity maxima (at x ∼±60 and x ∼±120 )on either side of the centre in the p -v -diagrams.128M.Dumke et al.:The interstellar medium in the edge-on galaxy NGC5907Fig.4.Contour map of the continuum emission of NGC 5907at 245GHz,overlaid onto an optical image extracted from the Digitized Sky Survey.The beam size of 15 is indicated by the filled white circle in the lower right corner.The rms noise depends on the location in the map;on the galaxy it is about 1.5mJy/beam area,contour levels are 4,8,...,24mJy/beam area.The thin black lines indicate the λ1.2mm centre and the major axis of the galaxy as given in Table 1Additionally this distribution with two rings fits the data better than a distribution with just one ring (at r ∼120 ).The result can be seen in Fig.3.The best fit was obtained for ring radii of r 1=3.7kpc and r 2=7.0kpc and widths (FWHM)of Θpeak =4.4kpc,Θring1=1.4kpc,and Θring2=2.3kpc for the central peak and the inner and outer ring respectively.However,the outer “ring”may in fact be spiral arms seen more or less tangentially.4.Cold dust in NGC 59074.1.Observational resultsA contour map of the λ1.2mm continuum emission,overlaid onto an optical image extracted from the Digitized Sky Survey,is shown in Fig.4.This λ1.2mm-map is already smoothed to a beamsize of 15 to improve the signal-to-noise ratio.The emission is concentrated along a narrow ridge which follows closely the dusty optical disk,but is less extended,per-haps because of the sensitivity limit of our data.Although the emission is enhanced near the centre,there is no evidence for a nuclear point source.Several local maxima are visible along the major axis,but with some difference between the northern and the southern half.Whereas in the north there are three separatepeaks at projected radii of about 1 ,2 ,and 3.5,they seem to be somehow smeared out in the southern half,except the one atx ∼3 .5.In Fig.5we show the λ1.2mm continuum map,smoothed to a resolution of 21 HPBW,together with an HI total intensity map as received from Sancisi (m.),and the positions observed in the CO lines.NGC 5907is a really exemplary galaxy for the existence of galactic warps in neutral hydrogen (Sancisi 1976).It is,moreover,the first “normal”galaxy where a warp in the outer disk was observed.But in contrast to NGC 4565,another normal edge-on galaxy recently observed at λ1.2mm (Neininger et al.1996),no indication for a warp of the ther-mal dust emission can be seen (although the northernmost peak seems to be slightly shifted westwards with respect to the major axis).This may of course be due to the decreasing sensitivity at the outer edges of our dust map,which we reach at radii of ∼300 ,where the HI-warp is only marginally detected.4.2.ISM distributions along the major axisFig.6shows the distribution of the λ1.2mm continuum emis-sion along the major axis,together with the line intensities of the 12CO(1−0)and the HI emission.The spatial resolution of all three data sets is 21 ,as given by the 12CO(1−0)data.The continuum emission shows (more clearly in this plot than in the maps)the existence of two bright maxima at the end of the emission ridge (x ∼±200 )and of two less pronounced ones at x ∼±120 ,even if the southeastern one seems to be smeared out.Besides these similarities between the northern and the southern half the distribution is slightly asymmetric on smaller scales.The emission is detected up to radii of ∼250 in the south and even further,up to ∼280 ,in the northern half (with a significance of 2σ).Since there is λ1.2mm continuum emission beyond the edge of the CO disk,dust associated with the atomic component makes a significant contribution to the 1.2mm flux.The distribution of the CO line-intensities (and therefore the column densities of the molecular gas)shows also a maximum in the central region and decreases with increasing distance from the centre.Two further maxima are apparent at x ∼±120 .These features may be due to molecular rings and/or spiral arms in the inner part of the disk.The HI distribution shows a different behaviour.It has a minimum near the centre,increases at x ≤80 ,stays then roughly constant with several local peaks up to x ∼±200 ,and drops again further outwards.Hence this component is much more extended than the molecular gas in this galaxy.If we compare the dust emission with both gas phases,we find that it correlates with the molecular gas in the inner part ofM.Dumke et al.:The interstellar medium in the edge-on galaxy NGC5907129Fig.5.Contour maps of theλ1.2mm con-tinuum emission(left)and the HI line emis-sion(middle),as well as positions observed in the CO lines(right),all at the same scale and aligned in declination.The spatial reso-lution of the two maps is21 .Contour lev-els are3,7,12,18,25,33mJy/beam area for theλ1.2mm map and8,15,25,40,55, 701020atoms cm−2for the HI mapthe disk.At large radii,on the other hand,where CO is no longer detected,it seems to follow the HI emission.This result con-firms qualitatively that for NGC4565of Neininger et al.(1996). At smaller scales,wefind the two outer peaks in the dust emis-sion at x∼±200 corresponding to local maxima in the HI distribution,although there is a small displacement,especially on the southeastern side.From both CO peaks at x∼±120 only the northwestern one has a clear counterpart in the dust distribution,whereas in the southeastern half the dust emission shows just a small enhancement at this radius.4.3.Disk thicknessGarc´ıa-Burillo et al.(1997)estimated from their Plateau de Bure observations of the central region of NGC5907an inclina-tion of86.5◦and a thickness of the molecular disk of≤3 .In or-der to check if this is in agreement with our observations,wefit-ted the observed z-distribution(the averaged spectra are shown in Fig.7)with a Gaussian profiing the beamwidths given in Sect.2.1we determined a deconvolved thickness(FWHM) of the CO emission ridge of(13±5) ,somewhat thicker than in NGC4565(Neininger et al.1996).Additional off-axis ob-servations at x=60 have shown that this apparent thickness is nearly constant along the major axis.In order to estimate the extent of the atomic gas and the thermal dust emission perpen-dicular to the plane,we performed cuts along the minor axis of both maps.These lead to a mean beam deconvolved FWHM of the emission of(47±4) for the HI and(16±4) for the λ1.2mm emission.Since the galaxy is not perfectly seen edge-on,but under an inclination of i=86.5◦,a particular fraction of the off-axis emission is just projected from large radii to large z.We modelled this emission,using the radial CO profile obtained in Sect.3.2and a similar model,consisting of three rings,for the HI emission.The best agreement between model and data is found for a disk with a thickness(FWHM)of8 (which corresponds to∼400pc)for the CO and of28 (∼1.5kpc)for the HI.Both values seem to be unexpectedly large for a non-interacting spiral with only moderate star forming activity.We should note,however,that it is difficult to account for a warp in this simple modelling,and the results are very sen-sitive to the exact values of the inclination and the telescope beamwidth.Therefore,due to the large uncertainties,a thin molecular disk cannot be ruled out.4.4.Dust properties4.4.1.Non-dust contributions to the observedfluxUsing a ring integration method,we have determined the total flux density atλ1.2mm and found S1.2mm=605±55mJy.This value,however,cannot be attributed to thermal dust radiation alone.The broad band emission measured with the bolome-ter at245GHz rather consists of several components:thermal dust emission,free-free radiation,synchrotron radiation,and the CO(2−1)and some weaker lines.Since we are mainly in-terested in thefirst,we have to determine the contributions due to the other processes and to subtract them.The contribution of the12CO(2−1)line to the surface brightness measured with the bolometer can be calculated throughF line=2kν3c−3∆νbolΩbeam I CO(2−1)≈0.058I CO(2−1)(1) (with I CO(2−1)=lineT mb(12CO(2−1))dv in Kkms−1)for a bolometer bandwidth of70GHz and a beamwidth of11 for the continuum observations.With an assumed contribution of other isotopes and lines from other molecules of about10%of 12CO we estimate a totalflux density due to line contributions of S line=52±4mJy.The contribution of the continuum emission due to thermal and relativistic electrons is more difficult to determine since the130M.Dumke et al.:The interstellar medium in the edge-on galaxy NGC 5907300200100-100-200-300x offset [arcsec]010203040I C O [K k m /s ]SENW403020100I 1.2mm [mJy/b.a.]HI1.2 mmCO(1-0)Fig.6.The distribution of the 12CO(1−0)(thick dashed line,left scale)and the λ1.2mm continuum (thick solid line,right scale)along the major axis of NGC 5907.The HI distribution (thin solid line,arbi-trary units)is also shown.The spatial resolution in all three curves is 21radio continuum flux density at ν=1−10GHz originates partly in a double background source in the southern half of the galaxy (Hummel et al.1984;Dumke et al.1995).Furthermore the spectral behaviour of this background source is unknown,and the fraction of the galaxies’thermal emission is difficult to estimate.We used a total flux density of 47±5mJy at a frequency of 10.55GHz as derived by Dumke et al.(1995),a thermal fraction of 30%at this frequency and a nonthermal spectral index of −0.85which are typical for spiral galaxies (Niklas et al.1997)to calculate a value of S sync+ff =13±4mJy at 245GHz,which is about 2%of the total flux density.Besides these integrated values,we had to estimate the non-dust contributions along the major axis.The fraction of the CO lines,i.e.the line-to-continuum ratio,was calculated for each position from the CO(2−1)line following Eq.1and subtracted.Again we assumed that a fraction of 10%of the 12CO(2−1)-line stems from other lines in the bolometer band.For the free-free and synchrotron emission,we subtracted a fraction of 2%at each position,in accordance with the value estimated above.4.4.2.Dust temperaturesAfter subtracting the contributions of molecular lines and of synchrotron and free-free radiation,we determined a total flux density at 245GHz due to thermal dust emission ofS dust =540±60mJy .Including published IRAS flux densities (Young et al.1989)our observations allow to estimate color temperatures for the dust.We fitted a two-component modified Planck function to the data,using the points from 25µm to 1.2mm and under the assumption of a dust spectral index of 2(e.g.Chini et al.1986).The observed spectrum and the fitted curves (as well as their sum)are shown in Fig.8.The estimated temperatures for the two components to which we refer as cold and warm dust are 18K and 54K respectively.This result shows thatcoldFig.7.Averaged 12CO(1−0)and (2−1)spectra of the cuts through the centre.The portion of the spectra shown in each box ranges from v hel =300km /s to v hel =1000km /s and from T mb =−0.1K to T mb =0.4Kdust is necessary to explain the thermal continuum emission at λ1.2mm.The warmer dust alone which can be detected in the far-infrared by IRAS is not sufficient to account for the strong mm-emission and to explain our data.Although NGC 5907is a relatively inactive galaxy which does not show any signs of remarkable star forming activity (e.g.Dumke et al.1995),the dust emission is slightly enhanced at smaller galactocentric radii,and the dust may be somewhat warmer in this region.The FIR emission of NGC 5907was mapped by Wainscoat et al.(1987),using the IRAS CPC in-strument,at λλ50and 100µm with a resolution of 75 and 89 respectively.These maps were used to obtain spectra at different positions along the major axis of NGC 5907.We found that the temperature of the cold dust is somewhat higher in the central region (∼20K)than the value we got from the integrated flux densities,and drops to ∼16K at the outer disk.A similar de-crease is also found for other normal disk galaxies like NGC 891(Gu´e lin et al.1993),NGC 4565(Neininger et al.1996),or our Milky Way (Cox &Mezger 1989).。
atom-ZXXX
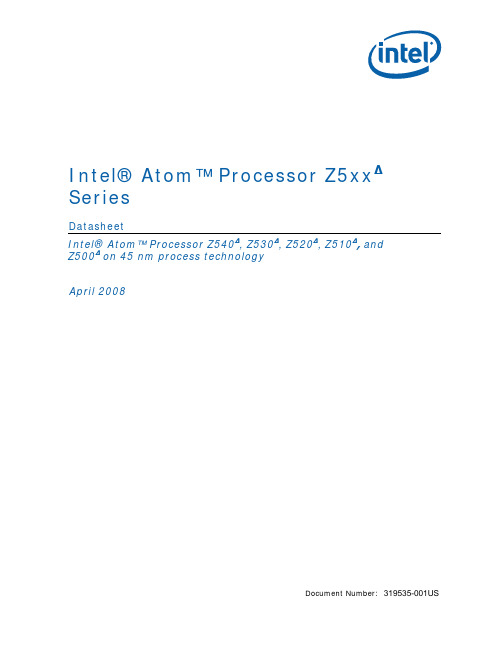
Intel® Atom™ Processor Z5xx∆SeriesDatasheetIntel® Atom™ Processor Z540∆, Z530∆, Z520∆, Z510∆, andZ500∆on 45 nm process technologyApril 2008Document Number: 319535-001USINFORMATION IN THIS DOCUMENT IS PROVIDED IN CONNECTION WITH INTEL® PRODUCTS. NO LICENSE, EXPRESS ORIMPLIED, BY ESTOPPEL OR OTHERWISE, TO ANY INTELLECTUAL PROPERTY RIGHTS IS GRANTED BY THIS DOCUMENT. EXCEPTAS PROVIDED IN INTEL'S TERMS AND CONDITIONS OF SALE FOR SUCH PRODUCTS, INTEL ASSUMES NO LIABILITYWHATSOEVER, AND INTEL DISCLAIMS ANY EXPRESS OR IMPLIED WARRANTY, RELATING TO SALE AND/OR USE OF INTELPRODUCTS INCLUDING LIABILITY OR WARRANTIES RELATING TO FITNESS FOR A PARTICULAR PURPOSE, MERCHANTABILITY,OR INFRINGEMENT OF ANY PATENT, COPYRIGHT OR OTHER INTELLECTUAL PROPERTY RIGHT. Intel products are not intendedfor use in medical, life saving, or life sustaining applications.Intel may make changes to specifications and product descriptions at any time, without notice.Designers must not rely on the absence or characteristics of any features or instructions marked “reserved” or “undefined.” Intelreserves these for future definition and shall have no responsibility whatsoever for conflicts or incompatibilities arising fromfuture changes to them.The Intel® Atom™ processor Z5xx∆ series may contain design defects or errors known as errata which may cause the product todeviate from published specifications. Current characterized errata are available on request.∆Intel processor numbers are not a measure of performance. Processor numbers differentiate features within each processorfamily, not across different processor families. Over time processor numbers will increment based on changes in clock, speed,cache, FSB, or other features, and increments are not intended to represent proportional or quantitative increases in anyparticular feature. Current roadmap processor number progression is not necessarily representative of future roadmaps. See/products/processor_number for details.Intel® Virtualization Technology requires a computer system with an enabled Intel® processor, BIOS, virtual machine monitor(VMM) and, for some uses, certain platform software enabled for it. Functionality, performance or other benefits will varydepending on hardware and software configurations and may require a BIOS update. Software applications may not becompatible with all operating systems. Please check with your application vendor.Hyper-Threading Technology (HT Technology) requires a computer system with a processor supporting Hyper-ThreadingTechnology and HT Technology enabled chipset, BIOS and operating system. Performance will vary depending on the specifichardware and software you see. See /technology/ hypertheading/ for more information including details onwhich processor supports HT Technology.Intel, Intel Atom, Intel SpeedStep, Intel Core 2 Duo, and the Intel logo are trademarks of Intel Corporation in the U. S. andother countries.*Other names and brands may be claimed as the property of others.Copyright © 2008 Intel Corporation. All rights reserved.2 DatasheetContents1Introduction (7)1.1Major Features (7)1.2Terminology (8)1.3References (10)2Low Power Features (11)2.1Clock Control and Low-power States (11)2.1.1Package/Core Low-Power State Descriptions (13)2.1.1.1Normal State (C0, C1) (13)2.1.1.1.1C1/AutoHalt Powerdown State (13)2.1.1.1.2C1/MWAIT Powerdown State (14)2.1.1.2C2 State (14)2.1.1.2.1Stop-Grant State (14)2.1.1.2.2Stop-Grant Snoop State (15)2.1.1.3C4 State (15)2.1.1.3.1Sleep State (15)2.1.1.3.2Deep Sleep State (16)2.1.1.3.3Deeper Sleep State (16)2.1.1.3.4Intel® Atom™ Processor Z5xx Series C5 (17)2.1.1.4C6 State (17)2.1.1.4.1Intel® Deep Power-Down Technology State(Package C6 State) (18)2.2Dynamic Cache Sizing (20)2.3Enhanced Intel SpeedStep® Technology (21)2.4Enhanced Low-Power States (22)2.5FSB Low Power Enhancements (23)2.5.1Split V TT (23)2.5.2CMOS Front Side Bus (23)3Electrical Specifications (25)3.1FSB, GTLREF, and CMREF (25)3.2Power and Ground Pins (25)3.3Decoupling Guidelines (26)3.3.1V CC Decoupling (26)3.3.2FSB AGTL+ Decoupling (26)3.4FSB Clock (BCLK[1:0]) and Processor Clocking (26)3.5Voltage Identification and Power Sequencing (26)3.6Catastrophic Thermal Protection (29)3.7Reserved and Unused Pins (29)3.8FSB Frequency Select Signals (BSEL[2:0]) (29)3.9FSB Signal Groups (30)3.10CMOS Asynchronous Signals (31)3.11Test Access Port (TAP) Connection (31)3.11.1Maximum Ratings (31)3.12Processor DC Specifications (32)Datasheet 33.13AGTL+ FSB Specifications (41)4Package Mechanical Specifications and Pin Information (43)4.1Package Mechanical Specifications (43)4.1.1Processor Package Weight (43)4.2Processor Pinout Assignment (45)4.3Signal Description (52)5Thermal Specifications and Design Considerations (61)5.1Thermal Specifications (64)5.1.1Thermal Diode (64)5.1.2Intel® Thermal Monitor (66)5.1.3Digital Thermal Sensor (68)5.1.4Out of Specification Detection (69)5.1.5PROCHOT# Signal Pin (69)4 DatasheetFiguresFigure 1. Thread Low-Power States (12)Figure 2. Package Low-Power States (12)Figure 3. Deep Power-Down Technology Entry Sequence (18)Figure 4. Deep Power-Down Technology Exit Sequence (18)Figure 5. Exit Latency Table (19)Figure 6. Active V CC and I CC Loadline (36)Figure 7. Deeper Sleep V CC and I CC Loadline (37)Figure 8. Package Mechanical Drawing (44)Figure 9. Pinout Diagram (Top View, Left Side) (45)Figure 10. Pinout Diagram (Top View, Right Side) (46)TablesTable 1. References (10)Table 2. Coordination of Thread Low-Power States at the Package Level/Core Level..13Table 3. Voltage Identification Definition (27)Table 4. BSEL[2:0] Encoding for BCLK Frequency (29)Table 5. FSB Pin Groups (30)Table 6. Processor Absolute Maximum Ratings (32)Table 7. Voltage and Current Specifications for Intel® Atom™ Processor Z540,Z530, Z520, and Z510 (33)Table 8. Voltage and Current Specifications for Intel® Atom™ Processor Z500 (35)Table 9. FSB Differential BCLK Specifications (38)Table 10. AGTL+/CMOS Signal Group DC Specifications (39)Table 11. Legacy CMOS Signal Group DC Specifications (40)Table 12. Open Drain Signal Group DC Specifications (40)Table 13. Pinout Arranged By Signal Name (47)Table 14. Signal Description (52)Table 15. Power Specifications for Intel® Atom™ Processor Z540, Z530, Z520,and Z510 (62)Table 16. Power Specifications for Intel® Atom™ Processor Z500 (63)Table 17. Thermal Diode Interface (65)Table 18. Thermal Diode Parameters using Transistor Model (65)Datasheet 5Revision HistoryRevision Number Description RevisionDate-001 •Initial release April 2008§6 DatasheetIntroductionDatasheet 71 IntroductionThe Intel® Atom™ processor Z5xx series is built on a new 45-nanometer Hi-k low power micro-architecture and 45 nm process technology — the first generation of low-power IA-32 micro-architecture specially designed for the new class of Mobile Internet Devices (MIDs). In the Intel® Centrino® Atom™ processor technology platform, the Intel® Atom™ processor Z5xx series supports the Intel® System Controller Hub (Intel SCH), a single-chip component design for low-power. This document contains electrical, mechanical and thermal specifications for the following processors: • Intel® Atom™ processor Z540, Z530, Z520, Z510, and Z500Note: In this document, Intel® Atom™ processor Z5xx series refers to the Intel® Atom™processor Z540, Z530, Z520, Z510, and Z500 Note: In this document, the Intel® Atom™ processor Z5xx series is referred to as“processor”. The Intel System Controller Hub (Intel SCH) is referred to as “Intel SCH”.1.1 Major FeaturesThe following list provides some processor key features:• New single-core processor for mobile devices offering enhanced performance • On die, primary 32-kB instructions cache and 24-kB write-back data cache • 100-MHz and 133-MHz Source-Synchronous front side bus (FSB) ⎯ 100 MHz: Intel® Atom™ processor Z500⎯ 133 MHz: Intel® Atom™ processor Z540, Z530, Z520, and Z510 • Supports Hyper-Threading Technology 2-threads • On die 512-kB, 8-way L2 cache • Support for IA 32-bit architecture• Intel® Virtualization Technology (Intel® VT)• Intel® Streaming SIMD Extensions 2 and 3 (Intel® SSE2 and Intel® SSE3) and Supplemental Streaming SIMD Extensions 3 (SSSE3) support • Supports new CMOS FSB signaling for reduced power • Micro-FCBGA8 packaging technologies• Thermal management support using TM1 and TM2 • FSB Lane Reversal for flexible routing • Supports C0/C1(e)/C2(e)/C4(e)• Intel® Deep Power-Down Technology (C6) • L2 Dynamic Cache Sizing• New Split-V TT support for lowest processor power state• Advanced power management features including Enhanced Intel SpeedStep® Technology• Execute Disable Bit support for enhanced securityIntroduction8 Datasheet1.2 TerminologyTerm Definition#A “#” symbol after a signal name refers to an active low signal, indicating a signal is in the active state when driven to a low level. For example,when RESET# is low, a reset has been requested. Conversely, when NMI is high, a non-maskable interrupt has occurred. In the case of signals where the name does not imply an active state but describes part of a binarysequence (such as address or data), the “#” symbol implies that the signal is inverted. For example, D[3:0] = “HLHL” refers to a hex ‘A’, and D[3:0]# = “LHLH” also refers to a hex “A” (H= High logic level, L= Low logic level).Front Side Bus (FSB) Refers to the interface between the processor and system core logic (also known as the Intel SCH component).AGTL+Advanced Gunning Transceiver Logic. Used to refer to Assisted GTL+ signaling technology on some Intel processors.CMOS Complementary metal-Oxide semiconductor.Storage ConditionsRefers to a non-operational state. The processor may be installed in aplatform, in a tray, or loose. Processors may be sealed in packaging or exposed to free air. Under these conditions, processor landings should not be connected to any supply voltages, have any I/Os biased, or receive any clocks. Upon exposure to “free air” (i.e., unsealed packaging or a device removed from packaging material) the processor must be handled in accordance with moisture sensitivity labeling (MSL) as indicated on the packaging material.Enhanced Intel SpeedStep® Technology Technology that provides power management capabilities to low power devices.Processor Core Processor core die with integrated L1 and L2 cache. All AC timing and signal integrity specifications are at the pads of the processor core. Intel®Virtualization Technology (Intel® VT) Processor virtualization which when used in conjunction with VirtualMachine Monitor software enables multiple, robust independent software environments inside a single platform. TDP Thermal Design PowerV CCThe processor core power supplyVR Voltage Regulator V SSThe processor groundIntroductionDatasheet 9Term DefinitionV CC HFM V CC at Highest Frequency Mode (HFM). V CC LFM V CC at Lowest Frequency Mode (LFM). V CC ,BOOT Default V CC Voltage for Initial Power Up. V CCP AGTL+ Termination Voltage. V CCPC6 AGTL+ Termination Voltage. V CCA PLL Supply voltage.V CCDPPWDN V CC at Deep Power-Down Technology (C6). V CCDPRSLP V CC at Deeper Sleep (C4). V CCF Fuse Power Supply.I CCDESI CCDES for Intel® Atom™ processor Z5xx series Recommended DesignTarget power delivery (Estimated).I CC I CC for Intel® Atom™ processors Z5xx series is the number that can be use as a reflection on battery life estimates. I AH, I CC Auto-Halt I SGNT I CC Stop-Grant. I DSLP I CC Deep Sleep.dI CC /dt V CC Power Supply Current Slew Rate at Processor Package Pin (Estimated). I CCA I CC for V CCA Supply. P AH Auto Halt Power. P SGNT Stop Grant Power. P DPRSLP Deeper Sleep Power.P DC6 Deep Power-Down Technology (C6). T JJunction Temperature.Introduction10 Datasheet1.3 ReferencesMaterial and concepts available in the following documents may be beneficial when reading this document.Table 1. ReferencesDocumentDocumentNumber/Location Intel® System Controller Hub (Intel® SCH) Datasheet 319537 Intel® Atom™ Processor Z5xx Series Specification Update 319538 Intel® 64 and IA-32 Architectures Software Developer's ManualsVolume 1: Basic ArchitectureVolume 2A: Instruction Set Reference, A-M Volume 2B: Instruction Set Reference, N-Z Volume 3A: System Programming GuideVolume 3B: System Programming Guide/pr oducts/processor/manual s/index.htmAP-485, Intel® Processor Identification and CPUID Instruction Application Note/de sign/processor/applnots/241618.htm§2Low Power Features2.1Clock Control and Low-power StatesThe processor supports low power states at the thread level and the core/packagelevel. Thread states (TCx) loosely correspond to ACPI processor power states (Cx). Athread may independently enter the TC1/AutoHALT, TC1/MWAIT, TC2, TC4 or TC6 lowpower states, but this does not always cause a power state transition. Only when boththreads request a low-power state (TCx) greater than the current processor state willa transition occur. The central power management logic ensures the entire processorenters the new common processor power state. For processor power states higherthan C1, this would be done by initiating a P_LVLx (P_LVL2 and P_LVL3) I/O read tothe chipset by both threads. Package states are states that require externalintervention and typically map back to processor power states. Package states forprocessor include Normal (C0, C1), Stop Grant and Stop Grant Snoop (C2), DeeperSleep (C4), and Deep Power-Down Technology (C6).The processor implements two software interfaces for requesting low power states:MWAIT instruction extensions with sub-state hints and P_LVLx reads to the ACPIP_BLK register block mapped in the processor’s I/O address space. The P_LVLx I/Oreads are converted to equivalent MWAIT C-state requests inside the processor and donot directly result in I/O reads on the processor FSB. The monitor address does notneed to be setup before using the P_LVLx I/O read interface. The sub-state hints usedfor each P_LVLx read can be configured in a software programmable MSR by BIOS. Ifa thread encounters a chipset break event while STPCLK# is asserted, then it assertsthe PBE# output signal. Assertion of PBE# when STPCLK# is asserted indicates tosystem logic that individual threads should return to the C0 state and the processorshould return to the Normal state.Figure 1 shows the thread low-power states. Figure 2 shows the package low-powerstates. Table 2 provides a mapping of thread low-power states to package low powerstates.Figure 1. Thread Low-Power StatesFigure 2. Package Low-Power StatesTable 2. Coordination of Thread Low-Power States at the Package Level/Core LevelNOTE:1 AutoHALT or MWAIT/C1.NOTE:To enter a package/core state, both threads must share a common low power state. If the threads are not in a common low power state, the package state will resolve to thehighest common power C state.2.1.1Package/Core Low-Power State DescriptionsThe following state descriptions assume that both threads are in a common low powerstate. For cases when only one thread is in a low power state no change in powerstate will occur.2.1.1.1Normal State (C0, C1)This is the normal operating state for the processor. The processor remains in theNormal state when the processor/core is in the C0, C1/AutoHALT, or C1/MWAIT state.C0 is the active execution state.2.1.1.1.1C1/AutoHalt Powerdown StateC1/AutoHALT is a low-power state entered when one thread executes the HALTinstruction while the other is in the TC1 or greater thread state. The processor willtransition to the C0 state upon occurrence of SMI#, INIT#, LINT[1:0] (NMI, INTR), orFSB interrupt messages. RESET# will cause the processor to immediately initializeitself.A System Management Interrupt (SMI) handler will return execution to either Normalstate or the AutoHALT Powerdown state. See the Intel® 64 and IA-32 ArchitecturesSoftware Developer's Manuals, Volume 3A/3B: System Programmer's Guide for moreinformation.The system can generate a STPCLK# while the processor is in the AutoHALTPowerdown state. When the system de-asserts the STPCLK# interrupt, the processorwill return to the HALT state.While in AutoHALT Powerdown state, the processor will process bus snoops. Theprocessor will enter an internal snoopable sub-state (not shown in Figure 1) to processthe snoop and then return to the AutoHALT Powerdown state.2.1.1.1.2C1/MWAIT Powerdown StateC1/MWAIT is a low-power state entered when one thread executes the MWAIT(C1)instruction while the other thread is in the TC1 or greater thread state. Processorbehavior in the MWAIT state is identical to the AutoHALT state except that Monitorevents can cause the processor to return to the C0 state. See the Intel® 64 and IA-32Architectures Software Developer's Manuals, Volume 2A: Instruction Set Reference, A-M and Volume 2B: Instruction Set Reference, N-Z, for more information.2.1.1.2C2 StateIndividual threads of the dual-threaded processor can enter the TC2 state by initiatinga P_LVL2 I/O read to the P_BLK or an MWAIT(C2) instruction. Once both threads haveC2 as a common state, the processor will transition to the C2 state; however, theprocessor will not issue a Stop-Grant Acknowledge special bus cycle unless theSTPCLK# pin is also asserted by the chipset.While in the C2 state, the processor will process bus snoops. The processor will entera snoopable sub-state described the following section (and shown in Figure 1), toprocess the snoop and then return to the C2 state.2.1.1.2.1Stop-Grant StateWhen the STPCLK# pin is asserted, each thread of the processors enters the Stop-Grant state within 1384 bus clocks after the response phase of the processor-issuedStop-Grant Acknowledge special bus cycle. When the STPCLK# pin is de-asserted, thecore returns to its previous low-power state.Since the AGTL+ signal pins receive power from the FSB, these pins should not bedriven (allowing the level to return to V CCP) for minimum power drawn by thetermination resistors in this state. In addition, all other input pins on the FSB shouldbe driven to the inactive state.RESET# causes the processor to immediately initialize itself, but the processor willstay in Stop-Grant state. When RESET# is asserted by the system, the STPCLK#,SLP#, DPSLP#, and DPRSTP# pins must be de-asserted prior to RESET# de-assertion.When re-entering the Stop-Grant state from the Sleep state, STPCLK# should be de-asserted after the de-assertion of SLP#.While in Stop-Grant state, the processor will service snoops and latch interruptsdelivered on the FSB. The processor will latch SMI#, INIT#, and LINT[1:0] interruptsand will service only one of each upon return to the Normal state.The PBE# signal may be driven when the processor is in Stop-Grant state. PBE# willbe asserted if there is any pending interrupt or Monitor event latched within theprocessor. Pending interrupts that are blocked by the EFLAGS.IF bit being clear willstill cause assertion of PBE#. Assertion of PBE# indicates to system logic that theentire processor should return to the Normal state.A transition to the Stop-Grant Snoop state occurs when the processor detects a snoopon the FSB (see Section 2.1.1.2.2). A transition to the Sleep state (seeSection 2.1.1.3.1) occurs with the assertion of the SLP# signal.2.1.1.2.2Stop-Grant Snoop StateThe processor responds to snoop or interrupt transactions on the FSB while in Stop-Grant state by entering the Stop-Grant Snoop state. The processor will stay in thisstate until the snoop on the FSB has been serviced (whether by the processor oranother agent on the FSB) or the interrupt has been latched. The processor returns tothe Stop-Grant state once the snoop has been serviced or the interrupt has beenlatched.2.1.1.3C4 StateIndividual threads of the processor can enter the C4 state by initiating a P_LVL4 I/Oread to the P_BLK or an MWAIT(C4) instruction. Attempts to request C3 will alsocovert to C4 requests. If both processor threads are in C4, the central powermanagement logic will request that the entire processor enter the Deeper Sleeppackage low-power state using the sequence through the Sleep and Deep Sleep statesall described in the following sections.To enable the package level Intel Enhanced Deeper Sleep state, Dynamic Cache Sizingand Intel Enhanced Deeper Sleep state fields must be configured in thePMG_CST_CONFIG_CONTROL MSR. Refer to Section 2.1.1.3.3 for further details onIntel Enhanced Deeper Sleep state.2.1.1.3.1Sleep StateThe Sleep state is a low-power state in which the processor maintains its context,maintains the phase-locked loop (PLL), and stops all internal clocks. The Sleep state isentered through assertion of the SLP# signal while in the Stop-Grant state and is onlya transition state for Intel Atom™ processor Z5xx series. The SLP# pin should only beasserted when the processor is in the Stop-Grant state. SLP# assertion while theprocessor is not in the Stop-Grant state is out of specification and may result inunapproved operation.In the Sleep state, the processor is incapable of responding to snoop transactions orlatching interrupt signals. No transitions or assertions of signals (with the exception ofSLP#, DPSLP#, or RESET#) are allowed on the FSB while the processor is in Sleepstate. Snoop events that occur while in Sleep state or during a transition into or out ofSleep state will cause unpredictable behavior. Any transition on an input signal beforethe processor has returned to the Stop-Grant state will result in unpredictablebehavior.If RESET# is driven active while the processor is in the Sleep state, and held active asspecified in the RESET# pin specification, then the processor will reset itself, ignoringthe transition through Stop-Grant state. If RESET# is driven active while the processoris in the Sleep state, the SLP# and STPCLK# signals should be de-assertedimmediately after RESET# is asserted to ensure the processor correctly executes theReset sequence.While in the Sleep state, the processor is capable of entering an even lower powerstate, the Deep Sleep state, by asserting the DPSLP# pin (see Section 2.1.1.3.2).While the processor is in the Sleep state, the SLP# pin must be de-asserted if anotherasynchronous FSB event needs to occur.2.1.1.3.2Deep Sleep StateThe Deep Sleep state is entered through assertion of the DPSLP# pin while in theSleep state and is also only a transition state for the Intel® Atom™ processor Z5xxseries. BCLK may be stopped during the Deep Sleep state for additional platform levelpower savings. As an example, BCLK stop/restart timings on appropriate chipset-based platforms with the CK540 clock chip are as follows:•Deep Sleep entry: the system clock chip may stop/tristate BCLK within 2 BCLKsof DPSLP# assertion. It is permissible to leave BCLK running during Deep Sleep.•Deep Sleep exit: the system clock chip must start toggling BCLK within 10 BCLKperiods within DPSLP# de-assertion.To re-enter the Sleep state, the DPSLP# pin must be de-asserted. BCLK can be re-started after DPSLP# de-assertion as described above. A period of 15 microseconds(to allow for PLL stabilization) must occur before the processor can be considered tobe in the Sleep state. Once in the Sleep state, the SLP# pin must be de-asserted tore-enter the Stop-Grant state.While in Deep Sleep state, the processor is incapable of responding to snooptransactions or latching interrupt signals. No transitions of signals are allowed on theFSB while the processor is in Deep Sleep state. When the processor is in Deep Sleepstate, it will not respond to interrupts or snoop transactions. Any transition on aninput signal before the processor has returned to Stop-Grant state will result inunpredictable behavior.2.1.1.3.3Deeper Sleep StateThe Deeper Sleep state is similar to the Deep Sleep state, but further reduces corevoltage levels. One of the potential lower core voltage levels is achieved by enteringthe base Deeper Sleep state. The Deeper Sleep state is entered through assertion ofthe DPRSTP# pin while in the Deep Sleep state. The following lower core voltage levelis achieved by entering the Intel Enhanced Deeper Sleep state which is a sub-state ofDeeper Sleep state. Intel Enhanced Deeper Sleep state is entered through assertion ofthe DPRSTP# pin while in the Deep Sleep only when the L2 cache has been completelyshut down. Refer to Section 2.1.1.3.4 for further details on reducing the L2 cache andentering Intel Enhanced Deeper Sleep state.In response to entering Deeper Sleep, the processor drives the VID codecorresponding to the Deeper Sleep core voltage on the VID[6:0] pins.Exit from Deeper Sleep or Intel Enhanced Deeper Sleep state is initiated by DPRSTP#de-assertion when the core requests a package state other than C4 or the corerequests a processor performance state other than the lowest operating point.2.1.1.3.4Intel® Atom™ Processor Z5xx Series C5As mentioned previously in this document, each C state has latency and transitorypower costs associated with entering/exiting idle states. When the processor isinterrupted, it must awake to service some request(s). If these requests occur at ahigh frequency, it is possible that more power will be consumed entering/exiting thestates than will be saved. To alleviate this concern, the Intel Atom™ processor Z5xxseries implements a new state called ‘Intel Atom™ processor Z5xx series C5’. TheIntel Atom™ processor Z5xx series C5 is not exposed to software. The only way toenter the C5 state is using a hardware promotion of C4 (with the cache ways shrunkto zero).When the processor is in C4, the chipset assumes the processor has data in its cache.Often, the processor has fully flushed its cache. To avoid waking up the processor toservice snoops when there is no data in its caches, the processor will automaticallypromote C4 requests to C5 (when the cache is flushed). The chipset treats C5 as anon-snoopable state. Therefore, all snoops will be completed from the I/O DMAmasters without waking up the processor.While similar, the Intel Atom™ processor Z5xx series C5 differs from the Core 2 DuoT5000/T7000 C5 implementation. In the Intel Atom™ processor Z5xx series C5, theV CC will not be powered below the retention of caches voltage; there is no need toinitialize the processor’s caches on a C5 exit, and C5 is not architecturally enumeratedto software. This state is the same as the Intel Atom™ processor Z5xx series’s C5. 2.1.1.4C6 StateC6 is a new low power state being introduced on the Intel Atom™ processor Z5xxseries. C6 behavior is the same as Intel Enhanced Deeper Sleep with the addition ofan on-die SRAM. This memory saves the processor state allowing the processor tolower its main core voltage closer to 0 V. It is important to note that V CC cannot belower while only 1 thread is in C6 state.The processor threads can enter the C6 state by initiating a P_LVL6 I/O read to theP_BLK or an MWAIT(C6) instruction. To enter C6, the processor’s caches must beflushed. The primary method to enter C6 used by newer operating systems (thatsupport MWAIT) will be through the MWAIT instruction.When the thread enters C6, it saves the processor state that is relevant to theprocessor context in an on-die SRAM that resides on a separate power plane V CCP (I/Opower supply). This allows the core V CC to be lowered to any arbitrary voltageincluding 0 V. The microcode performs the save and restore of the processor state onentry and exit from C6 respectively.To improve the amount of power reduction possible in the Deep Power-DownTechnology state, a split V TT is implemented. See Section2.5.1 for additionalinformation.。
- 1、下载文档前请自行甄别文档内容的完整性,平台不提供额外的编辑、内容补充、找答案等附加服务。
- 2、"仅部分预览"的文档,不可在线预览部分如存在完整性等问题,可反馈申请退款(可完整预览的文档不适用该条件!)。
- 3、如文档侵犯您的权益,请联系客服反馈,我们会尽快为您处理(人工客服工作时间:9:00-18:30)。
a r X i v :h e p -l a t /0304010v 1 16 A p r 20031Thermal Width Broadening of the 0++Glueball Spectrum near T cNoriyoshi Ishii 1,Hideo Suganuma 2,and Hideo Matsufuru 31RadiationLaboratory,RIKEN (The Institute of Physical and Chemical Research),2–1Hirosawa,Wako,Saitama 351–0198,Japan 2Faculty of Science,Tokyo Institute of Technology,2–12–1Ohkayama,Meguro,Tokyo 152–8552,Japan 3Yukawa Institute for Theoretical Physics,Kyoto University,Kitashirakawa-Oiwake,Sakyo,Kyoto 606–8502,Japan We study the 0++glueball correlator constructed with SU(3)anisotropic quenched lattice QCD at various temperature taking into account the possible existence of the thermal width in the ground-state peak.For this purpose,we adopt the Breit-Wigner ansatz,analysing the lattice data obtained with 5,500–9,900gauge configurations at each T .The results indicate the significant thermal width broadening as Γ(T c )∼300MeV with a reduction in the peak center as ∆ω0(T c )∼100MeV in the vicinity of the critical temperature T c .§1.Introduction At finite temperature/density,QCD changes its vacuum structure such as the reduction of the string tension,the partial chiral restoration,etc,even below the critical temperature T c of the QCD phase transition.These changes are expected to affect the hadrons leading to the changes in the various hadronic properties,since hadrons are composite particles consisting of quarks and gluons.The hadronic pole-mass shifts are thus considered to serve as the important precritical phenomena of the QCD phase transition near the critical temperature T c ,and have been exten-sively studied by using various QCD-motivated low-energy effective theories.1),2),3),4)These studies suggest the pole-mass reductions of charmoniums,light q ¯q mesons and the glueball near the critical temperature.As for the lattice QCD,it has been more popular to study the spatial correla-tions (the screening mass)than the temporal correlations (the pole-mass)at finite temperature.This is because the temporal extension of the lattice is subject to 1/T .Accordingly,number of the temporal lattice data decreases at finite temperature,which used to be the main obstacle for the lattice QCD to measure the hadronic pole-mass.Recently,by using the anisotropic lattice,which has a finer lattice spac-ing in the temporal direction than in the spatial direction,the accurate measurement of the pole-mass with the lattice QCD has become possible at finite temperature.5),6)Quenched-level Monte Carlo calculations reveal the profound results that the pole-masses of the q ¯q mesons are almost unchanged from their zero-temperature values in the confinement phase,6),7)while the pole-mass of the 0++glueball shows the 300MeV reduction near the critical temperature.8)Although these thermal effects are weaker than anticipated by the effective model studies,these tendencies are consis-tent with the recent quenched-level lattice studies on the screening mass at finitetypeset using P T P T E X.cls Ver.0.892N.Ishii,H.Suganuma and H.Matsufuru temperature.9),10)In theseanalysis,thebound-state peaks in the spectral function are assumed to be narrow enough.However,at finite temperature,each bound-state acquires the thermal width through the interaction with the heat bath.The ther-mal width is expected to grow up with temperature,which may lead to a possible collapse of the narrow-peak assumption in some cases.In this paper,we first dis-cuss what is the expected consequence of the thermal width broadening.Then,we propose the Breit-Wigner ansatz for the fit-function for the temporal correlator at finite temperature.We finally show the results of the Breit-Wigner analysis of the 0++glueball correlator at finite temperature.11)§2.The Breit-Wigner AnsatzWe begin by considering the temporal correlator G (τ)≡Z (β)−1Tr e −βH φ(τ)φ(0)and its spectral representation asG (τ)= ∞−∞dω2sinh(βω/2)ρ(ω),(2.1)where H denotes the QCD Hamiltonian,Z (β)≡Tr(e −βH )the partition func-tion,φ(τ)is the zero-momentum projected glueball operator 8)represented in the imaginary-time Heisenberg picture as φ(τ)≡e τH φ(0)e −τH .Here,ρ(ω)denotes the spectral functionρ(ω)≡ m,n| n |φ|m |22sinh(βω/2).(2.4)Thermal Width Broadening of the0++Glueball Spectrum near T c3 Here,2sinh(βω/2)in the denominator works as a biased factor,which enhances the smallerωregion while suppressing the largerωregion.Consequently,the pole-mass m(T),which is approximated with the peak position of W(ω),is smaller than the peak centerω0of the spectral functionρ(ω),i.e.,the observed hadron“mass”.11) What is the appropriate functional form of thefit-function?Tofind this,we consider the retarded Green function G R(ω).At T=0,bound-state poles of G R(ω)are located on the realω-axis.At T>0,bound-state poles are moving into the complex ω-plane with increasing temperature.Suppose that a bound-state pole is located at ω=ω0−iΓasG R(ω)=AπIm 1πǫ2πcosh(ω(β/2−τ))N c 1N cγG i,j≤3ReTr(1−P i4(s)),(3.1)where Pµν(s)∈SU(3)denotes the plaquette operator in theµ-ν-plane.The lattice parameter and the bare anisotropic parameter arefixed asβlat≡2N c/g2=6.25and4N.Ishii,H.Suganuma and H.MatsufuruγG=3.2552,respectively,so as to reproduce renormalized anisotropyξ≡a s/a t= 4.5),6)These parameter set reproduces a−1s=2.341(16)GeV and a−1t=9.365(66) GeV,where the scale unit is introduced from the on-axis data of the static inter-√quark potential with the string tensionThermal Width Broadening of the0++Glueball Spectrum near T c5 ate one.Above T c,however,the particle spectrum is expected to change drastically, and a proper assumption on the spectral function becomes less trivial.In this re-spect,it is interesting to apply the recently developed maximum entropy method (MEM),13),14)which requires no assumption on the shape of the spectral function.Comparing our results8),11)with the related lattice QCD results,6),7)the quenched lattice QCD indicates that no significant thermal effects on the pole-mass have been so far observed in the q¯q sector,6),7)whereas a significant thermal effects can be ob-served in the glueball sector.These behaviors are consistent with the thermal effects on the screening mass.9),10)To discuss the experimental observability,it is important to estimate the effects of the dynamical quarks.One of the most significant contributions of the light dynamical quarks in unquenched QCD is the“string breaking”phenomenon,i.e.,the light dynamical quark screens the coloredflux and the longflux tubes are expected to be broken into pieces.The string breaking thus affects the long distance behavior of the inter-particle interactions,which could lead to a possible changes in the structures of hadrons in quenched QCD.In the case of the0++glueball,since its size is known to be rather compact as R≃0.4fm,8),11)such changes of the glueball structure is expected to be less significant.As for the mixing effect with the0++q¯q mesons in unquenched QCD,a recent unquenched lattice QCD study shows that there is no quark mass dependence on the glueball mass,which suggests that the mixing is actually rather small.However,it is in principle desirable to estimate the size of this mixing explicitly,which is left for the future studies.AcknowledgmentsThe lattice QCD Monte Carlo calculations have been performed partly on NEC-SX5at Osaka University and partly on HITACHI SR8000at KEK.References1)T.Hatsuda,and T.Kunihiro,Phys.Rev.Lett.55(1985),158.2)T.Hashimoto,K.Hirose,T.Kinki,and O.Miyamura,Phys.Rev.Lett.57(1986),2123.3)T.Hatsuda,Y.Koike,and S.-H.Lee,Phys.Rev.D47(1993),1225.4)H.Ichie,H.Suganuma,and H.Toki,Phys.Rev.D52(1995),2944.5)T.R.Klassen,Nucl.Phys.B533(1998),557.6)QCD-Taro Collaboration,P.de Forcrand,Phys.Rev.D63(2001),054501.7)T.Umeda,R.Katayama,O.Miyamura,and H.Matsufuru,Int.J.Mod.Phys.A16(2001),2215.8)N.Ishii,H.Suganuma,and H.Matsufuru,Phys.Rev.D66(2002),014507.9) ermann,and P.Schmidt,Eur.Phys.J.C20(2001),541.10)S.Datta,and S.Gupta,hep-lat/0208001.11)N.Ishii,H.Suganuma,and H.Matsufuru,Phys.Rev.D66(2002),094506.12) C.J.Morningstar,and M.Peardon,Phys.Rev.D66(1999),034509.and referencestherein.13)M.Asakawa,T.Hatsuda,and Y.Nakahara,Prog.Part.Nucl.Phys.46(2001),459.14)M.Asakawa,T.Hatsuda,and Y.Nakahara,hep-lat/0208059.15) A.Hart,and M.Teper,Phys.Rev.D65(2002),034502.。