利用浮选柱对煤显微组分的分离
利用浮选柱对煤显微组分的分离
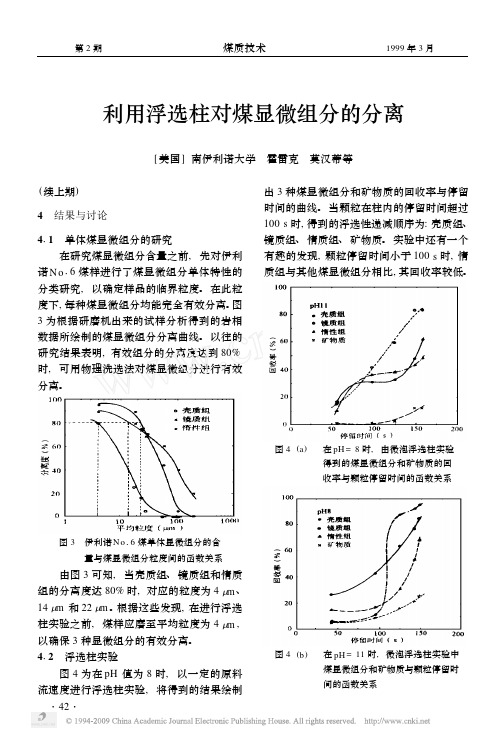
表 2 用 L even sp iel 方程和实验回收率值 (T P = 2146m in, 吹气速率 15% ) 计算的单体显微组 分和矿物质的浮选动态速率
在 pH 值为 11 时, 浮选性递减的顺序变 为: 镜质组、 壳质组、 惰质组、 矿物质, 见
显微组分
pH = 8
k m in
pH = 11
得到的煤显微组分和矿物质的回 收率与颗粒停留时间的函数关系
图 3 伊利诺 N o 16 煤单体显微组分的含 量与煤显微组分粒度间的函数关系
由图 3 可知, 当壳质组、 镜质组和惰质 组的分离度达 80% 时, 对应的粒度为 4 Λm、 14 Λm 和 22 Λm。根据这些发现, 在进行浮选 柱实验之前, 煤样应磨至平均粒度为 4 Λm , 以确保 3 种显微组分的有效分离。 412 浮选柱实验
第 2 期 煤质技术 1999 年 3 月
利用浮选柱对煤显微组分的分离
[ 美国 ] 南伊利诺大学 霍雷克 莫汉蒂等
(续上期)
4 结果与讨论
411 单体煤显微组分的研究 在研究煤显微组分含量之前, 先对伊利
诺 N o 16 煤样进行了煤显微组分单体特性的 分类研究, 以确定样品的临界粒度。 在此粒 度下, 每种煤显微组分均能完全有效分离。图 3 为根据研磨机出来的试样分析得到的岩相 数据所绘制的煤显微组分分离曲线。 以往的 研究结果表明, 有效组分的分离度达到 80% 时, 可用物理洗选法对煤显微组分进行有效 分离。
7156 4147 3123 3111 7114 3150 5171 1214 8121 1917 1819 2011 5018 1614
9117 2182 1144 1141 5177 1151 4115 1911 3150 4613 1313 6319 1815 6914
塔里木盆地煤岩生物降解的生物标志化合物证据
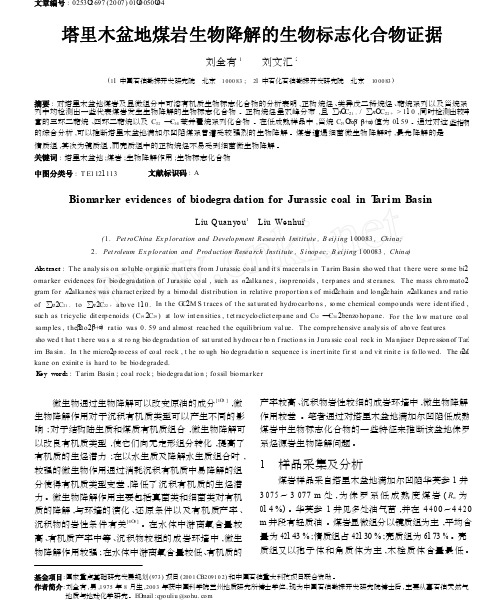
文章编号: 0253Ο2697 (2007) 01Ο0050Ο04塔里木盆地煤岩生物降解的生物标志化合物证据刘全有1刘文汇2( 11 中国石油勘探开发研究院北京100083 ; 21 中石化石油勘探开发研究院北京100083)摘要: 对塔里木盆地煤岩及显微组分中可溶有机质生物标志化合物的分析表明,正构烷烃、类异戊二稀烷烃、萜烷系列以及甾烷系列中均检测出一些代表煤岩发生生物降解的生物标志化合物。
正构烷烃呈双峰分布,且∑nΟC21 - / ∑nΟC22+ > 11 0 ,同时检测出较丰富的三环二萜烷、四环二萜烷以及C32 —C34 苯并藿烷系列化合物。
在低成熟样品中,甾烷C29Ο5αβ/ (β+α) 值为01 59 。
通过对这些指标的综合分析,可以推断塔里木盆地满加尔凹陷煤系曾遭受较强烈的生物降解。
煤岩遭遇细菌微生物降解时,最先降解的是惰质组,其次为镜质组,而壳质组中的正构烷烃不易受到细菌微生物降解。
关键词: 塔里木盆地;煤岩;生物降解作用;生物标志化合物中图分类号: T E1121 113 文献标识码: ABiom arker evidences of biodegra dat i on f or Jura s sic coal in T ari m Ba s inL i u Q u a n yo u1 L i u We n h u i2( 1. Pet roChi n a E x p l o r at i o n a n d De v el o p m e nt Rese a r c h I nst i t u te , B ei j i n g100083 , Chi n a;2 . Pet role u m E x p l o r at i o n a n d P r o d uct i o n Rese a r c h I n st i t ute , S i no p ec , B ei j i n g100083 , Chi n a)Abstract : The analy si s o n so l u ble o r g anic mat t er s f r o m J ura s sic co al and it s maceral s i n Tarim Ba s in sho w ed t h at t h ere were so m e bi2 o m ar k er evidence s fo r bio d egradatio n of J ura s sic co al , such a s n2al k a n es , i s op r enoids , t erp a n e s a n d st erane s. The ma s s ch ro m ato2 gram fo r n2al k a n es wa s charact erized by a bi m o d al di s t r ibutio n in relative p r opo r tio n s of mid2chain and lo n g2chain n2al k a n e s a n d ratio ∑n2C21 - ∑n2C22 + ab o v e 11 0.In t h e G C2M S t r ace s of t h e sat u rat ed hydro ca r b o n s , so m e chemical co m po u nds were i dent if ied , of tosuch a s t r icyclic dit erp e noids ( C19 2C29 ) at lo w int en sitie s , t et r acyclo clict erp a n e a n d C32 —C34 2benzo h op a n e .Fo r t h e lo w mat u re co al samp le s , t h eβ2to2(β+α) ratio wa s 0 . 59 a n d al m o s t reached t h e equili brium val ue .The co m p r ehen sive analy si s of ab o v e f e at u ressho wed t hat t here wa s a st ro ng bio degradatio n of sat urat ed h ydro ca r b o n f ractio n s in J ura ssic co al rock in Ma njiaer Dep re ssio n of Tar2 im Ba sin. In t he micro2p ro cess of co al rock , t he ro ugh bio degradatio n sequence i s inert inite f ir st a nd vit rinit e i s fo llo wed. The n2al2 kane o n exinit e i s ha r d to be bio d egraded.K ey words : Ta r im Ba s in ; co al rock ; bio d egra dat io n ; fo s sil bio m a r k er微生物通过生物降解可以改变原油的成分[ 1Ο3 ] ,微生物降解作用对于沉积有机质类型可以产生不同的影响;对于结构陆生质和煤质有机质组合,微生物降解可以改良有机质类型,使它们向无定形组分转化,提高了有机质的生烃潜力;在以水生质及降解水生质组合时, 较强的微生物作用通过消耗沉积有机质中易降解的组分使得有机质类型变差,降低了沉积有机质的生烃潜力。
煤的分离实验报告
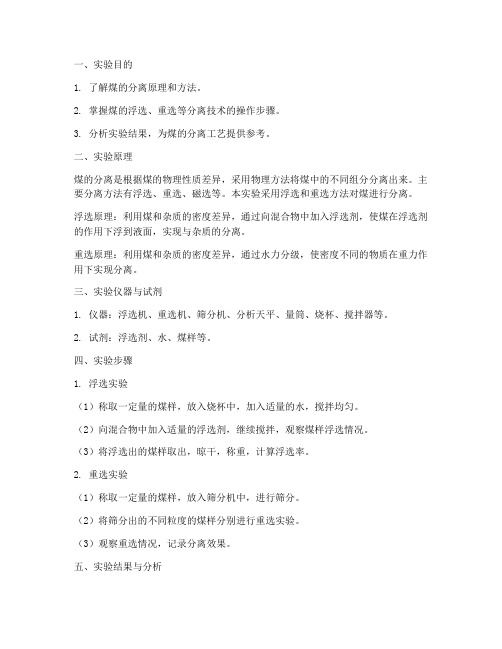
一、实验目的1. 了解煤的分离原理和方法。
2. 掌握煤的浮选、重选等分离技术的操作步骤。
3. 分析实验结果,为煤的分离工艺提供参考。
二、实验原理煤的分离是根据煤的物理性质差异,采用物理方法将煤中的不同组分分离出来。
主要分离方法有浮选、重选、磁选等。
本实验采用浮选和重选方法对煤进行分离。
浮选原理:利用煤和杂质的密度差异,通过向混合物中加入浮选剂,使煤在浮选剂的作用下浮到液面,实现与杂质的分离。
重选原理:利用煤和杂质的密度差异,通过水力分级,使密度不同的物质在重力作用下实现分离。
三、实验仪器与试剂1. 仪器:浮选机、重选机、筛分机、分析天平、量筒、烧杯、搅拌器等。
2. 试剂:浮选剂、水、煤样等。
四、实验步骤1. 浮选实验(1)称取一定量的煤样,放入烧杯中,加入适量的水,搅拌均匀。
(2)向混合物中加入适量的浮选剂,继续搅拌,观察煤样浮选情况。
(3)将浮选出的煤样取出,晾干,称重,计算浮选率。
2. 重选实验(1)称取一定量的煤样,放入筛分机中,进行筛分。
(2)将筛分出的不同粒度的煤样分别进行重选实验。
(3)观察重选情况,记录分离效果。
五、实验结果与分析1. 浮选实验结果实验结果显示,浮选剂加入后,煤样浮选效果较好,浮选率较高。
说明浮选方法可以有效分离煤中的杂质。
2. 重选实验结果实验结果显示,不同粒度的煤样在重选过程中,分离效果明显。
较细的煤样重选效果较好,而较粗的煤样重选效果较差。
六、实验结论1. 煤的分离实验结果表明,浮选和重选方法可以有效分离煤中的杂质。
2. 浮选方法对煤的分离效果较好,适用于高杂质的煤样。
3. 重选方法对不同粒度的煤样分离效果明显,适用于不同粒度的煤样分离。
七、实验注意事项1. 实验过程中,注意安全,防止意外事故发生。
2. 严格按照实验步骤进行操作,确保实验结果的准确性。
3. 实验过程中,注意观察实验现象,及时调整实验参数。
八、实验总结本次实验通过对煤的分离实验,了解了煤的分离原理和方法,掌握了浮选、重选等分离技术的操作步骤。
ccf 浮选柱回收技术
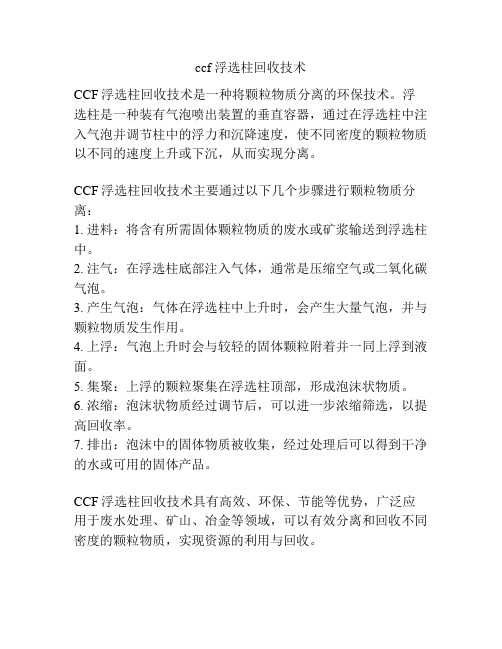
ccf 浮选柱回收技术
CCF浮选柱回收技术是一种将颗粒物质分离的环保技术。
浮选柱是一种装有气泡喷出装置的垂直容器,通过在浮选柱中注入气泡并调节柱中的浮力和沉降速度,使不同密度的颗粒物质以不同的速度上升或下沉,从而实现分离。
CCF浮选柱回收技术主要通过以下几个步骤进行颗粒物质分离:
1. 进料:将含有所需固体颗粒物质的废水或矿浆输送到浮选柱中。
2. 注气:在浮选柱底部注入气体,通常是压缩空气或二氧化碳气泡。
3. 产生气泡:气体在浮选柱中上升时,会产生大量气泡,并与颗粒物质发生作用。
4. 上浮:气泡上升时会与较轻的固体颗粒附着并一同上浮到液面。
5. 集聚:上浮的颗粒聚集在浮选柱顶部,形成泡沫状物质。
6. 浓缩:泡沫状物质经过调节后,可以进一步浓缩筛选,以提高回收率。
7. 排出:泡沫中的固体物质被收集,经过处理后可以得到干净的水或可用的固体产品。
CCF浮选柱回收技术具有高效、环保、节能等优势,广泛应用于废水处理、矿山、冶金等领域,可以有效分离和回收不同密度的颗粒物质,实现资源的利用与回收。
煤显微组分荧光光谱测定方法

煤显微组分荧光光谱测定方法一、样品制备
1. 样品采集:选择具有代表性的煤样,确保样品无污染。
2. 样品处理:将煤样破碎至一定粒度,以便于后续的显微组分分离。
3. 显微组分分离:利用显微镜技术将煤样中的显微组分分离出来,分别收集。
二、荧光光谱仪操作
1. 仪器开机:先打开稳压电源,再打开仪器电源。
2. 波长校正:根据实验需求,校正激发和发射波长。
3. 激发方式选择:根据不同的显微组分,选择合适的激发方式。
4. 样品测量:将分离好的显微组分放入样品池中,进行荧光光谱测量。
5. 数据记录:记录不同显微组分的荧光光谱数据。
三、激发波长选择
1. 根据研究目的确定合适的激发波长范围。
2. 针对不同显微组分,选择能产生最大荧光强度的激发波长。
3. 注意排除非目标组分的干扰荧光。
四、发射波长扫描
1. 对选定的激发波长进行扫描,获取不同显微组分的荧光光谱。
2. 注意观察荧光光谱的峰值和形状,为后续解析提供依据。
五、荧光光谱解析
1. 根据荧光光谱的峰值和形状,识别不同的显微组分。
2. 分析荧光光谱的变化规律,推断组分之间的相互影响。
六、组分含量计算
1. 利用标准曲线法或内标法计算各显微组分的含量。
2. 确保计算方法的准确性和可靠性。
七、重复性验证
1. 对同一样品进行多次测量,评估方法的重复性。
2. 比较不同实验人员或不同实验条件下获得的结果,验证方法的可靠性。
低阶煤煤岩显微组分分离及其热解气化过程的研究
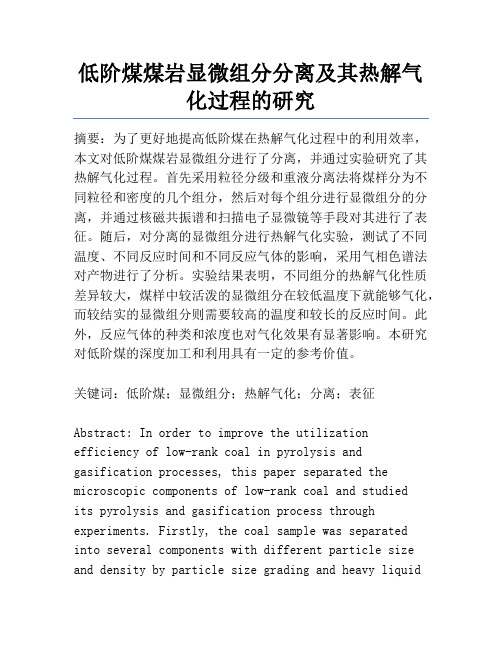
低阶煤煤岩显微组分分离及其热解气化过程的研究摘要:为了更好地提高低阶煤在热解气化过程中的利用效率,本文对低阶煤煤岩显微组分进行了分离,并通过实验研究了其热解气化过程。
首先采用粒径分级和重液分离法将煤样分为不同粒径和密度的几个组分,然后对每个组分进行显微组分的分离,并通过核磁共振谱和扫描电子显微镜等手段对其进行了表征。
随后,对分离的显微组分进行热解气化实验,测试了不同温度、不同反应时间和不同反应气体的影响,采用气相色谱法对产物进行了分析。
实验结果表明,不同组分的热解气化性质差异较大,煤样中较活泼的显微组分在较低温度下就能够气化,而较结实的显微组分则需要较高的温度和较长的反应时间。
此外,反应气体的种类和浓度也对气化效果有显著影响。
本研究对低阶煤的深度加工和利用具有一定的参考价值。
关键词:低阶煤;显微组分;热解气化;分离;表征Abstract: In order to improve the utilizationefficiency of low-rank coal in pyrolysis and gasification processes, this paper separated the microscopic components of low-rank coal and studiedits pyrolysis and gasification process through experiments. Firstly, the coal sample was separatedinto several components with different particle size and density by particle size grading and heavy liquidseparation methods. Then, the microscopic components of each component were separated and characterized by NMR and SEM. Subsequently, the separated microscopic components were subjected to pyrolysis andgasification experiments, and the effects of different temperatures, reaction times, and reaction gases were tested. The products were analyzed by gas chromatography. The experimental results showed that the pyrolysis and gasification properties of different components were quite different. The more active microscopic components in the coal sample could be gasified at lower temperatures, while the more solid microscopic components required higher temperatures and longer reaction times. In addition, the type and concentration of reaction gas also had a significant effect on the gasification effect. This study has certain reference value for the deep processing and utilization of low-rank coal.Keywords: low-rank coal; microscopic components; pyrolysis and gasification; separation; characterizationIn summary, this study shows that the pyrolysis and gasification of low-rank coal can be separated into two stages based on the different gasification temperatures required for the different componentspresent in the coal sample. The microscopic components require higher temperatures and longer reaction times compared to the gaseous components. This finding indicates that the gasification of low-rank coal has complex processes, and the optimization of reaction conditions plays a crucial role in enhancing the gasification efficiency.Moreover, the type and concentration of reaction gas also affect the gasification effect. For example, when CO₂ was used as the reaction gas, the gasificationrate increased due to the interaction between CO₂ and char during the reaction process. Furthermore, the increase in H₂ concentration in the reaction atmosphere enhanced the gasification rate of the coal sample, which was attributed to the higher reactivity of H₂ compared to other gases.In conclusion, this study provides insights into the gasification behavior of low-rank coal and highlights the importance of separating and characterizing different components in the coal sample for optimizing gasification conditions. The findings in this study have certain reference value for the deep processing and utilization of low-rank coalFurthermore, the study suggests that the gasification behavior of low-rank coal is highly dependent on the composition and properties of the coal sample. Therefore, it is important to conduct a comprehensive analysis of various coal components, including moisture, ash, and volatile matter, in order to tailor the gasification process to the specificcharacteristics of the coal.Additionally, the study highlights the potential benefits of using certain gases, such as H₂, for enhancing the gasification rate of low-rank coal. However, it is important to note that the use of H₂may not be practical or cost-effective in certain situations. Therefore, further research is needed to identify alternative gases or enhance the reactivityof other gases for efficient gasification of low-rank coal.The study also emphasizes the importance of optimizing gasification conditions, such as temperature, pressure, and gas flow rate, for efficient gasification of low-rank coal. It is crucial to strike a balance between maximizing gasification rate and minimizingundesirable side reactions, such as methane production or tar formation.Overall, the findings of this study have practical implications for the utilization of low-rank coal as a potential energy source. Gasification of low-rank coal can provide a cleaner and more efficient method of energy production, compared to traditional combustion methods. However, further research is needed to optimize gasification processes, improve gasification efficiency, and reduce environmental impactsThe utilization of low-rank coal as an energy source has several advantages that make it an attractive alternative to higher-rank coals. Low-rank coals are abundant and widely distributed around the world, which makes them potentially less expensive than higher-rank coals. They also have a lower environmental impact, as they contain less sulfur and other impurities than higher-rank coals. In addition, the gasification of low-rank coal can provide a versatile and flexible source of energy that can be used to produce a wide range of products, including electricity, chemicals, and transportation fuels.However, there are also several challenges associated with the utilization of low-rank coal as an energy source. One of the main challenges is the high moisture content of low-rank coals, which can make them more difficult to store and transport. Anotherchallenge is the lower energy density of low-rank coal, which can make it more expensive to extract andprocess than higher-rank coals. In addition, low-rank coals can be more difficult to gasify efficiently, due to their higher reactivity and tendency to produce undesirable side reactions.To address these challenges, further research is needed to optimize gasification processes, improve gasification efficiency, and reduce environmental impacts. One area of research that shows promise isthe development of innovative gasificationtechnologies that can better handle the properties of low-rank coals. For example, researchers are exploring the use of combined gasification and combustion processes, which can improve gasification efficiency and reduce the production of undesirable side reactions.Another area of research that shows promise is the development of integrated gasification combined cycle (IGCC) technologies, which can offer significant advantages over traditional coal-fired power plants. IGCC systems combine gasification with a combinedcycle power plant, which can increase energy efficiency, reduce emissions, and provide a more flexible source of energy. However, the development ofthese technologies is still in its early stages, and further research is needed to optimize their performance and economics.Overall, the utilization of low-rank coal as a potential energy source offers several advantages and challenges that need to be carefully considered. While gasification of low-rank coal can provide a cleaner and more efficient method of energy production, compared to traditional combustion methods, further research is needed to optimize these processes and reduce their environmental impact. With continued innovation and research, low-rank coal could play an important role in meeting the world's energy needs while reducing greenhouse gas emissions and other environmental impactsIn conclusion, low-rank coal is a significant but underutilized energy resource that presents both opportunities and challenges for sustainable development. While it has the potential to provide a cost-effective and reliable source of energy, its extraction and processing can have negative environmental and social impacts. However, through continued innovation and research, low-rank coal could become an important contributor to the global energy mix while reducing its carbon footprint and otherenvironmental impacts. It is imperative that solutions are found to overcome challenges associated with its use, so that the potential benefits can be fully realized。
洗煤机械浮选柱的工作原理
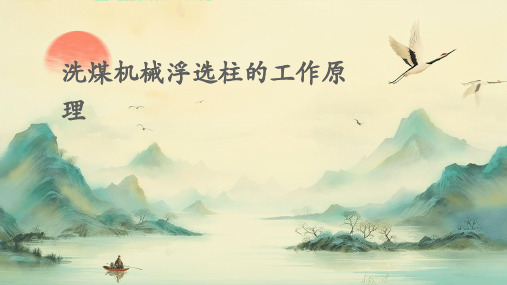
不同地区的煤炭性质存在差异,浮选柱可能无法 完全满足各种煤质的处理需求。
维护成本高
浮选柱内部结构复杂,维护和修理成本较高。
如何克服局限性
优化设计
针对不同粒度、不同性质的煤炭, 优化浮选柱的设计,提高处理效 果。
引入先进技术
采用新型的空气悬浮技术、智能控 制技术等,提高浮选柱的性能和适 应性。
定制化服务
根据客户需求,提供定制化的浮选柱设计和生产服务,满足个性化 需求。
国际化发展
加强国际合作与交流,引进国外先进技术和管理经验,提高浮选柱 的国际竞争力。
对行业的意义与影响
01
提高资源利用率
通过高效能的浮选柱,提高煤炭 资源的利用率,减少浪费和环境 污染。
02
促进产业升级
03
增强国际竞争力
推动洗煤机械行业的产业升级和 技术进步,提高整个行业的生产 效率和产品质量。
浮选柱的工作原理详解
气泡的形成与作用
压缩空气在浮选柱内被分散成微 小气泡,这些气泡附着在煤粒表 面,增加其浮力,促使煤粒上浮。
煤粒的附着与分离
气泡与煤粒的附着程度取决于煤 粒表面的物理化学性质,通过控 制浮选单元的类型和数量,可实
现不同煤粒的有效分离。
流动状态与控制
煤浆在浮选柱内的流动状态影响 气泡与煤粒的接触和分离效果, 通过合理设计塔体结构和控制进 料速度,可实现高效稳定的浮选
洗煤机械浮选柱的工作原 理
• 引言 • 洗煤机械浮选柱的工作原理 • 浮选柱的优点与局限性 • 实际应用与案例分析 • 未来发展与展望
01
引言
目的和背景
01
洗煤机械浮选柱是洗煤过程中的 重要设备之一,其工作原理是利 用浮选柱内的充气和矿浆循环, 使煤和矸石分离。
煤中不同显微组分孔隙结构特征研究
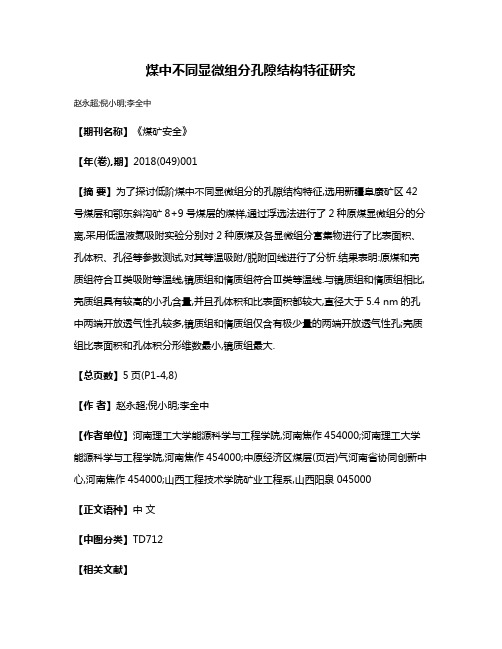
煤中不同显微组分孔隙结构特征研究赵永超;倪小明;李全中【期刊名称】《煤矿安全》【年(卷),期】2018(049)001【摘要】为了探讨低阶煤中不同显微组分的孔隙结构特征,选用新疆阜康矿区42号煤层和鄂东斜沟矿8+9号煤层的煤样,通过浮选法进行了2种原煤显微组分的分离,采用低温液氮吸附实验分别对2种原煤及各显微组分富集物进行了比表面积、孔体积、孔径等参数测试,对其等温吸附/脱附回线进行了分析.结果表明:原煤和壳质组符合Ⅱ类吸附等温线,镜质组和惰质组符合Ⅲ类等温线.与镜质组和惰质组相比,壳质组具有较高的小孔含量,并且孔体积和比表面积都较大,直径大于5.4 nm的孔中两端开放透气性孔较多,镜质组和惰质组仅含有极少量的两端开放透气性孔;壳质组比表面积和孔体积分形维数最小,镜质组最大.【总页数】5页(P1-4,8)【作者】赵永超;倪小明;李全中【作者单位】河南理工大学能源科学与工程学院,河南焦作454000;河南理工大学能源科学与工程学院,河南焦作454000;中原经济区煤层(页岩)气河南省协同创新中心,河南焦作454000;山西工程技术学院矿业工程系,山西阳泉045000【正文语种】中文【中图分类】TD712【相关文献】1.基于压汞和低温氮吸附联合试验的不同变质程度煤全孔隙结构特征研究 [J], 张召召;潘结南;李猛;王凯2.淮北煤田煤中不同显微组分的热解特征研究 [J], 宇天伟; 冯松宝; 闫顺风3.不同变质程度煤孔隙结构特征研究 [J], 范子伟4.煤的不同显微组分傅里叶红外光谱特征研究——以任楼煤矿为例 [J], 董致成;冯松宝;闫顺风5.基于SAXS的不同变质程度煤纳米级孔隙结构特征研究 [J], 张钰;李勇;王延斌;王壮森;赵石虎;韩文龙因版权原因,仅展示原文概要,查看原文内容请购买。
- 1、下载文档前请自行甄别文档内容的完整性,平台不提供额外的编辑、内容补充、找答案等附加服务。
- 2、"仅部分预览"的文档,不可在线预览部分如存在完整性等问题,可反馈申请退款(可完整预览的文档不适用该条件!)。
- 3、如文档侵犯您的权益,请联系客服反馈,我们会尽快为您处理(人工客服工作时间:9:00-18:30)。
镜+ 惰 壳+ 矿 148
3710
148
1619
图 5 伊利诺煤分离煤显微组分的柱浮选流程
由于煤中壳质组仅占很小比例, 因此, 希 望在 pH 值为 8 时, 通过第一步的操作使其 回收率达最大值。 回收近 8711% 的壳质组, 从而使壳质组和镜质组从煤中分离出来。 为 了从镜质组中分离壳质组, 第一步浮选使用 两个附加的净化过程 (柱B 和 C, 见图 5) , 操 作条件为: pH = 8, 停留时间为 125 s。最后 的浮选产品为壳质组, 从净化器和重净化器 中流出的尾液即为镜质组。
8711 2144 6114 4133
8718 2134 5171 4110
3214 3170 5416 9125
2716 3107 5018 1815
50~ 90 美元 t 之间。根据目前煤的市场价格 推算, 煤显微组分可使煤的价格提高约 20~ 60 美元 t, 与其增加的操作成本相比, 煤的 精选在经济上合理。
要是基于显微组分间不同的浮选动力学。 换 诺煤进行的调查发现, 煤显微组分的价格在
言之, 改变系统中不同柱子原料的组成, 不 影响显微组分产物的等级。 然而, 如果浮选 柱在其载流能力极限条件下操作, 由于回流 和选择性分离的机理, 这种情况将不会出现。 通过泡沫层提供的选择机理在浮选柱的额定 能力下运转, 可提高煤显微组分的分离效率。
图 4 (b)。当惰质组的回收率减至约 50% 时, 镜质组的回收率保持不变, 然而壳质组的回 收率变化较大。 主要是因为壳质组粒子表面 能的增大, 从而降低其浮选性。 回收率的降 低与M iller 等人的发现不同。这可能是伊利 诺煤中壳质组主要由孢子体构成, 其憎水性 比树脂体低很多。因此, 在 pH 值较高时, 由 于携带到浮选精煤中的矿物质量减少, 使矿 物质的排除率增高。
壳质组 镜质组 惰质组 矿物质
1192 1103 0157 0113
0130 0197 0126 0105
壳质组动态速率降至 1 位数, 而镜质组 降低并不明显。因此, 这些发现清楚表明, 煤 显微组分浮选性与其表面憎水性和动电性呈 函数关系。
为了验证用离心沉浮分离数据计算煤显 微组分浮选动态速率的准确性, 结合各单体 显微组分的回收率值来计算煤颗粒的 K 值。 用这一 K 值与用同一样品通过高温灰分分 析数据得到的可燃物回收率而确定的 K 值 进 行 比 较。 根 据 在 pH 值 为 8、 停 留 时 间 (T P ) 为 2146 m in 的实验数据比较可知, 当 灰含量从 715% 降至 212% 时, 可燃物的回收 率为 80%。表 3 表明, 两个动态比的值几乎 相等, 即验证了煤显微组分分析的准确性。 413 煤显微组分分离流程
7156 4147 3123 3111 7114 3150 5171 1214 8121 1917 1819 2011 5018 1614
9117 2182 1144 1141 5177 1151 4115 1911 3150 4613 1313 6319 1815 6914
在料流 7 中, 镜质组的含量也由 7915% 提高到 8718%。在镜质组分离第一阶段的浮 选物中, 含有较高的镜质组, 将其与料流 7 混 合可得到较高的收率。 镜质组提纯效果较好 的是壳质组分离的第二阶段。 在此阶段的尾 流中, 镜质组的含量达到 9211%。最低的分 离 是 壳 质 组, 由 原 料 中 的 3176% 提 高 到 8174%。 表 5 中的低分离效率预示其较差的 提纯。 主要可能是由于在此条件下, 壳质组 与镜质组之间浮选动力学相差较小的原因。
得到的煤显微组分和矿物质的回 收率与颗粒停留时间的函数关系
图 3 伊利诺 N o 16 煤单体显微组分的含 量与煤显微组分粒度间的函数关系
由图 3 可知, 当壳质组、 镜质组和惰质 组的分离度达 80% 时, 对应的粒度为 4 Λm、 14 Λm 和 22 Λm。根据这些发现, 在进行浮选 柱实验之前, 煤样应磨至平均粒度为 4 Λm , 以确保 3 种显微组分的有效分离。 412 浮选柱实验
出 3 种煤显微组分和矿物质的回收率与停留 时间的曲线。 当颗粒在柱内的停留时间超过 100 s 时, 得到的浮选性递减顺序为: 壳质组、 镜质组、 惰质组、 矿物质。 实验中还有一个 有趣的发现, 颗粒停留时间小于 100 s 时, 惰 质组与其他煤显微组分相比, 其回收率较低。
图 4 (a) 在 pH = 8 时, 由微泡浮选柱实验
由于原料中镜质组的含量相当高, 所以 希望在第一柱 (柱A ) 的尾流中主要为镜质 组。 因此, 在 pH 为 11 和停留时间为 148 s 时, 用两级浮选柱法对尾流再处理, 预计分 离效果稍高于 50% , 使镜质组从惰质组中分 离, 见图 5 的柱D 和 E。最后在柱 F 中, 当 pH 为 8、停留时间为 148 s 时, 惰质组从矿 物质中分离出。 由于该过程在亲水性矿物质 和弱憎水性的惰质组颗粒间进行, 因此, 加 了一个收集器, 以提高惰质组浮选性。
为了评价煤显微组分分离系统的分离性 能, 进行了一系列的浮选柱实验。流程图中, 料流 1 为显微组分分离系统的原料煤, 料流
·44·
4、7 和 13 为壳质组、镜质组和惰质组产物。 从每一料流中得到的显微组分的量见表 5 和 图 6。 通过该系统可得到每一显微组分的含 量, 而惰质组的提纯是最有效的。 原料中惰 质组的含量为 715% , 提纯后达 5018%。 从 惰质组中分离镜质组效果也非常明显, 镜质 组的含量由第一阶段尾流 ( 料流 8) 中的 6713% 降至惰质组产品流中的 2716%。研究 中有一有趣发现, 尽管使用了充足的洗选水 以消除水力的夹带, 但惰质组产物中仍含大 量 矿物质。 事实上, 在镜质组分离过程中 (柱D 和 E) , 浮选物中也有相当量的矿物质。 这一发现表明在煤的颗粒中含有细散的矿物 质, 特别是在惰质组显微组分中。
表 5 柱浮选法分离煤显微组分系统中 每一料流的显微组分含量 (% )
料流 壳质组 镜质组 惰质组 矿物质
1
3176
2
5140
3
6199
4
8174
5
2100
6
2188
7
2134
8
1118
9
0186
10
1174
11
1193
12
1164
13
3107
14
1147
7915 8713 8813 8617 8511 9211 8718 6713 8714 7816 6519 1414 2716 1218
表 6 煤显微组分离系统得到的单体 显微组分产物的测值和计算值
显微组分 原煤 壳质组产物 镜质组产物 惰质组产物
(w t% ) 测值 计算值 测值 计算值 测值 计算值 测值
镜质组 79151 8715 壳质组 3176 1114 惰质组 7156 0191 矿物质 9117 0120
8617 8174 3111 1141
将煤研磨至显微组分单体分离粒度所增 加的成本是煤精选过程中最主要成本之一。 据介绍, 将煤粒度降至 10 Λm 所需电费约 5 ~ 7 美元 t。 然而, 所需研磨的程度 (或粒 径) 与煤中每种显微组分的单体特性有关。例 如: 在美国西部煤树脂体的最大粒度为 600 Λm , 当回收率为 80% 时, 树脂体可从 7% 提 高到 80%。研磨相同试样, 使其粒度上限为 7 Λm。此过程是电力业对煤的常规操作, 树 脂体的回收率达到 95% , 可获得相同的提纯
表 3 由高温灰分分析结果计算的可燃物回收率得到的 K 值与 用离心沉浮分离数据得到的煤显微组分回收率的比较
显微组分
镜质组 壳质组 惰质组 总 计
原 煤 MM F 含量
(w t% )
8713 4142 8128 100
显微组分 回收率
(w t% )
8414 94188 68113
ቤተ መጻሕፍቲ ባይዱ
显微组分 产 品
由实验得到的分离性能值与由图 4 (a) 和 (b) 由组分回收率预测得到的值相比较。
第 2 期 煤质技术 1999 年 3 月
超细煤
100
(1)
脱镜质组!
2417 (9)
D!
脱镜质组! 419 (11)
E!
1412 (10)
惰质组产物! 110 (13)
为进一步解释 pH 值对煤显微组分浮选 工艺的影响, 用 L even sp iel’s 方程计算 3 种 煤显微组分的浮选动态速率 (K) , 以确定柱 中混合程度和在轴向分散系中组分的回收率。
用煤显微组分回收率的实验数据, 计算 每一煤显微组分的 K 值。实验中, 颗粒停留 时间 (T P) 为 2146 m in, 收集区内的气流为 15%。 见表 2, 在 pH 为 11 时, 计算出岩相 组分的浮选动态速率, 比相应的 pH 值为 8 时低。
F!
913 (12)
#A
3819 (8)
6111
(2)
∀ 4117
B (3)
1914
∀
(5)
C
1215 (6)
2912 # 壳质组产物 (4)
3119 (7) #
镜质组产物
矿物质!
813 (14) 图 6 煤显微组分分离系统中从每一料流得到的物料收率
用M eloy 研究的系统分析技术以及组分的 燃料相同, 约 110 美元 t。单体显微组分的纯
第 2 期 煤质技术 1999 年 3 月
利用浮选柱对煤显微组分的分离
[ 美国 ] 南伊利诺大学 霍雷克 莫汉蒂等
(续上期)
4 结果与讨论
411 单体煤显微组分的研究 在研究煤显微组分含量之前, 先对伊利