Phylogenetic diversity of endophyte assemblages
DNA barcoding Phylogenetic diversity DNA条形码技术 谱系多样性

Outline of phyloGenerator workflow
Stages of the program are coloured yellow, programs used are blue, inputs are white and outputs are white with two lines around them. Optional steps have dashed lines.
SAPD of other plots
Rare species in Yasuni have significant higher cumulative PD than expected; supporting niche differentiation hypothesis. In BCI and Dinghushan,the contribution of rare species to community PD is not significantly different from random;supporting environmental filtering hypothesis
• Compare with DHS plot,the contribution of rare
species to community PD is more obviously.
• Different species have different contribution to the community PD. The species that are rare and contribute more to the community PD should be priority protection.
芸芥(erucasativamill)发状根愈伤组织诱导及发状根次生代谢物分析
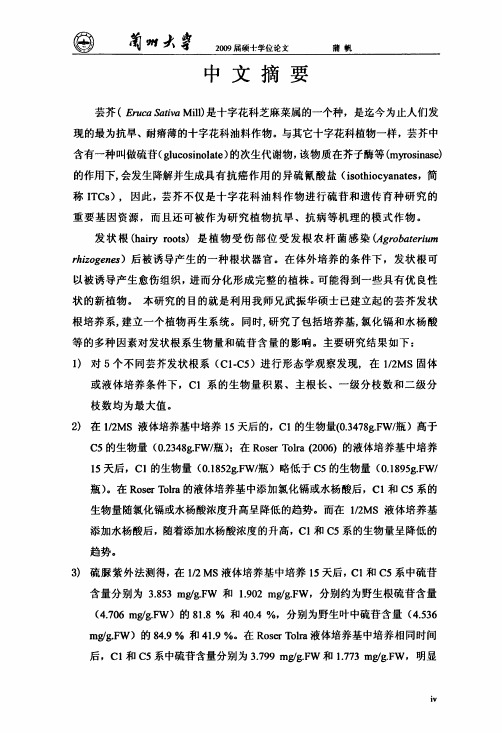
◎
萄研大害
2009届硕士学位论文
蒲帆
地低于在l/2 MS液体培养基中的硫苷含量。在添加了CdCl2(50、100岬) 的RoserT0lra液体培养基中培养15天后,Cl系中的硫苷含量(4.177m眺. FW和6.85lm∥g.FW)均高于C5系中的硫苷含量(2.812 m眺.FW和4.26l mg/g.FW),且当CdCl2浓度为100岬时,C1中的硫苷含量为最高(6.851m∥g.
Cl(0.18529.FV堋ask)cmtul司in the Roser Tolra liquid media after 1 5d was slightly lower
than which of C5(0.1 8959.F、v/n硒k).Cultured in the Roser Tolra liquid media with CdCl2 or鼢licylic孔id after l 5d,the biomass accumulation of C l and C5 decreased a8 the concentration of the CdCl2 or鼢licylic acid increased.Cultured in l/2 MS liquid media with 鞠】icylic acid after l 5d, the biomass accumulation of C 1 and C5 decrcased as the concentration of the salicylic acid increased.
2)The biomass accumulation of C l(0.34789.FⅣ/nask)cultIlred in l/2 MS solid/liquid
media anerl 5d w雒higllcr tll锄which of C5(o.23489.FW/flaSk).ne biomass accumulation of
分子进化树构建方法
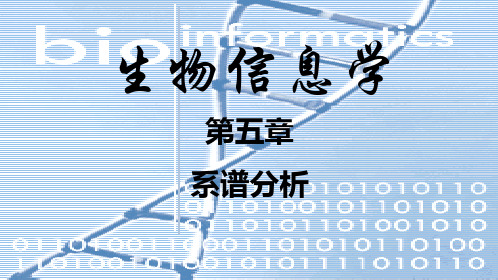
MP法建树流程
Sequence1 Sequence2 Sequence3
Sequence4
Position 1
Position 1 2 3 T G C T A C A G G A A G
If 1 and 2 are grouped a total of four changes are needed.
5
genetic change
系统发生树术语
Rooted tree vs. Unrooted tree
无 A 有 根 根 树 B 树 two major ways to root trees:
A
10 3 2 5
C D
By midpoint or distance
d (A,D) = 10 + 3 + 5 = 18 Midpoint = 18 / 2 = 9
Distance Uses only pairwise distances Minimizes distance between nearest neighbors Very fast Easily trapped in local optima Good for generating tentative tree, or choosing among multiple trees Maximum parsimony Uses only shared derived characters Minimizes total distance Maximum likelihood Uses all data Maximizes tree likelihood given specific parameter values Very slow Highly dependent on assumed evolution model Good for very small data sets and for testing trees built using other methods
叶绿体系统发育基因组学的研究进展
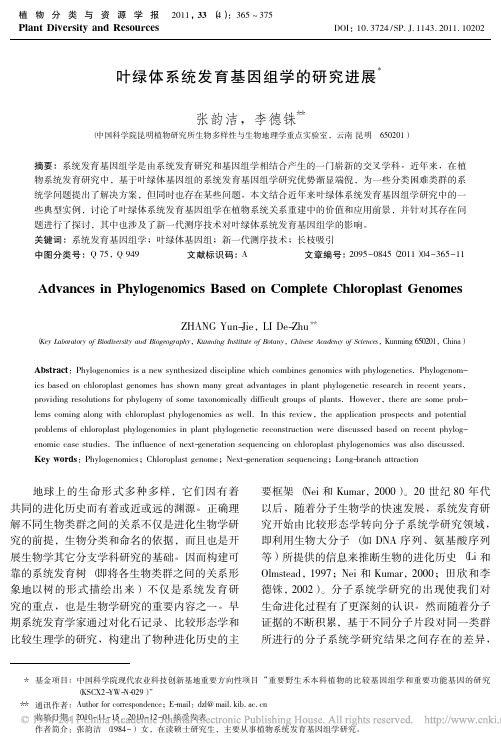
叶绿体系统发育基因组学的研究进展*张韵洁,李德铢**(中国科学院昆明植物研究所生物多样性与生物地理学重点实验室,云南昆明650201)摘要:系统发育基因组学是由系统发育研究和基因组学相结合产生的一门崭新的交叉学科。
近年来,在植物系统发育研究中,基于叶绿体基因组的系统发育基因组学研究优势渐显端倪,为一些分类困难类群的系统学问题提出了解决方案,但同时也存在某些问题。
本文结合近年来叶绿体系统发育基因组学研究中的一些典型实例,讨论了叶绿体系统发育基因组学在植物系统关系重建中的价值和应用前景,并针对其存在问题进行了探讨,其中也涉及了新一代测序技术对叶绿体系统发育基因组学的影响。
关键词:系统发育基因组学;叶绿体基因组;新一代测序技术;长枝吸引中图分类号:Q75,Q949文献标识码:A文章编号:2095-0845(2011)04-365-11 Advances in Phylogenomics Based on Complete Chloroplast GenomesZHANG Yun-Jie,LI De-Zhu**(Key Laboratory of Biodiversity and Biogeography,Kunming Institute of Botany,Chinese Academy of Sciences,Kunming650201,China)Abstract:Phylogenomics is a new synthesized discipline which combines genomics with phylogenetics.Phylogenom-ics based on chloroplast genomes has shown many great advantages in plant phylogenetic research in recent years,providing resolutions for phylogeny of some taxonomically difficult groups of plants.However,there are some prob-lems coming along with chloroplast phylogenomics as well.In this review,the application prospects and potential problems of chloroplast phylogenomics in plant phylogenetic reconstruction were discussed based on recent phylog-enomic case studies.The influence of next-generation sequencing on chloroplast phylogenomics was also discussed.Key words:Phylogenomics;Chloroplast genome;Next-generation sequencing;Long-branch attraction地球上的生命形式多种多样,它们因有着共同的进化历史而有着或近或远的渊源。
蝴蝶的生命周期 英语作文
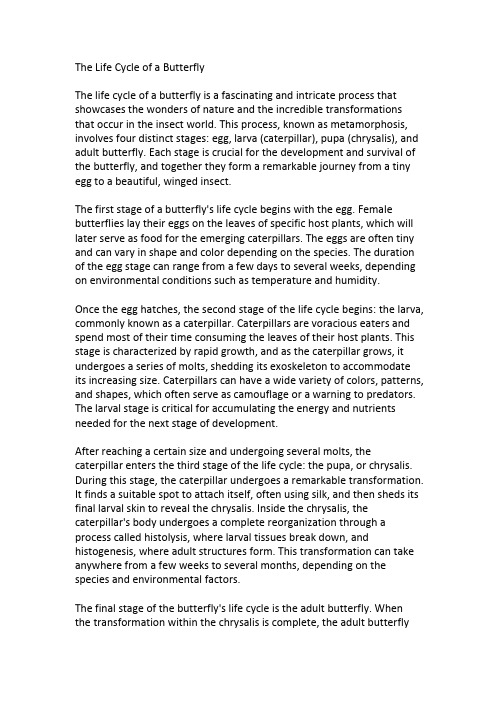
The Life Cycle of a ButterflyThe life cycle of a butterfly is a fascinating and intricate process that showcases the wonders of nature and the incredible transformations that occur in the insect world.This process,known as metamorphosis, involves four distinct stages:egg,larva(caterpillar),pupa(chrysalis),and adult butterfly.Each stage is crucial for the development and survival of the butterfly,and together they form a remarkable journey from a tiny egg to a beautiful,winged insect.The first stage of a butterfly's life cycle begins with the egg.Female butterflies lay their eggs on the leaves of specific host plants,which will later serve as food for the emerging caterpillars.The eggs are often tiny and can vary in shape and color depending on the species.The duration of the egg stage can range from a few days to several weeks,depending on environmental conditions such as temperature and humidity.Once the egg hatches,the second stage of the life cycle begins:the larva, commonly known as a caterpillar.Caterpillars are voracious eaters and spend most of their time consuming the leaves of their host plants.This stage is characterized by rapid growth,and as the caterpillar grows,it undergoes a series of molts,shedding its exoskeleton to accommodate its increasing size.Caterpillars can have a wide variety of colors,patterns, and shapes,which often serve as camouflage or a warning to predators. The larval stage is critical for accumulating the energy and nutrients needed for the next stage of development.After reaching a certain size and undergoing several molts,the caterpillar enters the third stage of the life cycle:the pupa,or chrysalis. During this stage,the caterpillar undergoes a remarkable transformation. It finds a suitable spot to attach itself,often using silk,and then sheds its final larval skin to reveal the chrysalis.Inside the chrysalis,the caterpillar's body undergoes a complete reorganization through a process called histolysis,where larval tissues break down,and histogenesis,where adult structures form.This transformation can take anywhere from a few weeks to several months,depending on the species and environmental factors.The final stage of the butterfly's life cycle is the adult butterfly.When the transformation within the chrysalis is complete,the adult butterflyemerges.This process,known as eclosion,involves the butterfly breaking free from the chrysalis and expanding its wings.Initially,the wings are soft and crumpled,but within a few hours,they harden and become strong enough for flight.The adult butterfly's primary focus is on reproduction and feeding.Butterflies are known for their striking colors and patterns,which serve various purposes,including attracting mates,camouflage,and warning predators.Adult butterflies feed on nectar from flowers,using their long proboscis to reach deep into the blossoms.They play a vital role in pollination, transferring pollen from one flower to another as they feed.This mutualistic relationship benefits both the butterflies and the plants, contributing to the health and diversity of ecosystems.In conclusion,the life cycle of a butterfly is a remarkable journey that highlights the beauty and complexity of nature.From the tiny egg to the vibrant adult butterfly,each stage of metamorphosis is a testament to the adaptability and resilience of these incredible insects.Understanding and appreciating the life cycle of butterflies not only deepens our connection to the natural world but also underscores the importance of conserving their habitats and ensuring the survival of these delicate and enchanting creatures for future generations to enjoy.。
Molecular Phylogenetic Analyses and Real Life Data

a rX i v:q-bi o/513v2[q-b io.G N]29J a n25Molecular Phylogenetic Analyses and Real Life Data Kerstin Hoef-Emden February 9,2008Universit¨a t zu K¨o ln,Botanisches Institut,Lehrstuhl I,Gyrhofstr.15,50931K¨o ln,Germany e-mail:kerstin.hoef-emden@uni-koeln.de 1What is Molecular Phylogeny?Most probably,all life existing today on earth shares a common ancestry billions of years back in the past.A set of indispensable genes necessary for maintenance of basic cell functions were passed on from the unknown common ancestor to its extant descendants by asexual and/or sexual reproduction.During the course of evolution,the genes,the numbers of genes,their functions and the sizes of the genomes (i.e.the total DNA content of a cell)became modified.If genes originate from a common ancestor gene and fulfill the same function in a cell,they are said to be homologous.The degree of divergence between homologous genes is considered a measure for their relatedness (and also for the relatedness of the organisms).In molecular phylogeny,the relationships among,usually extant,organisms are examined by comparing homologous DNA or protein sequences (i.e.the gene products).The relationships are displayed as trees with branch (or edge)lengths reflecting the degrees of genetic divergence.Each branch tip represents an extant sequence;the internal nodes or vertices represent unknown ancestors to the terminal nodes.The branching pattern and branch lengths describe the evolutionary pathways leading to the sequences at the terminal nodes.Clusters of terminal branches connected to a common ancestor are termed clades.The construction of phylogenetic trees has been shown to be a NP-hard problem;the number of possible trees increases exponentially with the numberof DNA or protein sequences included in the phylogenetic analyses [1].Due to the large amount of data and the complexity of the task,phylogenetic trees cannot be inferred without help of computers.Numerous studies addressing the problems of molecular phylogenetic analy-ses methods in theory or practice have been published.First publications about phylogenetic methods date back into the 60s.The methods and evolutionary models were refined in the course of time,but problems still remain.The cited references in this review represent only few examples from a vast amount of1literature.Also only some of the mostly used methods in molecular phylogeny are presented.For digging into the mathematics behind the phylogenetic analyses methods introduced below,one may start with Joe Felsenstein’s book [2].2Phylogenetic Analyses MethodsDNA sequences are based on a four-letter-code representing the four nucleotides (A for adenin,C for cytosin,G for guanin,T for thymin),whereas protein sequences are based on a twenty-letter-code representing the twenty different amino acids.Prior to the phylogenetic analyses,an alignment of the sequences has to be assembled (the single sequence is also termed a “taxon”,because it represents a species,genus,individual or strain).If sequences of homologous genes e.g.show differences in lengths due to insertions or deletions,gaps have to be inserted to place functionally corresponding positions in the same vertical column of the alignment (Fig.1).Non-alignable regions such as insertions ofFig.1:Excerpt from an alignment of nuclear ITS2sequences.The ITS2or internal transcribed spacer 2expands between two RNA coding genes of the ribosomal operon.The ribosomal operon is transcribed in one piece.The two internal transcribed spac-ers between the RNA coding regions fold up in a specific way and are excised.Since the two ITS regions solely function as spacers,they are under low selective pressure and,thus,display high mutation rates.The example alignment shows ITS2regions of closely related organisms belonging to one genus.The sequences are oriented in hori-zontal direction,whereas functionally corresponding positions are arranged in columns.Several gaps had to be inserted due to insertions of nucleotides in the sequences 1and 5.several nucleotides need to be excluded from the phylogenetic analyses.Improp-erly aligned sequences or inclusions of non-alignable regions in the phylogenetic analyses may result in artefactual phylogenetic trees.In most standard methods for inferring phylogenetic trees,an optimality criterion and a tree search algorithm have to be chosen.The optimality criterion is used to determine the best among the considered trees by defining a type of “scoring”system.Optimality criteria are e.g.maximum parsimony,distance matrix or maximum likelihood [2].In unweighted maximum parsimony,each mutation from one nucleotide or amino acid to another,e.g.from a C to a G,costs one “penalty”point.All point mutations are considered equally likely.The mutations along a given tree are summed up and the best tree or maximum parsimony tree is the one with2the lowest sum of penalty points.Unweighted maximum parsimony uses integer values and often several to many equally parsimonious trees are found.In distance analyses,the sequences are pair-wise compared.Their genetic divergences are transformed into distance values and listed in a triangular dis-tance matrix.Whereas maximum parsimony treats all mutations as equally likely,the computation of distance matrices allows for different mutation rates and other variations of parameters(i.e.evolutionary models,see chapter below). To infer trees from a distance matrix,usually the neighbor-joining algorithm is used(see below).Maximum likelihood is a probablistic and the computationally most costly method(Fig.2).It searches for the tree that optimizes the probability of observ-ing the data.The likelihood of a tree is expressed as negative natural logarithm. The maximum likelihood method also allows for different evolutionary models, but differs from distance matrix methods in that it uses discrete characters and may result in more than one optimal tree(however,rarely more than two).The numbers of sequences used to infer phylogenetic trees in biological re-search projects almost always prohibited exhaustive searches of the complete tree space due to limitations of computation time.Thus,maximum parsimony or maximum likelihood were usually combined with heuristic tree search al-gorithms.For a heuristic search afirst tree is generated e.g.by adding the sequences step-by-step to the growing tree.Thisfirst tree is then subjected to local and/or global rearrangements by swapping internal branches or cutting the tree into pieces and rejoining the parts in different places.This procedure is supposed to overcome potential local optima and tofind the global optimum. The construction of a tree by neighbor-joining,the preferred method used with distance matrices,starts with a star-like tree.The pair of sequences with the lowest genetic divergence is joined(i.e.they are said to be neighbors)and the distance matrix recalculated.These steps are repeated with the next closest related sequences or clusters of sequences until the tree is completely resolved.In Bayesian analyses,posterior probabilities for trees and evolutionary pa-rameters are calculated using the Bayes theorem[3].With the Bayes formula the posterior probability of a tree given the data is calculated using prior probabil-ities of the data and the tree,and the likelihood of a tree.Since it is impossible to calculate all trees and evolutionary parameters from the space of the joint posterior probability distribution,samples are drawn using Metropolis-coupled Markov chain Monte Carlo simulations.This means,at start of a Bayesian anal-ysis,several chains are initialized to search for the global optimum in the space of the joint posterior probability distribution.Once initialized,the chains cross the space for several hundredthousands to millions of generations by slightly modifying the parameters(tree topology,branch lengths,evolutionary model parameters).Trees and evolutionary model parameters are sampled only from the cold chain;the other so-called heated chains traverse the space more easily and exchange their status data from time to time with the cold chain.By doing so,the heated chains help the cold chain to reach the global optimum,which comprises a set of the best trees and evolutionary parameters.The presumed global optimum is found when the likelihoods of the trees sampled from the cold chain reach stationarity.The phylogenetic trees inferred by the above mentioned methods are usu-ally bifurcating trees.They may be rooted or unrooted.In rooted trees,the closest related sistergroup is used to define the direction of evolution in the3Fig.2:Computation of the likelihood of a tree.To obtain the overall likelihood value of a tree,for each position of the alignment the probabilities of all possible combinations of ancestral character states are computed.The site-wise likelihood comprises the sum of all probabilities.The site-wise log likelihoods are then multiplied and result in the log likelihood of a given tree.4sequences.To e.g.examine the relationships among chimpanzee,gorilla and man,the orangutan would be the appropriate outgroup.Unrooted trees are like looking onto the treetop from above without knowing where the stem is.In unrooted trees it is not possible to tell,where evolution started and in which direction the sequences evolve.3Models of Molecular EvolutionIn addition to exponentially growing numbers of possible trees,phylogenetic analyses are further complicated by the fact that substitution rates of nu-cleotides or amino acids may vary.Evolutionary models are an attempt to approximate the complexity of molecular evolution as close as possible.The proportions of the four nucleotides in a DNA sequence may differ from gene to gene and,thus,need to be considered in phylogenetic analyses(base fre-quencies).To account for differing substitution rates for the six types of point mutations,a substitution rate matrix is used(Fig.3A).However,depending in the positions in the alignment,these rates may be higher or lower.Some positions are highly conserved and do not change at all.Others evolve at dif-fering rates(Fig.3B).Both parameters,the proportion of invariable sites and site-specific rate variation,modelled as a gamma-distribution(Fig.3C),belong to the among-site substitution rate variation and can be explained by functional constraints on the gene products.For most data sets used in biological studies,it is impossible to infer phy-logenetic trees in a reasonable time by optimizing all likelihood parameters at once during a maximum likelihood analysis,i.e.tree topology,branch lengths of the trees,base frequencies,substitution rate matrix,proportion of invari-able sites and continuously gamma-distributed among-site rate variation.An often practised approach consisted of determiningfirst the parameters of the evolutionary modelfitting best the data[4].Tofind the appropriate evolution-ary model,a tree is inferred with a fast method(usually distance matrix with neighbor-joining)and the likelihood values for this tree are calculated for each available evolutionary model.The modelfitting best the data is then chosen by e.g.hierarchical likelihood ratio tests(hLRT)or by the Akaike informa-tion criterion(AIC).Also,a discrete instead of a continuous gamma-distributed among-site rate variation is used to reduce computation times(Fig.3C).Thus, during heuristic tree search only tree topology and branch lengths need to be optimized,whereas the evolutionary model parameters have been already es-timated from the data set using an approximate tree topology prior to the heuristic tree search.An additional evolutionary parameter,the covarion/covariotide model takes lineage-specific evolutionary rates into consideration,plete sequences may evolve faster than others.The covarion/covariotide model,however,until today was only implemented in Bayesian phylogenetic analyses programmes.Protein coding DNA sequences are in vivofirst transcribed into messenger RNA,then translated into a protein consisting of a string of amino acids(Fig. 3B).The function of the protein is determined by folding up into tertiary and quarternary structures and by amino acids with specific chemical properties in specific positions.Maximum likelihood analyses of DNA sequences are quite time intensive.Maximum likelihood analyses with20character states for the5Fig.3:Substitution rate matrices and among-site rate variation.Fig.3A.Examples for substitution rate matrices.To the left,the most complex type implemented in phylogeny software programmes,the general time reversible model(GTR)with six different substitution rates.To the right,a modified GTR model,the Tamura-Nei model with three different mutation rates.Fig.3A.Among-site rate variation in RNA and protein coding DNA.Sites with high mutation rates are usually found in loop regions of RNA secondary structure,whereas helices are more conserved(left).In protein coding DNA,the third position of the codons is usually the most variable. The degenerate code allows for several codons to represent the same amino acid. In this example,codons for the amino acids serine,arginine and valine are shown. Between DNA and protein,a transcription step to messenger RNA is necessary.Bold face,positions with higher mutation rates.Fig.3C.Modelling the among-site rate variation using a gamma distribution.Examples for continuous gamma distribution with different shape parameters to the left and a discrete gamma distribution with seven rate categories to the right.The discrete gamma distribution approximates a continuous gamma distribution with a shape parameterαof1.6amino acids are even more time-consuming.Thus,in protein phylogenies,sub-stitution rate matrices were usually not computed from the data sets,instead pre-defined sustitution rate matrices empirically derived from large alignments of other proteins were used[2].Phylogenetic trees can also be inferred from the DNA sequences of protein coding genes,which however offers some pitfalls.In protein coding genes,three nucleotides code for one amino acid,but the genetic code is degenerate.This means that several three-nucleotide combinations may code for the same amino acid(e.g.six codons are known to code for arginine,leucine or serine;see Fig. 3B).As a consequence,a nucleotide change in one codon position may be either without effect on the amino acid(=silent or synonymous substitution),or cause a change of one amino acid to another(=nonsynonymous substitution). Only nonsynonymous substitutions can result in a loss or decrease of function, and,thus are subject to functional constraints.However,the sophisticated evolutionary model parameters mentioned above were infirst place developed to cope with RNA coding genes.The three-nucleotide codon structure is ignored and synonymous and nonsynonymous mutations are treated equally.Also,often several evolutionary pathways are possible to evolve from one codon to another, which further complicates the evolutionary model parameters.Often the third positions of codons show nucleotide biases towards higher GC or AT contents.However,from theoretical and simulation studies,but also empirically,it be-came obvious that using wrong assumptions about the underlying evolutionary processes may result in biased phylogenetic trees.4Simulation StudiesThe accuracy of a method comprises consistency,efficiency and robustness.A method is consistent,if it infers the correct phylogenetic tree with an infinite amount of data.Efficiency describes the sensitivity of a method concerning the lengths of sequences.The shorter the sequences can be for a method to con-verge to the correct tree topology,the more efficient is the method.Robustness considers using wrong assumptions about the underlying evolutionary model.A method is robust,if it infers the correct phylogenetic tree although a wrong evolutionary model was used.Since biologists use DNA or protein sequences of finite lengths,in practice only consistency and robustness of a method are of interest.In a simulation study by Huelsenbeck[5],e.g.four-taxon data sets of differ-ing sequence lengths were generated in silico from a random starting sequence according to pre-specified evolutionary models and phylogenetic trees(see pa-rameter space in Fig.4A).Different phylogenetic analyses methods were then used to infer trees from the data sets and the conditions determined that caused the methods to infer wrong tree topologies.The so-called long branch attraction artefact(LBA)is the most well-known phenomenon causing biased tree ually,LBAs were found in phylogenetic trees with extremely long termi-nal(i.e.branches with high evolutionary rates)but short internal branches(Fig. 4B).In most test situations,maximum likelihood outperformed other methods, but it also failed infinding the correct tree,if the assumed evolutionary models were too different from the evolutionary processes under which the simulated data sets had evolved.75Phylogenetic Analyses and Real Life Data Since divergent branch lengths were almost always found in phylogenetic anal-yses of in vivo evolved sequences,the effects of potential LBAs were a frequent matter of concern[6].Especially in large scale phylogenies comprising sequences of very different organisms,long-branch taxa were often gathered ladder-like close to the root of the trees,which may indicate a potential bias caused by LBAs.The farther back in time the examined relationships of organisms reach, the worse the resolution at the internal branches of a tree.It was found that an addition of sequences to the data set and a complex evolutionary model with a gamma-distributed among-site rate variation were the best options to reduce artefacts in a phylogenetic tree[7],[8].Especially,adding more sequences of the problematic type could break up long branches,increase the resolution in this part of a tree and thereby neutralise the LBA.An example of how taxon sampling and choice of evolutionary model may affect the results of a molecular phylogeny can be found in the cryptophytes,a group consisting of microscopicflagellated unicells.Most of the genera in this group are algae,i.e.they contain a pigmented plastid which is used to turn the energy of light into chemical energy by photosynthesis.Two genera are,however, colourless.Goniomonas is phagotrophic;it feeds from ingesting bacteria.The other genus,formerly classified as Chilomonas feeds from organic molecules,but still harbours a leukoplast,i.e.a colourless plastid.In a phylogenetic analysis with a low number of nuclear18S ribosomal DNA sequences,Goniomonas and “Chilomonas”clustered together indicating a relationship of both genera[9]. In a later analysis,sequences of the photosynthetic genus Cryptomonas were added[10].It turned out that Goniomonas was the most basally diverging taxon,whereas“Chilomonas”was a colourless Cryptomonas.The clade with the genera Cryptomonas and“Chilomonas”seemed to be the most basal group of the plastid-bearing cryptophytes.Thus,the sisterhood of Goniomonas and “Chilomonas”were caused by a LBA due to inappropriate taxon sampling.The analysis in[10],however,was done using maximum likelihood under a simple evolutionary model,i.e.without considering an among-site rate variation.In a study using a complex evolutionary model with among-site rate variation,the basal position of the Cryptomonas/“Chilomonas”clade was also shown to be an artefact caused by long branch attraction[11].Thus,long branch attraction artefacts are a real problem in phylogenies inferred from in vivo evolved sequences.The best options to cope with LBAs,i.e. adding more taxa,and using complex evolutionary models and robust methods, however,collide with another problem biologists were and are still confronted with computation times.The larger the amount of sequences,the more reliable the phylogenetic analyses methods do work,but exponentially more time is also needed to obtain results.Bayesian analysis was introduced as a potential faster alternative to maxi-mum likelihood analysis[3].However,for large data sets Markov chains often need to be run for more generations to reach a plateau of likelihood values, which also increases comutation times.In addition,the posterior probabilities given for the different branches of the consensus tree,in which the sampled trees are summarised,are more optimistic than support values obtained from nonparametric bootstrapping using the maximum likelihood criterion(i.e.a sub-sampling method with at least100,often more than100subsample data sets,to9test the stability of the branches of a tree).Bayesian analysis may be speeded up by running the different Markov chains on separate CPUs of a computing server or a cluster.In heuristic tree searches using the maximum likelihood criterion,some par-allelised versions of programmes have been introduced e.g.[12].The tasks of tree generation and tree evaluation were distributed among a master(tree gen-eration and comparison)and worker programmes(calculation of branch lengths and likelihoods).Another attempt to decrease computation times was quartet-puzzling[13]. In quartet-puzzling,trees are computed from quartets of n sequences of a larger data set using the maximum likelihood criterion and weighted accordingly.The best of the three possible4-trees for each quartet are used tofirst assemble a large number of n-trees(quartet-puzzling)andfinally to obtain a consensus n-tree.This method is much faster than a heuristic trees search,but more vulnerable to LBA.Among hundreds to thousands computed four-taxon trees, only a low number of biased4-trees suffices to pass on a topological error to the final n-tree.In simulation studies,global character maximum likelihood almost always outperformed quartet-puzzling or related methods[14].Other studies tried to overcome LBA and exponentially growing computing times with longer sequences,e.g.by using complete genomes to infer phyloge-netic trees.Phylogenetic analyses of longer sequences increase the computing times only linearly.Since sequencing of complete genomes need much more time and resources than that of single genes or smaller sets of genes,the taxon sam-pling in these studies generally was lower.It has been shown,however,that long sequences cannot compensate for an extended taxon sampling.The low number of taxa included in a genome-scale analysis resulted in high bootstrap support even for biased tree topologies[15].Also genome-scale alignments cannot be re-fined by eye anymore.They depend in automatic alignment algorithms,which may perform badly by producing more or less biologically meaningless align-ments[16].A better option than using complete genomes presumably is to sequence a set a of genes,to refine the alignment of each gene by eye,and to concatenate the genes[17].Additional problems occur,if the evolution of a gene and/or a group of organisms cannot be described by bifurcating trees.In sexually reproducing populations,the examined gene may be present in differing alleles.Each indi-vidual of a population inherits two alleles,one from its mother,the other from its father.In addition,parts of the alleles can be exchanged by genetic recombi-nation.Genetic material may also be transferred between unrelated organisms, e.g.by infection with viruses,by endosymbiosis or in bacteria by exchange of plasmids.Whereas the inheritance of genes from parents to child is called verti-cal gene transfer,the exchange of genetic material between unrelated organisms is called lateral gene transfer.The results of sexual reproduction or lateral gene transfers are genetic chimaeras and reticulate evolutionary trees.6ConclusionsUntil yet,there seems to be no easy way out of the treadmill of extremely increasing computing times for phylogeneticists.New algorithms to reduce time consumption in phylogenetic analysis have been proposed until recently,e.g.[18].10However,only if the algorithms are offered in software programmes suitable for the tasks of phylogenetic analysis,if they are presented in an understandable way to biologists and if they prove to be robust,they will accepted and used. References[1]Steel M(1992)The complexity of reconstructing trees from qualitativecharacters and subtrees.J.Classif.9(1):91–116[2]Felsenstein J(2003)Inferring phylogenies.Sinauer Associates,Publishers,Sunderland[3]Huelsenbeck JP,Ronquist F,Nielsen R,Bollback JP(2001)Bayesian in-ference of phylogeny and its impact on evolutionary biology.Science294 (5550):2310–2314[4]Posada D,Crandall KA(1998)Modeltest:testing the model of DNA sub-stitution.Bioinformatics14(9):817–818.[5]Huelsenbeck JP(1995)Performance of phylogenetic methods in simulation.Syst.Biol.44(2):17–48[6]Anderson FE,Swofford DL(2004)Should we be worried about long-branchatrraction in real data sets?Investigations using metazoan18S rDNA.Mol.Phylogenet.Evol.33(2):440–451[7]Graybeal A(1998)Is it better to add taxa or characters to a difficultphylogenetic problem?Syst.Biol.49(1):9–17[8]Bruno WJ,Halpern AL(1999)Topological bias and inconsistency of max-imum likelihood using wrong models.Mol.Biol.Evol.16(4):564–566 [9]Cavalier-Smith T,Couch JA,Thorsteinsen KE,Gilson P,Deane JA,HillDRA,McFadden GI(1996)Cryptomonad nuclear and nucleomorph SSU rRNA phylogeny.Eur.J.Phycol.31(4):315–328[10]Marin B,Klingberg M,Melkonian M(1998)Phylogenetic relationshipsamong the Cryptophyta:analyses of nuclear-encoded SSU rRNA sequences support the monophyly of extant plastid-containing lineages.Protist149(3):265–276[11]Hoef-Emden K,Marin B,Melkonian M(2002)Nuclear and nucleomorphSSU rDNA phylogeny in the Cryptophyta and the evolution of cryptophyte diversity.J.Mol.Evol.55(2):161–179[12]Stewart CA,Hart D,Berry DK,Olsen GJ,Wernert EA,Fischer W(2001)Parallel implementation and performance of fastDNAml–a program for maximum likelihood phylogenetic inference.Proc.SC2001,Denver,CO, November2001[13]Strimmer K,von Haeseler A(1996)Quartet puzzling:a quartet maximum-likelihood method for reconstructing tree topologies.Mol.Biol.Evol.13(7): 964–96911[14]Ranwez V,Gascuel O(2001)Quartet-based phylogenetic inference:im-provements and limits.Mol.Biol.Evol.18(6):1103–1116[15]Soltis DE,Albert VA,Savolainen V,Hilu K,Qiu Y-L,Chase MW,FarrisJS,Stefanovi´c S,Rice DW,Palmer JD,Soltis PS(2004)Genome-scale data, angiosperm relationships,and‘ending incongruence’:a cautionary tale in phylogenetics.Trends Plant Sci.9(10):477–483[16]Pollard DA,Bergman CM,Stoye J,Celniker SE,1Eisen MB(2004)Bench-marking tools for the alignment of functional noncoding DNA.BMC Bioin-formatics5:6[17]Bapteste E,Brinkmann H,Lee JA,Moore DV,Sensen CW,Gordon P,Durufl´e L,Gaasterland T,Lopez P,M¨u ller M,Philippe H(2002)The anal-ysis of100genes supports the grouping of three highly divergent amoebae: Dictyostelium,Entamoeba,and A 99(3):1414–1419[18]Guindon S,Gascuel O(2003)A simple,fast,and accurate algorithm toestimate large phylogenies by maximum likelihood.Syst.Biol.52(5):696–70412。
植物LEAFY 同源基因的研究进展

植物学通报 2005, 22 (5): 605 ̄613①通讯作者。
Author for correspondence. E-mail: silandai@收稿日期: 2004-11-18 接受日期: 2005-03-24 责任编辑: 白羽红植物LEAFY 同源基因的研究进展1马月萍 2陈 凡 1戴思兰①1(北京林业大学园林学院 北京 100083) 2(中国科学院遗传与发育生物学研究所 北京 100101)摘要 本文就近10年来LEAFY (简写为LFY )同源基因的研究进展做了综合分析。
通过对19种植物中已分离到的LFY 同源基因的序列比较分析发现: LFY 同源基因编码区核苷酸和氨基酸序列同源性都较高;在双子叶植物基因组中, 拷贝数却有所不同。
该基因的表达特性显示其在不同植物中表达的时间和空间有所差异。
根据已知序列推导的氨基酸序列构建的系统进化树表明, 单子叶植物与裸子植物的亲缘关系近于双子叶与裸子植物的亲缘关系。
上述研究资料为植物成花机理研究提供了重要参考, 且在研究植物系统进化方面也具有重要的意义。
关键词 高等植物, 花发育, LFY 同源基因Studies of LEAFY Homologue Genes in Higher Plants1MA Yue-Ping 2CHEN Fan 1DAI Si-Lan ①1(College of Landscape Architecture, Beijing Forestry University , Beijing 100083 )2(Institute of Genetics and Development Biology, the Chinese Academy of Sciences , Beijing 100101)Abstract We review and summarize the progress of studies of LEAFY genes. Sequence com-parative analysis of the orthologs of genes isolated from a wide variety of plant species showed high similarity in coding sequence at the nucleotide and amino acid levels but different copy numbers, especially in diploid angiosperms. Orthologous genes, however, have variant ex-pression patterns in different plants. Phylogenetic analysis of the predicted protein sequences of the orthologs supported the currently accepted theory of phylogenetic partnerships of plant species. The information summarized here is helpful not only for better understanding the mechanisms of plant flowering but also for further promoting studies of the evolution of flowering plants.Key words Flowering plants, Flower development, LEAFY homologue gene高等植物的成花是一个非常重要的过程,它不仅关系着物种的延续, 且与人类生活息息相关。
水处理微生物学—细菌的遗传(中英文对照

局限性转导( restricted transduction)
Section E Bacterial genetics 细菌的遗传
E10 Transformation 转化
1.Overview(概述)
1.1 转化(transformation):细菌能够从它们的周围环境中自然 吸收DNA,并将该片断掺入染色体基因组的现象称为转化。 1.2 20世纪20年代末期Fred Griffith发现这样一个现象: 1.3 在环境中基因自然转移,转化作用大概是一个重要的机制。
2.A typical phage life cycle(典型噬菌体的生活周期)
2.4 噬菌体装配(phage assembly):一旦噬菌体的衣壳成分和核酸 被完全合成,新的噬菌体便自发组装。 2.5 释放(release):噬菌体通过裂解细胞壁而释放,完成噬菌体 的一个生活周期,具有这种周期的噬菌体称为烈性噬菌体。噬菌 体用酶软化细胞壁,裂解细胞后释放出大约50~100个噬菌体;也 有的线性噬菌体穿过细胞壁后释放,并不损伤细胞。
Carbon cycle
图
Exercise
1. State the Carbon cycle in the earth. 2. State the Nitrogen cycle in the earth. 3. State the Sulfur cycle in the earth.
Section E Bacterial genetics 细菌的遗传
E9 Transduction 转导
1.Transduction(转导)
1.1 转导(transduction):通过噬菌体颗粒的媒介,把一个细胞 的DNA转移到另一个细胞的机制称为转导. 1.2 转导现象在细菌和噬菌体中被广泛发现,在自然界遗传信息 的转移中起着显著的作用。转导在遗传学上也是一个重要的工具, 它可以用于细菌之间转移基因,也可以通过它绘制细菌染色体的 遗传图谱。
- 1、下载文档前请自行甄别文档内容的完整性,平台不提供额外的编辑、内容补充、找答案等附加服务。
- 2、"仅部分预览"的文档,不可在线预览部分如存在完整性等问题,可反馈申请退款(可完整预览的文档不适用该条件!)。
- 3、如文档侵犯您的权益,请联系客服反馈,我们会尽快为您处理(人工客服工作时间:9:00-18:30)。
Phylogenetic diversity of endophyte assemblages associated with the tropical seagrass Enhalus acoroides in ThailandJ.Sakayaroj &S.Preedanon &O.Supaphon &E.B.G.Jones &S.PhongpaichitReceived:10September 2009/Accepted:29October 2009/Published online:21January 2010#Kevin D.Hyde 2010Abstract Seagrasses are flowering plants inhabiting coast-al and marine environments,with a worldwide distribution.They serve as feeding,breeding and nursery grounds for economically important marine organisms including endan-gered species.The tropical seagrass Enhalus acoroides was collected from Had Khanom-Mu Ko Thale Tai National Park,southern Thailand.The objectives of this study were to investigate for the presence of endophyte assemblages in E.acoroides ,as well as to describe the diversity of endophytes based on LSU,ITS1,2,5.8S rDNA sequence analyses.Forty-two fungal assemblages were isolated and identified through molecular data.This resulted in a diversity of fungal groups of Ascomycota (98%)and Basidiomycota (2%).Three major Ascomycota classes including the Sordariomycetes (36%),Eurotiomycetes (33%)and Dothideomycetes (24%)were determined.The predominant ascomycete orders were the Hypocreales,followed by the Eurotiales and the Capnodiales ,respec-tively.Additionally one taxon belonged to the Russulales ,Basidiomycota and was possibly mycorrhiza.This study confirms that E.acoroides harbors a wide diversity of fungal endophytes,and provides a baseline for further studies on fungal-host plant interactions.Keywords Endophyte .Enhalus .rDNA .SeagrassIntroductionSeagrasses are the only flowering plants or angiosperms inhabiting coastal and marine environments,with a world-wide distribution in temperate and tropical regions (Logan 1992;Phang 2000).The ecological role of seagrasses is very important,as they filter estuarine and coastal waters of nutrients and contaminants and are closely linked to other communities e.g.coral reef and mangrove ecosystems (Wilson 1998;Phang 2000).Little information is available for fungi associated with seagrasses,especially fungal endophytes (Raghu-kumar 2008).Studies on fungi associated with healthy seagrasses have been carried out by Newell and Fell (1980),who initially recorded a rhizomycelial chytrid genus Nowakowskiella in Thalassia testudinum leaves.Subsequently,Kuo (1984)and Kuo et al.(1990)observed reticulated fungal hyphae in the intercellular spaces and cell walls of Zostera muelleri leaves.They suggested that the hyphae are symbiotic in function but not pathogenic.Fungi may obtain solutes from the host plant through the apoplastic transport processes and oxygen from the air lacunae system in host tissues (Kuo 1984).Later,fungal endophytes from more seagrass species:T.testudinum ,Halodule bermudensis and Syringodium filiforme were reported by Wilson (1998).This study demonstrated the presence of 11endophytic fungi including one bacterium.There was a statistically significant difference between fungal endophyte assemblages of different seagrass species collected from the four sites at different seasons (Wilson 1998).Moreover,Nielsen et al.(1999)did not find root-associated vesicular arbuscular mycorrhizae (V AM)J.Sakayaroj (*):S.Preedanon :E.B.G.JonesPhylogenetics Laboratory,National Center for Genetic Engineering and Biotechnology,113Pahonyothin Road,Klong 1,Klong Luang,Pathumthani 12120,Thailand e-mail:jariyask@biotec.or.thO.Supaphon :S.PhongpaichitDepartment of Microbiology,Faculty of Science,Prince of Songkla University,Hat Yai,Songkhla 90112,ThailandFungal Diversity (2010)42:27–45DOI 10.1007/s13225-009-0013-9from Z.marina and T.testudinum.They hypothesized that due to the effect of low oxygen levels and high salinity there was reduced fungal colonization of seagrass species(Nielsen et al.1999).Subsequently,Devarajan et al.(2002)reported six endophytic fungi from leaf blades,petioles and rhizomes of the tropical seagrass Halophila ovalis.Most of the endophytes were anamorphic fungi.Additionally,diverse endophytic assemblages from T.testudinum,Z.japonica and Z.marina in Hong Kong and the Philippines were reported by Alva et al.(2002).Ninety-five fungal isolates were recovered with23anamorphic fungi as the most abundant group.Among these isolates,over75%were able to produce lignin modifying enzymes(Alva et al.2002).Recently, damaged and undamaged leaves of T.testudinum from Puerto Rico were studied for the presence of endophytes.A total of13species from eight genera were isolated and screened for bioactive compound production(Rodríguez 2008).Thailand is a biodiversity rich country in the tropical zone with circa6,000fungi reported(Jones and Hyde 2004).However,only34%of the described marine fungi have been documented for Thailand(Jones et al.2006)with most studies restricted to investigations of lignicolous species on marine and mangrove wood(Jones et al. 2006).There is no report on fungi associated with other marine substrata,especially the molecular study of seagrass endophytes.Therefore,as part of the Khanom MarineBiodiversity Project at Hat Khanom-Mu Ko Thale Tai National Park,the present study was undertaken to develop baseline research of marine microorganisms,in order to encourage awareness in conservation of the bioresources in the local marine protected area.The objectives of this study were(1)to investigate for endophyte assemblages in Enhalus acoroides,and,(2)to describe the diversity of endophytes based on LSU,ITS1,2,5.8S rDNA sequence analyses.Materials and methodsPlant and sampling sitesThe tropical seagrass Enhalus acoroides(family Hydro-charitaceae)(Phang2000)was collected from two sites at Had Khanom-Mu Ko Thale Tai National Park.Enhalus acoroides is the most abundant and common species in the National Park.It is the largest seagrass species with the strap-like leaves1-2cm wide and30cm-1.5m long (Fig.1).Had Khanom-Mu Ko Thale Tai National Park is located offshore in Nakhon Si Thammarat Province,southern Thailand.Tarai Island(09°19′16.56″N,99°46′44.52″E)and Taen Island(09°22′15.68″N,99°57′11.80″E)were chosen as the collecting sites in this study(Fig.2). Sample collections and isolation of endophytesHealthy symptomless,intact,undamaged E.acoroides leaves were randomly collected over the four collection periods in October2007,December2007,March2008and April2008.Samples were placed in plastic bags and kept in cold storage while transportation to the laboratory.The surfaces of all leaves were gently scraped by fingers and washed in running tap water for10min before placement in the sterilizing solutions.Preliminary experiments were conducted based on the studies of Wilson(1998)and Alva et al.(2002)and the following protocol for surface-sterilization was established(25%ethanol for3min,3%sodium hypochlorite for10seconds,25%ethanol for3min and distilled water).Seagrass leaf tissues used for endophytic isolation included:the innermost youngest,middle and outermost oldest tissues;upper,middle and lower sections. After immersion of leaves in different solutions,they were blotted dry with sterile paper towels.Five segments(0.5×0.5cm)were cut from each leaf with a flamed scalpel on a sterile Petri plate,and placed onto potato dextrose agar(PDA) with and without salt containing0.5g/l penicillin Gand Fig.1Enhalus acoroides used in this study a The strap-shaped leaves arise directly from the rhizomes and can reach1.5m in length,b Strap-like symptomless leaves used for endophyte isolationstreptomycin.All plates were incubated in the dark at 25°C and observations made daily for 4weeks.Emerging fungal hyphae were subcultured onto fresh PDA plates,with or without salt depending on the original substrate from which the colony grew.Live cultures were deposited at BIOTEC Culture Collection (BCC).Overall fungal isolation rate (IR)and colonization rates (CR)were determined as described by Petrini et al.(1982).Isolation Rate (IR)=Total number of isolates yielded by a given sample divided by total number of leaf segments in that sample.Colonization rates (CR)=Total number of leaf disc segments in a sample yielding ≥one isolate divided by total number of leaf disc segments in that sample.Morphological and molecular identificationEndophytic fungi obtained were identified based on their morphology and sporulation on selected medium,and confirmed by rDNA sequence analyses.DNA extraction,PCR amplification and DNA sequencing A fine powder of fungal mycelia was placed into 400μL lysis buffer.The tube was then incubated at 70°C for 30min.Geno-mic DNA extraction was performed following the procedure by O ’Donnell et al.(1997).DNA was amplified with Taq DNA polymerase from DyNAzyme ™II DNA Polymerase Kit,FINNZYMES,rge subunit and ITS1,2, 5.8S ribosomal DNA were amplified using fungal universal primers (White et al.1990;Landvik 1996).PCR products were purified and directly sequenced by the Macrogen (Korea).Phylogenetic analysisThe sequences were analyzed along with other sequences obtained from the GenBank database with suitable out-group taxa.Sequences were aligned in Clustal W 1.6program (Thompson et al.1994)and refined visually BioEdit version 6.0.7(Hall 2004).Alignments were entered into PAUP 4.0b10(Swofford 2002).Phylogenetic trees were generated using maximum parsimony analysis.All characters were equally weighted,followed by heuristic searches with a stepwise starting tree,a random stepwise addition of 100replicates and tree-bisection-reconnection (TBR)branch-swapping algorithm.Gaps were treated as missing data.The maximum number of retained trees was restricted to one thousand (option MAXTREES 1000).The most parsimonious trees (MPTs)were tested for the best topology with the Kishino-Hasegawa (K-H)maximum likelihood test (Kishino and Hasegawa 1989)to find the most likely tree for the dataset.The tree length,consistency indices (CI),retention indices (RI)and rescaled consistency indices (RC)were calculated for each tree generated.Finally,1,000replicates of bootstrapping (BP)analysis (Felsenstein 1985)were performed (full heuristic searches,stepwise addition of sequence,10replicates of random addition of taxa,TBR branch-swapping algorithm).Bayes-ian phylogenetic inference was calculated with MrBayes 3.0b4with general time reversible (GTR)model of DNA substitution and a gamma distribution rate variation across sites (Huelsenbeck and Ronquist 2001).Four Markov chains were run from random starting trees for 5,000,000generations and sampled every 100generations.The first 100,000generations were discarded as burn-in of the chain.A majority rule consensus tree of all remaining trees,as well as the posterior probabilities (PP),was calculated.ResultsDiversity of culturable endophytic fungi from Enhalus acoroidesTo identify the endophytic fungi,microscopic morphology was studied for those that sporulated on selected medium.However,most of the isolates were sterile mycelia (Table 1).Phylogenetic placement of fungal assemblages based on LSU rDNA sequence analysisThe alignment of 131taxa comprising 2,576nucleotide bases resulted from an LSU rDNA dataset.Maximum parsimony analysis yielded 44MPTs of 2,576changes,CIFig.2Map of Had Khanom Mu-Ko Thale Tai National Park showing the two collecting sitesTable1Endophytic fungi isolated and identified in the current studyOriginal code BCC code Sampling site Morphologicalidentification Proposed molecularidentificationAccession numberLSU rDNA ITS1,2,5.8S rDNAKH0017729614Tarai Island Sterile mycelia Hypocreales sp.GU017527GU017486 KH0018428682Tarai Island Sterile mycelia Phoma sp.GU017528GU017487 KH0019828647Tarai Island Sterile mycelia Hypocreales sp.GU017529GU017488 KH0020228691Tarai Island Sterile mycelia Hypocreales sp.GU017530GU017489 KH0020328692Tarai Island Sterile mycelia Hypocreales sp.GU017531GU017490 KH0020628650Taen Island Sterile mycelia Hypocreales sp.GU017532GU017491 KH0022328659Tarai Island Sterile mycelia Hypocreales sp.GU017533GU017492 KH0022428660Tarai Island Sterile mycelia Hypocreales sp.GU017534GU017493 KH0025129616Taen Island Aspergillus sp.Aspergillus penicillioides GU017535GU017494 KH0025429617Tarai Island Sterile mycelia Mycosphaerella sp.GU017536GU017495 KH0025629618Taen Island Aspergillus sp.Aspergillus penicillioides GU017537GU017496 KH0026529300Taen Island Sterile mycelia Pleosporales sp.GU017538GU017497 KH0027729305Tarai Island Cladosporium sp.Cladosporium sp.GU017539GU017498 KH0027829306Taen Island Sterile mycelia Bipolaris sp.GU017540GU017499 KH0027929619Taen Island Aspergillus sp.Aspergillus penicillioides GU017541GU017500 KH0028029307Tarai Island Cladosporium sp.Cladosporium sphaerospermum GU017542GU017501 KH0028129620Tarai Island Sterile mycelia Scolecobasidium sp.GU017543GU017502 KH0028229308Tarai Island Sterile mycelia Calosphaeriaceae sp.GU017544GU017503 KH0028329621Tarai Island Sterile mycelia Scolecobasidium sp.GU017545GU017504 KH0029029624Taen Island Cladosporium sp.Cladosporium sp.GU017546GU017505 KH0029129625Tarai Island Sterile mycelia Nigrospora sp.GU017547GU017506 KH0029229626Tarai Island Sterile mycelia Nigrospora sp.GU017548GU017507 KH0029329627Tarai Island Sterile mycelia Hypocreales sp.GU017549GU017508 KH0029429628Tarai Island Sterile mycelia Hypocreales sp.GU017550GU017509 KH0029529629Tarai Island Sterile mycelia Hypocreales sp.GU017551GU017510 KH0029629630Tarai Island Sterile mycelia Hypocreales sp.GU017552GU017511 KH0030029916Tarai Island Sterile mycelia Mycosphaerellaceae sp.GU017553GU017512 KH0030230380Tarai Island Sterile mycelia Peniophora sp.a GU017554GU017513 KH0030330381Tarai Island Penicillium sp.Penicillium sp.GU017555GU017514 KH0030430382Tarai Island Penicillium sp.Penicillium sp.GU017556−KH0030530383Tarai Island Sterile mycelia Herpotrichiellaceae sp.GU017557GU017515 KH0030929918Tarai Island Penicillium sp.Penicillium sp.GU017558GU017516 KH0031029919Tarai Island Penicillium sp.Penicillium sp.GU017559GU017517 KH0031129920Tarai Island Penicillium sp.Penicillium sp.GU017560GU017518 KH0031229921Tarai Island Penicillium sp.Penicillium sp.GU017561GU017519 KH0031329922Tarai Island Penicillium sp.Penicillium sp.GU017562GU017520 KH0031429923Tarai Island Penicillium sp.Penicillium sp.GU017563GU017521 KH0031529924Tarai Island Penicillium sp.Penicillium sp.GU017564GU017522 KH0031629925Tarai Island Cladosporium sp.Cladosporium sp.GU017565GU017523 KH0031729926Taen Island Sterile mycelia Phaeosphaeriopsis sp.GU017566GU017524 KH0031929928Tarai Island Penicillium sp.Penicillium sp.GU017567GU017525 KH0032129930Taen Island Sterile mycelia Hypocreales sp.GU017568GU017526a Basidiomyceteof0.401,RI of0.880and RC of0.353.The phylogenetic tree showed that our endophytes were a diverse fungal group and mainly distributed within the Ascomycota (98%).The most frequent group at36%,were Sordario-mycete species,while33%grouped in the Eurotiomycetes, 24%in the Dothideomycetes and5%were placed with the anamorphic Pezizomycotina.One strain was a member of the Agaricomycetes in the Basidiomycota(2%)(Fig.3).Ten fungal strains were located in the Dothideomycetes within two well defined orders,the Pleosporales and Capnodiales.The Pleosporales included five endophytic assemblages with77%BP and 1.00PP support and distributed in several subgroups.KH317was closely related to an unidentified Pleosporales species FJ176899with84% BP and0.97PP.Endophyte KH278and two Cochiobolus species formed a subclade,but with weak low statistical support.Many Phoma species and fungal endophytes formed a clade with our fungal assemblage KH184,with100%BP and0.98PP support.Moreover,the other two fungal endophytes,KH265and KH300,always form a distinct branch without any reference species in the Pleosporales.Phylogenetic analysis of the Capnodiales confirmed the placement of five endophytes,with100%BP and1.00PP support values.KH254had close affiliation with many Mycosphaerella species(BP<50%,PP<0.95),while anoth-er four strains were well placed in the Cladosporium subclade,with100%BP and1.00PP support.Fifteen fungal strains were well placed in the Sordar-iomycetes within three orders,the Hypocreales,Calosphaer-iales and Trichosphaeriales(100%BP, 1.00PP).The Hypocreales was the dominant group within the Sordario-mycetes comprising12fungal assemblages.Six isolates (KH294,KH296,KH295,KH293,KH321,KH177)formed a subclade and had affinity with Cordyceps sinensis AB067710and Hypomyces viridigriseus AM779858,al-though with weak support(<50%BP,<0.95PP).Moreover, another six endophytes(KH202,KH224,KH198,KH223, KH203,KH206)formed a subgroup(100%BP,1.00PP) with the genera Geosmithia,Hapsidospora and an uniden-tified hypocrealean sequence.Molecular evidence of endophyte KH282revealed that it had a close affiliation with the genera Phaeoacremonium and Togninia(100%BP,1.00PP).Additionally two isolates, KH291,KH292were well distributed in the Trichosphaer-iales.Their LSU sequences matched with sequences of fungal endophytes AB438561,EU541479,Nigrospora sp. EU852533,Nigrospora oryzae FJ176892and Apiospora-ceae sp.EF564155with100%BP,1.00PP support.Two fungal endophytes were placed in Pezizomycotina incertae sedis as a basal clade of the Sordariomycetes. KH281and KH283were monophyletic and related to various species of Scolecobasidium spp.and Fusicladium amoenum EU035425,with100%BP,1.00PP support.Fourteen fungal strains were placed in the Eurotiomy-cetes within two orders,the Chaetothyriales and Eurotiales, the latter with most taxa(13fungal assemblages).Ten strains(KH314,KH303,KH312,KH313,KH315,KH319, KH309,KH311,KH310,KH304)were closely related and formed a subclade with the genera Penicillium and Eupenicillum(79%BP, 1.00PP).While another three assemblages(KH251,KH279,KH256)were monophyletic and well delineated in the Eurotium/Emericella subclade.Endophyte KH305belonged to the Chaetothyriales with strong support(100%BP,1.00PP),in a subclade to the genera Sarcinomyces,Phaeococcomyces,Exophiala and Glyphium.Only one fungal strain(KH302)was classified in the Agaricomycetes,Basidiomycota.The LSU sequence anal-ysis showed that KH302had close affiliation with genus Peniophora,and in particular with Peniophora incarnata AF506425(<50%BP,<0.95PP).Phylogenetic placement of fungal assemblages at species levelIn order to determine the specific relationships of isolated species alignments of ITS1,2,5.8S rDNA sequences were incorporated into four data matrices comprising representa-tives from the Eurotiomycetes,Dothideomycetes,Sordar-iomycetes and Basidiomycota.The ITS1,2,5.8S rDNA data matrix of the Dothideo-mycetes comprised taxa from the Pleosporales,Capno-diales,Botryosphaeriales and Pezizomycotina incertae sedis.Maximum parsimony analysis from66taxa of756 base pairs yielded1,000MPTs of2,747changes,CI of 0.448,RI of0.788and RC of0.353(Fig.4).The Pleosporales was supported by high statistical support of 80%BP and 1.00PP.KH317clustered within the Phaeosphaeriopsis/Phaeosphaeria subclade,with Phaeos-phaeriopsis musae DQ885894as the most similar taxon (99.5%.).Therefore,KH317,based on sequence homology, can be tentatively identified as a Phaeosphaeriopsis sp.KH278was placed in a subclade comprising the genus Bipolaris,Pleosporaceae sp.and an uncultured endophytic fungus.The most related species of KH278was Bipolaris sp. EU668993,with nucleotide similarity of99.8%(100%BP, 0.97PP).Thus,it should be named as a Bipolaris sp. Additionally,KH184clustered with several Phoma species and uncultured endophytic fungi with a sequence similarity between96.8–98.6%.This grouping was well established by 100%BP rge subunit rDNA analysis supported the placement of KH184in the genus Phoma, suggesting that it could be identified as a Phoma sp. Moreover,endophyte KH265was well placed in the lower Pleosporales subclade which comprised the genera Massar-ina,Lophiostoma and fungal endophyte AF383957.KH265was closely related to Massarina sp.DQ863675with90.5% sequence similarity(<50%BP,0.95PP).Therefore,KH265 can be tentatively identified as a pleosporaceous species.The Capnodiales comprising two subclades was well delineated in the Dothideomycetes.Four fungal assemb-lages(KH280,KH316,KH277,KH290)were closely related to several Cladosporium species(98.3–100% nucleotide similarity).KH280can be named as C. sphaerospermum,as it had identical sequence similaritywith C.sphaerospermum from GenBank.The other three strains can referred to as Cladosporium sp.,with a lower sequence homology.Additionally,KH254and KH300had affinity with the genera Mycosphaerella,Teratosphaeria and uncultured marine ascomycete.KH254formed a sister group to the Mycosphaerellaceae,consistent with the LSU rDNA phylogeny.KH300had no close reference species and thus can only be referred to the Mycosphaerellaceae.Another two fungal assemblages,KH281and KH283, had close affiliation with Pezizomycotina incertae sedis (100%BP, 1.00PP).This lineage comprised several Scolecobasidium species isolated from marine habitats (De la Cruz et al.,unpublished;Preedanon2007)and an unidentified ascomycete EF191422.KH281was highly similar to Scolecobasidium sp.EU714392(99.6%nucleo-tide similarity),while KH283clustered as a sister taxon with lower sequence similarity(94.3%).Thus KH281and KH283were identified as Scolecobasidium spp.Analyses of ITS1,2,5.8S sequences of the Sordariomy-cetes were further performed.Maximum parsimony analyses of48taxa of600base pairs resulted in1,000MPTs of1,101 changes,CI of0.592,RI of0.856and RC of0.507(Fig.5). The statistical values from MP and Bayesian inferences were well supported at the ordinal levels for the Hypocreales, Trichosphaeriales and Calosphaeriales.Six endophytes (KH294,KH295,KH296,KH293,KH321,KH177)belong-ing to the Hypocreales formed a monophyletic group without similar taxa,with nucleotide similarity between90–100%. KH294,KH295,KH296were highly similar(99.1–100% similarity),while KH293and KH321had identical sequence (99.7%similarity)and KH177formed a sister taxon without any related species.The most closely related taxon was an uncultured Hypocreales species EF619703,but with low sequence similarity(83.5%).Another six fungal endophytes (KH206,KH198,KH203,KH202,KH224,KH223)were well placed in a second Hypocreales clade,with Geosmithia as a basal related genus.They were monophyletic with identical(100%)sequence similarity,with a leaf litter ascomycete AF502729the closest taxon with97.8% nucleotide similarity(100%BP,1.00PP).Therefore,as a result of the lack of known reference species,the isolate can only be referred to the Hypocreales.Two endophytes(KH291,KH292)were placed in the Trichosphaeriales comprising several Nigrospora species.KH292clustered with a fungal endophyte EU360454,an uncultured fungus FJ213549,Nigrospora oryzae EU427044, EU918714and Nigrospora sp.EU714382,respectively. The nucleotide similarities of the group were96.7–99.7%. KH291was most similar to Nigrospora sp.EU714386and fungal endophyte EU360458,with lower nucleotide similar-ity(94.3–94.5%).The LSU phylogeny supported the identification of these endophytes as Nigrospora species.KH282had affiliation with the genera Phaeoacremonium and Togninia in the Calosphaeriales,and formed a long branch with Phaeoacremonium inflatipes AF118140, AF197990,P.rubrigenum AF118139and P.griseorubrum EU128926(98%BP,1.00PP),but had no closely related taxa.Sequence similarities were relatively low(89.3–90.6%), and the fungus should be referred to the Calosphaeriaceae.Maximum parsimony analysis of46taxa of614base pairs from the Eurotiomycetes yielded1,000MPTs of640 changes,CI of0.783,RI of0.934and RC of0.731(Fig.6). This tree comprised three subclades in two orders,Euro-tiales and Chaetothyriales.Nine fungal assemblages (KH303,KH315,KH314,KH313,KH312,KH311, KH310,KH309,KH319)grouped in a Penicillium sub-group with100%identical sequence similarity with several sequences of Penicillium griseofulvum and P.citrinum.The ITS region of endophyte KH304was not successfully amplified,however,it was well placed within this group based on LSU rDNA sequence analysis.Therefore,as the result of sequence homology to GenBank database,these endophytes can be named as Penicillium spp.Three fungal strains(KH256,KH251,KH279)grouped within a subgroup comprising several Aspergillus penicil-lioides strains,with high nucleotide similarities(99.5–100%,100%BP,1.00PP).Thus this species is identified as A.penicillioides.One isolate(KH305)belonging to the Chaetothyriales had affiliation with the genera Coniosporium,Phaeococco-myces,Exophiala and an uncultured ascomycete(98%BP, 1.00PP).However,KH305had no closely related species from the GenBank database and its nucleotide similarity with these grouping was relatively low(83.3–87.6%),and the isolate is referred to the Herpotrichiellaceae.The ITS1,2,5.8S data matrix of the Agaricomycetes (Basidiomycota)comprised members from the Russulales, Agaricales and Polyporales.Maximum parsimony analysis Fig.3Phylogenetic tree generated from maximum parsimony of LSU rDNA sequences(2,576base pairs)from endophytes isolated from this study within different classes of Ascomycota and Basidiomycota. Chytridiomycota is used as an outgroup.Thickened branches represent clades with Bayesian posterior probabilities greater than 0.95.Bootstrap support values higher than50%are shown above each branch.Tree length=2,576changes,CI=0.401,RI=0.880,RC= 0.353.Branch lengths are proportional to number of character state changesbPhaeosphaeria avenaria f.sp. EF 590322 Phaeosphaeria nodorum EF 590320sp EF 99Phaeosphaeria sp. EF 590325Fungal endophyte DQ 979424 Fungal endophyte DQ 979418Fungal endophyte DQ 97Fungal endophyte DQ 979458Pleosporales sp. FJ 176899KH 317Leptosphaeria maculans DQ 4709468084Cochliobolus heterostrophus AY 544645Cochliobolus sativus DQ 678045KH 278Pl h b 15261483Pleospora herbarum AY 152614 Pleospora herbarum DQ 678049Pleospora papaveracea AY 152615Alternaria alternata 7710058Alternaria alternataDQ 678082Fungal endophyte EF 420043Massarina phragmiticola DQ 813510Massarina arundinacea 10010096Massarina arundinacea DQ 813509Massarina ramunculicola DQ 528762Phoma sojicola EU 167568 Phoma pinodella EU 1675659277PleosporalesKH 184Fungal endophyte EF 420037Fungal endophyte EF 420033Ph h b 10064Phoma herbarum DQ 678066Phoma herbarum AY 293791Lophiostoma macrostomum AB 433274Lophiostoma crenatum 100Lophiostoma crenatum DQ 678069KH 265Fungal endophyte EF 420041Fungal endophyte EF 98998190Fungal endophyte EF420054Preussia terricola AY 544686Ulospora bilgramii DQ 384108Lepidosphaeria nicotiae DQ 6780677793p p Q KH 300Botryosphaeria viticola DQ 678087Mycosphaerella ambiphylla DQ 246219100Mycosphaerella molleriana DQ 246223Mycosphaerella nubilosa DQ 246229Phaeophleospora eucalypti DQ 246224 M h ll t i i 8010087DOTHIDEOMYCETES Mycosphaerella tasmaniensis DQ 246233Mycosphaerella suberosa DQ 246235KH 254Mycosphaerella parkii 71Mycosphaerella parkii DQ 246245Mycosphaerella madeirae DQ 204756KH 290Cladosporium uredinicola 98100100CapnodialesCladosporium uredinicolaDQ 008147Cladosporium cladosporioides DQ 008145Cladosporium sphaerospermum AY 342104KH 280Cladosporium sphaerospermum AY 342102KH 277KH 316100KH 294KH 296KH 29510098KH 293KH 321KH 177Cordyceps sinensis 100Cordyceps sinensisAB 067710Hypomyces viridigriseus AM 779858Fungal endophyte EF 420077 Niesslia exilis AY 48972099Acremonium breve FJ 176882Niesslia exilis AY 489718KH 20296HypocrealesKH 224KH 198KH 223100KH 203KH 206Geosmithia lavendula AM 949861sp AM 9684Geosmithia sp. AM 421074Hapsidospora irregularis AF 096192 Hypocreales sp. DQ 327628Bionectriaceae sp. AB 2948059688o ec aceae sp.9805Phaeoacremonium tuscanum EU 863527Togninia minima AY 761082Phaeoacremonium hungaricum EU 863530 5299CalosphaerialesPhaeoacremonium sphinctrophorum DQ 173152KH 282Fungal endophyte AB 438561100100KH 292Fungal endophyte EU 541479Nigrospora sp. EU 85253370TrichosphaerialesSORDARIOMYCETES KH 291Nigrospora oryzae FJ 176892Apiosporaceae sp. EF 564155 Scolecobasidium variabile 10731074100Scolecobasidium variabile EU 107310 Scolecobasidium verruculosum EU 107308KH 283KH 2819610097Pezizomycotina Scolecobasidiella avellanea EF 204505Fusicladium amoenum EU 035425100Incertae Sedis。