rGO-Fe3O4 合成
多孔石墨烯的制备与应用研究进展

多孔石墨烯的制备与应用研究进展白瑞; 牛永安; 刘皓; 卢翠英; 高平强; 刘丽娜【期刊名称】《《河南科学》》【年(卷),期】2019(037)009【总页数】7页(P1408-1414)【关键词】石墨烯; 多孔石墨烯; 制备方法; 应用【作者】白瑞; 牛永安; 刘皓; 卢翠英; 高平强; 刘丽娜【作者单位】榆林学院化学与化工学院陕西榆林 719000; 沈阳化工大学材料科学与工程学院沈阳 110000【正文语种】中文【中图分类】O646石墨烯[1-4],一种由碳原子以sp2杂化形成呈六边形蜂巢晶格的二维碳纳米片层,作为碳家族(包括无定型碳、石墨、金刚石、富勒烯、碳纳米管、石墨炔等)中的一名新成员,因其具有独特的电子迁移性、超大的比表面积、高的机械强度、优异的耐腐蚀性和表面化学结构易调控性等特性而受到了广泛的关注,在微纳电子器件、吸附、催化、能量存储与转化等领域崭露头角[5-8]. 然而,原始的石墨烯相对来说价格昂贵、水溶性差、有效比表面积低、吸附能力和可回收性低,以及复杂的后处理限制了它们的实际应用. 因此,许多研究者受石墨烯优异性能的启发,对石墨烯相关材料进行了广泛的实验和理论研究.石墨烯被视为构建其他sp2碳质材料的基本结构单元. 通过石墨烯的组装获得石墨烯相关材料不仅能实现对石墨烯微观结构和宏观织构的调控,同时也能衍生出独特的新性质,将会极大拓展石墨烯的应用范围[9]. 因此,越来越多的学者开始研究如何通过石墨烯的结构组装来控制材料形貌和内部架构,从而达到满足各种应用的目的. 多孔石墨烯[10-11]在这种背景下应运而生.1 多孔石墨烯的制备多孔石墨烯是一类具有纳米级孔结构的石墨烯相关材料[12]. 近年来,为了满足不同应用的需要,人们致力于开发各种形貌、结构和性能的多孔石墨烯的合成方法. 目前一般将其制备方法分为自组装法、模板辅助法和直接沉积法三大类[13]. 1.1 自组装法自组装法是获得三维多孔石墨烯最常用的策略之一. 目前已经开发了许多基于该策略的方法. 自组装法[14]是指基本结构单元(分子、纳米材料、微米或更大尺度的物质)在一定条件下自发形成有序结构的一种技术. 在自组装的过程中,基本结构单元在基于非共价键的相互作用下自发的组织或聚集为一个稳定、具有一定规则几何外观的结构.但是,石墨烯液相分散性差、化学活性低,因此以石墨烯为原料的进行自组装存在一定的困难. 作为石墨烯重要的衍生物(氟化石墨烯[15-16]、氢化石墨烯[17-18]和氧化石墨烯[19-20])之一——氧化石墨烯,由于其片层之间的范德华吸引力与其表面官能团的静电排斥力之间存在力平衡,这使得氧化石墨烯在水性溶剂中很好地分散.与石墨烯相比,氧化石墨烯克服了上述缺点,是实现石墨烯自组装的优选材料. 通过氧化石墨烯分散体的凝胶化过程和后还原过程来产生三维多孔石墨烯网络状结构是最典型的自组装方法. 目前引发氧化石墨烯分散体凝胶化的方法很多,如加入交联剂(如PVA、DNA、金属离子、聚合物及有机分子等)、加入弱还原剂(如硼氢化钠、柠檬酸钠、维生素C、氢碘酸等)改变分散体系的pH或者对分散体进行超声波处理[21]. 除了凝胶化这一基本方法外,氧化石墨烯片层的自组装还可以通过冷冻干燥法、电化学沉积法、流延成型法、真空抽滤法和溶胶凝胶法等其他方法实现. 然后通过水热法或者化学还原法获得还原石墨烯(rGO)结构的三维多孔石墨烯. Kumar[22]等采用一锅微波法合成了三维Fe3O4/rGO杂化材料,如图1所示. 研究表明这种杂化材料的三维网状结构是由Fe3O4纳米粒子诱导形成的,且该材料具有优异的电化学性质,比电容达455 F/g、扫描速度达8 mV/s以及良好的循环稳定性.图1 一锅微波法合成三维Fe3O4/rGO杂化材料流程图[22]Fig.1 The procedure of Fe3O4/rGO composite materials synthesized by one-pot microwave approach[22]1.2 模板辅助法模板法是制备微孔或中孔最有效的途径之一. 与自组装法相比,采用模板辅助法可以更准确地控制多孔石墨烯的微观形貌和孔结构尺寸. 目前已经报道的制备多孔石墨烯的模板辅助法有化学气相沉积(CVD)法、冰模板法、高分子聚合物法、水滴模板法等.Li[23]以NiCl2·6H2O作为多孔Ni骨架的催化剂前躯体,在600 ℃、Ar/H2气氛中还原前躯体形成3D多孔交联的Ni骨架,以多孔Ni骨架为模板,使用甲烷为碳源,采用快速CVD法在几秒钟到几分钟内进行高效生长,在生长过程中经高温退火,最后通过在FeCl3/HCl溶液中刻蚀掉模板制备了高密度的多孔石墨烯(3D-GMO),如图2所示. 这种3D-GMO具有高电导率(12 S/cm)、大比表面积(560 m2/g)以及对重金属离子具有超高的吸附容量(Cd2+434 mg/g、Pb2+882 mg/g、Ni2+1683 mg/g、Cu2+3820 mg/g)和快速解析的特点. 另外,泡沫镍、阳极氧化铝(AAO)、MgO、金属纳米结构甚至金属盐也可作为模板制备3DGM.图2 商业镍泡沫生长石墨烯和多孔交联镍生长石墨烯的比较[23]Fig.2 Comparison between commercial Ni foam-grown graphene and our porous cross-linked Ni-grown graphene[23]注:a,b是泡沫镍去除前后;c,d多孔交联镍刻蚀前后.此外,还可以另一种方便的方式获得三维多孔石墨烯. 通过将氧化石墨烯片组装到3D模板上,然后将氧化石墨烯还原为还原石墨烯. 目前已经发展了许多组装技术,如电泳沉积、浸渍涂覆、高压釜回流模板辅助冷冻干燥等. 这些方法中使用的模板除了金属基底还可以是非金属基底,已报道的非金属包括二氧化硅纳米颗粒(NPs)、聚苯乙烯(PS)微球、聚甲基丙烯酸甲酯(PMMA)微球、Nafion支架、纤维素和纺织纤维等都可以作为制备了三维多孔石墨烯的模板[24-25].图3 模板法制备的三维空心碳结构的过程Fig.3 The procedure for the preparation of three-dimensional hollow carbon structures by template methodHan[26]等报道了一种具有高效吸附性能的三维多孔石墨烯材料的制备方法. 通过采用模板辅助冷冻法和热还原法制备了具有优异热稳定性和大比表面积的亚微米级多孔石墨烯材料. Moon[27]等报告了一种合成良好控制的三维碳纳米结构方法,如图3所示. 他们采用氧化石墨烯为原料、球形的氧化铝包覆二氧化硅(ACS)为模板,通过氧化石墨烯在ACS的氧化铝催化位点聚合,然后在高温900 ℃、Ar 气氛下碳化形成石墨烯填充球形模板周围的复合物,最后HF刻蚀获得了三维空心碳机构的方法. 他们还发现根据氧化石墨烯的含量可以系统地调整复合材料的形貌(从层状复合材料到三维中孔结构,再到微孔材料). 由此获得的高比表面积和高孔隙率的复合材料在高电流密度下能显著提高其电容.1.3 直接沉积法通过在导电衬底上直接沉积获得三维多孔石墨烯结构是一种简单的方法. 目前已经有研究者采用等离子体技术在金和不锈钢衬底上进行直接沉积,获得了牢固附着在衬底上的三维多孔石墨烯. 这种多孔石墨烯在片层边缘有众多的活性位点适合于传感应用. Mao[28]等制备了一种生物传感器,该生物传感器由在金电极上垂直生长的石墨烯片和抗体偶联物组成,可以提供免疫球蛋白2 ng/mL的低检测限. 此外,具有设计特征的金属衬底也可以容易地控制三维多孔石墨烯结构,这可能使得能够针对不同的应用构建各种传感器结构.除上述三类办法外,在最近的一项工作中,Niu[29]等采用所谓的“发酵”策略,类似于烘焙面包的过程来制备多孔石墨烯膜,其中致密的氧化石墨烯膜充当“面团”的作用. 采用AAO(氧化铝)膜过滤氧化石墨烯分散液,然后从AAO膜上剥离获得氧化石墨烯薄膜. 然后在90 ℃高压釜中放置10 h后,还原石墨烯膜上形成大量气孔,这是由于GO的还原使气相物质从致密膜中快速释放. 由于石墨烯的多孔结构和疏水性,与没有孔结构的石墨烯膜相比,该多孔石墨烯膜对有机溶剂如机油和石油的吸附能力有所提高.2 多孔石墨烯的应用2.1 超级电容器超级电容器具有功率密度高、循环寿命长、快速充放电等优点,具有广阔的应用前景[30]. 活性炭、碳纳米管、石墨烯等材料以其低成本、高导电性、高比表面积等特点被广泛应用于超级电容器电极的构造. 但是,由于π-π层叠的相互作用和范德华在基平面间的吸引,氧化石墨烯或石墨烯片趋向于形成层叠石墨烯结构,导致其表面积的显著损失. 氧化石墨烯或石墨烯片的重新填充和聚集也阻碍了石墨烯材料在许多应用中的大规模使用和工艺,特别是用作储能器件,将导致电解质离子难以进入密集填充的石墨烯片材之间的间隙[31].三维多孔石墨烯的独特性能和多孔结构不仅提高了电解质对电极表面的易接触性,而且为电极表面修饰的活性物质提供了导电通道,提高了电化学性能.为了改善三维多孔石墨烯超级电容性能,人们已经广泛地探索出了由具有高理论电容的电容材料(如金属氧化物导电聚合物)和三维多孔石墨烯组成的复合多孔石墨烯电极. 该复合电极具有较高的比容量和能量密度以及具有较好的倍率性能和较长的循环寿命[32]. 复合材料的超级电容器性能的提高通常来自于石墨烯和其他组分的协同作用. 具体为:首先,电容材料不仅对整个复合电极产生伪电容,而且还起到了隔离材料的作用. 石墨烯片之间的间隙,导致电解液对电极的可获得性的提高. 第二,互连的石墨烯片为复合材料提供导电通道,使得复合材料中的电荷传输迅速,并且在高充放电电流密度下保持了电极的良好性能[33].2.2 气体分离净化从当前研究水平和技术水平来看,无论是科学研究还是工业应用,对高纯气体(如He、Ar或H2等)需求量很大. 与传统的气体分离方法(如低温蒸馏、变压吸附)相比,膜分离具有较低的能源成本、对使用规模要求低并且具有较少的机械复杂性[34]. 气体膜分离的原理是根据不同气体的扩散速率差异进行的. 目前已开发的各种传统膜,包括金属、沸石、聚合物等[35-36],其膜厚度范围在10~103 nm. 但是,膜的透过性与它的厚度成反比,这在一定程度上制约了其分离性能. 只有一个原子厚度的石墨烯被认为是理想的膜分离材料[37].但是,即使是最小的气体原子氦,完美的石墨烯也是不可渗透的(因为密集填充的蜂窝状晶格足够防止任何原子和分子穿过它). 为了探索石墨烯潜在的高渗透性,在石墨烯中引入孔隙是必要的. Bieri[38]等成功合成聚苯型多孔石墨烯,这种多孔石墨烯只有一个原子厚度、具有按规律排布的孔以及均匀的空隙大小,在气体净化中开始应用.2.3 储氢材料氢气作为可再生能源具有储量丰富、清洁、高效的特点而备受关注,预计在未来将是主要能源. 但是,氢气制造业面临的最大挑战是寻找一种安全有效储氢材料. 石墨烯具有高体积密度,氢气分子可以吸附在石墨烯的两侧[39]. 但是,在石墨烯片层和氢气之间范德华力相互作用比较弱,这会导致其储氢性能下降. 引入杂原子,特别是金属原子被认为是一种增强石墨烯片层和氢气相互作用的方式[40]. 多孔的石墨烯有助于避免金属聚集现象产生. Du[41]等研究者采用第一原理计算研究Li修饰的多孔石墨烯的储氢性能,并预测金属修饰多孔石墨烯是一种有前途的储氢材料. Reunchan[42]采用第一原理计算研究了不同金属原子在多孔石墨烯吸附氢分子(H2)中的作用. 对每个金属原子在多孔石墨烯中的结合位点和结合能进行研究计算,结果表明H2在碱金属、碱土金属或过渡金属分别修饰的多孔石墨烯介质表现出不同吸附特性. 其中钙修饰的多孔石墨烯被认为是大容量储氢材料. Ao[43]将铝修饰多孔石墨烯作为储氢材料对其进行密度泛函计算. 结果表明,铝修饰的多孔石墨烯的储氢容量为10.5 wt%,氢吸附能从1.11 eV降低到0.41 eV,在常压下氢气可实现有效的存储/释放. 此外,该研究还发现由于不同吸附位点的吸附能量不同,氢气可以分三个阶段逐渐释放,这在实际储氢应用中是理想的. 并且通过对体系的原子电荷、电子分布和态密度的分析研究,剖析了铝原子修饰的多孔石墨烯改善储氢性能的机理.2.4 DNA检测快速、低成本、可靠的DNA测序是近年来人们最受好评的创新之一,它可以为高通量、无标记、廉价的个性化基因组测序技术铺平道路[44]. 生物纳米孔材料与传统材料相比由于具有使用成本低、分辨率高、操作简单等优点有望成为下一代DNA测序的有力工具. 已报道的研究发现单链RNA和DNA分子在电场作用下可以通过纳米通道以及四种DNA碱基或核苷酸在纳米孔中不同程度地阻碍了离子电流,导致测量电流下降的现象[45-46]. Schneider[47]等也证实了随着电流变化,单个DNA分子会通过纳米孔时迁移. 这些开创性的基础研究为纳米孔相关DNA测序奠定了坚实的基础. 导电性能好、机械强度高的含有纳米级孔的石墨烯可被用于DNA分子的检测[48-49]. Yu[50]课题组采用全原子分子动力学模拟方法研究了四种不同DNA链在功能化石墨烯纳米孔中的易位行为. 结果发现当四种DNA碱DNA碱基通过氢化孔和羟基化孔时,它们可以通过不同的离子电流来识别. 对于氢化纳米孔,四个碱基的离子电流差异主要归因于碱基和离子之间不同的静电相互作用. 对于羟基化纳米孔,除了静电相互作用外,核苷酸在纳米孔内的位置和离子在核苷酸周围的停留时间也对离子电流起重要作用. Prasongkit[51]等提出使用石墨烯中的线缺陷来改善基于纳米孔的DNA测序装置中的核糖核酸酶的选择性. 他们使用量子力学/分子力学和非平衡格林函数相结合的方法来研究电导调制. 通过大量从分子动力学模拟中产生的不同取向的采样研究,从理论上证明了基于石墨烯的电子器件利用线缺陷来区分四个核苷酸酶是可能的. 该研究有助于今后更好地设计一种新的DNA测序装置.3 结语三维多孔石墨烯材料已被证明具有广阔的研究价值. 到目前为止,已有一系列结构不同、功能各异的三维多孔石墨烯被报道. 这些新型材料不仅保留了单个二维石墨烯片的固有特性,而且也探索了多孔石墨烯实际应用的可能性. 但是,多孔石墨烯的可控制备和推广应用方面仍存在不少技术瓶颈,有一些关键问题尚未解决. 未来面对的挑战有:①如何精确控制多孔石墨烯的孔隙形貌;②不同孔隙的协同作用可使多孔石墨烯材料发挥优势,因此如何控制不同的空隙(即微孔、中孔和大孔)组合对多孔石墨烯是至关重要的;③多孔石墨烯制备原料内容单一,目前主要致力于制备具有无机和碳组分的多孔石墨烯. 这些问题在一定程度上限制了其实际应用领域的扩展. 基于当前的研究工作显示,挖掘多孔石墨烯的非凡潜力并不是很难的事情. 随着科学技术的不断发展和探索,合成具有可控孔结构的多功能多孔石墨烯材料必将成为石墨烯材料的研究热点. 而且,多孔石墨烯材料在能量转换和存储设备中各项性能优良,我们相信这些设备的商业化会在不久的将来实现.【相关文献】[1] NOVOSELOV K S,GEIM A K,MOROZOV S V,et al. Electric field effect in atomically thin carbon films[J]. Science,2004,306(5696):666-669.[2] NOVOSELOV K S,GEIM A K,MOROZOV S V,et al.Two-dimensional gas of massless dirac fermions in graphene[J].Nature,2005,438(7065):197.[3]张璐.石墨烯材料的研究进展及应用[J].教育现代化,2018,5(36):275-278.[4] LI X S,CAI W W,AN J H,et rge-area synthesis of high-quality and uniform graphene films on copper foils[J].Science,2009,324:1312-1314.[5] SOAVI G,WANG G,ROSTAMI H,et al. Broadband,electrically tunable third-harmonic generation in graphene[J]. Nature Nano-technology,2018,13:583-588. [6] CAO Y,FATEMI V,FANG S,et al.Unconventional superconductivity in magic-angle graphene superlattices[J].Nature,2018,556(7699):43.[7] LEE C,WEI X,KYSAR J W,et al.Measurement of the elastic properties and intrinsic strength of monolayer graphene[J].Science,2008,321:385-388.[8]许婧,邢悦,郝思嘉,等.石墨烯/聚合物基复合材料3D打印成型研究进展[J].材料工程,2018,46(7):1-11.[9]杨绍斌,籍遥函,沈丁.氧化石墨烯液晶相结构的调控及应用进展[J].化工进展,2019,38(3):1443-1451.[10] ITO Y,TANABE Y,SUGAWARA K,et al. Three-dimensional porous graphene networks expand graphene-based electronic device applications[J].Physical Chemistry Chemical Physics,2018,20(9):6024-6033.[11] STRAUSS V,MARSH K,KOWAL M D,et al. A simple route to porous graphene from carbon nanodots for supercapacitor applications[J].Advanced Materials,2018,30(8):1704449.[12] XU P T,YANG J X,WANG K S,et al.Porous graphene:properties,preparation,and potential applications[J].Chinese Science Bulletin,2012,57(23):2948-2955. [13] CAO X H,YIN Z Y,ZHANG H.Three-dimensional graphene materials:preparation structures and application in supercapacitors[J].Energy Environ Science,2014,7:1850-1865.[14] YU P,BAO R Y,SHI X J,et al. Self-assembled high-strengthhydroxyapatite/graphene oxide/chitosan composite hydrogel for bone tissue engineering [J].Carbohydrate Polymers,2017,155:507-515.[15] YE R,HAN X,KOSYNKIN D V,et al. Laser-induced conversion of teflon intofluorinated nanodiamonds or fluorinated Graphene[J].ACS Nano,2018,12(2):1083-1088.[16] AGUILAR-BOLADOS H,CONTRERAS-CID A,YAZDANI-PEDRAM M,etal.Synthesis of fluorinated graphene oxide by using an easy one-pot deoxyfluorination reaction[J].Journal of Colloid and Interface Science,2018,524:219-226.[17] COMPTON O C,AN Z,PUTZ K W,et al.Additive-free hydrogelation of graphene oxide by ultrasonication[J].Carbon,2012,50(10):3399-3406.[18] LOZADA-HIDALGO M,ZHANG S,HU S,et al. Scalable and efficient separationof hydrogen isotopes using graphene-based electrochemical pumping[J].Nature Communications,2017,8:15215.[19] ABRAHAM J,VASU K S,WILLIAMS C D,et al. Tunable sieving of ions using graphene oxide membranes[J]. Nature Nanotechnology,2017,12(6):546.[20] GAO W.The chemistry of graphene oxide[M]//Graphene oxide.Switzerland:Springer,Cham,2015:61-95.[21] ZHU Y,MURALI S,CAI W,et al.Graphene and graphene oxide:synthesis,properties,and applications[J].Advanced Materials,2010,22(35):3906-3924. [22] KUMAR R,SINGH R K,VAZ A R,et al.Self-assembled and one-step synthesis of interconnected 3D network of Fe3O4/reduced graphene oxide nanosheets hybrid forhigh-performance supercapacitor electrode[J]. ACS Applied Materials & Interfaces,2017,9(10):8880-8890.[23] LI W W,GAO S,WU L,et al.High-density three-dimension graphene macroscopic objects for high-capacity removal of heavy metal ions[J].Scientific Reports,2013,3:2125.[24] TANG C,LI B Q,ZHANG Q,et al. CaO-templated growth of hierarchical porous graphene for high-power lithium-sulfur battery applications[J].Advanced Functional Materials,2016,26(4):577-585.[25] HAN S,WU D Q,LI S,et al.Porous graphene materials for advanced electrochemical energy storage and conversion devices[J].Advanced Materials,2014,26,849-864.[26] HAN Q,YANG L,LIANG Q,et al. Three-dimensional hierarchical porous graphene aerogel for efficient adsorption and preconcentration of chemical warfare agents[J].Carbon,2017,122:556-563.[27] MOON G,SHIN Y,CHOI D,et al.Catalytic templating approaches for three-dimensional hollow carbon/graphene oxide nanoarchitectures[J].Nanoscale,2013,5(14):6291-6296.[28] MAO S,YU K,CHANG J,et al.Direct growth of vertically-oriented graphene for field-effect transistor biosensor[J].Scientific Reports,2013,3:1696.[29] NIU Z,CHEN J,HNG H H,et al.A leavening strategy to prepare reduced graphene oxide foams[J].Advanced Materials,2012,24(30):4144-4150.[30] SIMON P,GOGOTSI Y,DUNN B.Where do batteries end and supercapacitors begin?[J].Science,2014,343(6176):1210-1211.[31]柴雅琼,李章朋,吴尊理,等.多孔石墨烯的制备及应用[J].人工晶体学报,2018,47(3):612-616.[32] QIE L,CHEN W,XU H,et al.Synthesis of functionalized 3D hierarchical porous carbon for high-performance supercapacitors[J].Energy&Environmental Science,2013,6(8):2497-2504.[33] NG S W L,YILMAZ G,ONG W L,et al. One-step activation towards spontaneous etching of hollow and hierarchical porous carbon nanospheres for enhanced pollutant adsorption and energy storage[J].Applied Catalysis B:Environmental,2018,220:533-541.[34]关磊,张力嫱,张宇航,等.三维多孔泡沫石墨烯的制备与应用研究进展[J].合成化学,2018,26(5):383-388.[35] KIM S,LEE Y M.Rigid and microporous polymers for gas separation membranes [J].Progress in Polymer Science,2015,43:1-32.[36] LIN R B,XIANG S,XING H,et al. Exploration of porous metal-organic frameworks for gas separation and purification[J].Coordination Chemistry Reviews,2017.[37] XU Q,XU H,CHEN J,et al.Graphene and graphene oxide:advanced membranes for gas separation and water purification[J].Inorganic Chemistry Frontiers,2015,2(5):417-424.[38] BIERI M,TREIER M,CAI J,et al. Porous graphenes:two-dimensional polymer synthesis with atomic precision[J]. Chemical Communications,2009(45):6919-6921.[39] DIMITRAKAKIS G K,TYLIANAKIS E,FROUDAKIS G E.Pillared graphene:a new 3-D network nanostructure for enhanced hydrogen storage[J].Nano Letters,2008,8(10):3166-3170.[40] KUMAR R,OH J H,KIM H J,et al.Nanohole-structured and palladium-embedded 3D porous graphene for ultrahigh hydrogen storage and CO oxidation multifunctionalities[J].ACS Nano,2015,9(7):7343-7351.[41] DU A,ZHU Z,SMITH S C.Multifunctional porous graphene for nanoelectronics and hydrogen storage:new properties revealed by first principle calculations[J].Journal of the American Chemical Society,2010,132(9):2876-2877.[42] REUNCHAN P,JHI S H. Metal-dispersed porous graphene for hydrogen storage [J]. Applied Physics Letters,2011,98(9):093103.[43] AO Z,DOU S,XU Z,et al.Hydrogen storage in porous graphene with Al decoration[J].International Journal of Hydrogen Energy,2014,39(28):16244-16251.[44]董天宇.DNA测序技术[J].当代化工研究,2018(11):71-73.[45] SCHNEIDER G F,DEKKER C.DNA sequencing with nanopores[J].Nature Biotechnology,2012,30(4):326.[46] WELLS D B,BELKIN M,COMER J,et al.Assessing graphene nanopores for sequencing DNA[J].Nano Letters,2012,12(8):4117-4123.[47] FRIED J P,SWETT J L,BIAN X,et al. Challenges in fabricating graphene nanodevices for electronic DNA sequencing[J].MRS Communications,2018,8(3):703-711.[48]董子杰,张璞,李社红,等.柔性石墨烯平面电极对多巴胺的灵敏检测[J].分析化学,2018(7):1039-1046.[49] SCHNEIDER G F,KOWALCZYK S W,CALADO V E,et al. DNA translocation through graphene nanopores[J]. Nano Lett,2010,10:3163-3167.[50] YU Y,LU X,DING H,et al. Computational investigation on DNA sequencing using functionalized graphene nanopores[J].Physical Chemistry Chemical Physics,2018,20(14):9063-9069.[51] PRASONGKIT J,MARTINS E F,DE SOUZA F A L,et al. Topological line defects around graphene nanopores for DNA sequencing[J].The Journal of Physical Chemistry C,2018,122(13):7094-7099.。
四甲基氢氧化铵转相四氧化三铁

四甲基氢氧化铵转相四氧化三铁四甲基氢氧化铵(TMAOH)是一种常用的阳离子表面活性剂,具有良好的溶解性和表面活性,广泛应用于化学、材料和生物领域。
而四氧化三铁(Fe3O4)是一种重要的磁性材料,具有良好的磁性、导电性和光催化性能,被广泛应用于磁性材料、催化剂和生物医学领域。
本文将探讨四甲基氢氧化铵转相四氧化三铁的合成方法及其应用领域。
一、四甲基氢氧化铵转相四氧化三铁的合成方法1. 水热法合成:将适量的四甲基氢氧化铵溶解在水溶液中,并将其与适量的FeCl2和FeCl3混合,经过水热反应,在一定的温度和压力下反应一段时间后,得到四氧化三铁纳米颗粒。
该方法简单、操作方便,且可以控制合成产物的尺寸和形貌。
2. 沉淀法合成:将四甲基氢氧化铵与FeCl2和FeCl3溶液混合,加入适量的碱溶液,如氢氧化钠(NaOH),在搅拌的条件下,慢慢滴加氨水(NH3·H2O),使溶液pH值升高,产生Fe3O4沉淀。
然后,用无水醇洗涤沉淀,最后干燥得到四氧化三铁。
3. 热分解法合成:将四甲基氢氧化铵与适量的铁盐混合,通过热分解反应,得到四氧化三铁。
该方法可在较高温度下得到纯度较高的四氧化三铁产物,但需要精确控制反应温度和时间。
二、四甲基氢氧化铵转相四氧化三铁的应用领域1. 磁性材料:由于四氧化三铁具有良好的磁性能,可以应用于磁记录材料、磁性流体、磁性储存材料等领域。
将四氧化三铁纳米颗粒与聚合物复合,可以制备出具有优异磁性的磁性纳米复合材料。
2. 催化剂:四氧化三铁具有良好的催化活性和稳定性,可应用于催化剂领域。
将四氧化三铁纳米颗粒与其他催化剂复合,可以制备出具有高效催化性能的复合催化剂,广泛应用于有机合成、环境污染治理等领域。
3. 生物医学:四氧化三铁具有优异的生物相容性和生物活性,可应用于生物医学领域。
将四氧化三铁用于磁共振成像(MRI)、磁导航等技术,可以用于肿瘤诊断、药物传递和热疗等方面。
4. 光催化:四氧化三铁具有良好的光催化性能,可应用于光催化领域。
Fe3O4制备
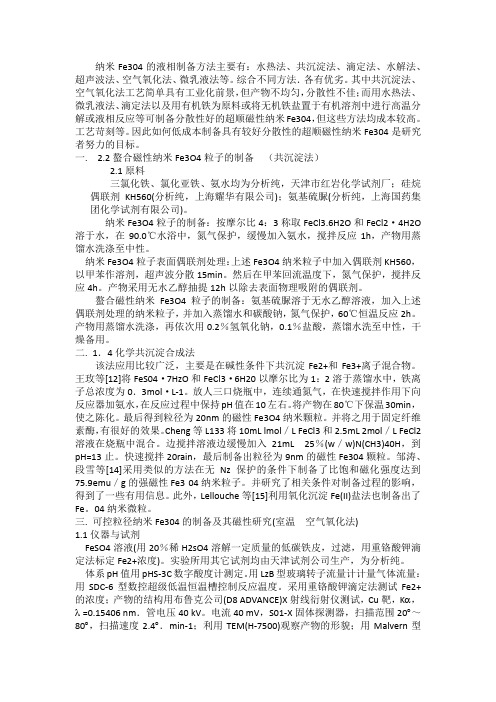
纳米Fe304的液相制备方法主要有:水热法、共沉淀法、滴定法、水解法、超声波法、空气氧化法、微乳液法等。
综合不同方法.各有优劣。
其中共沉淀法、空气氧化法工艺简单具有工业化前景,但产物不均匀,分散性不佳;而用水热法、微乳液法、滴定法以及用有机铁为原料或将无机铁盐置于有机溶剂中进行高温分解或液相反应等可制备分散性好的超顺磁性纳米Fe304,但这些方法均成本较高。
工艺苛刻等。
因此如何低成本制备具有较好分散性的超顺磁性纳米Fe304是研究者努力的目标。
一.2.2螯合磁性纳米Fe3O4粒子的制备(共沉淀法)2.1原料三氯化铁、氯化亚铁、氨水均为分析纯,天津市红岩化学试剂厂;硅烷偶联剂KH560(分析纯,上海耀华有限公司);氨基硫脲(分析纯,上海国药集团化学试剂有限公司)。
纳米Fe3O4粒子的制备:按摩尔比4:3称取FeCl3.6H2O和FeCl2·4H2O 溶于水,在90.0℃水浴中,氮气保护,缓慢加入氨水,搅拌反应1h,产物用蒸馏水洗涤至中性。
纳米Fe3O4粒子表面偶联剂处理:上述Fe3O4纳米粒子中加入偶联剂KH560,以甲苯作溶剂,超声波分散15min。
然后在甲苯回流温度下,氮气保护,搅拌反应4h。
产物采用无水乙醇抽提12h以除去表面物理吸附的偶联剂。
螯合磁性纳米Fe3O4粒子的制备:氨基硫脲溶于无水乙醇溶液,加入上述偶联剂处理的纳米粒子,并加入蒸馏水和碳酸钠,氮气保护,60℃恒温反应2h。
产物用蒸馏水洗涤,再依次用0.2%氢氧化钠,0.1%盐酸,蒸馏水洗至中性,干燥备用。
二.1.4化学共沉淀合成法该法应用比较广泛,主要是在碱性条件下共沉淀Fe2+和Fe3+离子混合物。
王玫等[12]将FeS04·7HzO和FeCl3·6H20以摩尔比为1:2溶于蒸馏水中,铁离子总浓度为0.3mol·L-1。
放人三口烧瓶中,连续通氮气,在快速搅拌作用下向反应器加氨水,在反应过程中保持pH值在10左右。
rGOFe3O4活化过硫酸盐处理酸性红73
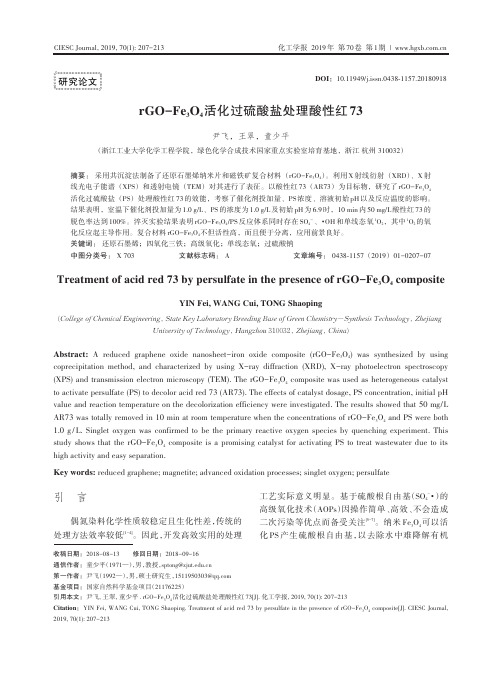
Abstract: A reduced graphene oxide nanosheet-iron oxide composite (rGO-Fe3O4) was synthesized by using coprecipitation method, and characterized by using X-ray diffraction (XRD), X-ray photoelectron spectroscopy (XPS) and transmission electron microscopy (TEM). The rGO-Fe3O4 composite was used as heterogeneous catalyst to activate persulfate (PS) to decolor acid red 73 (AR73). The effects of catalyst dosage, PS concentration, initial pH value and reaction temperature on the decolorization efficiency were investigated. The results showed that 50 mg/L AR73 was totally removed in 10 min at room temperature when the concentrations of rGO-Fe O3 4 and PS were both 1.0 g / L. Singlet oxygen was confirmed to be the primary reactive oxygen species by quenching experiment. This study shows that the rGO-Fe3O4 composite is a promising catalyst for activating PS to treat wastewater due to its high activity and easy separation.
fe3o4纳米颗粒合成
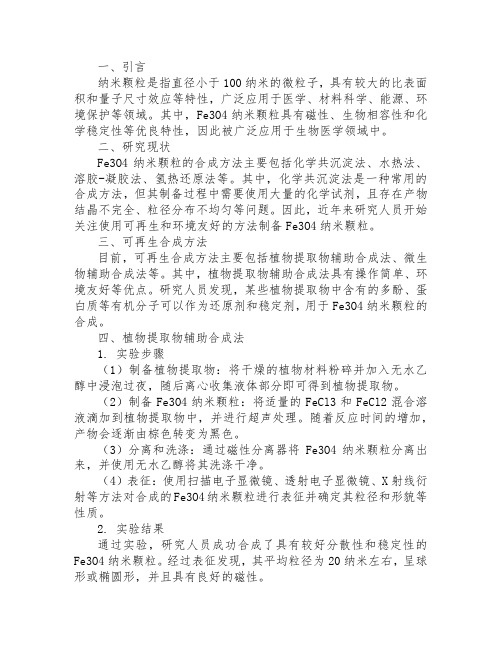
一、引言纳米颗粒是指直径小于100纳米的微粒子,具有较大的比表面积和量子尺寸效应等特性,广泛应用于医学、材料科学、能源、环境保护等领域。
其中,Fe3O4纳米颗粒具有磁性、生物相容性和化学稳定性等优良特性,因此被广泛应用于生物医学领域中。
二、研究现状Fe3O4纳米颗粒的合成方法主要包括化学共沉淀法、水热法、溶胶-凝胶法、氢热还原法等。
其中,化学共沉淀法是一种常用的合成方法,但其制备过程中需要使用大量的化学试剂,且存在产物结晶不完全、粒径分布不均匀等问题。
因此,近年来研究人员开始关注使用可再生和环境友好的方法制备Fe3O4纳米颗粒。
三、可再生合成方法目前,可再生合成方法主要包括植物提取物辅助合成法、微生物辅助合成法等。
其中,植物提取物辅助合成法具有操作简单、环境友好等优点。
研究人员发现,某些植物提取物中含有的多酚、蛋白质等有机分子可以作为还原剂和稳定剂,用于Fe3O4纳米颗粒的合成。
四、植物提取物辅助合成法1. 实验步骤(1)制备植物提取物:将干燥的植物材料粉碎并加入无水乙醇中浸泡过夜,随后离心收集液体部分即可得到植物提取物。
(2)制备Fe3O4纳米颗粒:将适量的FeCl3和FeCl2混合溶液滴加到植物提取物中,并进行超声处理。
随着反应时间的增加,产物会逐渐由棕色转变为黑色。
(3)分离和洗涤:通过磁性分离器将Fe3O4纳米颗粒分离出来,并使用无水乙醇将其洗涤干净。
(4)表征:使用扫描电子显微镜、透射电子显微镜、X射线衍射等方法对合成的Fe3O4纳米颗粒进行表征并确定其粒径和形貌等性质。
2. 实验结果通过实验,研究人员成功合成了具有较好分散性和稳定性的Fe3O4纳米颗粒。
经过表征发现,其平均粒径为20纳米左右,呈球形或椭圆形,并且具有良好的磁性。
五、结论通过植物提取物辅助合成法,可以制备出具有良好分散性和稳定性的Fe3O4纳米颗粒。
该方法具有操作简单、环境友好等优点,并有望应用于生物医学等领域中。
但是,目前该方法仍面临一些问题,如产物粒径分布不均匀、反应时间长等,需要进一步改进和优化。
基于共价键作用的四氧化三铁-还原氧化石墨烯复合材料的合成及其储锂性能
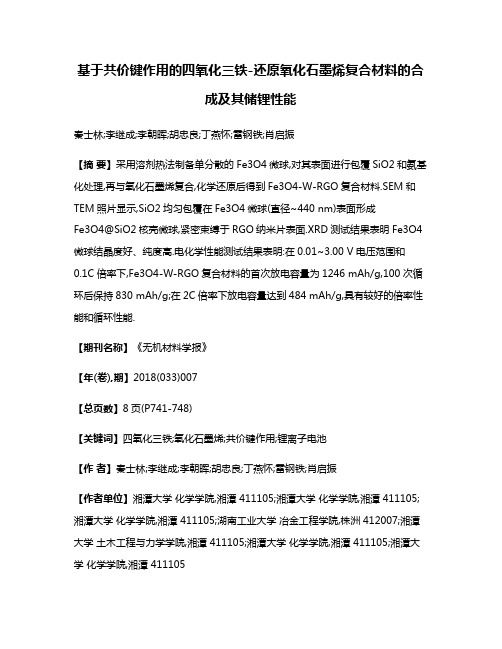
基于共价键作用的四氧化三铁-还原氧化石墨烯复合材料的合成及其储锂性能秦士林;李继成;李朝晖;胡忠良;丁燕怀;雷钢铁;肖启振【摘要】采用溶剂热法制备单分散的Fe3O4微球,对其表面进行包覆SiO2和氨基化处理,再与氧化石墨烯复合,化学还原后得到Fe3O4-W-RGO复合材料.SEM和TEM照片显示,SiO2均匀包覆在Fe3O4微球(直径~440 nm)表面形成Fe3O4@SiO2核壳微球,紧密束缚于RGO纳米片表面.XRD测试结果表明Fe3O4微球结晶度好、纯度高.电化学性能测试结果表明:在0.01~3.00 V电压范围和0.1C倍率下,Fe3O4-W-RGO复合材料的首次放电容量为1246 mAh/g,100次循环后保持830 mAh/g;在2C倍率下放电容量达到484 mAh/g,具有较好的倍率性能和循环性能.【期刊名称】《无机材料学报》【年(卷),期】2018(033)007【总页数】8页(P741-748)【关键词】四氧化三铁;氧化石墨烯;共价键作用;锂离子电池【作者】秦士林;李继成;李朝晖;胡忠良;丁燕怀;雷钢铁;肖启振【作者单位】湘潭大学化学学院,湘潭 411105;湘潭大学化学学院,湘潭 411105;湘潭大学化学学院,湘潭 411105;湖南工业大学冶金工程学院,株洲 412007;湘潭大学土木工程与力学学院,湘潭 411105;湘潭大学化学学院,湘潭 411105;湘潭大学化学学院,湘潭 411105【正文语种】中文【中图分类】TQ174锂离子电池由于能量密度高, 已经在便携式电子设备上得到了广泛应用。
碳基负极材料(石墨)的比容量一般低于400 mAh/g[1], 基于化学转化反应机理的过渡金属化合物负极材料具有比碳材料更高的比容量[2-7]。
例如, 在0.01~3.00 V(vs.Li+/Li)电压范围和0.1 A/g电流密度下, MoS2/石墨烯复合材料的比容量为800 mAh/g[7], SnO2为609 mAh/g[8], V2O3/C复合材料为774.8 mAh/g[9], h-MoO3@C纳米纤维为946.6 mAh/g[10]。
fe3o4纳米微球的溶剂热法控制合成

fe3o4纳米微球的溶剂热法控制合成fe3o4是一种重要的可溶性无机纳米材料,由于具有优异的磁性和光学性能,因此在生化探测、储能器件、仿生药物等方面受到极大关注。
然而,传统的热溶解法一般是以大量溶剂的形式进行合成,并存在反应不适宜。
同时,由于大量的溶剂的使用,研究者也面临着排放和处理溶剂的挑战。
为了克服上述问题,研究人员提出了一种基于溶剂热法控制反应(Solvent-thermal-controlled reaction,STCR)新方法来合成Fe3O4纳米微球。
该方法可降低储能耗费,减少溶剂使用量以及促进反应的速率。
的工作原理是,当溶剂热量达到反应的一定温度和压力时,就可以促进Fe3O4纳米微球的反应和形成。
STCR从原理上讲,它是一种单步溶剂热处理反应,无需加入额外的可混合液体的额外反应溶剂,以促进纳米物质的合成。
这种方法可以减少反应要素的使用,并且可以节省大量的储能资源。
另外,STCR 也可以改善反应环境,减少反应杂质,并有效抑制氧化反应。
STCR技术的应用可以有效地控制Fe3O4纳米微球的形成,使其具有优异的磁性和光学性能。
研究工作表明,用STCR生产的Fe3O4纳米微球具有良好的磁性和光学特性,同时也具有优异的稳定性和耐久性。
另外,STCR技术的应用还可以解决Fe3O4纳米微球的多晶结构问题。
在常规反应溶剂中,由于氧化物的形成不易被沉淀,因此Fe3O4纳米微球的多晶结构很难控制。
但是使用STCR技术,反应溶剂可以被控制住,因此有助于避免Fe3O4纳米微球的多晶结构问题。
通过以上简述,可以得出结论,STCR技术在Fe3O4纳米微球的合成中具有重要的意义。
它不仅能够有效地改善反应环境,减少消耗的储能资源,而且能够控制Fe3O4纳米微球的形成,使其具有优异的磁性和光学特性,同时也具有优异的稳定性和耐久性。
然而,STCR技术也存在一些局限性。
它的反应能量消耗较高,因此只能用于少量生产,同时由于它对反应温度和压力的要求较高,因此反应过程比较复杂。
总结氨基化二氧化硅包四氧化三铁80nm,10种常见制备Fe3O4的方法

总结氨基化二氧化硅包四氧化三铁80nm,10种常见制备Fe3O4的方法今天小编瑞禧整理并分享关于总结氨基化二氧化硅包四氧化三铁80nm,10种常见制备Fe3O4的方法:制备四氧化三铁(Fe3O4)纳米颗粒的方法多样,下面列举了一些常见的制备方法:共沉淀法:将Fe2+和Fe3+离子在碱性溶液中共沉淀,然后还原得到Fe3O4纳米颗粒。
水热法:在高温高压水热条件下,将铁盐与还原剂在水中反应,形成Fe3O4纳米颗粒。
溶剂热法:将有机溶剂中的铁源与还原剂反应,生成Fe3O4纳米颗粒,之后纳米颗粒从溶剂中分离出来。
热分解法:将含有铁的有机化合物在高温下分解,生成Fe3O4纳米颗粒。
共溶剂热法:将铁源和有机溶剂一起加热,在高温下反应生成Fe3O4纳米颗粒。
微乳法:在水/油的微乳体系中,通过化学反应得到Fe3O4纳米颗粒,微乳体系提供了适合纳米颗粒生长的小空间环境。
胶体化学合成法:利用胶体化学方法,通过在溶液中控制铁离子的核生成和生长,形成Fe3O4纳米颗粒。
电化学法:利用电化学沉积或电化学氧化还原反应,在电极上制备Fe3O4纳米颗粒。
微波辅助法:在微波辐射下,通过化学反应合成Fe3O4纳米颗粒,微波加速了反应速度。
激光烧结法:利用激光烧结技术,将铁粉或铁化合物激光加热到高温,形成Fe3O4纳米颗粒。
纳米材料因其特别的物理、化学和生物性质,在生物医学、环境监测、信息科技等领域中得到广应用。
四氧化三铁(Fe304)因其良好的磁性、合成简便和生物相容性等特点,成为了研究和应用的热点材料之一。
尤其是超顺磁性Fe3O4纳米粒子,因其良好的磁响应性能和分散性,成为了药物传输、生物成像的理想载体。
在生物医学中,纳米粒子的应用需要满足一定的生物相容性和稳定性。
因此,表面修饰成为了超顺磁性Fe3O4纳米粒子应用研究中的重要环节。
以上来源于文献整理,如有侵权,请联系删除,RL2023.10。
- 1、下载文档前请自行甄别文档内容的完整性,平台不提供额外的编辑、内容补充、找答案等附加服务。
- 2、"仅部分预览"的文档,不可在线预览部分如存在完整性等问题,可反馈申请退款(可完整预览的文档不适用该条件!)。
- 3、如文档侵犯您的权益,请联系客服反馈,我们会尽快为您处理(人工客服工作时间:9:00-18:30)。
Chem. Res. Chin. Univ., 2015, 31(4), 508―513doi: 10.1007/s40242-015-4487-6———————————*Corresponding author. E-mail: zhouwei@ Received December 15, 2014; accepted April 8, 2015.Supported by the National Natural Science Foundation of China(Nos.51438011, 51102005), the Foundation for the Author of National Excellent Doctoral Dissertation of China(No.201331) and the Program for New Century Excellent Talents in University, China(No.NCET-13-0032).© Jilin University, The Editorial Department of Chemical Research in Chinese Universities and Springer-Verlag GmbHOne-pot Hydrothermal Synthesis of rGO-Fe 3O 4 HybridNanocomposite for Removal of Pb(II) viaMagnetic SeparationCAO Wei, MA Yiran, ZHOU Wei * and GUO LinSchool of Chemistry and Environment, Beihang University, Beijing 100191, P. R. ChinaAbstract The reduced graphene oxide-Fe 3O 4(rGO-Fe 3O 4) hybrid nanocomposite was prepared via a one-pot facile hydrothermal method for adsorption of heavy metal ions. The results of compositional and morphological characteri-zations show that the Fe 3O 4 NPs with an average diameter of 20 nm have been uniformly dispersed in rGO sheets. Due to the higher specific surface area of rGO and the magnetic properties of Fe 3O 4 nanoparticles, the prepared rGO-Fe 3O 4 hybrid nanosheets showed good adsorption capacity for the removal of Pb(II) from wastewater by simple magnetic separation. The result of control tests show that the adsorption capacity of rGO-Fe 3O 4 can be influenced by the ratio of ferric chloride(FeCl 3) to graphene oxide(GO) during the process of sample preparation and the initial concentration of Pb(II). A better adsorption capacity was 30.68 mg/g at n (FeCl 3)/m (GO) ratio of 1:5(mmol:mg) at pH=7.0 with the initial concentration of Pb(II) ions of 80 mg/L, and the experimental data were well fitted with the Langmuir adsorption model. The composite with absorbed Pb(II) can be easily collected by magnetic separation from wastewater because of the excellent magnetism of Fe 3O 4 NPs. The rGO-Fe 3O 4 hybrid nanocomposite provides an ef-fective and environment-friendly absorbent with great application potential in water purification. Keywords Graphene; Nanocomposite; Adsorption; Magnetic separation1 IntroductionEnvironmental contamination has become an urgent issue nowadays, especially the pollution of heavy metal ions from metal plating, alloy manufacturing, metallurgy, chemical ma- nufacturing and mining and battery industries. Heavy metal ions are easy to accumulate in living organisms but hard to biodegrade, which can cause mass mortality of aquatic lives and cancers even death of human beings. Tremendous efforts have been devoted to degrading heavy metal ions with various methods including chemical precipitation [1], adsorption [2], membrane filtration [3] and photocatalytic degradation [4], etc. Among these methods, adsorption is a widely used traditional method because of its high efficiency, low cost, ease of opera-tion and regeneration [5]. And various adsorbents, such as silica gel [6], activated alumina [7], fly ash [8], coconut shell [9], sugarcane bagasse [10] and many kinds of carbon materials have been deve- loped.As a new type of two-dimensional carbon-based nano- material with one-atom thickness, graphene arouses global attention because of its superior performances in many fields. Due to its large specific surface area(2630 m 2/g)[11], graphene has been considered as an ideal absorbent for water purifica- tion. However, graphene composing of carbon atoms only haspoorly solubility in water, thus graphene oxide(GO) and re-duced graphene oxide(rGO) with oxygen-groups on their sur-faces are used as adsorbents instead. But the separation was rather difficult when GO or rGO sheets were used as their light-weight thin layers [12]. Therefore, magnetic NPs could be introduced to form (r)GO-magnetic NPs hybrid adsorbents and simple magnetic separation could be achieved.Magnetite(Fe 3O 4) is an excellent ferromagnetic material with high saturation magnetization and good biocompatibili-ty [13] and has been used as added components of adsorbents for magnetic separation with an external magnet and without cen-trifugation or filtration. There are lots of ways to synthesize Fe 3O 4 nanoparticles with different sizes and morpholo-gies [14―16]. For the above reasons, rGO-Fe 3O 4 would be an ideal absorbent combining the merits of adsorption properties of rGO with the easy separation of magnetic nanoparticles.In this work, we presented a one-pot facile method to produce the rGO-Fe 3O 4 hybrid nanocomposite for the removal of Pb(II) ions. The Fe 3O 4 nanoparticles with an average diame-ter of 20 nm were loaded on the both sides of rGO sheets through in situ reduction of FeCl 3. The adsorption of Pb(II) ions and the magnetic separation were carried out to confirm the good adsorption capacity and the easy magnetic separation of hybrid sheets.No.4CAO Wei et al.5092 Experimental2.1 MaterialsAll the chemicals were analytical grade and used without further purification. The ultrapure water(Milli-Q) was used throughout the adsorption experiments.2.2 Synthesis of GOGO was prepared from natural graphite powder(325 meshes) via a modified Hummers method [17]. In brief, 15 mL of concentrated sulfuric acid was mixed with 3.0 g of graphite powder, 2.5 g of K 2S 2O 8 and 2.5 g of P 2O 5. The mixture was kept stirring for 6 h at 80 °C, and then poured into 500 mL of deionized water under stirring. After centrifugation and drying, the black product was mixed with 115 mL of concentrated sul-furic in an ice bath. After that, 15 g of KMnO 4 was slowly added and the mixture was stirred for 2 h at 35 °C. Deionized water of 250 mL was added to the mixture and the solution was stirred for another 2 h at 20 °C. An additional of 700 mL of deionized water was added, followed by 10 mL of H 2O 2(30%, mass fraction) added. Finally, the mixture was centrifuged and washed with HCl(10%, mass fraction) and deionized water, respectively, until the solution was neutral. The condensed GO aqueous solution, which could remain stable for several months, was stored for further use.2.3 Synthesis of rGO-Fe 3O 4 NanocompositeThe rGO-Fe 3O 4 hybrid nanocomposite was prepared through one-pot hydrothermal method. In a typical synthesis process, 3 mmol of FeCl 3 was dissolved in 20 mL of ethylene glycol(EG) and sonicated for 30 min, followed by 1 mL of hydrazine(80%, mass fraction) added under stirring. Subse-quently, 10 mL of GO(1.50 mg/mL) was dispersed in the solu-tion under sonication. Finally, 10 mmol of urea was added un-der continuous stirring. The mixture was then transferred into a 40 mL Teflon-lined stainless steel autoclave at 180 °C and kept for 10 h. Then the autoclave was cooled to room temperature. The obtained black product was washed with deionized water and ethanol, separately, several times and dried at 60 °C in an oven. Fe 3O 4 NPs were synthesized in the same procedure without adding GO for comparison.2.4 CharacterizationThe morphology and structure of the hybrid composite were studied by means of scanning electron microscopy(SEM, Quanta 250 FEG )and transmission electron microscopy(TEM, JEOL JEM-2100F). TEM specimens were obtained by dipping carbon-capped copper grids into the solution. The structure and composition of the as-prepared product was characterized on a Rigaku Dmax2200 X-ray diffractometer with Cu K α radia-tion(λ=0.15416 nm). The XRD specimen was prepared by flat-tening the powder on small slides. The magnetic measurement was performed on an MPMS-XL SQUID magnetometer at 300 K. Raman spectroscopy measurements were performed on aLabRAM HR800 system with an excitation wavelength of 633 nm and the silicon peak at 520 cm –1 as a reference. Fourier transform infrared(FTIR) spectra were obtained on a Thermo Nicolet iN10MX instrument. The adsorption experiment was performed in a water bath oscillator(SHZ-82A), and the con-centration of heavy metal ions in the aqueous solution was analyzed via an inductively coupled plasma optical emission spectrometer(ICP-OES, Agilent 710).2.5 Adsorption ExperimentsThe adsorption experiments were carried out by adding 40 mg of the rGO-Fe 3O 4 nanocomposite to the Pb(II) aqua-solutions with different initial concentrations in 100 mL conical flasks. The initial concentrations varied from 20 mg/L to 100 mg/L while the pH maintained at 7.0. The mixture was put on a shaking table at room temperature and shaken for 10 h. After reaching the equilibrium, the adsorbent was separated from the solution via a permanent magnet. The equilibrium concentration of Pb(II) in the supernatant was analyzed by means of ICP-OES. The amount of Pb(II) adsorbed by rGO-Fe 3O 4 was revealed as different values between the initial and the equilibrium concentrations of Pb(II). The adsorption capacity of adsorbent for Pb(II)(q e , mg/g) was calculated accor- ding to the following equation:q e =(c i –c e )V /m (1)where c i and c e are the initial and the final concentrations of Pb(II)(mg/L), respectively; V is the volume of Pb(II) solu-tion(mL); m is the mass of the absorbent(mg).3 Results and DiscussionThe morphology of the rGO-Fe 3O 4 hybrid nanocomposite was studied by means of SEM and TEM. Fig.1(A) and (B) display the low and high magnified SEM images of the sample, respectively, which show that NPs are well dispersed on the 2D crumpled rGO sheets because rGO sheets could prevent the Fe 3O 4 NPs from aggregation. TEM image in Fig.1(C) also shows that NPs with an average diameter of 20 nm have beenFig.1 Low(A) and high(B) magnification SEMimages, TEM(C) and HRTEM(D) images of the rGO-Fe 3O 4 hybrid composite(D) corresponds to the marked frame in (C).510Chem. Res. Chin. Univ.Vol.31loaded on the thin layers. The HRTEM image in Fig.1(D) cor-responding to the marked frame in Fig.1(C), shows the particle with a lattice spacing of 0.24 nm, which could be ascribed to the (222) planes of Fe 3O 4.Fig.2(A) shows the XRD pattern of the rGO-Fe 3O 4 hybrid nanocomposite. GO has a strong peak at 2θ=10.1°, corres-ponding to the (001) plane with interplanar spacing of 0.87nm [18], which couldn’t be observed here. Instead, fromFig.2(A), the broad peak around 2θ=24.6° marked with asterisk shows up, indicating GO has been reduced to rGO [19]. The diffraction peaks of the as-synthesized product at 2θ=18.5°, 30.3°, 35.8°, 37.4°, 43.5°, 53.9°, 57.5°, 63.1°, 71.7° and 74.7° can be assigned to the crystalline planes of (111), (220), (311), (222), (400), (422), (511), (440), (620) and (533) of Fe 3O 4, in agreement with the component of Fe 3O 4(JCPDS No.75-0449).Fig.2 XRD patern(A), FTIR spectra(B) and Ramanspectrum(C) of rGO-Fe 3O 4 nanocomposite(B) a . rGO-Fe 3O 4; b . Fe 3O 4; c . GO.FTIR spectra of GO, Fe 3O 4 and rGO-Fe 3O 4 nanocompo-sites are shown in Fig.2(B). The spectrum of GO shows O ―H stretching vibration in the region of 3000―3400 cm –1, C =O(carbonyl and carboxylic groups) stretching vibration at 1735 cm –1, C =C stretching peak(unoxidized sp 2 C hybridiza-tion) at 1610 cm –1, O ―H bend vibration at the peaks at 1406 cm –1 as well as C ―O(epoxy), C ―O(alkoxy) stretching and C ―O(epoxy) bend vibrations at 1232, 1048 and 870 cm –1,respectively [20]. For Fe 3O 4, the peak at 572 cm –1 is a characte-ristic peak corresponding to the stretching vibration of Fe ―O [21]. While rGO-Fe 3O 4 shows two peaks at 1526 and 1192 cm –1, which correspond to the aromatic C =C stretch and C ―O stretch, respectively, indicating the successful reduction of GO to rGO. Also, the band intensity of Fe ―O vibration increases and shifts, indicating the formation of Fe ―O bond between rGO and Fe 3O 4, and a high iron loading in rGO-Fe 3O 4.Raman spectroscopy has been proved to be an essential tool to study the ordered/disordered crystal structures of carbo-naceous materials. The structural change of GO to rGO during the reaction was further investigated by Raman spectroscopy. As shown in Fig.2(C), two prominent peaks at 1330 and 1590 cm –1 correspond to the well documented D band and G band, respectively. Usually, G band can be assigned to the E 2g phonon of sp 2 bonds of carbon atoms, and D band is associated with local defects and disorders, especially the defects located at the edges. Thus, the intensity ratio of D band to G band(I D /I G ) is usually used as a measure of the disorder. For GO, the intensity of G band is higher than that of D band with I D /I G <1, while opposite result could be got after reduction [22]. As for our rGO-Fe 3O 4 composite, the ratio of I D /I G was 1.83, indicating the reduction of GO to rGO after the reaction process.Fig.3(A) and (B) show the magnetization properties of the rGO-Fe 3O 4 nanocomposite and pure Fe 3O 4 NPs under applied magnetic field ranging from –2 T to 2 T at 300 K, respectively. Fe 3O 4 NPs were synthesized following the preparation proce-dure of rGO-Fe 3O 4 composite without adding GO. The mor-phology of Fe 3O 4 NPs is shown in Fig.3(C).The values of saturation magnetization(M s ) of Fe 3O 4 NPs and rGO-Fe 3O 4 composite are 68.03 and 60.00 A·m 2/kg, re-spectively. The small decrement of the saturation magnetization of the rGO-Fe 3O 4 composite might be due to the lessened quan-tity of Fe 3O 4 in the composites. That is, the rGO sheets have no obvious effect on the magnetism of the loaded Fe 3O 4 NPs. Be-sides, the value of M s for the as-synthesized composite is higher than those of other rGO-Fe 3O 4 nanocomposites reported [23,24], which might depend on the loading amount of Fe 3O 4 NPs on rGO sheets.When the rGO-Fe 3O 4 composite is used as the adsorbent for heavy metal ions, it is considered the most adsorption ca-pacity comes from rGO while Fe 3O 4 mainly provides magne- tism. The adsorption capacity can be improved by adding more GO in the reaction system, thus a series of composites with different ratios of n (FeCl 3):m (GO) was synthesized. But the data shown in Fig.4 reveal an opposite result. At the same initial concentrations of 80 mg/L of Pb(II), the adsorption of Pb(II) can be affected by the ratio of the two substances. When the ratio of n (FeCl 3):m (GO) is 1:5(mmol:mg), the adsorption capacity of the composite reaches the maximum value of 30.68 mg/g, while a higher and a lower ratio of n (FeCl 3) to m (GO) can both lead to a decrement of adsorption capacity. The phe-nomenon could be attributed to the Fe 3O 4 NPs anchored on rGO, which keeps layered rGO from stacking, and thus gives the composite smaller adsorption surfaces and active sites. The composites can stack easily and can form thick layers with lower specific surface areas when more GO sheets are addedNo.4 CAO Wei et al.511 Fig.3 Magnetization curves of Fe3O4(a) and rGO-Fe3O4(b) nanocomposites at 300 K(A), theenlarged partial magnetization curve ofrGO-Fe3O4 composites(B) and SEM image ofpure Fe3O4 NPs(C)Fig.4 Adsorption of Pb(II) on a series of rGO-Fe3O4nanocomposites prepared by adding differentratios of FeCl3 and GOExperiment conditions: initial Pb(II) concentration: 80 mg/L; pH=7.0;temperature: (25±2) °C; contact time: 10 h.during the composite preparation. While at higher ratios ofFeCl3, serious agglomerations of Fe3O4 NPs occur, and also dothe too lower content of rGO as main adsorbent, which can leadto a lower adsorption capacity. Thus, a ratio of n(FeCl3):m(GO)of 1:5(mmol:mg) matches best to obtain the maximum adsorp-tion. That is, Fe3O4 NPs provide magnetic properties and areused as structure stabilizer for the hybrid nanocomposite.The adsorption isotherm of Pb(II) on the rGO-Fe3O4composite with n(FeCl3):m(GO) ratio of 1:5(mmol:mg) isshown in Fig.5. The initial concentrations of Pb(II) ions rangefrom 20 mg/L to 100 mg/L. It is clearly observed that the Pb(II)adsorption increases rapidly with the initial Pb(II) concentra-tions less than 80 mg/L. At an initial concentration(c0) of 80mg/L, adsorption capacity reaches a maximum of 30.68 mg/g.The inset of Fig.5 shows the digital photos of the magneticresponse of the nanocomposites towards an exterior magneticfield. It can be seen that the rGO-Fe3O4 composite after ad-sorbing Pb(II) has been quickly separated from the solution bya magnet. It is essentially important for the convenient recy-cling of the rGO-Fe3O4 nanocomposite.Fig.5 Adsorption isotherm of Pb(II) on therGO-Fe3O4 hybrid compositeExperiment conditions: pH=7.0, temperature: (25±2) °C, contact time: 10 h.Insect photos of the rGO-Fe3O4 composite responding to a magnet in Pb(II)solution before(left) and after(right) adsorption.The adsorption capacities of various adsorbents for theremoval of Pb(II) ions are summarized in Table 1. Comparedwith activated carbon[25], CNTs[26] or graphene[27], the as pre-pared rGO-Fe3O4 shows higher adsorption capacity because theFe3O4 NPs can prevent the composite sheets from stacking(providing more specific surface area) and more oxygen-containing functional groups on the surfaces(providing moreadsorption sites via electrostatistic interaction for positivecharge heavy metal ions). However, compared to those adsor-bents, such as Fe3O4-poly(L-cysteine/2-hydroxyethyl acry-late)[28], thiol-functionalized CNTs/Fe3O4[29] and Fe3O4/cyclo-dextrin polymer[30], the adsorption capacity of our product isrelative lower. It can be ascribed to those functionalized adsor-bents with much more functional groups, which can bind moreheavy metal ions. But the synthesizing procedures of theabove adsorbents are more comparable with that of ourone-pot hydrothermal method. Obviously, the organicTable 1 Comparison of adsorption capacities of dif-ferent adsorbents for the removal of Pb(II)Type of adsorbentAdsorption capacityof Pb(II) /(mg·g–1)ReferencePowdered activated carbon 26.90 [25]Multiwalled carbon nanotubes 3.00 [26]Magnetic graphene 6.00 [27]Fe3O4-poly(L-cysteine/2-hydroxyethyl acrylate)38.69 [28]Thiol-functionalized CNTs/Fe3O465.40 [29]Fe3O4/cyclo-dextrin polymer 64.50 [30]512 Chem. Res. Chin. Univ. Vol.31molecules-functionalized materials provide higher adsorptioncapacity, which would be the key point of our further work.The experimental data for the Pb(II) adsorption on therGO-Fe 3O 4 composite were further analyzed via the Langmuir and Freundlich adsorption isotherm models. The equations ofthese two isotherm models are displayed in Eqs.(2) and (3), respectively.e m e e L m11c q c q K q =+ (2) e F e lg lg 1/lg q K n c =+ (3)where c e is the equilibrium concentration of Pb(II)(mg/L), q e isthe adsorption capacity of Pb(II) on adsorbent at equilibrium state(mg/g), q m is the maximum adsorption capacity(mg/g), K L is the Langmuir binding constant, which is related to the energy of adsorption [31]. K F and n are Freundlich constants that are related to adsorption energy and adsorption intensity, respec-tively. The Langmuir isotherm is based on the assumptions that the sorption is a monolayer coverage, the surface of the absor-bent is homogenous, on which can only one adsorbed atom be accommodated, and every adsorption site has equal adsorbate affinity and is independent with its neighboring sites [32]. Freun-dlich model is an empirical equation that is applicable to highly heterogeneous surfaces [31].Fig.6 showed the Langmuir and Freundlich isotherms for the adsorption of Pb(II) ions on the rGO-Fe 3O 4 composite. The results show that the R 2 value for the adsorption of Langmuir and Freundlich isotherm models are 0.998 and 0.918, respec-tively, indicating the adsorption behavior fitted to the Langmuir isotherm model well. In addition, the value of the q max obtained from the Langmuir model has good agreement with the expe-rimental data(30.68 mg/g). The results reveal that the adsorp-tion process is mainly a monolayer adsorption, that is, aFig.6 Langmuir(A) and Freundlich(B) isothermmodels for the adsorption of Pb(II) ions on the rGO-Fe 3O 4 nanocomposite larger specific surface area would lead to a better adsorption. From the Langmuir isotherm model, the essential charac-teristics can be expressed by a separation factor, R L , which can be obtained from the following equation: L L e11R K c =+ (4)where c e is the equilibrium concentration of Pb(II)(mg/L), K L is the Langmuir binding constant, which is related to the energy of adsorption. The value of R L represents the shape of the Langmuir isotherm and the nature of the adsorption process, which is considered to be a favorable one when the value is within the range of 0―1[33]. In this work, the calculated value of the R L is in the range of 0.466―0.911, which proves that the adsorption of Pb(II) by the rGO-Fe 3O 4 composite is favorable.4 ConclusionsThe rGO-Fe 3O 4 hybrid nanocomposite was synthesized via a one-pot facile hydrothermal method. The results of the SEM, TEM and XRD characterizations of the composite show that approximately spherical Fe 3O 4 nanoparticles with about 20 nm in diameter have been deposited on the surfaces of rGO sheets. The adsorption experiments of Pb(II) ions on the rGO-Fe 3O 4 composite show that the adsorption capacity was influenced by the amount of Fe 3O 4 nanoparticles in the hybrid material, which could prevent rGO sheets from stacking. The adsorption capacity was also related to the initial concentration of Pb(II). At low concentration, adsorption capacity was proportional to the initial concentration and reached a maxi-mum value at a concentration of 80 mg/L. The maximum adsorption capacity was 30.68 mg/g, which was obtained at n (FeCl 3)/m (GO) ratio of 1:5(mmol:mg) at pH=7.0. The data fitted well with the Langmuir model. Besides, the magnetic nanocomposite after adsorbing Pb(II) ions could be easily se-parated under a magnet. The results showed that the simply prepared rGO-Fe 3O 4 hybrid nanocomposite was an effective and environment friendly absorbent in water purification.References[1] Blowes D. W., Ptacek C. J., Jambor J. L., Environ. Sci. Technol.,1997, 31(12), 3348[2] Babel S., Kurniawan T. A., J. Hazard. Mater., 2003, 97, 219[3] Gao H. N., Sun Y . M., Zhou J. J., Xu R., Duan H. W., ACS Appl.Mater. Interfaces , 2013, 5, 425[4] Fu F. L., Wang Q., J. Environ. Manag., 2011, 92, 407[5] Aguado J., Arsuaga J. M., Arencibia A., Lindo M., Gascón V ., J. Ha-zard. Mater., 2009, 163, 213[6] Gupta V . K., Carrott P. J. M., Ribeiro Carrott M. M. L., Suhas, Crit.Rev. Env. Sci. Tec., 2009, 39, 783[7] Parida S. K., Dash S., Patel S., Mishra B. K., Adv. Colloid InterfaceSci., 2006, 121, 77[8] Lin T. F., Wu J. K., Water Res., 2001, 35, 2049[9] An H. K., Park B. Y ., Kim D. S., Water Res., 2001, 35, 3551 [10] Amuda O. S., Giwa A. A., Bello I. A., Biochem. Eng. J., 2007, 36,174[11] Zhou Y ., Jiang L., Yan J. W., Wang C. L., Xiao N., Chem. J. ChineseUniversities , 2014, 35(3), 619No.4 CAO Wei et al. 513[12]Paredes J. I., Rodil S. V., Alonso A. M., Tascon J. M. D., Langmuir,2008, 24, 10560[13]Huang C. C., Bai H., Li C., Shi J. Q., Chem. Commun., 2011, 47,4962[14]Hua M., Zhang S. J., Pan B. C., Zhang W. M., Lü L., Zhang Q. X., J.Hazard. Mater., 2012, 211, 317[15]Li F., Du X. Y., Yang R. C., Chem. J. Chinese Universities, 2011,32(8), 1688[16]Zubir N. A., Yacou C., Motuzas J., Zhang X. W., Diniz da Costa J. C.,Sci. Rep., 2014, 4, 4594[17]Hummers J. W. S., Offeman R. E., J. Am. Chem. Soc., 1958, 80, 1339[18]Xu C., Wang X., Small, 2009, 5, 2212[19]Zhang W. X., Cui J. C., Tao C. A., Wu Y. G., Li Z. P., Ma L., Wen Y.Q., Li G. T., Angew. Chem. Int. Ed., 2009, 48, 5864[20]Tian Y., Yu B. B., Li X., Li K., J. Mater. Chem., 2011, 21, 2476[21]Thomas H. R., Marsden A. J., Walker M., Wilson N. R., Rourke J. P.,Angew. Chem. Int. Ed., 2014, 53, 7613[22]Chandra V., Park J., Chun Y., Lee J. W., Hwang I. C., Kim K. S., ACSNano, 2010, 4(7), 3979[23]Zhang Y., Chen B., Zhang L. M., Huang J., Chen F. H., Yang Z. P.,Yao J. L., Zhang Z. J., Nanoscale, 2011, 3, 1446 [24]Yang X., Chen C. L., Li J. X., Zhao G. X., Ren X. M., Wang X. K.,RSC Adv., 2012, 2, 8821[25]Reddad Z., Gerente C., Andres Y., Cloirec P. L., Environ. Sci. Tech-nol., 2002, 36(9), 2067[26]Abraham T. N., Kumar R., Misra R. K., Jain S. K., J. Appl. Polym.Sci., 2012, 125, 670[27]Gollavelli G., Chang C. C., Ling Y. C., ACS Sustainable Chem. Eng.,2013, 1(5), 462[28]Hua R., Li Z. K., Chem. Eng. J., 2014, 249, 189[29]Zhang C., Sui J. H., Li. J., Tang Y. L., Cai W., Chem. Eng. J., 2012,210, 45[30]Badruddoza A. Z. M., Shawon Z. B. Z., Tay D. W. J., Hidajat K.,Uddin M. S., Carbohyd. Polym., 2013, 91, 322[31]Madadrang C. J., Kim H. Y., Gao G., Wang N., Zhu J., Feng H., Gor-ring M., Kasner M. L., Hou S., ACS Appl. Mater. Interfaces, 2012, 4(3), 1186[32]Fan L. L., Luo C. N., Li X. J., Lu F. G., Qiu H. M., Sun M., J. Ha-zard. Mater., 2012, 215, 272[33]Yang Y. F., Xie Y. L., Pang L. C., Li M., Song X. H., Wen J. G., ZhaoH. Y., Langmuir, 2013, 29, 10727。