Zircon U-Pb and Hf isotope constraints on the Mesozoic tectonics and crustal evolution
锆石U_Pb同位素定年的原理_方法及应用_高少华
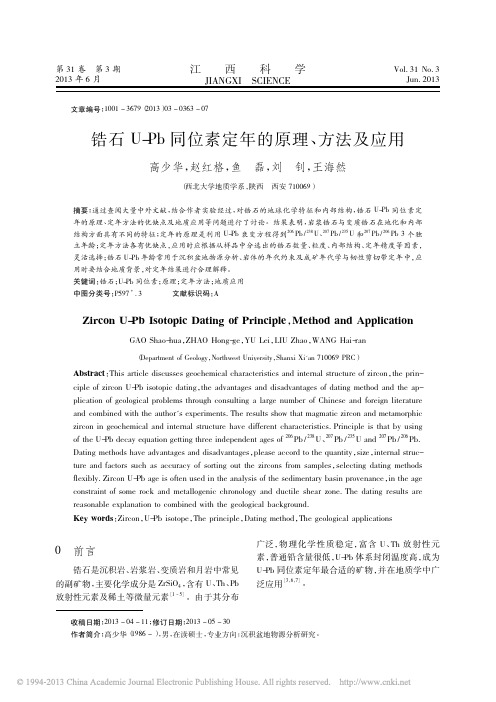
立年龄; 定年方法各有优缺点,应用时应根据从样品中分选出的锆石数量、粒度、内部结构、定年精度等因素,
灵活选择; 锆石 U-Pb 年龄常用于沉积盆地物源分析、岩体的年代约束及成矿年代学与韧性剪切带定年中,应
用时要结合地质背景,对定年结果进行合理解释。
关键词: 锆石; U-Pb 同位素; 原理; 定年方法; 地质应用
收稿日期: 2013 - 04 - 11; 修订日期: 2013 - 05 - 30 作者简介: 高少华( 1986 - ) ,男,在读硕士,专业方向: 沉积盆地物源分析研究。
·364·
江西科学
2013 年第 31 卷
1 锆石的地球化学特征和内部结构
1. 1 锆石的地球化学特征 锆石 的 氧 化 物 中 ω ( ZrO2 ) 占 67. 2% 、ω
Abstract: This article discusses geochemical characteristics and internal structure of zircon,the principle of zircon U-Pb isotopic dating,the advantages and disadvantages of dating method and the application of geological problems through consulting a large number of Chinese and foreign literature and combined with the author's experiments. The results show that magmatic zircon and metamorphic zircon in geochemical and internal structure have different characteristics. Principle is that by using of the U-Pb decay equation getting three independent ages of 206 Pb / 238 U、207 Pb / 235 U and 207 Pb / 206 Pb. Dating methods have advantages and disadvantages,please accord to the quantity,size,internal structure and factors such as accuracy of sorting out the zircons from samples,selecting dating methods flexibly. Zircon U-Pb age is often used in the analysis of the sedimentary basin provenance,in the age constraint of some rock and metallogenic chronology and ductile shear zone. The dating results are reasonable explanation to combined with the geological background. Key words: Zircon,U-Pb isotope,The principle,Dating method,The geological applications
秦皇岛柳江地区长龙山组石英砂岩物_省略_屑锆石U_Pb_Hf同位素的证据_第五春
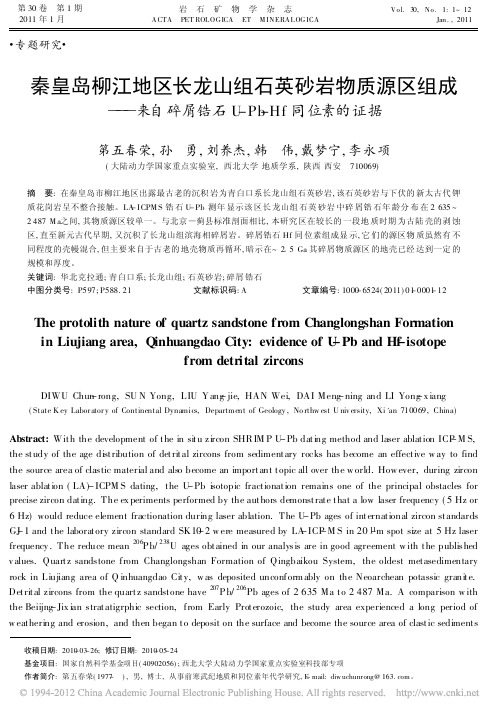
第30卷第1期2011年1月岩石矿物学杂志ACTA PET ROLOGICA ET M INERALOGICAVol.30,No.1:1~12Jan.,2011#专题研究#秦皇岛柳江地区长龙山组石英砂岩物质源区组成)))来自碎屑锆石U-Pb-Hf同位素的证据第五春荣,孙勇,刘养杰,韩伟,戴梦宁,李永项(大陆动力学国家重点实验室,西北大学地质学系,陕西西安710069)摘要:在秦皇岛市柳江地区出露最古老的沉积岩为青白口系长龙山组石英砂岩,该石英砂岩与下伏的新太古代钾质花岗岩呈不整合接触。
LA-I CPM S锆石U-Pb测年显示该区长龙山组石英砂岩中碎屑锆石年龄分布在2635~ 2487M a之间,其物质源区较单一。
与北京)蓟县标准剖面相比,本研究区在较长的一段地质时期为古陆壳的剥蚀区,直至新元古代早期,又沉积了长龙山组滨海相碎屑岩。
碎屑锆石Hf同位素组成显示,它们的源区物质虽然有不同程度的壳幔混合,但主要来自于古老的地壳物质再循环,暗示在~215Ga其碎屑物质源区的地壳已经达到一定的规模和厚度。
关键词:华北克拉通;青白口系;长龙山组;石英砂岩;碎屑锆石中图分类号:P597;P588.21文献标识码:A文章编号:1000-6524(2011)01-0001-12The protolith nature of quartz sandstone from Changlongshan Formationin Liujiang area,Q inhuangdao City:evidence of U-Pb and Hf-isotopefrom detrital zirconsDIWU Chun-rong,SU N Yong,LIU Yang-jie,HAN Wei,DAI Meng-ning and LI Yong-x iang (State K ey Labor ator y of Continental Dynamics,Department of Geology,No rthw est U niv ersity,Xi'an710069,China)Abstract:With the development of the in situ zircon SHRIM P U-Pb dating method and laser ablation ICP-M S, the study of the age distribution of detrital zircons from sedimentary rocks has become an effective w ay to find the source area of clastic material and also become an important topic all over the w orld.How ever,during zircon laser ablation(LA)-ICPM S dating,the U-Pb isotopic fractionation remains one of the principal obstacles for precise zircon dating.The ex periments performed by the authors demonstrate that a low laser frequency(5Hz or 6Hz)would reduce element fractionation during laser ablation.The U-Pb ages of international zircon standards GJ-1and the laboratory zircon standard SK10-2w ere measured by LA-ICP-M S in20L m spot size at5Hz laser frequency.The reduce mean206Pb/238U ages obtained in our analysis are in good agreement w ith the published v alues.Quartz sandstone from Changlongshan Formation of Qingbaikou System,the oldest metasedimentary rock in Liujiang area of Q inhuangdao City,w as deposited unconform ably on the Neoarchean potassic granite. Detrital zircons from the quartz sandstone have207Pb/206Pb ages of2635Ma to2487Ma.A comparison w ith the Beiijng-Jix ian stratatigrphic section,from Early Proterozoic,the study area experienced a long period of w eathering and erosion,and then began to deposit on the surface and become the source area of clastic sediments收稿日期:2010-03-26;修订日期:2010-05-24基金项目:国家自然科学基金项目(40902056);西北大学大陆动力学国家重点实验室科技部专项作者简介:第五春荣(1977-),男,博士,从事前寒武纪地质和同位素年代学研究,E-mail:diw uchunrong@。
东昆仑造山带北缘早志留世黑云母花岗岩成因

BAIFenfei1,ZHANGHongbing2,3,JUYinjuan2,3,ZHAOJunying4,ZHOUKang1
(1.ReseachInstituteofYanchangPetroleum(Group)Co.,Ltd.,Xi’an710065,China; 2.DepartmentofGeology,NorthwestUniversity,Xi’an710069,China; 3.StateKeyLaboratoryofContinentalDynamic,Xi’an710069,China;
4.InstituteofExplorationandDevelopment,SINOPECShanghaiOffshoreOil& GasCompany,Shanghai200120,China)
Abstract:EarlyPaleozoicgranitesintheEastKunlunorogenicbeltpreserveimportantinformationa boutthetectonicandcrustalevolution,whichalsoretainedthecrustalmagmaresponseduringtheCale donianorogenyinthisarea.AdetailedexaminationhasbeenmadeofzirconUPbage,LuHfisotope
墙[12-13]。该地区岩浆岩出露面积约为 2400km2, 呈现多期 次 不 同 类 型 的 特 点,侵 入 岩 呈 东 西 向 展 布,岩石种 类 包 括 花 岗 闪 长 岩 类、二 长 花 岗 岩 类、 钾长花岗 岩 等;早 古 生 代 花 岗 岩 主 要 位 于 昆 北 断 裂以北地 区 内,分 布 较 为 集 中。 而 中 生 代 花 岗 岩 主要分布于昆北断裂的北西方向和昆南断裂的东 南方向,部 分 地 区 与 早 古 生 代 花 岗 岩 相 互 侵 入 穿 插,其余地区呈零星分布,形成时代为前寒武纪至 中生代(图 1)。
锆石U-Pb定年new
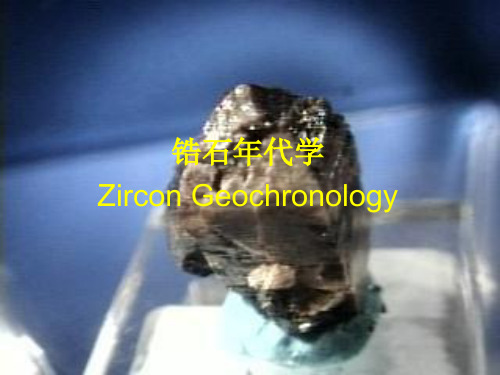
一致曲线ConcordiaFra bibliotek谐和图
锆石的优势
而且具有非常强的抗侵蚀能力,锆石中的U-Pb体系 封闭温度>750 oC, 形成后Pb的扩散封闭温度可以高 达900 oC,锆石形成广,所以锆石是目前测定岩浆结 晶和峰期变质作用年龄最理想的矿物。
锆石形成时有少量初始(普通)Pb的存在,在年龄计算 中需要扣除。但由于锆石中普通Pb很低,则只需测定 204Pb的含量,再根据地球Pb演化模式获得206Pb/204Pb、 207Pb/204Pb比值,估算出普通Pb并进行扣除即可获得放 射成因铅。
TIMS and SHRIMP
Glastonbury Complex, USA (Aleinikoff et al, 2002) 450.5 ± 1.6 Ma (MSWD = 1.11) TIMS 448.2 ± 2.7 Ma (MSWD = 1.3) SHRIMP 单个的分析点精度更高(Pidgeon et al, 1996),如 207/206年龄是2635~2691 ± 1~4 Ma; 平均值 2655 ± 3 Ma (6.8). SHRIMP 2644~2681 ± 4~16 Ma, 2654 ± 5 Ma 又如国内的数据:北秦岭德河黑云二长花岗片麻岩: 924.3~1030.6 ± 1.8~8.0 Ma, 平均值964.4 ± 5.2 Ma (TIMS); 207/206 905~999 ± 19~47 Ma,平均值943 ± 18 Ma (SHRIMP)
208
Pb 204 Pb i
208
等时线方程
理论上, 上述等时线也能象Rb-Sr和Sm-Nd体系一样 进行岩石定年。但是,U、Th、Pb的活动性相当大, 使得U-Pb等时线定年受到很大的限制。
福建平潭岛花岗质岩石成因:来自锆石U-Pb定年、O-Hf同位素及黑云母矿物化学的约束
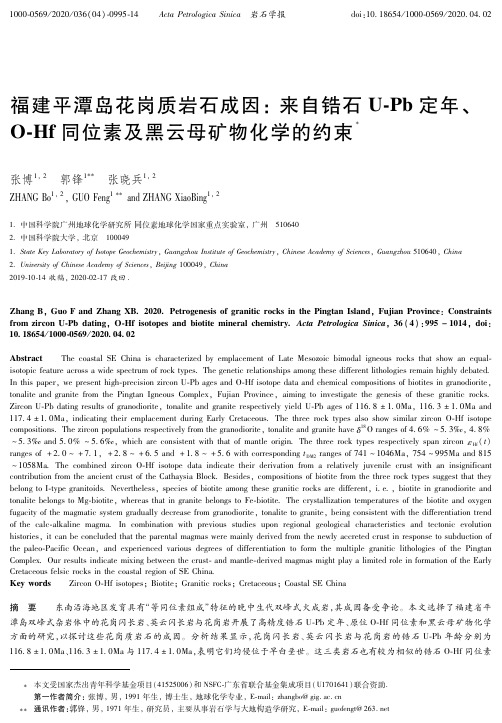
10000569/2020/036(04)099514 ActaPetrologicaSinica 岩石学报
doi:1018654/10000569/20200402
福建平潭岛花岗质岩石成因:来自锆石 UPb定年、 OHf同位素及黑云母矿物化学的约束
张博1,2 郭锋1 张晓兵1,2 ZHANGBo1,2,GUOFeng1 andZHANGXiaoBing1,2
1中国科学院广州地球化学研究所 同位素地球化学国家重点实验室,广州 510640 2中国科学院大学,北京 100049 1StateKeyLaboratoryofIsotopeGeochemistry,GuangzhouInstituteofGeochemistry,ChineseAcademyofSciences,Guangzhou510640,China 2UniversityofChineseAcademyofSciences,Beijing100049,China 20191014收稿,20200217改回
本文受国家杰出青年科学基金项目(41525006)和 NSFC广东省联合基金集成项目(U1701641)联合资助. 第一作者简介:张博,男,1991年生,博士生,地球化学专业,Email:zhangbo@gig.ac.cn
中国矿物岩石地球化学学会第16 届侯德封矿物岩石地球化学青年科学家奖评选公告
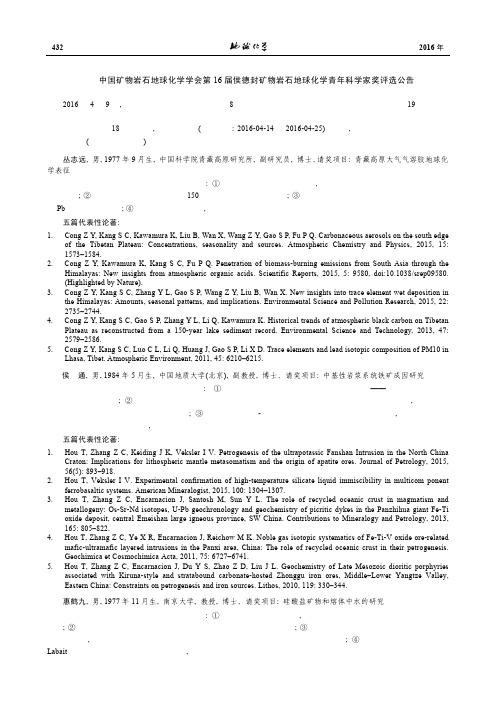
4322016年中国矿物岩石地球化学学会第16届侯德封矿物岩石地球化学青年科学家奖评选公告2016年4月9日, 中国矿物岩石地球化学学会第8届侯德封奖评选工作委员会在杭州召开了评审会。
19名评选委员会委员到会。
会议由评选委员会主任刘丛强院士主持。
本次共评选出18名获奖人, 经网上公示(公示时间: 2016-04-14至2016-04-25)无异议, 评选结果生效。
现将评选结果公告如下(以姓氏拼音为序)。
丛志远, 男, 1977年9月生, 中国科学院青藏高原研究所, 副研究员, 博士。
请奖项目: 青藏高原大气气溶胶地球化学表征与请奖项目有关的主要学术成就及创新点: ①利用分子标志物的指示作用, 揭示了南亚大气污染物向青藏高原的传输过程; ②通过湖泊沉积物重建了过去150年间大气黑碳的历史变化; ③明确了青藏高原气溶胶中痕量元素浓度水平及Pb同位素组成特征; ④针对雪冰介质的特点, 开发了有机酸标志物的前处理方法。
五篇代表性论著:1. Cong Z Y, Kang S C, Kawamura K, Liu B, Wan X, Wang Z Y, Gao S P, Fu P Q. Carbonaceous aerosols on the south edgeof the Tibetan Plateau: Concentrations, seasonality and sources. Atmospheric Chemistry and Physics, 2015, 15: 1573–1584.2. Cong Z Y, Kawamura K, Kang S C, Fu P Q. Penetration of biomass-burning emissions from South Asia through theHimalayas: New insights from atmospheric organic acids. Scientific Reports, 2015, 5: 9580, doi:10.1038/srep09580.(Highlighted by Nature).3. Cong Z Y, Kang S C, Zhang Y L, Gao S P, Wang Z Y, Liu B, Wan X. New insights into trace element wet deposition inthe Himalayas: Amounts, seasonal patterns, and implications. Environmental Science and Pollution Research, 2015, 22: 2735–2744.4. Cong Z Y, Kang S C, Gao S P, Zhang Y L, Li Q, Kawamura K. Historical trends of atmospheric black carbon on TibetanPlateau as reconstructed from a 150-year lake sediment record. Environmental Science and Technology, 2013, 47: 2579–2586.5. Cong Z Y, Kang S C, Luo C L, Li Q, Huang J, Gao S P, Li X D. Trace elements and lead isotopic composition of PM10 inLhasa, Tibet. Atmospheric Environment, 2011, 45: 6210–6215.侯通, 男, 1984年5月生, 中国地质大学(北京), 副教授, 博士。
Zircon U-Pb ages and Hf isotopic characteristics of the Dehe biotite monzonitic gneiss pluton in
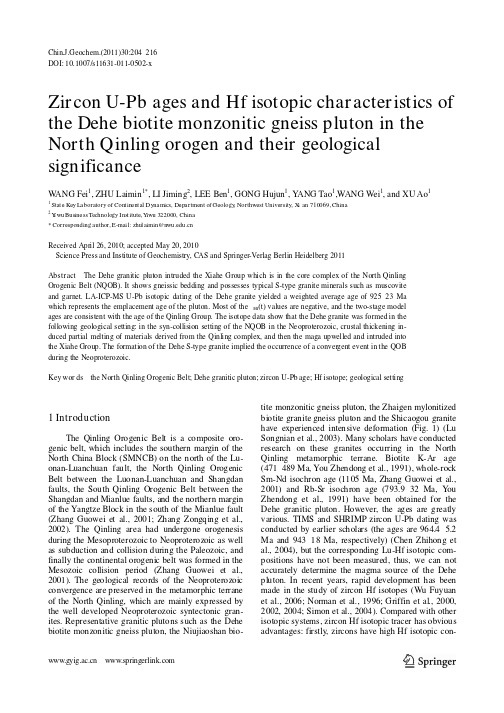
Chin.J.Geochem.(2011)30:204–216DOI:10.1007/s11631-011-0502-xy Zir con U-Pb ages and Hf isotopic char acter istics of the Dehe biotite monzonitic gneiss pluton in the North Qinling orogen and their geological significanceW ANG Fei 1,ZHU Laimin 1*,LI Jiming 2,LEE Ben 1,GONG Hujun 1,Y ANG Tao 1,WANG Wei 1,and XU Ao 11Stat e Key Laboratory of Cont inent al Dynamics,Department of Geology ,Northwest University,X i ’an 710069,China 2Y i wu Bus iness Technology Ins t itute,Y iwu 322000,China*Corresponding author,E-mai l:zhul aimin@Received April 26,2010;accepted May 20,2010Science Press and Institute of Geochemistry,CAS and Springer-Verlag Berlin Heidelberg 2011Abstract The Dehe granitic pluton intruded the Xiahe Group which is in the core complex of the North Qinling Orogenic Belt (NQOB).It shows gneissic bedding and possesses typical S-type granite minerals such as muscovite and -ICP-MS U-Pb isotopic dating of the Dehe granite yielded a weighted average age of 925±23Ma which represents the emplacement age of the pluton.Most of the εHf (t)values are negative,and the two-stage model ages are consistent with the age of the Qinling Group.The isotope data show that the Dehe granite was formed in the following geological setting:in the syn-collision setting of the NQOB in the Neoproterozoic,crustal thickening in-duced partial melting of materials derived from the Qinling complex,and then the maga upwelled and intruded into the Xiahe Group.The formation of the Dehe S-type granite implied the occurrence of a convergent event in the QOB during the Neoproterozoic.Key wor ds the North Qinling Orogenic Belt;Dehe granitic pluton;zircon U-Pb age;Hf isotope;geological setting1IntroductionThe Qinling Orogenic Belt is a composite oro-genic belt,which includes the southern margin of the North China Block (SMNCB)on the north of the Lu-onan-Luanchuan fault,the North Qinling Orogenic Belt between the Luonan-Luanchuan and Shangdan faults,the South Qinling Orogenic Belt between the Shangdan and Mianlue faults,and the northern margin of the Yangtze Block in the south of the Mianlue fault (Zhang Guowei et al.,2001;Zhang Zongqing et al.,2002).The Qinling area had undergone orogenesis during the Mesoproterozoic to Neoproterozoic as well as subduction and collision during the Paleozoic,and finally the continental orogenic belt was formed in the Mesozoic collision period (Zhang Guowei et al.,2001).The geological records of the Neoproterozoic convergence are preserved in the metamorphic terrane of the North Qinling,which are mainly expressed by the well developed Neoproterozoic syntectonic gran-ites.Representative granitic plutons such as the Dehe biotite monzonitic gneiss pluton,the Niujiaoshan bio-tite monzonitic gneiss pluton,the Zhaigen mylonitized biotite granite gneiss pluton and the Shicaogou granite have experienced intensive deformation (Fig.1)(Lu Songnian et al.,2003).Many scholars have conducted research on these granites occurring in the North Qinling metamorphic terrane.Biotite K-Ar age (471–489Ma,You Zhendong et al.,1991),whole-rock Sm-Nd isochron age (1105Ma,Zhang Guowei et al.,2001)and Rb-Sr isochron age (793.9±32Ma,Y ou Zhendong et al.,1991)have been obtained for the Dehe granitic pluton.However,the ages are greatly various.TIMS and SHRIMP zircon U-Pb dating was conducted by earlier scholars (the ages are 964.4±5.2Ma and 943±18Ma,respectively)(Chen Zhihong et al.,2004),but the corresponding Lu-Hf isotopic com-positions have not been measured,thus,we can not accurately determine the magma source of the Dehe pluton.In recent years,rapid development has been made in the study of zircon Hf isotopes (Wu Fuyuan et al.,2006;Norman et al.,1996;Griffin et al.,2000,2002,2004;Simon et al.,2004).Compared with other isotopic systems,zircon Hf isotopic tracer has obvious advantages:firstly ,zircons have high Hf isotopic con-www.g tents,low176Lu/177Hf ratios,and no obvious accumu-lation of radiogenic Hf,so that the error of76Lu/177Hf ratios caused by uncertainty in age is limited(Knud-sen et al.,2001;Kinny and Mass,2003);secondly,the zircon Hf isotopic system possesses a high closure temperature,and zircons can maintain the original Hf isotopic composition,even in the high-grade meta-morphic condition of granulite facies,therefore,the measured Hf isotopic composition can approximately represent that of the parental system;thirdly,zircons are highly resistent against weathering.Even residual zircons from the ancient crust which have experienced many geological events can still maintain the nature of the early crust.So,they can preserve the protolithic characteristics of different rocks(Wu Fuyuan et al., 2007).Therefore,zircons can be used as a powerful tool in constraining the origin and evolution of mag-mas and the interaction between the crust and the mantle(Griffin et al.,2004;Y ang Jinhui et al.,2006, 2007;Zhu Laimin et al.,2010a,b).In this paper we used LA-ICP-MS zircon U-Pb ages,REE,Hf isotopic composition and petrography data in combination with the geochemistry and Sr-Nd isotope data ob-tained by previous scientific workers to discuss the source and lithogenic background of the Dehe pluton. 2Regional geology and sample petrography As shown in Fig.1,the Dehe biotite monzonitic gneiss pluton is situated in the core complex of the NQOB.A large number of Early Neoproterozoic gran-ite plutons are well developed in the Qinling complex and these plutons extend as long as about400km from the eastern part of Xixia County,Henan Province to the western part of the T aibai area,Shannxi Prov-ince.Some of these plutons such as the Dehe biotite monzonitic gneiss pluton(You Zhengdong et al., 1991),the Niujiaoshan biotite monzonitic gneiss plu-ton(Wang Tao et al.,1994,1998),the Zhaigen my-lonitized biotite granite gneiss pluton(Wang Shiyan, 1994),the Shicaogou granite pluton(Chen Danlin et al.,2004)and the Caiao granite pluton(Zhang Hong-fei et al.,1993)have been identified.The Qinling complex consists mainly of the Qinling Group and the newly established Xiahe Group(Wang Tao et al., 1997).The former includes a set of amphibolitic facies biotite(two-mica)plagioclase(monzonitic) gneiss,marble,calcium silicate rocks with amphibo-lite.And the latter consists mainly of low meta-am-phibolitic facies-greenschist facies two-mica quartz schist,quartzite,calcareous schist,greenschist,and with a small amount of plagioclase amphibolite,cal-cium silicate-marble,and lies in the core of the Qinling complex and separates the Qinling Group into two parts.As shown in Fig.1,the Kuanping and Er-langping groups are both located in the northern part of the Qinling Group.The Kuanping Group consists of middle or low meta-greenschist and amphibolite, and the original rock of the Kuanping Group is vol-canic rock which was formed in the Mesoproterozoic rift valley small ocean basin environment(from1600 to1000Ma?)and experienced the Jinningian intensive deformational transformation(Zhang Guowei et al., 2001).The Erlangping Group is composed of ophio-lite and volcanic-sedimentary rock.The main body of the Erlangping Group was formed in the Paleozoic, and the age of the the basic volcanic rock from the Erlangping Group ranges from900to600Ma(Zhang Zongqing et al.,1994),all suggesting that it may have mixed with some Neoproterozoic igneous rock.The Danfeng,Wuguan and Liuling groups are all situated on the south of the Qinling complex.The Wuguan Group is composed mainly of low meta-amphibolitic facies marble-calcareous schist,mica schist,felsic schist and amphibolite,located between the Danfeng Group and the Liuling Group.The original rock of the Wuguan Group is volcanic-sedimentary rock.The whole-rock Sm-Nd isochron age of the meta-basic volcanic rock is1382±30Ma,which indicates that the Wuguan Group may be formed in the Mesoprotero-zoic rift valley environment(Pei Xianzhi et al.,1997). The Danfeng Group was formed in the Paleozoic and consists of ophiolite and volcanic-sedimentary rock (Zhang Guowei et al.,2001),but the whole-rock Sm-Nd isochron age of the basic rock ranges from 1000to700Ma(Zhang Zongqing et al.,1994).The main body of the Liuling Group is greenschist facies sedimentary rock formed in the Paleozoic.The Dehe biotite monzonitic gneiss pluton lo-cated at Dehe Village,Xixia County,and the sur-rounding rocks are calc-silicate fels and leptynites, which belong to the Xiahe Group(Lu Songnian et al., 2003).The residual intrusive contact relationship be-tween the surrounding rocks and the Dehe pluton,en-claves from the surrounding rocks and local chilled margins occurring at the edge of the pluton can be seen there.The Dehe pluton is about6km2and dis-plays an NNW-directed oval shape on the surface.The samples(3332'16.4"N;3332'16.4"E)used for zir-con U-Pb isotopic dating and Lu-Hf isotopic analysis are(light)gray biotite monzonitic gneiss pluton which has penetrative gneissic layerings and also shows bends and deformation of the gneissic structures.As the K-feldspar phenocrysts are well developed,the rock generally exhibits porphyry-like(blastoporphy-ritic)textures(Fig.2a).The contents of the perthite feldspar in K-feldspar are high,equivalent to those of plagioclase.Under the microscope,the matrix of the pluton is medium hypidiomorphic or idiomorphic granular,and also exhibits mylonitic texture,with quartz directional distribution into wire-drawing.The dark-colored minerals are mainly micas with obviousdirectional distribution together with light-coloredminerals arrange for banding-textures.Besides the K-feldspar phenocrysts,the major minerals also in-clude K-feldspar,plagioclase,quartz,biotite,andsmall amounts of muscovite and garnet (Fig.2b,c,and d).The accessory minerals are mainly zircon,sphene andgarnet.Fig.1.Geological sketch map of the tectonics in the core of the Qinling orogeni c belt and the Neoproterozoic grani toids (after W ang Tao et al.,2005).Fig.2.The characteri stics of petrofabri c and mineral compos i tion of the Dehe pluton.a.K-feldspar phenocryst,gneissic layerings,porphyry-like (blastoporphyritic)texture,and the K-feldspar phenocryst with texture of rotation and eye-s hape in a handy specimen;b.tartan twinni ngs of the microcline and polysynthetic t winnings of the plagiocl ase (under perpendicular polar-ized light micros cope);c.t he coexistence of garnet,bi oti te and muscovite with irregular flakes and leaf-s hape under perpendicular pol arized light mi croscope (under perpendicular polarized li ght microscope);d.biotite with leaf-shape and directional distribution into thin stripe texture;quartz with the characteristics of irregular granular,wavy exti nction and dentate cont act (under perpendicular pol arized l ight mi croscope).Pl.Plagio-clase;Qz.quart z;Mi.microcline;Bi.biotite;Kf.K-feldspar;Mus.mus covit e;Grt.garnet.The K-feldspar is composed of two parts: K-feldspar phenocrysts and K-feldspar in the matrix. The K-feldspar phenocrysts are light-red in color with non-uniform size.The large K-feldspar phenocrysts, with particle sizes approximating to3cm×4cm,ex-hibit Carlsbad twinnings in hand samples(under the microscope we found that they are mainly perthites). As it experienced intensive metamorphic deforma-tional transformation,the Dehe granite exhibits well developed gneissic structures,and the K-feldspar phenocrysts have characteristics of rotation,eye-shaped or diagonal distribution,and account for5%. K-feldspars in the matrix are hypidiomorphic to an-hedral granular and account for30%–35%,the major-ity of them are microcline-perthites,followed by microclines with tartan twinnings(Fig.2b).Plagio-clases are hypidiomorphic or anhedral granular,with polysynthetic twinnings,which mainly are oligo-clase-andesines and account for25%–30%of the minerals.Under the microscope,irregular feldspars, qutarz,etc.gather into augen or banded texture,which exhibits the characterics of recrystallization.Quartz is irregular granular and accounts for30%–35%,and the large granular quartz shows wavy extinction and ex-hibits dentate contact.In contrast,the small granular ones with obvious tendency of directional distribution have the characteristics of being elongated.And dark-colored minerals as such as biotite,etc.,together with the small granular quartz gather into light and dark-colored banded texture(Fig.2d).Biotites are the dominating dark-colored minerals and account for more than95%of the dark-colored minerals,5%–8% of all the minerals.And as shown in Fig.2c,the bio-tite with the characteristics of leaf-shaped or twisted strips show an obvious tendency of directional distri-bution and gather into thin stripe or streak texture. Muscovites with irregular flakes,leafs or twisted strips,together with the biotites,gather into direc-tional strip-texture(Fig.2c).And the muscovites are equivalent to the biotites in diameter.The garnets are idiomorphic to hypidiomorphic granular and often coexist with other minerals.The contents of the gar-nets are equivalent to those of the muscovites.3Sampling and analytical methodsThe U-Pb ages,REE,and Hf isotopes of zircons were analyzed at the State Key Laboratory of Conti-nental Dynamics of the Northwest University,Xi’an, China.Zircons were separated by using heavy liquid and magnetic separation methods at the Institute of Geophysics and Geochemistry,Ministry of Land and Resources.Pure zircons were finally handpicked un-der a binocular microscope,then mounted in epoxy resin and polished until the grain interiors were ex-posed.Before analysis,the surface was cleaned using 3%HNO3to remove any lead contamination.The CL images were obtained using an electron microscope from FEI(USA)equipped with a Mono CL3+cathode luminescence spectroscope produced by Gatan Cor-poration(USA).The Agilient7500a with Shiele Touch(Agilient)coupled with MC-ICP-MS were em-ployed for in-situ zircon U-Pb dating and Lu-Hf iso-topic analysis.The GeoLas200M laser-ablation sys-tem(MiroLas,Germany)consists of a COMPexpro 102ArF excimer laser(Lambad Physik,Germany, wavelength of193nm)and MicroLas the optical sys-tem.The ICP-MS for zircon U-Pb dating,Lu-Hf iso-topic analysis and trace element analysis was em-ployed as the same Laser-Ablation,and the signals were received by ICP-MS and MC-ICP-MS inde-pendently(Y uan Honglin et al.,2008).Helium was used as a carrier gas to transport the ablated aerosal from the laser-ablation cell to the ICP-MS torch and the Excimer laser energy of90mJ/pulse was used for ablation at a repetition rate of10Hz.The laser spot was44m in diameter.The gas background acquisi-tion time and signal acquisition time were30and40 seconds.The sampling method of laser ablation is single point of ablation in the LA-ICP-MS experiment.Be-fore analysis the synthetic silicate glass standard ref-erence material NIST610developed by the National Institute of Standards and Technology(NIST)was employed to keep the instrument in good condition of optimization,the maximum sensitivity,the smallest ThO+/Th+(<2%)and the lowest background values, and the data were acquired in peak-jumping-pulse-counting mode with one-point measured per peak.After finishing every5spots we measured one zircon91500.Raw data were processed using the GLITTER program(V ersion 4.4)(Jackson et al., 2004).The standard zircon91500was used as the ex-ternal standard for isotope ratio fractionation correc-tion in the calculation of the zircon,NIST6100was used as the external standard,and Si was used as the internal standard in the element concentrations.The concordia ages and diagrams were obtained using the ISOPLOT(Ver3.0)(Ludwig,2003).The detailed ex-perimental testing procedure and parameters are shown in the reference of Yuan Honglin et al.(2004).In-situ zircon Lu-Hf isotopic measurements,in-terference of176Lu on176Hf was corrected by measur-ing the intensity of the interference-free175Lu,using the recommended176Lu/175Lu ratio of0.02669to cal-culate176Lu/177Hf.Similarly,the isobaric interference of176Yb on176Hf was corrected by using the com-mended176Yb/172Yb ratio of0.5886(Chu et al.,2002) to calculate176Hf/177Hf ratios.Zircons of91500andGJ-1were used as the reference standards for cali-brating and controlling the condition of analytical in-strumentation.The measured176Hf/177Hf ratios are0.282134±0.000032(n=6,2σ)for91500and0.282032±0.000024(n=6,2σ)for GJ-1,which are ingood agreement with the recommended176Hf/177Hfratios of0.2823075±0.0000058(2σ)for91500and0.282015±0.0000019(2σ)for GJ-1(Wu Fuyuan et al.,2006;Elhlou et al.,2006).We have adopted a decayconstant for176Lu of1.867×10-11a-1(Soderlund et al.,2004).Initial176Hf/177Hf ratio,denoted asεHf(t),iscalculated relative to the chondritic reservoir with a 176Hf/177Hf ratio of0.282772and176Lu/177Hf of 0.0332(Blichert-T oft and Albarede,1997).Two-stageHf model ages(T DM2)are calculated by assuming amean176Lu/177Hf value of0.0093for the average up-per continental crust(V ervoort et al.,1996,1999).4Analytical results4.1LA-ICP-MS zir con U-Pb datingZircons are abundant in the Dehe biotite monzo-nitic gneiss pluton,most of which are idiomorphic,biconical short or long columnar crystals(Fig.3).Most of them are light red and some are colorless.TheTh/U ratios of the zircons are high,mostly are greaterthan0.4,and only individuals are greater than0.1butstill less than0.4,which are between magmatic zirconand metamorphic zircon(Rubatto and Gebauer,2000;Moller et al.,2003).The Th/U ratios can not be used to effectively identify the causes of zircons(Wu Yuanbao and Zheng Y ongfei,2004),because many of magmatic zircon Th/U ratios can be low,even lower than0.1(Wu Y uanbao et al.,2002;Gebauer,1996; Hidaka et al.,2002).Chondrite-normalized REE dis-tribution patterns show that the zircons have positive Ce anomalies,obviously negative Eu anomalies(Fig.4),a large∑REE(ranging from4644.18×10-6–17043.69×10-6,averaging8639.33×10-6)and a gradual enrichment in HREE,and exhibit the typical charac-teristics of the magmatic zircons.As shown in Fig.3, the CL images are relatively simple and also have clear intensively oscillatory zonings,which also sug-gest that the zircons are typical magmatic zircons.The U-Pb isotopic composition and ages are listed in Table1.With a common lead correction,the 206Pb/238U ages are more suitable to be adopted forthe young zircons which are younger than1Ga (Griffin et al.,2004).The ages and diagrams were obtained using the ISOPLOT(Ver 3.0)(Ludwig, 2003).In the206Pb/238U-207Pb/235U concordia dia-gram,3points(the206Pb/238U weighted average ages of DH-1-15,DH-1-03,DH-1-17are1271±14, 1003±13,952±11Ma,respectively)deviate from the concordia line indicating lead loss,21analytical spots are concentrated near the concordia line(Fig.5),and yield a weighted average206Pb/238U age of 925±23Ma which represents the emplacement age of the pluton(Table2).Table1LA-ICP-MS zircon U-Pb data for the Dehe plutonContent(10-6)Isotopic ratio Age(Ma)Sample No.Pb232Th238U Th/U 207Pb/206Pb1σ207Pb/235U1σ206Pb/238U1σ207Pb/206Pb1σ207Pb/235U1σ206Pb/238U1σDH-1-01367.4317.5524.40.610.072160.0010 1.423390.02000.143020.00169901389988629 DH-1-02640.7210.8873.60.240.069830.0009 1.448870.01970.150430.00179231390989039 DH-1-03428.9215.0530.00.410.079030.0011 1.835230.02670.168370.0019117313105810100310 DH-1-04323.880.0369.20.220.074580.0016 1.682700.03010.163650.001910574410021197710 DH-1-05389.4400.8464.60.860.067810.0010 1.535890.02280.164250.001986314945998010 DH-1-06471.5150.1641.00.230.073390.0011 1.548200.02380.152970.0017102515950991810 DH-1-07313.262.4441.00.140.067780.0011 1.433960.02310.153420.0018862169031092010 DH-1-08328.3128.3477.90.270.068700.0011 1.377880.02270.145450.001789017880108759 DH-1-09337.6104.8427.80.240.072010.0012 1.672600.02720.168430.00199861699810100311 DH-1-10191.7166.7242.70.690.072310.0012 1.566200.02660.157070.0018995179571194010 DH-1-11407.1230.8563.00.410.072740.0012 1.539950.02570.153520.00181007179461092110 DH-1-12134.899.1179.40.550.071980.0014 1.523570.03050.153490.0019985229401292010 DH-1-1391.373.9127.50.580.070400.0013 1.406640.02650.144900.0017940208921187210 DH-1-14355.4252.2442.60.570.071480.0012 1.585990.02710.160900.0019971179651196210 DH-1-15387.6112.7365.70.310.088130.0021 2.648230.05570.217950.0026138547131415127114 DH-1-16209.866.9280.20.240.069320.0013 1.526290.02850.159680.0019908209411195511 DH-1-17286.0323.2354.30.910.080390.0015 1.765170.03360.159220.001912072010331295211 DH-1-18354.483.9492.50.170.071480.0013 1.515470.02860.153760.0018971209371292210 DH-1-19625.5168.4951.90.180.069160.0013 1.337530.02470.140240.001790420862118469 DH-1-20294.6178.0375.70.470.073130.0022 1.605530.04500.159230.00201018639721895211 DH-1-21245.181.8299.50.270.073590.0015 1.750780.03530.172530.0021103022102713102611 DH-1-22284.0104.9408.10.260.075150.0016 1.521320.03180.146800.00181073239391388310 DH-1-23209.097.0295.50.330.072210.0022 1.461020.04010.146750.0018992639141788310 DH-1-24351.2115.8435.30.270.071930.0015 1.690740.03600.170440.002198424100514101512Fig.3.CL i mages of zircons from the Dehe pluton.4.2Zir con Hf isotopic compositionExcept for DH-1-05(0.002876),176Lu/177Hf ratiosare all less than0.002,which exhibit a lower radiogenicHf isotopic accumulation after the formation of the zir-cons.The zircon average f Lu/Hf of the Dehe pluton is-0.96,which is obviously less than that of the maficcrust(-0.34,Amelin et al.,2000)and sialic crust(-0.72,V ervoort et al.,1996),thus the two-stage model agescan better reflect the average time that the magmasource kept in the crust.According to the related Hfisotopic formula(Wu Fuyuan et al.,2007),the sialiccrustal f Lu/Hf ratios were adopted to calculate theεHf(0),εHf(t),t DM1and t DM2,as listed in T able3.The Hf iso-topic composition is more homogeneous,and the 176Hf/177Hf ratios of all the zircons range from0.281862 to0.282296with a weighted average value of0.282166±0.000041(Fig.6);theεHf(t)values rangefrom-12.3to3.3with an average of-2.2,and most ofthe values are negative;the two-stage model ages rangefrom1484to2238Ma with an average of1740Ma.5Discussion5.1Magma sour ces and petr ogenesisThe ouput and formation of granitoids took place in the form of lithospheric tectonic movements;the formation and emplacement are the important aspects of orogenic tectonic evolution(Lu Songnian et al., 2003).Different types of granites were formed in dif-ferent tectonic geodynamic settings,and the mineral assemblages and geochemical characteristics also show some differences.Therefore,through the study on the granitic geological environments,rock fabrics, mineral assemblages and geochemical characteristics, we can distinguish the differences and tectonic envi-ronments of granitoids(Barbarin,1999;Maniar and Piccoli,1989).Fig.4.Chondrit e-normal ized REE patterns of zircon grains in the Dehepluton.Table3LA-ICP-MS zircon Hf isotopic compositions of the Dehe plutonSample No.Age(Ma)176Yb/177Hf176Lu/177Hf176Hf/177Hf2σεH f(0)εHf(t)2σt DM1t DM2f Lu/H f DH-1-018620.0517820.0018800.2822820.000024-17.30.6 1.414021539-0.94 DH-1-029030.0344570.0010940.2822110.000019-19.8-0.5 1.314711631-0.97 DH-1-0310030.0217020.0007460.2820810.000025-24.4-2.8 1.416381823-0.98 DH-1-049770.0260760.0009390.2820230.000024-26.5-5.5 1.417261940-0.97 DH-1-059800.0857790.0028760.2822960.000021-16.8 1.0 1.314201516-0.91 DH-1-069180.0429470.0013370.2821870.000030-20.7-1.2 1.515151677-0.96 DH-1-079200.0369850.0013060.2820870.000027-24.2-4.7 1.416531854-0.96 DH-1-088750.0303110.0009420.2822360.000026-18.9-0.2 1.414301589-0.97 DH-1-0910030.0363080.0013650.2821270.000027-22.8-1.5 1.416001762-0.96 DH-1-109400.0383360.0013330.2821400.000019-22.4-2.4 1.315811755-0.96 DH-1-119210.0269760.0009590.2820800.000028-24.5-4.7 1.416481855-0.97 DH-1-129200.0295310.0010280.2821710.000035-21.2-1.5 1.615241695-0.97 DH-1-138720.0210990.0007630.2821460.000018-22.1-3.3 1.215481746-0.98 DH-1-149620.0374550.0013320.2822880.000019-17.1 3.3 1.313721484-0.96 DH-1-1512710.0248590.0008620.2819570.000034-28.8-1.4 1.618141971-0.97 DH-1-169550.0181980.0006410.2822040.000025-20.1-1.6 1.414641614-0.98 DH-1-179520.0365830.0012650.2821010.000031-23.7-3.5 1.516321819-0.96 DH-1-189220.0189360.0007920.2818620.000029-32.2-12.3 1.519412238-0.98 DH-1-198460.0420410.0014810.2822040.000018-20.1-2.2 1.214961670-0.96 DH-1-209520.0419540.0014750.2822530.000016-18.3 1.8 1.214261553-0.96 DH-1-2110260.0115810.0004230.2821790.000024-21.0 1.4 1.414901631-0.99 DH-1-228830.0337890.0012220.2820870.000027-24.2-5.4 1.416491861-0.96 DH-1-238830.0346920.0011960.2821480.000026-22.1-3.3 1.415641753-0.96DH-1-2410150.0264280.0009290.2821010.000025-23.7-1.9 1.416171790-0.97-ICP-MS zircon U-Pb concordia diagram.The study conducted by Maniar(Maniar and Piccoli,1989)indicated that different granitoids have different mineral associations.In continental collision granites(CCG),the contents of perthite are equivalent to those of plagioclase,and the typical minerals are biotite,muscovite or garnet(Table4).In the Dehe pluton the contents of perthite are equivalent to those of plagioclase,and the dark-colored minerals are mainly biotite,muscovite etc.,aslo with a minor amount of garnet.From the table of the mineral as-semblages and geochemical characteristic compari-sons among the orogenic granites(Table4),it can be seen that the Dehe biotite monzonitic gneiss pluton has a typical mineral assemblage of the CCG.Y ou Zhendong et al.(1991)reported that the Dehe biotite monzonitic gneiss pluton has the high contents of SiO2and Al2O3,with SiO2=70.93%, Al2O3=13.28%,Na2O=2.33%,CaO=1.90%,K2O/ Na2O=1.67,Rittmann index(δ)=1.41,ACNK=1.15, and the Dehe pluton belongs to the aluminum satura-tion calc-alkaline series(You Zhendong et al.,1991). The granitic pluton has a high content ofΣREE (ΣREE=174.44×10-6),has undergone obvious frac-tionation between LREE and HREE(LREE/ HREE=6.571,La/Yb=1.25,La/Sm=5.92)and has a remarkable Eu anomaly(δEu=0.43).The chon-drite-normalized REE distribution patterns exhibit a right-inclined“V”-shaped curve which indicates the characteristics of the remelting granite(Fig.7).With the ocean ridge granite normalization,eleven trace elements such as K2O,Rb,Ba,Th,Nb,Ce,Hf,Zr,Sm, Y and Yb show a relative enrichment in incompatible elements of Rb and Th,and a depletion in HFSF of Zr, Hf,Y,and Yb which have the geochemical character-istics of Syn-COLG(Fig.7).Fig.6.Zircon Hf isotopic composition of the Dehe pluton.The Dehe pluton belongs to the calc-alkaline se-ries,and ACNK=1.15,K2O/CaO=1.23,Na2O/K2O =0.6,MgO/∑FeO=0.3,MgO/MnO=21.17,Al2O3/ (Na2O+K2O)=2.12(You Zhendong et al.,1991).Ac-cording to the table of the mineral assemblages and geochemical characteristic comparisons among the orogenic granites,the Dehe pluton possesses the same geochemical characteristics of the CCG(T able4).And the ocean ridge granite-normalized geological patterns show that the Dehe pluton has similar characteristics to the Tibetan Syn-COLG(Pearce et al.,1984)(Fig.7). In the Rb-(Y+Nb)and Rb-(Yb+Ta)discrimination diagrams(Pearce et al.,1984)the samples fall within the region of Syn-COLG(Fig.8).Studies on the zircon Hf isotopes indicated that granites with negativeεHf(t)values were formed from partial melting of the ancient lower crust(Griffin et al., 2004;Vervoort et al.,2000).TheεHf(t)values of zir-con from the Dehe pluton range from-12.3to3.3, with an average value of-2.2,with19values being negative and5values being slightly positive,ranging from0to3.3[theεHf(t)values of DH-1-01,DH-1-05, DH-1-14,DH-1-20and DH-1-21are0.6,1.0,3.3,1.8 and1.4,respectively].As shown in theεHf(t)-t dia-gram,most of the samples fall within an area under the depleted mantle and the chondritic evolution line, distributed between the1.6Ga and 2.0Ga crustal evolution lines,implying that the magma had origi-nated from partial melting of the ancient lower crust. And except for individual ratios,the initial87Sr/86Sr ratios are greater than0.706,which also are character-istic of crust-derived granites(Faure,1986)(Fig.5).Zircon U-Pb and whole-rock Sm-Nd isotopic dating of gneiss pluton and plagioclase amphibolite from the Qinling Group yielded ages ranging from 1987to2267Ma(Zhang Zongqing et al.,1994,1996). The comprehensive geological study also showed that the main body of the Qinling Group should be formed in the Paleoproterozoic(Zhang Guowei et al.,2001). The two-stage model ages,ranging from1.6to2.0Ga (Fig.9)measured in this paper,are consistent with the known age of the Qinling Group.The Nd isotopic study showed that the average value ofεNd(925Ma)is -5.1.As calculated with the“crustal Hf-Nd isotopic correlation”formula(V ervoort et al.,1999),theεHf (925Ma)is about-4,close to the average value of -2.2measured in this paper,implying that the magma may have originated from partial melting of materials derived from the Qinling Group.The Hf isotope data are supported by Sr isotopes,and they are all well in consistency(T able5).The initial87Sr/86Sr ratios,av-eraging0.7220±0.003,exhibit the Sr isotopic compo-sition of the S-type granites(Durrance,1986;Han Yinwen and Ma Zhendong,2003).As given in Table6, theεNd(t)values of the Dehe pluton are close to those of the paragneiss in the Qinling Group[εNd(t)=-5.5]. Therefore,the consistency between the above Sr-Nd and zircon Hf isotopic compositions suggests that the magma of the Dehe pluton may have originated mainly from partial melting of crustal materials de-rived from the Qinlingcomplex.Fig.7.Ocean ri dge granite-normalized geochemical patterns(afterY ou Zhendong et al.,1991).Table4Comparisons of the mineral assemblages and chemical characteristics among the orogenic granites IAG CAG CCG POG Dehe pl utonType Perthite<pl agio-clasePerthite<plagioclasePerthite≈plagio-clasePerthite<plagiocl ase Perthite≈plagioclaseTypical mineralBiotite±hornblende±pyroxeneBiotite±hornblende±epi doteBiotite、muscovite±t ourmaline±cordierite±chlorite±garnetBiotit e±hornbl endeorbiot ite±muscovi teBiotitemuscovi tegarnetSiO2content(%)60–6862–7670–7670–7870.93Alkali-lime indexCalcic tocalc-alkali neCalc-alkalineCalc-alkaline toalkali-calcicAlkali-calci c Calc-alkalineACNK PredominantlymetaluminousMetaluminous per-aluminousPeraluminousPeraluminous metaluminousPeralkaline(minor)1.15Na2O/CaO About0.1About<0.4About2.0–10.0About2.0–18.0 1.23Na2O/K2O About0.4–3.0About0.4–2.0About0.4–1.5About0.6–1.20.6MgO/∑FeO0.3–0.850.10–0.500.05–0.60.02–0.300.3MgO/MnO12.0–28.0 2.0–38.0 2.0–45.0 2.0–18.021.17 ANK>1.5>1.1>1.10.9–1.4 2.12Date source(Maniar and Pi ccoli,1989)(Y ou Zhendong et al.,1991)。
新疆阿克陶县乌孜别里地区流纹岩的形成时代及成因分析

第50卷 第6期Vol.50, No.6, 562–5782021年11月GEOCHIMICANov., 2021收稿日期(Received): 2020-01-03; 改回日期(Revised): 2020-03-21; 接受日期(Accepted): 2020-04-02基金项目: 新疆维吾尔自治区重点研发专项(2019B00011); 新疆维吾尔自治区重大科技专项(2018A03004); 国家自然科学基金(91962215, 41972088); 国家重点研发计划(2019YFC06005201); 第二次青藏科考项目(2019QZKK0802-01); 国家十二五科技支撑项目(2015BAB05B03); 中国科学院广州地球化学研究所135项目(135TP201601)作者简介: 李沛(1986–), 男, 博士研究生, 构造地质学专业。
E-mail:***************** 通讯作者(Corresponding author):WANGHe,E-mail:*************.cn;Tel:+86-20-85290986Geochimica▌ Vol. 50▌ No. 6▌ pp. 562–578▌Nov., 2021新疆阿克陶县乌孜别里地区流纹岩的形成时代及成因分析李 沛1,2, 王 核1*, 普 强3, 丘增旺1,2,闫庆贺1,2, 董 瑞1,2, 张晓宇1,2(1. 中国科学院 广州地球化学研究所 矿物学与成矿学重点实验室, 广东 广州 510640; 2. 中国科学院大学, 北京 100049; 3. 河北省地矿局第五地质大队, 河北 唐山 063000)摘 要: 西昆仑乌孜别里山口南侧一带火山岩地层的时代归属一直存有争议。
该套地层虽普遍发育以流纹岩为主的火山岩系, 但尚未有人对其开展系统的年代学与地球化学研究。
本次研究对该套地层中的流纹岩进行元素地球化学、锆石U-Pb 定年及Hf 同位素的研究。
- 1、下载文档前请自行甄别文档内容的完整性,平台不提供额外的编辑、内容补充、找答案等附加服务。
- 2、"仅部分预览"的文档,不可在线预览部分如存在完整性等问题,可反馈申请退款(可完整预览的文档不适用该条件!)。
- 3、如文档侵犯您的权益,请联系客服反馈,我们会尽快为您处理(人工客服工作时间:9:00-18:30)。
Zircon U –Pb and Hf isotopic constraints from eastern Transhimalayan batholiths on the precollisional magmatic and tectonic evolution in southern TibetHan-Yi Chiu a ,Sun-Lin Chung a ,⁎,Fu-Yuan Wu b ,Dunyi Liu c ,Yu-Hsuan Liang a ,I-Jhen Lin a ,Yoshiyuki Iizuka d ,Lie-Wen Xie b ,Yanbin Wang c ,Mei-Fei Chu aaDepartment of Geosciences,National Taiwan University,Taipei 106,TaiwanbInstitute of Geology and Geophysics,Chinese Academy of Sciences,Beijing 100029,China cInstitute of Geology,Chinese Academy of Geological Sciences,Beijing 100037,China dInstitute of Earth Sciences,Academia Sinica,Taipei 115,Taiwana b s t r a c ta r t i c l e i n f o Article history:Received 1May 2008Received in revised form 15January 2009Accepted 23February 2009Available online 9March 2009Keywords:ZirconU –Pb and Hf isotope Tethyan subductionTranshimalayan batholiths TibetTectonic evolutionBefore the Indian collision with Asia,northward subduction of the Neo-Tethyan oceanic lithosphere produced an Andean-type convergent margin in South Asia characterized by arc magmatism starting from the early Jurassic and lasting until the Eocene.The magmatic arc is now represented by widespread intrusive bodies or the so-called Transhimalayan batholiths in the Lhasa terrane of southern Tibet that have been divided into two main magmatic suites,i.e.,the northern plutonic belt and the southern Gangdese Batholith.Their temporal distribution,concerning how exactly the magmatic suites correlate eastwards and then southeastwards around the eastern Himalayan syntaxis,however,remains poorly constrained.Here we report the first combined zircon U –Pb and Hf isotopic study of the Transhimalayan batholiths from the eastern part of the Lhasa terrane (∼95–97.5°E and ∼28.5–30°N),SE Tibet.Zircon U –Pb dating results of 24granitoids indicate that the rocks were emplaced principally in the Early Cretaceous (∼133–110Ma)and subordinately in the Paleocene (∼66–57Ma),long after an older granite intrusion in the earliest Jurassic (∼198Ma).The zircon εHf (T )values range from +5to −20,yielding Hf crustal model ages (T DM C )between 0.8and 2.4Ga that peak at ∼1.7Ga,suggesting a major episode of crustal growth in the Proterozoic and a predominantly,or even exclusively,crustal source for the granitoid petrogenesis.These zircon U –Pb and Hf isotopic constraints,together with whole-rock geochemical characteristics,allow us to correlate them to S-type granitoids in the northern plutonic belt,rather than to the I-type Gangdese Batholith from the central part of the southern Lhasa terrane.In contrast to the Gangdese magmatism that formed with a signi ficant juvenile mantle input related to the Neo-Tethyan subduction,these S-type granitoids show geochemical similarities to the North American Cordilleran Interior batholiths that did not form directly from a subduction setting.Thus,we attribute the petrogenesis of the widespread Early Cretaceous granitoids in the northern belt and eastern Himalayan batholiths to a postcollisional regime due to the Late Jurassic –Early Cretaceous continental collision between the Lhasa and Qiangtang terranes.Under this framework,the S-type magmatism was generated in response to collision-induced crustal thickening,which may have capabilities of causing crustal anatexis by itself in the region.However,continued interplay with the Neo-Tethyan subduction zone processes such as back-arc extension could also have played a crucial,and long-lasting,role in the magma generation.©2009Elsevier B.V.All rights reserved.1.IntroductionIn southern Tibet,the elongated belt of granitoids that occurs in the Lhasa terrane north of the Yarlu –Tsangpo suture (Fig.1)has been termed the “Transhimalayan batholiths ”(Searle et al.,1987;for review and references therein)and widely regarded as a major component of the Andean-type continental margin along South Asia resulting from northward subduction of the Neo-Tethyan oceanic lithosphere before India started colliding with Asia (e.g.,Allègre et al.,1984;Debon et al.,1986;Searle et al.,1987;Harris et al.,1988;Yin and Harrison,2000;Chung et al.,2005;Kapp et al.,2005a;Mo et al.,2005;Wen et al.,2008a ).Knowledge about the precollisional history in this region,however,remains limited because previous studies mostly concen-trated on issues of postcollisional geology in particular those related to uplift of the Tibetan plateaus and surrounding mountain ranges.As part of a systematic study of the precollisional Transhimalayan magmatism (Chu et al.,2006;Lee et al.,2007,2009-this volume;Liang et al.,2008;Wen et al.,2008a,2008b ),this paper reports for the first time a combined in-situ analysis of zircon U –Pb ages and Lu –Hf isotopes for the eastern Transhimalayan batholiths,emplaced in the easternmost part of the Lhasa terrane,the southeastern Tibetan plateau (Fig.1).The results signi ficantly improve our understanding of the age distribution and geochemical characteristics of the eastern Transhimalayan batholiths,Tectonophysics 477(2009)3–19⁎Corresponding author.E-mail address:sunlin@.tw (S.-L.Chung).0040-1951/$–see front matter ©2009Elsevier B.V.All rights reserved.doi:10.1016/j.tecto.2009.02.034Contents lists available at ScienceDirectTectonophysicsj o u r n a l h o m e p a g e :w ww.e l s ev i e r.c o m /l o c a t e /t e c towhich,together with our comparative studies from the central part of the Lhasa terrane,shed new insights into not only the magmatic but also tectonic evolution in southern Tibet before the India –Asia collision.2.Background and samplesThe Lhasa terrane is widely believed to have dispersed from Gondwana during the Permian or Triassic (Allègre et al.,1984;Chang et al.,1986),then drifted northward and finally collided with the Qiangtang terrane during the Late Jurassic –Early Cretaceous (Kapp et al.,2005a ).The terrane is bordered by the Bangong –Nujiang suture in north and by the Yarlu –Tsangpo suture in south (Fig.1),with the latter resulting from closure of the Neo-Tethys ocean owing to the continental collision between India and Asia (Yin and Harrison,2000).The Transhimalayan batholiths,distributing for a distance of ∼2500km in the Lhasa terrane,have been divided into two principal magmatic suites:(1)a southern Gangdese belt represented by the gigantic Gangdese Batholith that consists dominantly of Late Cretaceous to Eocene granotoids with I-type geochemical composition (Debon et al.,1986;Searle et al.,1987;Wen et al.,2008a ),and (2)a northern plutonic belt of peraluminous or S-type granitoids such as those exposed in the Nyainqentanghla Range that were emplaced largely in the Early Cretaceous (Xu et al.,1985;Harris et al.,1990;Kapp et al.,2005b ).It is generally thought that the Gangdese Batholith,and associated Linzizong volcanic successions (Fig.1),are the magmatic products by northward subduction of the Neo-Tethyan oceanic lithosphere under South Asia.However,the petrogenesis of the northern plutonic belt remains an issue of debates and different models have been proposed,including:(1)crustal anatexis during and/or postdating the con-tinental collision between the Lhasa and Qiangtang terranes (Xu et al.,1985;Pearce and Mei,1988),(2)high-temperature crustal melting due to upwelling of the asthenosphere after the Lhasa –Qiangtang collision (Harris et al.,1990),and (3)a low-angle,or “shallow,”subduction of the Neo-Tethyan oceanic lithosphere (Coulon et al.,1986;Ding et al.,2003;Zhang et al.,2004;Kapp et al.,2005a;Chu et al.,2006).Besides,how these magmatic belts correlate to the east,and then southeastwards around the eastern Himalayan syntaxis,the answer is uncertain because there have been few studies on the eastern Transhimalayan batholiths east of 95°E since the pioneering works carried out in the late seventies (Tu et al.,1982).Therefore,we conducted field excursions along the main roads in the area from Bomi to Basu and Chayu (Shama),between ∼95–97.5°E and ∼30–28.5°N,SE Tibet (Fig.1),through which 80+granitoids of the eastern Transhimalayan batholiths were recovered for detailed petrochemical analyses (e.g.,Lin et al.,2007).The granitoids are generally fresh looking and composed mainly of median-grained monzogranites,granodiorites and granites that contain rather sim-ple mineral constituents including K-feldspar (∼40%),plagioclase (∼30%),quartz (∼20%),biotite (∼5–10%)and accessary minerals such as hornblende,muscovite,apatite and opaque phases.24among the samples were subjected to in-situ zircon U –Pb and Hf isotopic determination by this study.For the convenience,we divide the northwest –southeast-trending Transhimalayan batholiths inthisFig.1.Simpli fied geological map showing the sample localities and distribution of major intrusive bodies in the eastern Lhasa terrane,SE Tibet (Pan et al.,2004).New zircon U –Pb age results conducted by this paper and those reported by Booth et al.(2004)are given in the parentheses and hexagons,respectively (numbers in Ma).BNS —Bangong –Nujiang suture;YTS —Yarlu –Tsangpo suture;STDS —South Tibet Detachment System.4H.-Y.Chiu et al./Tectonophysics 477(2009)3–19region into three groups,namely,from north to south,the Basu –Ranwu,Bomi –Ranwu and Chayu –Shama batholiths (Fig.1).3.Analytical methodsZircons were separated from ∼3kg samples using conventional heavy-liquid and magnetic separation techniques.Cathodolumines-cence (CL)images (Fig.2)were taken at the Institute of Earth Sciences,Academia Sinica,Taipei for examining the internal structures of individual zircon grains and selecting suitable positions for U –Pb and Lu –Hf isotope determinations.3.1.Zircon U –Pb geochronologyZircon U –Pb isotopic analyses were performed using two analytical methods,the sensitive high-resolution ion microprobe (SHRIMP)and the laser ablation-inductively coupled plasma –mass spectrometry (LA-ICPMS).These include 10age results that were obtained using the SHRIMP II equipped at the Beijing SHRIMP Center,Institute of Geology,Chinese Academy of Geological Sciences and reported in Liang et al.(2008)for tracing the source provenances of the Irrawaddy detrital zircons,and 14new age data that were obtained by the LA-ICPMS recently set up at the Department of Geosciences,National Taiwan University.The analytical procedures for the SHRIMP method were same as those reported in Wen et al.(2008a).We describe below the LA-ICPMS techniques,using an Agilent 7500s quadrupole ICPMS and a New Wave UP213laser ablation system housed at NTU.The operating conditions and data acquisition parameters are summarized in Table 1.The laser ablation was performed with a helium carrier gas that can substantially reduce the deposition of ablated material onto the sample surface and greatly improve transport ef ficiency,and thus increase the signal intensities,as compared to “conventional ”ablationusing argon as the carrier gas (Eggins et al.,1998;Günther and Heinrich,1999;Jackson et al.,2004).During the experiments,about 1min was spent for measuring gas blank and the results indicate sensitivities of less than 1000counts per second (cps)for all measured isotopes.Calibration was performed using the GJ-1zircon standard (provided by the Australian Research Council National Key Centre for Geochemical Evolution and Metallogeny of Continents,atMacquarieFig.2.Cathodoluminescence (CL)images of representative zircons separate from the studied samples.Circles indicate the positions of LA-ICPMS U –Pb age analyses,sizing 30–50µ-MC-ICPMS Hf isotope analyses were performed on the same positions with a slightly larger,∼50–65µm,laser beam size.Table 1LA-ICPMS operating conditions and data acquisition parameters.Agilent 7500s ICPMS Forward power 1500W Gas flow rate:Plasma (Ar)15L/min Auxiliary (Ar)1L/minCarrier (He)0.65–0.7L/min Make-up (Ar)1.3–1.4L/min Oxide ion (ThO +/Th +)≦0.3%New Wave UP213laser ablation system Wavelength 213nm UV Repetition rate 4and 5Hz Spot size 30–50μm Energy8–14J/cm 2Data acquisitionData acquisition protocol Time-resolved analysis (TRA)Scanning modePeak hopping,1point per peakDwell time per isotope:206Pb 15ms 207Pb 30ms 208Pb 30ms 232Th 10ms 238U15msData acquisition time130s (60s gas blank and 70s ablation)5H.-Y.Chiu et al./Tectonophysics 477(2009)3–19University,Sydney),well established for a precise207Pb/206Pb age and an intercept age using isotope-dilution thermal ionization mass spectrometry(ID-TIMS)at608.5±0.4Ma(2σ)and608.5±1.5Ma (2σ),respectively(Jackson et al.,2004).The Harvard reference zircon 91500and Australian Mud Tank Carbonatite zircon(MT)were used as secondary standards for data quality control.All U–Th–Pb isotope ratios were calculated using the GLITTER4.0(GEMOC)software,and common lead was corrected using the common lead correction function proposed by Anderson(2002).The weighted mean U–Pb ages and concordia plots were carried out using Isoplot v.3.0(Ludwig, 2003).Our LA-ICPMS results for the secondary zircon standards91500and MT,obtained during April2006and October2007,are shown in the concordia diagrams(Fig.3).The intercept,206Pb/238U and207Pb/206Pb ages of zircon91500are1061.8±2.1Ma(2σ),1062.1±1.7Ma(2σ) and1066.7±2.1Ma(2σ),respectively(Fig.3a–c),which are in perfect accordance with the consensus results reported by Wiendenbeck et al. (1995)using ID-TIMS method that gave207Pb/206Pb age at1065.4±0.6Ma(2σ)and206Pb/238U age at1062.4±0.8Ma(2σ).The intercept,206Pb/238U and207Pb/206Pb ages of zircon MT are736.8±5.2Ma(2σ), 734.0±2.4Ma(2σ)and739.2±8.1Ma(2σ),respectively(Fig.3d–f), also well match the U–Pb concordia intercept age of732±5Ma reported by Black and Gulson(1978)using ID-TIMS method. Additionally,before starting routine U–Pb dating analysis,we established three“in-house”secondary zircons,which are igneous zircons separated from three Gangdese I-type granites,samples ST129A,ST143A and ST147A,whose206Pb/238U ages have been measured using the SHRIMP method that gave94.1±2.4Ma(2σ), 84.8±1.6Ma(2σ)and50.6±0.7Ma(2σ),respectively(Wen et al., 2008a).Our LA-ICPMS results of206Pb/238U ages of these three in-house zircon standards are96.4±0.6Ma(2σ),86.2±0.6Ma(2σ)and 49.9±0.2Ma(2σ),respectively(Fig.4),which are all in good consistency with the SHRIMP results.3.2.Zircon Lu–Hf isotopic measurementsIn-situ Lu–Hf isotopic measurements were subsequently per-formed by the LA-MC-ICPMS method using a Thermo FinniganNeptune Fig.3.U–Pb concordia diagrams of the zircon standards,(a)91500and(d)MT,analyzed by the LA-ICPMS at NTU.The long-term results and weighted averages of206Pb/238U and 207Pb/206Pb ages are also shown.6H.-Y.Chiu et al./Tectonophysics477(2009)3–19multicollectors-ICPMS and a Geolas CQ 193nm laser ablation system housed at the Institute of Geology and Geophysics,Chinese Academy of Sciences,Beijing.Detailed descriptions for the analytical techniques can be found in Wu et al.(2006).The Lu –Hf isotopes were measured on the dated spots of individual zircons to minimize zoning effect but the laser ablation size is ∼50–65µm,slightly larger than that of preexisting pits,sizing 30–50mm,made by the U –Pb dating.4.Analytical results 4.1.Zircon U –Pb agesThe zircons are mostly euhedral and reveal long to short prismatic forms (Fig.2),with average crystal lengths of ∼150–300µm and length-to-width ratios from 2:1to 3:1.Most zircons are transparent,colorless to pale brown and show oscillatory zoning indicative of mag-matic growth (Hoskin and Schaltegger,2003).Zircons with rounded or oval shape and complex internal textures are rare.Thus,the interpretation of the zircon U –Pb isotopic data (see below)is simple and the obtained ages are interpreted as representing the crystal-lization time of the zircons dated and thus the emplacement timing of the host rocks.The U –Pb age results are plotted in terms of concordia diagrams in Fig.5and summarized together with the coordinates of sample localities and rock types in Table 2.These include the 206Pb/238U ages of 14samples,based on a total of 215individual zircon analyses,obtained by the LA-ICPMS method and those of 10remaining samples,on 90zircon grains,reported in Liang et al.(2008)using the SHRMIP method.Given that in zircon U –Pb isotopic analysis,precise age measurements using 207Pb/235U and 207Pb/206Pb ratios are feasible usually only for Precambrian zircons,due essentially to the fact that 235U comprises less than 1%of natural U and thus relatively little 207Pb can be produced in the Phanerozoic (cf.Ireland and Williams,2003),the weighted mean of pooled 206Pb/238U ages are taken to represent the crystallization ages of the dated samples.As shown in Fig.5,all the mean ages are given at 95%con fidence level,i.e.,2s analytical uncertainties;coupled with their mean square of weighted deviates (MSWD).All U –Pb and Lu –Hf isotopic data are listed in Table 3.In the Basu –Ranwu batholiths (Fig.5a –h),eight samples dated indicate that the rocks were emplaced mainly in the Early Cretaceous (∼130–115Ma)except a granite (sample ET223A)that shows an apparently older emplacement age of ∼198Ma (197.7±1.5Ma;Fig.5b)in the Jurassic.In the Bomi –Ranwu batholiths,there are ten samples being dated and eight of them exhibit Early Cretaceous U –Pb ages clustering around ∼125–109Ma.Two remaining samples,i.e.,ET122E and ET014C (Table 2),show younger ages in the latest Cretaceous (∼66Ma)and Paleocene (∼59Ma),respectively.Six samples from the Chayu –Shama batholiths were dated and the results show two age populations (Table 2).These are a group of four granite samples from areas around the Chayu township (Fig.1)that yielded Early Cretaceous ages (∼133Ma)and another group of two samples from areas close to Shama that show younger ages in the Paleocene (∼60Ma).4.2.Zircon Hf isotopic ratiosA total of 305dated zircon grains from the 24samples were analyzed for 176Hf/177Hf isotopic ratios.The results are listed in Table 3and e Hf data are plotted in Fig.6,8and 9.The εHf (T )value,i.e.,parts in 104deviation of initial 176Hf/177Hf isotopic ratios between the sample and the chondritic uniform reservoir,and T DM C ,the zircon Hf isotope “crustal ”model age based on a depleted-mantle source and an assumption that the protolith of the zircon's host magma has the average continental crustal 176Lu/177Hf ratio of 0.015,were calculated after Grif fin et al.(2002)by utilizing the 176Lu –176Hf decay constants adopted in Scherer et al.(2001).Our conclusions would not be signi ficantly affected if the alternative decay constants proposed by more recent studies were used.In the Basu –Ranwu batholiths (Fig.6a –h),magmatic zircons exhibit exclusively negative εHf (T )values ranging from −1.9to −18.1except an “outlier ”grain from sample ET234A that shows positive and the highest value of +0.5(Fig.6d).The zircon εHf (T )values are uniform within each sample,generally b 4−εunit variations,i.e.,about the same as the external errors of the LA-MC-ICPMS analyses (Wu et al.,2006),except the Jurassic sample ET223A that displays a larger variation and the lowest εHf (T )value of −18.1(Fig.6b).In the Bomi –Ranwu batholiths (Fig.6i –r),zircon εHf (T )values show a slightly wider range from +5.0to −15.1.In addition,there are three samples,i.e.,ET122E (Fig.6k),ET120B (Fig.6m)and ET117B (Fig.6r),containing zircons that show positive εHf (T )values.Zircons from the Chayu –Shama batholiths,have exclusively negative εHf (T )values ranging from −4.4to −20.0(Fig.6s –x).Occasionally,Fig. 4.U –Pb concordia diagrams of three in-house Gangdese zircon standards,(a)ST129A,(b)ST143A and (c)ST147A,analyzed by the LA-ICPMS at NTU.The SHRIMP results are also listed for comparison.7H.-Y.Chiu et al./Tectonophysics 477(2009)3–198H.-Y.Chiu et al./Tectonophysics477(2009)3–19Fig.5.Concordia diagrams of zircon U–Pb results of the eastern Himalayan batholiths.Note that the“mean”values of each sample are206Pb/238U ages in Ma.there are grains of inherited zircon detected in some samples,which are listed in Table 3but not plotted in Fig.6.5.Discussion5.1.Age distribution of the eastern Transhimalayan batholithsOur results,combined with five zircon U –Pb ages reported by Booth et al.(2004)from the Bomi –Ranwu batholiths (Fig.1)were plotted as an age histogram (Fig.7a)that illustrates a major intrusive stage in the Early Cretaceous (∼133–110Ma)and a subordinate stage in the Paleocene (∼66–57Ma).This,however,does not imply the absence of igneous activity during Late Cretaceous time because the current dataset is far from being complete for making a conclusion on the age distribution.In fact,Liang et al.(2004)reported a detrital zircon study along the Yarlu –Tsangpo river system showing that zircons aged ∼80–90Ma are abundant in riverbank sediments recovered from the Parlung river flowing around the Bomi township.An inference is hence that magmatic rocks of Late Cretaceous ages,although not sampled so far from the surface exposures,are likely present in the region.Collectively,the age distribution pattern of the eastern Himalayan batholiths resembles in general to that of the northern plutonic belt (Fig.7b)but differs from that of the Gangdese Batholith (Fig.7c).The latter,emplaced in the southern margin of the central Lhasa terrane,shows a predominant stage of magmatism,or a magmatic “flare-up ”event at ∼50Ma,in early Eocene time (Lee et al.,2007,2009-this volume;Wen et al.,2008a ).In addition,this study identi fies the first outcrop of Jurassic igneous rocks from the eastern Lhasa terrane,i.e.,a granite body aged ∼198Ma in the Basu –Ranwu batholiths.Regardless of the scarcity,Jurassic magmatic records that are preserved/exposed sporadically in the central part (∼84–94°E)of the Lhasa terrane have been reported in numerous recent studies using the zircon U –Pb method.These include:(1)Liang et al.(2004)that first reported the existence of abundant Jurassic inherited zircons (aged ∼194–169Ma)in a Cretaceous tuffaceous sandstone from the Gangdese belt;(2)Kapp et al.(2005b)reporting abundant Jurassic inherited zircons (∼204–181Ma)in S-type granitoids from the Nyainqentanglha Range;(3)Chu et al.(2006)reporting Jurassic inherited zircons (∼203–193Ma)in two S-type granites from the northern plutonic belt,together with identifying an ∼188Ma granite body in the Gangdese Batholith;(4)Zhang et al.(2007a,b)reporting an ∼178Ma intrusion in the Gangdese Batholith and two Jurassic S-type granites (∼205and 202Ma)in the southern margin of the northern plutonic belt;(5)Leier et al.(2007)reporting Jurassic and older detrital zircons (∼180–220Ma)in the Tekena Formation;(6)Zhu et al.(2008)reporting a Jurassic dacite (∼174Ma)in the Yeba Formation,and (7)Wu et al.(2009)reporting abundant Jurassic detrital zircons (∼190–150Ma)from the Late Cretaceous Xigaze fore-arc basin.5.2.Zircon Hf isotopic characteristics of Transhimalayan batholiths U –Pb and Hf isotope data of magmatic zircons from the eastern Himalayan batholiths are plotted in Fig.8,from which several important features are observed:(1)they are dominated by negative εHf (T )values,(2)they show a wide spectrum of εHf (T )values ranging from +5to −18,i.e.,showing an ∼23units of εHf (T )variation,(3)zircons in two Cretaceous samples from the Bomi –Ranwu batho-liths have the highest and positive εHf (T )values,in contrast to those from the Paleocene Chayu –Shama batholiths that show the lowest and negative εHf (T )values,and (4)overall speaking,the Hf isotope compositions of the Chayu –Shama batholiths appear to have become more radiogenic through time,a tendency that is unseen or absent in the Basu –Ranwu and Bomi –Ranwu batholiths.Furthermore,also shown in Fig.8,the overall distribution pattern of the eastern Himalayan batholiths emplaced in the eastern Lhasa terrane differs markedly from that of the Gangdese Batholith from the southern part of the central Lhasa terrane (Chu,2006;Chu et al.,2006,in press;Zhang et al.,2007a;Wen et al.,2008a ).Hence,in contrast to the Gangdese Batholith that requires involvement of a depleted or juvenile mantle as the major source component in the magma generation,af filiated closely with the Neo-Tethyan subduction zoneTable 2Summary of sample localities and zircon U –Pb ages of the eastern Himalayan batholiths,SE Tibet.SampleLongitude (°E)Latitude (°N)Elevation (m)Rock type Age (Ma)±2σBasu –Ranwu batholiths ET224A 96.682029.90013843granite 130.00.9ET223A 96.686929.86903870granite197.7 1.5ET225A 96.689330.00583770granodiorite 124.00.8ET234A 96.709629.76494140granite 114.80.7ET234C 96.709629.76494140enclave115.70.6ET226A 96.725230.03213668granodiorite 125.30.7ET227A 96.786830.03833462granite 122.70.7ET227C 96.786830.03833462andesite127.60.6Bomi –Ranwu batholiths ET014C a 95.070030.11002176granite58.7 3.1ET103B a 95.384529.95422636granite (deformed)118.6 2.0ET122E 95.697129.76493745granite66.1 1.1ET125B a 95.716329.75654072granite (deformed)125.1 1.5ET120B a 96.020929.74173021granite 109.0 1.1ET104A a 96.604429.50753773granite 114.8 1.7ET219A 96.851529.39224201granite 125.00.6ET218A 96.868329.38554297granite 122.50.8ET106A a 96.869029.38554207granite 122.5 1.5ET117B a97.134329.32133848granite 116.9 1.8Chayu –Shama batholiths ET113B a 97.085228.56161644granite 59.1 3.2ET207A 97.086528.56391701granite56.50.4ET115E a97.249628.59911896granite (deformed)133.1 1.3ET210A 97.366128.61912166granite (deformed)130.40.7ET215A 97.403428.98492884granite 132.90.8ET116A a97.469828.67242319granite 132.60.9aSHRIMP results from Liang et al.(2008).9H.-Y.Chiu et al./Tectonophysics 477(2009)3–19Table3U–Pb and Hf isotope data of igneous zircons from eastern Himalayan batholiths.Spot Th/U206Pb/238U±1σ207Pb/235U±1σError corr.206Pb/238Uage(Ma±1σ)176Hf/177Hf±1σ176Lu/177Hf±1σεHf(T)±1σT DM T DMCET224A wt.mean=130.0±0.9Ma(2σ)ET224A-010.81300.02030.00020.13570.00200.6840129.0 1.00.28244360.0011084−8.910.2011471752 ET224A-020.87720.02050.00020.13990.00250.5663131.0 1.00.28240260.0007781−10.290.2311941841 ET224A-030.84750.02060.00020.13900.00230.6082131.0 1.00.28240770.00107011−10.130.2611961830 ET224A-04 1.51520.02010.00020.13830.00240.6087128.0 1.00.28240470.00134213−10.340.2512101841 ET224A-070.71940.02000.00020.13560.00220.6076128.0 1.00.28240160.0008064−10.400.2211971845 ET224A-080.38610.03590.00040.26600.00320.8034227.0 2.00.28237860.0023061−9.300.2212791849 ET224A-090.78130.02020.00020.14260.00320.4844129.0 1.00.282444100.0006035−8.820.3611301746 ET224A-11 1.49250.02060.00020.14380.00280.5460131.0 1.00.28242370.0007087−9.530.2611621792 ET224A-12 1.12360.02030.00020.13180.00310.4752130.0 1.00.28241370.0009214−9.930.2511831817 ET224A-140.94340.02060.00020.13920.00390.4135131.0 2.00.28239980.0007041−10.380.2911961846 ET224A-180.72460.02050.00020.13740.00230.6208131.0 1.00.28238770.0008233−10.800.2612161873 ET224A-200.37170.07160.00070.56560.00620.8736446.0 4.00.28240280.0014007−3.670.2912131659 ET224A-23 1.26580.02070.00020.14320.00170.8004132.0 1.00.28243580.0013404−9.130.2711651768 ET224A-240.56180.10810.0011 1.03610.01130.8887661.0 6.00.28242480.0008172 1.910.2811651470ET223A wt.mean=197.7±1.5Ma(2σ)ET223A-010.03320.03090.00030.21890.00220.9609196.0 2.00.28242750.0017393−8.130.1911891752 ET223A-020.04760.03170.00030.21970.00240.8950201.0 2.00.28246690.00220418−6.690.3011471664 ET223A-040.15900.05570.00060.42510.00480.8744349.0 3.00.28217780.00111516−13.620.2815192214 ET223A-050.00780.03160.00030.21770.00260.8204201.0 2.00.282141100.00156714−18.110.3515892385 ET223A-070.03940.03180.00030.22260.00330.6834202.0 2.00.282468130.00157123−6.510.4511251654 ET223A-100.05270.05330.00060.40560.00540.8273335.0 4.00.282488100.0020216−3.130.3511101540 ET223A-110.46300.03120.00030.22200.00530.4197198.0 2.00.28243080.0021789−8.050.2811991748 ET223A-130.03880.03090.00030.22360.00250.8501196.0 2.00.282537130.0020548−4.270.4510411508 ET223A-140.87720.09850.00100.80530.00970.8389605.0 6.00.28228590.0008173−4.230.3213591815 ET223A-150.02860.03070.00030.21460.00230.8662195.0 2.00.28234870.0019994−10.960.2513111931 ET223A-160.05800.03130.00030.22420.00240.9212199.0 2.00.28241390.0015325−8.530.3112021780 ET223A-170.02730.03080.00030.21260.00230.9118196.0 2.00.282509100.00339910−5.430.3411221581 ET223A-180.02280.03080.00030.21020.00220.9537195.0 2.00.28240570.0029455−9.090.2612621812 ET223A-190.18180.03080.00030.22290.00250.8866195.0 2.00.282283110.0019946−13.290.4014052077 ET223A-200.01920.03120.00030.21610.00230.9310198.0 2.00.282498120.00275359−5.710.4311191600 ET223A-210.27250.03420.00030.23710.00250.9182217.0 2.00.28248490.0029717−5.860.3111461624 ET223A-220.05000.03130.00030.21460.00240.9054198.0 2.00.282462100.0026686−6.970.3411691680ET225A wt.mean=124.0±0.8Ma(2σ)ET225A-020.80000.01890.00020.13360.00290.4948121.0 1.00.28249170.0007551−7.330.2510691646 ET225A-030.84030.01930.00020.13490.00470.3607123.0 2.00.28248370.0007504−7.590.2510811664 ET225A-050.87720.01950.00020.12310.00460.3326125.0 2.00.28246170.0005621−8.300.2411051710 ET225A-070.90910.01940.00020.13050.00410.3751124.0 1.00.28246980.0007933−8.070.2711011695 ET225A-08 1.01010.01900.00020.13180.00470.3557121.0 2.00.28246180.0006481−8.410.2811081714 ET225A-090.68490.01930.00020.12870.00210.6244123.0 1.00.28244880.0009853−8.850.2711361723 ET225A-100.95240.01920.00020.14140.00210.6785123.0 1.00.28245780.0011991−8.530.2811301723 ET225A-110.72460.01930.00020.13350.00230.5906123.0 1.00.28247870.0009997−7.790.2610951676 ET225A-120.98040.01940.00020.13540.00400.4034124.0 1.00.28247970.0007174−7.690.2410851671 ET225A-130.91740.01990.00020.13380.00330.4468127.0 1.00.28245960.0007861−8.340.2311141714 ET225A-140.90910.01960.00020.12850.00400.3745125.0 1.00.28249980.0007671−6.970.2710581625 ET225A-150.76920.02010.00020.13900.00410.4039128.0 2.00.28247680.0005703−7.730.2810851676 ET225A-16 1.02040.01970.00020.13800.00390.4113126.0 1.00.28248980.0007823−7.330.2710731649 ET225A-170.93460.01950.00020.12770.00410.3699124.0 1.00.28246180.0006751−8.320.2711081711 ET225A-180.98040.01920.00020.13150.00480.3456123.0 2.00.28248180.0006062−7.640.2710791666 ET225A-190.88500.01950.00030.13670.00520.3393124.0 2.00.28247680.0006211−7.810.3010861678 ET225A-200.71940.01940.00020.13000.00240.5980124.0 1.00.28248870.0009076−7.420.2510781653 ET225A-21 1.28210.01940.00020.13870.00420.4068124.0 2.00.28247570.0009921−7.850.2510981681ET234A wt.mean=114.8±0.7Ma(2σ)ET234A-010.70920.01790.00020.12080.00170.6793114.0 1.00.28262270.00124112−2.890.268971358 ET234A-020.78740.01790.00020.11980.00150.7387114.0 1.00.28259570.0016313−3.870.249451420 ET234A-030.69440.01780.00020.12840.00170.7119114.0 1.00.28261970.0013026−3.000.239031365 ET234A-040.78130.01810.00020.12610.00200.6362116.0 1.00.28265080.0011818−1.860.278571294 ET234A-060.67570.01790.00020.12300.00160.7293114.0 1.00.28262270.0016344−2.940.269081361 ET234A-070.63290.01820.00020.12260.00180.6796117.0 1.00.28261860.0011975−2.970.209021365 ET234A-080.66670.01790.00020.11970.00160.7119114.0 1.00.28261180.00224716−3.360.279381388 ET234A-090.72460.01770.00020.12040.00160.7351113.0 1.00.28262670.00193932−2.840.259091354 ET234A-100.88500.01830.00020.12140.00160.7615117.0 1.00.28260780.0014925−3.400.279261393 ET234A-120.75190.01790.00020.11890.00510.2716114.0 1.00.282720150.001727110.540.517681140 ET234A-160.65790.01780.00020.11990.00170.7279113.0 1.00.28261880.00172914−3.110.289161371 ET234A-180.78130.01820.00020.12190.00150.8102116.0 1.00.28261060.0020695−3.350.239361389 ET234A-190.89290.01830.00020.12440.00180.6777117.0 1.00.28264170.0013589−2.180.268741315 ET234A-200.74630.01800.00020.12250.00180.6688115.0 1.00.28261280.0019834−3.280.289301383 ET234A-220.75190.01790.00020.12130.00160.7499114.0 1.00.28262680.00209718−2.810.279121353 ET234A-230.67110.01800.00020.12800.00240.5609115.0 1.00.28262160.00084310−2.890.218901359 10H.-Y.Chiu et al./Tectonophysics477(2009)3–19。