光伏发电英文文献Ultra-High Efficiency Photovoltaic
光伏发电介绍英文作文
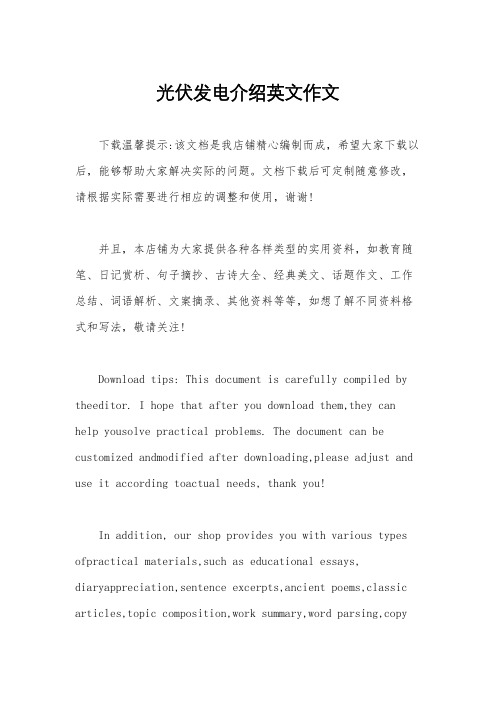
光伏发电介绍英文作文下载温馨提示:该文档是我店铺精心编制而成,希望大家下载以后,能够帮助大家解决实际的问题。
文档下载后可定制随意修改,请根据实际需要进行相应的调整和使用,谢谢!并且,本店铺为大家提供各种各样类型的实用资料,如教育随笔、日记赏析、句子摘抄、古诗大全、经典美文、话题作文、工作总结、词语解析、文案摘录、其他资料等等,如想了解不同资料格式和写法,敬请关注!Download tips: This document is carefully compiled by theeditor. I hope that after you download them,they can help yousolve practical problems. The document can be customized andmodified after downloading,please adjust and use it according toactual needs, thank you!In addition, our shop provides you with various types ofpractical materials,such as educational essays, diaryappreciation,sentence excerpts,ancient poems,classic articles,topic composition,work summary,word parsing,copyexcerpts,other materials and so on,want to know different data formats andwriting methods,please pay attention!Solar photovoltaic power generation, also known as solar power generation, is a technology that converts sunlight directly into electricity. It is a clean and renewable energy source that has been gaining popularity in recent years.The process of solar photovoltaic power generation involves the use of solar panels, which are made up of many photovoltaic cells. These cells are made of materials like silicon, which can convert sunlight into electricity. When sunlight hits the solar panels, the photovoltaic cells generate direct current (DC) electricity.One of the main advantages of solar photovoltaic power generation is its environmental friendliness. Unlike traditional energy sources like coal or natural gas, solar power generation does not produce any harmful emissions or pollutants. This makes it a sustainable and eco-friendly option for meeting energy needs.In addition to being environmentally friendly, solar photovoltaic power generation also offers economic benefits. Once the initial investment in solar panels is made, the cost of generating electricity is relatively low. This can lead to long-term cost savings for individuals and businesses that invest in solar power.Another advantage of solar photovoltaic powergeneration is its versatility. Solar panels can beinstalled on a wide range of surfaces, including rooftops, open fields, and even water surfaces. This flexibility makes it possible to generate electricity in diverse locations and environments.Despite its many advantages, solar photovoltaic power generation also faces some challenges. One of the main challenges is the intermittency of sunlight. Solar power generation is dependent on sunlight, which means that it is not always available, especially at night or during cloudy weather.Overall, solar photovoltaic power generation is a promising technology that offers numerous benefits. As technology continues to improve and costs continue to decrease, it is likely that solar power will play an increasingly important role in the global energy landscape.。
关于太阳能电池的英文文献

Recyclable organic solar cells on cellulose nanocrystal substratesYinhua Zhou 1,Canek Fuentes-Hernandez 1,Talha M.Khan 1,Jen-Chieh Liu 2,James Hsu 1,Jae Won Shim 1,Amir Dindar 1,Jeffrey P.Youngblood 2,Robert J.Moon 2,3&Bernard Kippelen 11Center for Organic Photonics and Electronics (COPE),School of Electrical and Computer Engineering,Georgia Institute ofTechnology,Atlanta,GA 30332,2School of Materials Engineering,Purdue University,West Lafayette,IN 47907,3U.S.Forest Service,Forest Products Laboratory,Madison,WI 53726.Solar energy is potentially the largest source of renewable energy at our disposal,but significant advances are required to make photovoltaic technologies economically viable and,from a life-cycle perspective,environmentally friendly,and consequently scalable.Cellulose nanomaterials are emerging high-value nanoparticles extracted from plants that are abundant,renewable,and sustainable.Here,we report on the first demonstration of efficient polymer solar cells fabricated on optically transparent cellulose nanocrystal (CNC)substrates.The solar cells fabricated on the CNC substrates display good rectification in the dark and reach a power conversion efficiency of 2.7%.In addition,we demonstrate that these solar cells can be easily separated and recycled into their major components using low-energy processes at room temperature,opening the door for a truly recyclable solar cell technology.Efficient and easily recyclable organic solar cells on CNC substrates are expected to be an attractive technology for sustainable,scalable,and environmentally-friendly energy production.Organic solar cells are an attractive technology because of their potential for low-cost fabrication,light weight,and good mechanical flexibility 1–5.Over the last decade,the power conversion efficiency (PCE)of champion small-area organic solar cells has improved from values around 3.5%up to 10.6%6.Despitehaving low PCEs and short lifetimes,recent cost-analysis studies suggest that organic solar cells could become competitive with other solar cell technologies if modules with PCE of 5%and a 5year lifetime could be produced 7,8.Polyethylene terephthalate (PET)9–11,polyethylene naphthalate (PEN)12,or polyethersulfone (PES)1,have been used for the demonstration of flexible organic solar cells.However,from a life-cycle perspective,these petroleum-based substrates are expensive and environmentally less attractive than easily recyclable or biodegradable substrates.Substrate materials which could be synthesized from renewable feedstocks,such as wood,at a low cost,are particularly attractive for the realization of a sustainable solar cell technology.Paper is considered an interesting substrate for organic solar cells,because it is inexpensive,low-weight,flexible and it can be recycled.Solution-processed polymer solar cells have been fabricated recently on paper sub-strates 13–15,but have shown limited performance because of the high surface roughness and porosity of paper substrates 13.Even with the use of thick (several to tens of m m)planarization layers,solar cells on paper showed low performance with maximum PCE values in the range between 0.4–1.4%14,15.Furthermore,the use of thick planarization layers increases the device complexity,cost and may further compromise the recyclability and biodegradability of such devices.Cellulose nanomaterials (CN)are cellulose-based nanoparticles that have good mechanical properties,high aspect ratio,low density,low thermal expansion,surfaces that can be readily chemically functionalized,low toxicity,are inherently renewable/sustainable,and have the potential to be produced in industrial-size quant-ities 16–s have been studied for a wide variety of potential applications,including reinforcement phases in polymer composites,protective coatings,barrier/filter membrane systems,antimicrobial films,network struc-tures for tissue engineering,and substrates for flexible electronics.Two general classes of CNs that can be extracted from plants,are cellulose nanocrystals (CNC,3–10nm wide by 50–500nm in length,Fig.1a)and cellulose nanofibers (CNF,4–20nm wide by .1m m in length).Neat and polymer composite films produced from CNCs and CNFs are attractive as substrates for organic electronic devices,and organic solar cells in particular,because they combine low density (1–1.5g/cm 3)with high tensile strength (30–240MPa),high elastic modulus (6–30GPa)and low coefficient of thermal expansion (CTE,2–25ppm/K)20,24–Cs are also found to be thermally stable up to 210u C,and after processing optimization,up to 350u C;hence,they are compatible withSUBJECT AREAS:SOLAR CELLSELECTRICAL AND ELECTRONICENGINEERINGELECTRONIC DEVICESELECTRONIC PROPERTIES ANDMATERIALSReceived23January 2013Accepted 11March 2013Published 25March 2013Correspondence and requests for materials should be addressed to B.K.(kippelen@ece.)the processing of organic semiconductors 27.Recently,polymer solar cells have been fabricated on CNF substrates.However,these devices exhibited poor performance (with a maximum PCE of 0.4%)and poor rectification,mainly because of the relatively rough surface of the CNF substrates (with a surface height variation of 40nm)22.In this work,we report on polymer solar cells on free-standing transparent CNC substrates with much lower surface roughness compared with the CNF-based films.The solar cells are fabricated with Ag/polymer surface modification as the bottom electrode and MoO 3/Ag as the top electrode without the need of aqueous solution.The solar cells show a large rectification in the dark and an average PCE of 2.7%and an average fill factor of 0.54under illumination.The performance of these polymer solar cells is shown to be limited primarily by the transmittance of the thin Ag layer used as the semi-transparent bottom electrode.Importantly,the polymer solar cells fabricated on CNC substrates are found to be easily recycled at room temperature by simply immersing them in water,where the CNC substrate is redispersed.The dissolution of the substrate in water leads to a separation of the rest of the components of the solar cell in the form of a thin polymer solar cell membrane comprised of the synthetic organic photoactive layer and the metal layers.These mem-branes can be easily filtered out of the water solution,and the organic and metal components can then be separated by immersing the membrane into an organic solvent in which the photoactive layer can be dissolved,leaving behind the metal and oxide electrode that can be filtered out of the solution.ResultsProperties of CNC substrates .Fig.1b illustrates the high optical transparency of the CNC film that is necessary for incident sun-light to pass through the substrate.The optical transparency is great-ly improved in thinner films as shown in Fig.S1in the supplementary information (SI).The limited transmittance of CNC films is believed to be due to scattering,not absorption,caused by the random distri-bution of CNCs (a few hundred nanometers long,Fig.1a)in the film,which causes refractive index inhomogeneities over areas with dimensions that are of the same order of magnitude of the wave-length of visible light.Scattering spreads the incident light into a large solid angle,reducing the intensity (energy per solid angle)reaching a detector and thus resulting in a reduced transmittance.However,when a solar cell is fabricated on a CNC substrate,after light passes through the substrate,even those components that arescattered far away from the sample normal can reach the active layer,where they can be absorbed and contribute to the current generated by the solar cell.Fig.1c shows the surface morphology of a CNC substrate.Averaged over three locations,the root-mean-square (RMS)value of the surface roughness is 1.860.6nm.The very smooth surface eliminates the need for any surface planariza-tion.To fabricate polymer solar cells on the CNC substrates,a transparent or semitransparent electrode is needed for light to reach the photoactive layer.For the bottom electrode (i.e.in contact with the CNC substrate),a semitransparent 20-nm thick Ag layer was deposited by vacuum thermal evaporation on a CNC substrate and was found to be conductive (i.e.above percolation).Ag films deposited simultaneously on bare glass were found to be below the percolation threshold due to wetting limitations,and con-sequently nonconductive.Based on our recent discovery 1,we modi-fied the Ag film using a thin layer of ethoxylated polyethylenimine (PEIE)to turn silver into an efficient electron-collecting electrode.For the top electrode,MoO 3/Ag was evaporated onto the photo-active layer of [poly[(4,8-bis-(2-ethylhexyloxy)-benzo[1,2-b:4,5-b 9]dithiophene)-2,6-diyl-alt-(4-(2-ethylhexanoyl)-thieno[3,4-b]thiophene)-2,6-diyl]:phenyl-C 61-butyric acid methyl ester](PBD-TTT-C:PCBM,Fig.2b)to collect holes.The device geometry is shown in Fig.2a.It should be noted that the spin-coating of PEIE from a 2-methoxyethanol solution did not damage the CNC substrates.The latter were also found to allow the spin-coating of the PBDTTT-C:PCBM photoactive layer from a chlorobenzene:1,8-diiodooctane (9753,v/v)solution.A fabricated solar cell is shown in Fig.2c.The high and specular reflectivity of the Ag top electrode further demonstrates the surface smoothness of the CNC substrates and the uniformity of the active layer on the CNC substrates.Performance of solar cells on CNC substrates .Fig.2d shows the current density-voltage (J –V )characteristic of a solar cell fabricated on a CNC substrate in the dark and under illumination.In the dark,the device shows low reverse saturation current and large rectification ratio of 103at 61V (Fig.2e).This indicates few pin holes and a large work function contrast between Ag/PEIE and MoO 3/Ag.Under 95mW/cm 2of AM 1.5G illumination,the devices show V OC 50.6560.01V,J SC 57.560.1mA/cm 2,and FF 50.5460.01,yielding PCE 52.760.1%,averaged over 3devices.Although this is still modest performance compared to state-of-the-art devices,it represents a significantimprovementFigure 1|(a)A transmission electron microscopy (TEM)image of isolated CNCs which comprise the film used as the substrate for solar cells;(b)a picture of the CNC film on top of paper to demonstrate its transparency;(c)an atomic force microscopy image of the surface of a CNC film.over previously demonstrated organic solar cells on paper-like or CNF substrates 14,22.Furthermore,we recently reported that devices with a structure:Glass/ITO/PEIE/PBDTTT-C:PCBM/MoO 3/Ag yield values of V OC 50.6860.01V,J SC 516.160.4mA/cm 2,FF 50.6160.01,and PCE 56.660.2%,averaged over 5devices 1.Remarkably,the V OC and FF of the solar cells on a CNC substrate are not that different to the ones obtained on a glass/ITO substrate.This is in contrast to previous realizations of polymer solar cells on paper-like substrates,wherein the electrical performance of the solar cells,namely the V OC and FF values,were found to be significantly lower than those on devices fabricated on glass or plastic substrates 13,14,22.Hence,the lower PCE value obtained,is mainly attributed to the smaller J SC value on solar cells processed on CNC/Ag substrates as compared to the J SC value obtained on glass/ITO substrates.This is caused by the lower transmittance of both the CNC substrates compared to glass,and of the 20-nm-thick Ag electrode compared to ITO 28.The ability to tune CNC substrates (composition,orien-tation,interfaces,etc.)should allow further optimization of its optical and mechanical properties.Likewise,if the Ag film (bottom elec-trode)can be replaced with a higher transmittance material (e.g.metal-oxide or conducting polymer)further improvements in the performance level of these polymer solar cells fabricated on CNC substrates can be achieved,and possibly comparable to devices fabricated on glass or petroleum-based flexible substrates.Recyclability of solar cells on CNC substrates .Recyclability of the CNC substrates and solar cells was tested by immersing them into distilled water.Fig.S2displays time-lapse images that illustrate the dissolution of the CNC substrates in water.The CNC film quicklyswells after being immersed into water and completely disintegrates within 30min.The redispersed CNC turns into a solid residue on the petri dish after the water has evaporated.This residue can potentially be recovered and recycled.As for the solar cell,the CNC substrate also swells rapidly producing clear warping of the photoactive layer and electrodes (insets of Fig.S2)until they turn into a free-standing film or membrane.This allows for the full separation of the solar cell components (substrate,organic and inorganic materials)at room temperature by using a filter paper.A video illustrating the process whereby the materials of a solar cell can be easily recovered is available in the SI.Fig.3a displays vials containing the solutions and the final residue on the filter paper to illustrate the final products of this recycling process.The process is described as follows:a solar cell was immersed into the vial containing distilled water until the CNC substrate disintegrated (shaking accelerates the disintegration to less than 10min)and the solid residues were filtered from the liquid using a filter paper.The resulting distilled water waste appears as a milky dispersion of CNCs in water,shown in vial #1in Fig.3a.Distilled water is shown as a clear liquid in vial #0in Fig.3a as a visual reference.The photoactive layer was then separated from the electrodes by rinsing the solid residues on the filter paper with chlorobenzene.This process resulted in a green-colored solution of a mixture of chlorobenzene and PBDTTT-C:PCBM,as shown in vial #2in Fig.3a.A second rinse with chlorobenzene revealed that most of the active layer could be dissolved during the first rinse,as illustrated by the clear color of the vial #3in Fig.3a.The solid waste left in the filter paper shown in #4in Fig.3a,therefore,corresponds primarily to the Ag and MoO 3used as electrodes on the solar cell.In this way,organic solar cells fabricated onCNCFigure 2|(a)Device structure of solar cells on CNC substrates:CNC/Ag/PEIE/PBDTTT-C:PCBM/MoO 3/Ag;(b)Chemical structure ofPBDTTT-C and PCBM;(c)a picture of a fabricated solar cell;(d)J–V characteristics of the solar cell on CNC substrate in the dark (thin black line)and under 95mW/cm 2of AM1.5illumination (thick red line);(e)the J–V characteristics on a semi-logarithmic scale in the dark (thin black line)and under illumination (thick red line).substrates can be easily separated into their major components using a minimal amount of solvents and energy.Furthermore,solar cells on CNC substrates that were exposed to low temperature flame (to burn off the polymer components),produced ashes from which the metal components could be recovered.Fig.3b displays images of solar cells burning and rapidly reducing into ashes.While burning may not be an ideal way to dispose of the devices,the ability to burn them,leaving a residue of ashes,is dramatically different than what could be obtained using glass or plastic substrates.DiscussionEfficient and recyclable polymer solar cells fabricated on free-stand-ing cellulose nanocrystal substrates have been demonstrated here.The cellulose nanocrystal substrates are optically transparent enab-ling light to go through,and have a low surface roughness (RMS value of 1.8nm)which is critical for the thin polymer solar cells to work nicely with very low reverse leakage current and large rectifica-tion ratio in the dark.Furthermore,the cellulose nanocrystal sub-strates allow the deposition of polymers using coating techniques from non-aqueous solutions.The polymer solar cells on cellulose nanocrystal substrates reached a power conversion efficiency of 2.7%,an unprecedented level of performance for polymer solar cell fabricated on recyclable substrates derived from renewable feed-stocks.The power conversion efficiency of these solar cells was found to be primarily limited by the low transmittance of the Ag bottom electrode.Optimization of the optical properties of the bottom elec-trode should lead to significant future improvements on the power conversion efficiency,for instance,by reducing the thickness of the Ag layer and by introducing a layer with a high reflective index material between the substrate and the Ag layer 29or by depositing a transparent metal-oxide electrode.If this is realized,polymer solarcells with a power conversion efficiency that is similar to that of solar cells fabricated on glass or petroleum-based plastic substrates should be achievable.We have shown that these solar cells can be easily separated into their major components using low-energy processes at room temperature,opening the door for a truly fully recyclable solar cell technology.Efficient and easily recyclable polymer solar cells on cellulose nanocrystal substrates could be an ideal technology for sustainable,scalable and environmentally-friendly energy pro-duction and could have an overreaching impact for the sustainability of printed electronics.MethodsPreparation and characterization of CNC samples .CNCs were produced at USDA Forest Service-Forest Products Laboratory (Madison,WI)following procedures described by Beck-Candanedo et al.30CNC suspensions were produced by sulfuric acid hydrolysis of softwood pulp (64%sulfuric acid,8to 1acid to pulp weight ratio,45u C,60minutes)followed by quenching with deionized water,centrifuge rinsing,washing,and then dialysis for about a week to remove remaining acid.Thesuspension was then ultrasonicated to disperse the CNCs via mechanical agitation and centrifuged a final time for macroparticle removal.Films were prepared by blending 1.65wt.%CNC suspension (30g)with 1wt.%glycerol solution (4.95g)for 24hours.Glycerol (Aldrich)was added to make the films more flexible for handling.The homogeneous glycerol/CNC water suspension was then poured into plastic 80mm diameter plastic petri dishes and allowed to dry at 23u C and 30%–40%relative humidity.The dried CNC/glycerol films were detached from petri dishes and cut into 2.5cm 32.5cm glycerol/CNC substrates.Note that the addition glycerol isconsistent with renewable and biodegrade theme of the CNC film and it is non-toxic and is a byproduct of biodiesel production.The optical transmittance of CNC substrates was measured using a spectroscopic ellipsometer apparatus (M-2000UI,J.A.Woollam Co.).The surface roughness of the CNC samples was measured under atmospheric conditions using atomic force microscopy (Dimension 3100,Veeco)equipped with a NanoScope III controller.Fabrication and characterization of solar cells on CNC substrates .First,the CNC films were attached to rigid glass substrates with a piece of curedpolydimethylsiloxane (PDMS).Then,a 20-nm thick Ag film was deposited on halfofFigure 3|(a)Vials (#0-3)and filter paper (#4)illustrating the separation of solar cells into their major components by immersion in water and chlorobenzene.Vial #0:distilled water;Vial #1:CNC redispersed in distilled water after solar cells were immersed into water;Vial #2:solution of photoactive layer in chlorobenzene obtained by rinsing the solid waste left after the immersion into water;Vial #3:solution generated by the second rinsing the solid waste with chlorobenzene;#4:solid residues left on the filter paper after the second rinsing with chlorobenzene.The inset is a close-up of the solid waste left on the filter paper showing residues of Ag and MoO 3.(b)Time lapse sequence of three frames illustrating the ignition of solar cells on CNC substrates:#1:an image of a solar cell before burning;#2:while burning;#3:after burning.Burning lasted less than 2s.the CNC substrates through a shadow mask,using a vacuum thermal evaporation system(SPECTROS,Kurt J.Lesker).Then,the polymer modification layer,PEIE (423475,Mw570,000g/mol,from Sigma-Aldrich)was deposited on Ag by spin-coating at a speed of4000rpm for1minute from a0.4wt.%2-methoxyethanol (284467,99.8%anhydrous,from Sigma-Aldrich Co.)solution and annealed on a hot plate at80u C for5minutes.The average thickness of the PEIE is estimated to be 10nm,from measurements by spectroscopic ellipsometry on independent films deposited on Si substrates1.After the substrates cooled down for10minutes,a layer of PBDTTT-C(Solarmer Materials Inc):PCBM(151.5by weight,Nano-C Inc.)was spin-coated on the substrates as the photoactive layer from a mixture of chlorobenzene:1,8-diiodooctane(9753,v/v)solution with a total concentration of 25mg/ml at a speed of1000rpm and10000rpm/s acceleration for1minute.The thickness of the photoactive layer was90nm.All the processing was done in aN2-filled glove box.Samples were transferred into the vacuum thermal evaporation system(SPECTROS,Kurt J.Lesker)and the top electrode of MoO3/Ag(15nm/150nm)was deposited to finish the device fabrication.Currentdensity-voltage(J–V)characteristics of the solar cells were measured inside theN2-filled glove box by using a source meter(2400,Keithley Instruments,Cleveland, OH)controlled by a LabVIEW program.To test the solar cell properties under illumination,a calibrated300W Oriel solar simulator(91160,Newport)with an intensity of95mW/cm2was used as the light source.Recycling and combustion of solar cells.A piece of CNC sample and a piece of solar cell on CNC substrate were immersed into distilled water in a glass petri dish until the CNC were redispersed at room temperature.Another piece of solar cell on a CNC substrate was immersed in distilled water in a vial.The solution was filtered using a P5 Filter paper(Fisher Scientific).The solid waste in the filter was rinsed with chlorobenzene and the waste collected in a vial.Chlorobenzene rinsing was repeated for the second time.For the combustion test,a piece of CNC sample and a piece of solar cell on CNC were ignited using a commercial lighter and burned inside a fume hood.1.Zhou,Y.H.et al.A Universal Method to Produce Low-Work Function Electrodesfor Organic Electronics.Science336,327–332(2012).2.Kippelen,B.&Bredas,anic photovoltaics.Energy Environ.Sci.2,251–261(2009).3.He,Z.et al.Enhanced power-conversion efficiency in polymer solar cells using aninverted device structure.Nat.Photon.6,593–597(2012).4.Brabec,C.J.et al.Polymer-fullerene bulk-heterojunction solar cells.Adv.Mater.22,3839–3856(2010).5.Sondergaard,R.,Hosel,M.,Angmo,D.,Larsen-Olsen,T.T.&Krebs,F.C.Roll-to-roll fabrication of polymer solar cells.Mater.Today15,36–49(2012).6.You,J.et al.A polymer tandem solar cell with10.6%power conversion efficiency.mun.4,1446(2013).7.Azzopardi,B.et al.Economic assessment of solar electricity production fromorganic-based photovoltaic modules in a domestic environment.Energy Environ.Sci.4,3741–3753(2011).8.Espinosa,N.,Garcia-Valverde,R.&Krebs,F.C.Life-cycle analysis of productintegrated polymer solar cells.Energy Environ.Sci.4,1547–1557(2011).9.Zhou,Y.et al.Investigation on polymer anode design for flexible polymer solarcells.Appl.Phys.Lett.92,233308(2008).10.Formica,N.et al.Highly stable Ag–Ni based transparent electrodes on PETsubstrates for flexible organic solar cells.Sol.Energy Mater.Sol.Cells107,63–68 (2012).11.Krebs,F.C.Polymer solar cell modules prepared using roll-to-roll methods:Knife-over-edge coating,slot-die coating and screen printing.Sol.Energy Mater.Sol.Cells93,465–475(2009).12.Wang,J.-C.et al.Highly efficient flexible inverted organic solar cells using atomiclayer deposited ZnO as electron selective layer.J.Mater.Chem.20,862–866 (2010).13.Wang,F.,Chen,Z.,Xiao,L.,Qu,B.&Gong,Q.Papery solar cells based ondielectric/metal hybrid transparent cathode.Sol.Energy Mater.Sol.Cells94, 1270–1274(2010).14.Kim,T.-S.et al.Solution-processible polymer solar cells fabricated on a paperysubstrate.Phys.Status Solidi RRL6,13–15(2012).15.Hu¨bler,A.et al.Printed Paper Photovoltaic Cells.Adv.Energy Mater.1,1018–1022(2011).16.Vartiainen,J.et al.Health and environmental safety aspects of friction grindingand spray drying of microfibrillated cellulose.Cellulose18,775–786(2011).17.Lin,N.,Huang,J.&Dufresne,A.Preparation,properties and applications ofpolysaccharide nanocrystals in advanced functional nanomaterials:a review.Nanoscale4,3274–3294(2012).voine,N.,Desloges,I.,Dufresne,A.&Bras,J.Microfibrillated cellulose–Itsbarrier properties and applications in cellulosic materials:A review.Carbohydr.Polym.90,735–764(2012).19.Habibi,Y.,Lucia,L.A.&Rojas,O.J.Cellulose Nanocrystals:Chemistry,Self-Assembly,and Applications.Chem.Rev.110,3479–3500(2010).20.Moon,R.J.,Martini,A.,Nairn,J.,Simonsen,J.&Youngblood,J.Cellulosenanomaterials review:structure,properties and nanocomposites.Chem.Soc.Rev.40,3941–3994(2011).21.Klemm,D.et al.Nanocelluloses:A New Family of Nature-Based Materials.Angew.Chem.Int.Ed.50,5438–5466(2011).22.Hu,L.et al.Transparent and conductive paper from nanocellulose fibers.EnergyEnviron.Sci.6,513–518(2013).23.Siro´,I.&Plackett,D.Microfibrillated cellulose and new nanocomposite materials:a review.Cellulose17,459–494(2010).24.Nogi,M.,Iwamoto,S.,Nakagaito,A.N.&Yano,H.Optically TransparentNanofiber Paper.Adv.Mater.21,1595–1598(2009).25.Fukuzumi,H.,Saito,T.,Iwata,T.,Kumamoto,Y.&Isogai,A.Transparent andHigh Gas Barrier Films of Cellulose Nanofibers Prepared by TEMPO-Mediated Oxidation.Biomacromolecules10,162–165(2008).26.Kim,J.,Yun,S.&Ounaies,Z.Discovery of cellulose as a smart material.Macromolecules39,4202–4206(2006).27.Yu,H.et al.Facile extraction of thermally stable cellulose nanocrystals with a highyield of93%through hydrochloric acid hydrolysis under hydrothermalconditions.J.Mater.Chem.A1,3938–3944(2013).28.Hsu,J.et al.Linear and nonlinear optical properties of Ag/Au bilayer thin films.Opt.Express20,8629–8640(2012).29.Salinas,J.-F.et al.Optical Design of Transparent Thin Metal Electrodes toEnhance In-Coupling and Trapping of Light in Flexible Polymer Solar Cells.Adv.Mater.24,6362–6367(2012).30.Beck-Candanedo,S.,Roman,M.&Gray,D.G.Effect of Reaction Conditions onthe Properties and Behavior of Wood Cellulose Nanocrystal Suspensions.Biomacromolecules6,1048–1054(2005).AcknowledgementsThis research was funded in part through the Center for Interface Science:Solar Electric Materials,an Energy Frontier Research Center funded by the U.S.Department of Energy, Office of Science,Office of Basic Energy Sciences under Award Number DE-SC0001084 (Y.Z.,J.S.,C.F.,A.D.),by the Air Force Office of Scientific Research(Grant No.FA9550-09-1-0418)(J.H.),by the Office of Naval Research(Grant No.N00014-04-1-0313) (T.K.,B.K.),and the US Department of Agriculture–Forest Service(Grant No.12-JV-11111122-098).Funding for CNC substrate processing was provided byUSDA-Forest Service(Grant No.11-JV-11111129-118)(R.J.M.,J.P.Y.,J.L.).The authors thank Rick Reiner and Alan Rudie from the U.S.Forest Service-Forest Products Laboratory (FPL)for providing CNC materials.Author contributionsB.K.,C.F.,R.J.M.and J.P.Y.conceived this project.Y.Z.,T.K.and J.S.designed and fabricated the solar cells.J.L.fabricated the CNC films.J.H.and A.D.collected the data of transmittance and surface morphology of the films.Y.Z.and C.F.performed the recycling experiments of solar cells.Y.Z.and C.F.wrote the draft of the manuscript,and all authors contributed to the edits of the manuscript.Additional informationSupplementary information accompanies this paper at / scientificreportsCompeting financial interests:The authors declare no competing financial interests. License:This work is licensed under a Creative CommonsAttribution-NonCommercial-NoDerivs3.0Unported License.To view a copy of this license,visit /licenses/by-nc-nd/3.0/How to cite this article:Zhou,Y.H.et al.Recyclable organic solar cells on cellulose nanocrystal substrates.Sci.Rep.3,1536;DOI:10.1038/srep01536(2013).。
光伏英语文件
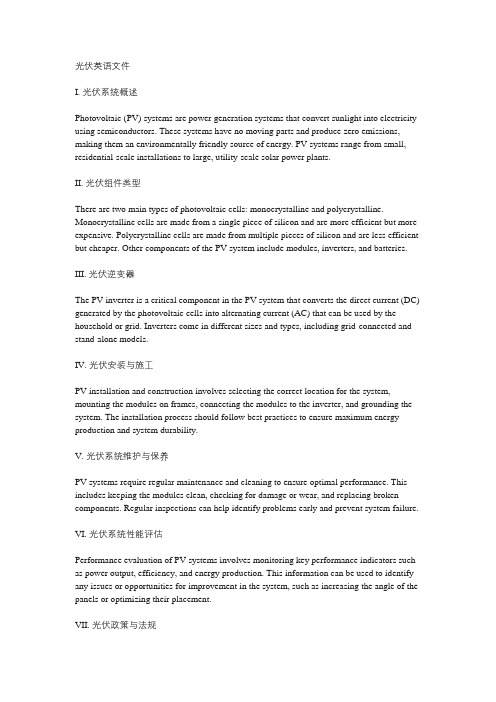
光伏英语文件I. 光伏系统概述Photovoltaic (PV) systems are power generation systems that convert sunlight into electricity using semiconductors. These systems have no moving parts and produce zero emissions, making them an environmentally friendly source of energy. PV systems range from small, residential-scale installations to large, utility-scale solar power plants.II. 光伏组件类型There are two main types of photovoltaic cells: monocrystalline and polycrystalline. Monocrystalline cells are made from a single piece of silicon and are more efficient but more expensive. Polycrystalline cells are made from multiple pieces of silicon and are less efficient but cheaper. Other components of the PV system include modules, inverters, and batteries.III. 光伏逆变器The PV inverter is a critical component in the PV system that converts the direct current (DC) generated by the photovoltaic cells into alternating current (AC) that can be used by the household or grid. Inverters come in different sizes and types, including grid-connected and stand-alone models.IV. 光伏安装与施工PV installation and construction involves selecting the correct location for the system, mounting the modules on frames, connecting the modules to the inverter, and grounding the system. The installation process should follow best practices to ensure maximum energy production and system durability.V. 光伏系统维护与保养PV systems require regular maintenance and cleaning to ensure optimal performance. This includes keeping the modules clean, checking for damage or wear, and replacing broken components. Regular inspections can help identify problems early and prevent system failure.VI. 光伏系统性能评估Performance evaluation of PV systems involves monitoring key performance indicators such as power output, efficiency, and energy production. This information can be used to identify any issues or opportunities for improvement in the system, such as increasing the angle of the panels or optimizing their placement.VII. 光伏政策与法规PV policies and regulations vary from country to country and region to region. They cover topics such as grid connection, subsidies, tariffs, and product standards. Staying up-to-date with these policies and regulations is essential for those involved in the PV industry.VIII. 光伏市场前景The PV market is rapidly growing as governments and private companies increase their commitment to renewable energy sources. Advances in technology have also helped reduce costs and improve efficiency, making PV systems more affordable and accessible. The future of the PV industry looks promising as we move towards a more sustainable energy future.。
光伏发电中英对照文献

外文参考文献译文及原文目录外文文献译文 (1)1.中国光伏发电的战略地位 (1)2.世界光伏产业现状和发展预测 (2)3.中国光伏发电市场和产业现状 (3)4.中国光复发电的市场预测和规划建议 (5)5.结论 (6)外文文献原文 (7)1.China's strategic position PV (7)2.The world's current situation and development of photovoltaic industryforecast (9)3.The Chinese PV market and industry statu s (10)4.China's PV market forecasting and planning proposals (13)5.Conclusions (15)外文文献译文1、中国光伏发电的战略地位1.1 中国的能源资源和可再生能源现状和预测;无论从世界还是从中国来看,常规能源都是很有限的,中国的一次能源储量远远低于世界的平均水平,大约只有世界总储量的10%。
从长远来看,可再生能源将是未来人类的主要能源来源,因此世界上多数发达国家和部分发展中国家都十分重视可再生能源对未来能源供应的重要作用。
在新的可再生能源中,光伏发电和风力发电是发展最快的,世界各国都把太阳能光伏发电的商业化开发和利用作为重要的发展方向。
根据欧洲JRC 的预测,到2030年太阳能发电将在世界电力的供应中显现其重要作用,达到10%以上,可再生能源在总能源结构中占到30%;2050 年太阳能发电将占总能耗的20%,可再生能源占到50%以上,到本世纪末太阳能发电将在能源结构中起到主导作用。
我国政府重视可再生能源技术的发展,主要有水能、风能、生物质能、太阳能、地热能和海洋能等。
我国目前可再生能源的发展现状如下:水能:我国经济可开发的水能资源量为3.9 亿千瓦,年发电量1.7 万亿千瓦时,其中5 万千瓦及以下的小水电资源量为1.25 亿千瓦。
毕业设计论文 外文文献翻译 光伏电力系统 中英文对照
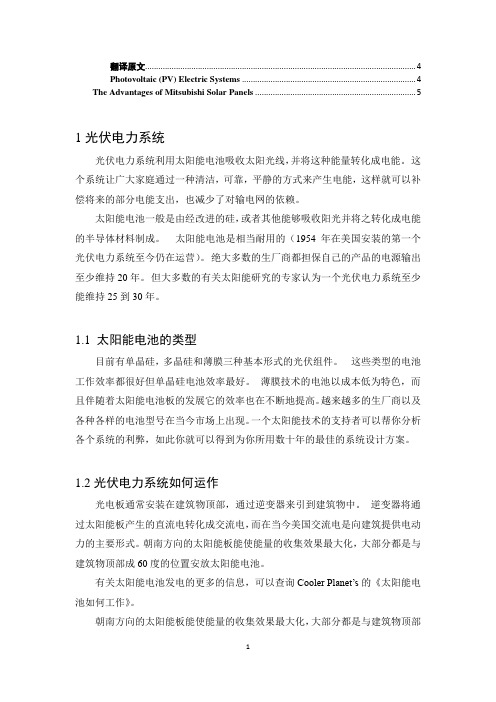
翻译原文 (4)Photovoltaic (PV) Electric Systems (4)The Advantages of Mitsubishi Solar Panels (5)1光伏电力系统光伏电力系统利用太阳能电池吸收太阳光线,并将这种能量转化成电能。
这个系统让广大家庭通过一种清洁,可靠,平静的方式来产生电能,这样就可以补偿将来的部分电能支出,也减少了对输电网的依赖。
太阳能电池一般是由经改进的硅,或者其他能够吸收阳光并将之转化成电能的半导体材料制成。
太阳能电池是相当耐用的(1954年在美国安装的第一个光伏电力系统至今仍在运营)。
绝大多数的生厂商都担保自己的产品的电源输出至少维持20年。
但大多数的有关太阳能研究的专家认为一个光伏电力系统至少能维持25到30年。
1.1 太阳能电池的类型目前有单晶硅,多晶硅和薄膜三种基本形式的光伏组件。
这些类型的电池工作效率都很好但单晶硅电池效率最好。
薄膜技术的电池以成本低为特色,而且伴随着太阳能电池板的发展它的效率也在不断地提高。
越来越多的生厂商以及各种各样的电池型号在当今市场上出现。
一个太阳能技术的支持者可以帮你分析各个系统的利弊,如此你就可以得到为你所用数十年的最佳的系统设计方案。
1.2光伏电力系统如何运作光电板通常安装在建筑物顶部,通过逆变器来引到建筑物中。
逆变器将通过太阳能板产生的直流电转化成交流电,而在当今美国交流电是向建筑提供电动力的主要形式。
朝南方向的太阳能板能使能量的收集效果最大化,大部分都是与建筑物顶部成60度的位置安放太阳能电池。
有关太阳能电池发电的更多的信息,可以查询Cooler Planet’s的《太阳能电池如何工作》。
朝南方向的太阳能板能使能量的收集效果最大化,大部分都是与建筑物顶部成60度的位置安放太阳能电池。
1.3 太阳能电池板与光伏建筑一体化太阳能电池板是用于捕获太阳光的平面板,他们以阵列的形式安装在建筑物顶部或者柱子上。
他们是传统的用于获得太阳能的阵列形式。
光伏发电英文文献

光伏发电英文文献本文介绍光伏发电的背景和意义,并概述了文献的目的和重要性。
光伏发电是利用太阳能转换成电能的一种清洁能源技术。
随着全球环境问题的日益严重,清洁能源的开发和利用成为各国的重点研究领域。
光伏发电作为其中一种重要的清洁能源形式,具有不污染、可再生、无噪音等优点,受到了广泛关注。
本文的目的是系统整理和分析光伏发电领域的英文文献,以提供给研究人员和相关领域的专业人士参考和借鉴。
本文对光伏发电技术、光伏电池的原理和性能、光伏发电系统的设计与优化等方面的研究文献进行了综述和总结,旨在促进光伏发电技术的发展与应用。
正确认识和深入理解光伏发电的背景和意义,对于推动清洁能源的发展,减少对传统能源的依赖具有重要意义。
本文的编写是基于独立决策和创造性思维的原则,旨在简单明了地介绍相关内容,避免出现法律复杂问题。
为保证文献的准确性和可信度,不引用无法确认来源的内容。
希望本文能为光伏发电领域的研究提供有价值的信息,促进光伏发电技术的发展和应用。
通过本文的阅读,读者将能够全面了解光伏发电的背景、意义以及文献的目的和重要性。
光伏发电技术是一种利用太阳能将光子转化为电能的技术。
该技术基于光伏效应,即光子被半导体吸收后,会产生光电效应,将光能转化为电能。
光伏发电系统中的关键组件是太阳能电池。
太阳能电池通常由硅材料制成,具有两个层次的结构:正负型半导体结构。
当太阳光照射到太阳能电池上时,光子会被吸收到半导体材料内,导致半导体中的电子从价带跃迁到导带,从而产生电荷。
光伏电池内的电荷分离后,电子通常通过电流路径流动,在外部电路中形成电流。
通过连接多个光伏电池,可以形成光伏电池阵列,并将产生的电能输出到电网或用于供电。
光伏发电技术的优势包括可再生、环保和可靠性高等方面。
它是一种清洁能源技术,对环境没有污染,并且可以实现长期稳定的电能供应。
总之,光伏发电技术的原理基于光伏效应,通过将太阳光转化为电能,实现可再生能源的利用。
光伏发电系统主要由以下部分组成:光伏电池(Photovoltaic Cells): 光伏电池是将太阳能直接转化为电能的基本装置。
光伏发电介绍英文作文
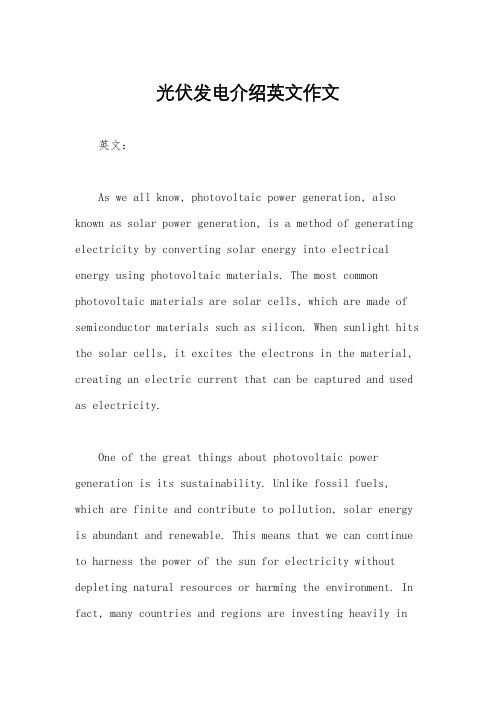
光伏发电介绍英文作文英文:As we all know, photovoltaic power generation, also known as solar power generation, is a method of generating electricity by converting solar energy into electrical energy using photovoltaic materials. The most common photovoltaic materials are solar cells, which are made of semiconductor materials such as silicon. When sunlight hits the solar cells, it excites the electrons in the material, creating an electric current that can be captured and used as electricity.One of the great things about photovoltaic power generation is its sustainability. Unlike fossil fuels, which are finite and contribute to pollution, solar energy is abundant and renewable. This means that we can continue to harness the power of the sun for electricity without depleting natural resources or harming the environment. In fact, many countries and regions are investing heavily insolar power as a clean and sustainable energy source.Another benefit of photovoltaic power generation is its versatility. Solar panels can be installed on a wide rangeof surfaces, from rooftops to open fields, making it a flexible option for generating electricity. In addition, solar panels can be used in both grid-connected and off-grid systems, providing power to remote areas that may not have access to traditional electricity sources.In my own experience, I have seen the impact of photovoltaic power generation firsthand. In my hometown, many households have installed solar panels on their roofsto generate electricity for their own use. This has notonly reduced their reliance on traditional power sources, but also saved them money on electricity bills. Furthermore, I have visited solar farms where vast fields of solarpanels are used to generate large amounts of electricityfor the local community. It's amazing to see how the powerof the sun can be harnessed to provide clean andsustainable energy for so many people.Overall, photovoltaic power generation is a promising and environmentally friendly method of generating electricity. With ongoing advancements in technology and increasing awareness of the importance of renewable energy, I believe that solar power will play an increasingly significant role in meeting our energy needs in the future.中文:众所周知,光伏发电,也被称为太阳能发电,是一种利用光伏材料将太阳能转换为电能的发电方法。
光伏发电系统外文翻译文献

文献信息:文献标题:A New Controller Scheme for Photovoltaics Power Generation Systems(光伏发电系统的一种新的控制方案)国外作者:Tamer T.N.Khatib,Azah Mohamed,Nowshad Amin文献出处:《European Journal of Scientific Research》,2009,Vol.33 No.3, pp515-524字数统计:英文1337单词,7006字符;中文2149汉字外文文献:A New Controller Scheme for Photovoltaics PowerGeneration SystemsAbstract:This paper presents a new controller scheme for photovoltaic (PV) power generation systems. The proposed PV controller scheme controls both the boost converter and the battery charger by using a microcontroller in order to extract maximum power from the PV array and control the charging process of the battery. The objective of the paper is to present a cost effective boost converter design and an improved maximum power point tracking algorithm for the PV system. A MATLAB based simulation model of the proposed standalone PV system has been developed to evaluate the feasibility of the system in ensuring maximum power point operation.1.IntroductionRecently, the installation of PV generation systems is rapidly growing due to concerns related to environment, global warming, energy security, technology improvements and decreasing costs. PV generation system is considered as a clean and environmentally-friendly source of energy. The main applications of PV systems are in either standalone or grid connected configurations. Standalone PV generationsystems are attractive as indispensable electricity source for remote areas. However, PV generation systems have two major problems which are related to low conversion efficiency of about 9 to 12 % especially in low irradiation conditions and the amount of electric power generated by PV arrays varies continuously with weather conditions. Therefore, many research works are done to increase the efficiency of the energy produced from the PV arrays.The solar cell V-I characteristics is nonlinear and varies with irradiation and temperature. But there is a unique point on the V-I and P-V curves, called as the maximum power point (MPP), at which at this point the PV system is said to operate with maximum efficiency and produces its maximum power output. The location of the MPP is not known but can be traced by either through calculation models or search algorithms. Thus, maximum power point tracking (MPPT) techniques are needed to maintain the PV array’s operating point at its MPP. Many MPPT techniques have been proposed in the literature in which the techniques vary in many aspects, including simplicity, convergence speed, hardware implementation and range of effectiveness. However, the most widely used MPPT technique is the perturbation and observation (P&O) method. This paper presents a simple MPPT algorithm which can be easily implemented and adopted for low cost PV applications. The objective of this paper is to design a novel PV controller scheme with improved MPPT method.The proposed standalone PV controller implementation takes into account mathematical model of each component as well as actual component specification. The dc–dc or boost converter is the front-end component connected between the PV array and the load. The conventional boost converter may cause serious reverse recovery problem and increase the rating of all devices. As a result, the conversion efficiency is degraded and the electromagnetic interference problem becomes severe under this situation. To increase the conversion efficiency, many modified step-up converter topologies have been investigated by several researchers. V oltage clamped techniques have been incorporated in the converter design to overcome the severe reverse-recovery problem of the output diodes. In this paper, focus is also given in the boost converter design. Another important component in the standalone PV systemsis the charge controller which is used to save the battery from possible damage due to over-charging and over-discharging. Studies showed that the life time of a battery can be degraded without using a charge controller.The proposed new controller scheme for the standalone PV system controls both the boost converter and the charge controller in two control steps. The first step is to control the boost converter so as to extract the maximum power point of the PV modules. Here, a high step-up converter is considered for the purpose of stepping up the PV voltage and consequently reducing the number of series-connected PV modules and to maintain a constant dc bus voltage. A microcontroller is used for data acquisition that gets PV module operating current and voltage and is also used to program the MPPT algorithm. The controller adopts the pulse width modulation (PWM) technique to increase the duty cycle of the generated pulses as the PV voltage decreases so as to obtain a stable output voltage and current close to the maximum power point. The second control step is to control the charge controller for the purpose of protecting the batteries. By controlling the charging current using the PWM technique and controlling the battery voltage during charging, voltages higher than the gassing voltage can be avoided.2.Design of the Proposed Photovoltaic SystemMost of the standalone PV systems operate in one mode only such that the PV system charges the battery which in turns supply power to the load. In this mode of operation, the life cycle time of the battery may be reduced due to continuous charging and discharging of the battery. The proposed standalone PV system as shown in terms of a block diagram in Figure 1 is designed to operate in two modes: PV system supplies power directly to loads and when the radiation goes down and the produced energy is not enough, the PV system will charge the battery which in turns supply power to the load. To manage these modes of operation, a controller is connected to the boost converter by observing the PV output power.3.MethodologyFor the purpose of estimating the mathematical models developed for the proposed standalone PV system, simulations were carried in terms of the MATLAB codes. Each PV module considered in the simulation has a rating of 80 Watt at 1000 W/m2, 21.2 V open circuit voltage, 5A short circuit current. The PV module is connected to a block of batteries with of sizing 60 Ah, 48 V.4.Results and DiscussionThe simulation results of the standalone PV system using a simple MPPT algorithm and an improved boost converter design are described in this section. Simulations were carried out for the PV system operating above 30o C ambient temperature and under different values of irradiation. Figure 9 shows the PV array I-V characteristic curve at various irradiation values. From the figure, it is observed that the PV current increase linearly as the irradiation value is increased. However, the PV voltage increases in logarithmic pattern as the irradiation increases. Figure 10 shows the PV array I-V characteristic curve at various temperature values. It is noted from the figure that, the PV voltage decreases as the ambient temperature is increased.Figure 4 compares the PV array P-V characteristics obtained from using the proposed MPPT algorithm and the classical MPPT P&O algorithm. From this figure, it can be seen that by using the proposed MPPT algorithm, the operating point of PV array is much closer to the MPP compared to the using the classical P&O algorithm.In addition, the proposed boost converter is able to give a stable output voltage as shown in Figure 5. In terms of PV array current, it can be seen from Figure 6 that the PV current is closer to the MPP current when using the improved MPPT algorithm. Thus, the track operating point is improved by using the proposed MPPT algorithm. In terms of efficiency of the standalone PV system which is calculated by dividing the load power with the maximum power of PV array, it is noted that the efficiency of the system is better with the proposed MPPT algorithm as compared to using the classical P&O algorithm as shown in Figure 7.5.ConclusionThis paper has presented an efficient standalone PV controller by incorporating an improved boost converter design and a new controller scheme which incorporates both a simple MPPT algorithm and a battery charging algorithm. The simulation results show that the PV controller using the simple MPPT algorithm has provided more power and better efficiency (91%) than the classical P&O algorithm. In addition, the proposed boost converter design gives a better converter efficiency of about 93%. Such a PV controller design can provide efficient and stable power supply for remote mobile applications.中文译文:光伏发电系统的一种新的控制方案摘要:本文提出了一种新的光伏(PV)发电系统控制器方案。
- 1、下载文档前请自行甄别文档内容的完整性,平台不提供额外的编辑、内容补充、找答案等附加服务。
- 2、"仅部分预览"的文档,不可在线预览部分如存在完整性等问题,可反馈申请退款(可完整预览的文档不适用该条件!)。
- 3、如文档侵犯您的权益,请联系客服反馈,我们会尽快为您处理(人工客服工作时间:9:00-18:30)。
Ultra-High Efficiency Photovoltaic Cells for Large Scale Solar Power GenerationYoshiaki NakanoAbstract The primary targets of our project are to dras-tically improve the photovoltaic conversion efficiency and to develop new energy storage and delivery technologies. Our approach to obtain an efficiency over40%starts from the improvement of III–V multi-junction solar cells by introducing a novel material for each cell realizing an ideal combination of bandgaps and lattice-matching.Further improvement incorporates quantum structures such as stacked quantum wells and quantum dots,which allow higher degree of freedom in the design of the bandgap and the lattice strain.Highly controlled arrangement of either quantum dots or quantum wells permits the coupling of the wavefunctions,and thus forms intermediate bands in the bandgap of a host material,which allows multiple photon absorption theoretically leading to a conversion efficiency exceeding50%.In addition to such improvements, microfabrication technology for the integrated high-effi-ciency cells and the development of novel material systems that realizes high efficiency and low cost at the same time are investigated.Keywords Multi-junctionÁQuantum wellÁConcentratorÁPhotovoltaicINTRODUCTIONLarge-scale photovoltaic(PV)power generation systems, that achieve an ultra-high efficiency of40%or higher under high concentration,are in the spotlight as a new technology to ease drastically the energy problems.Mul-tiple junction(or tandem)solar cells that use epitaxial crystals of III–V compound semiconductors take on the active role for photoelectric energy conversion in such PV power generation systems.Because these solar cells operate under a sunlight concentration of5009to10009, the cost of cells that use the epitaxial crystal does not pose much of a problem.In concentrator PV,the increased cost for a cell is compensated by less costly focusing optics. The photons shining down on earth from the sun have a wide range of energy distribution,from the visible region to the infrared region,as shown in Fig.1.Multi-junction solar cells,which are laminated with multilayers of p–n junctions configured by using materials with different band gaps,show promise in absorbing as much of these photons as possible,and converting the photon energy into elec-tricity with minimum loss to obtain high voltage.Among the various types of multi-junction solar cells,indium gallium phosphide(InGaP)/gallium arsenide(GaAs)/ger-manium(Ge)triple-junction cells that make full use of the relationship between band gaps and diverse lattice con-stants offered by compound semiconductors have the advantage of high conversion efficiency because of their high-quality single crystal with a uniform-size crystal lat-tice.So far,a conversion efficiency exceeding41%under conditions where sunlight is concentrated to an intensity of approximately5009has been reported.The tunnel junction with a function equivalent to elec-trodes is inserted between different materials.The positive holes accumulated in the p layer and the electrons in the adjacent n layer will be recombined and eliminated in the tunnel junction.Therefore,three p–n junctions consisting of InGaP,GaAs,and Ge will become connected in series. The upper limit of the electric current is set by the mini-mum value of photonflux absorbed by a single cell.On the other hand,the sum of voltages of three cells make up the voltage.As shown in Fig.1,photons that can be captured in the GaAs middle cell have a smallflux because of the band gap of each material.As a result,the electric currentoutputAMBIO2012,41(Supplement2):125–131 DOI10.1007/s13280-012-0267-4from the GaAs cell theoretically becomes smaller than that of the others and determines the electric current output of the entire tandem cell.To develop a higher efficiency tandem cell,it is necessary to use a material with a band gap narrower than that of GaAs for the middle cell.In order to obtain maximum conversion efficiency for triple-junction solar cells,it is essential to narrow down the middle cell band gap to 1.2eV and increase the short-circuit current density by 2mA/cm 2compared with that of the GaAs middle cell.When the material is replaced with a narrower band gap,the output voltage will drop.However,the effect of improving the electric current balance out-performs this drop in output voltage and boosts the effi-ciency of the entire multi-junction cell.When a crystal with such a narrow band gap is grown on a Ge base material,lattice relaxation will occur in the middle of epitaxial crystal growth because the lattice constants of narrower band-gap materials are larger than that of Ge (as shown in Fig.2).As a result,the carrier transport properties will degrade due to dislocation.Researchers from the international research center Solar Quest,the University of Tokyo,aim to move beyond such material-related restrictions,and obtain materials and structures that have effective narrow band gaps while maintaining lattice matching with Ge or GaAs.To achieve this goal,we have taken three approaches as indicated in Fig.3.These approaches are explained in detail below.DILUTE NITROGEN-ADDED BULK CRYSTAL Indium gallium nitride arsenide (InGaNAs)is a bulk material consists of InGaAs,which contains several percent of nitrogen.InGaNAs has a high potential for achieving a narrow band gap while maintaining lattice matching with Ge or GaAs.However,InGaNAs has a fatal problem,that is,a drop in carrier mobility due to inhomogeneousdistribution of nitrogen (N).To achieve homogeneous solid solution of N in crystal,we have applied atomic hydrogen irradiation in the film formation process and addition of a very small amount of antimony (Sb)(Fig.3).The atomic hydrogen irradiation technology and the nitrogen radical irradiation technology for incorporating N efficiently into the crystal can be achieved only through molecular beam epitaxy (MBE),which is used to fabricate films under high vacuum conditions.(Nitrogen radical irradiation is a technology that irradiates the surface of a growing crystal with nitrogen atoms that are resolved by passing nitrogen through a plasma device attached to the MBE system.)Therefore,high-quality InGaNAs has been obtained only by MBE until now.Furthermore,as a small amount of Sb is also incorporated in a crystal,it is nec-essary to control the composition of five elements in the crystal with a high degree of accuracy to achieve lattice matching with Ge or GaAs.We have overcome this difficulty by optimizing the crystal growth conditions with high precision and devel-oped a cell that has an InGaNAs absorption layer formed on a GaAs substrate.The short-circuit current has increased by 9.6mA/cm 2for this cell,compared with a GaAs single-junction cell,by narrowing the band gap down to 1.0eV.This technology can be implemented not only for triple-junction cells,but also for higher efficiency lattice-matched quadruple-junction cells on a Ge substrate.In order to avoid the difficulty of adjusting the compo-sition of five elements in a crystal,we are also taking an approach of using GaNAs with a lattice smaller than that of Ge or GaAs for the absorption layer and inserting InAs with a large lattice in dot form to compensate for the crystal’s tensile strain.To make a solid solution of N uniformly in GaNAs,we use the MBE method for crystal growth and the atomic hydrogen irradiation as in the case of InGaNAs.We also believe that using 3D-shaped InAs dots can effectively compensate for the tensile strainthatFig.1Solar spectrum radiated on earth and photon flux collected by the top cell (InGaP),middle cell (GaAs),and bottom cell (Ge)(equivalent to the area of the filled portions in the figure)occurs in GaNAs.We have measured the characteristics of a single-junction cell formed on a GaAs substrate by using a GaNAs absorption layer with InAs dots inserted.Figure 4shows that we were able to succeed in enhancing the external quantum efficiency in the long-wavelength region (corresponding to the GaNAs absorp-tion)to a level equal to GaAs.This was done by extending the absorption edge to a longer wavelength of 1200nm,and increasing the thickness of the GaNAs layer by increasing the number of laminated InAs quantum dot layers.This high quantum efficiency clearly indicates that GaNAs with InAs dots inserted has the satisfactory quality for middle cell material (Oshima et al.2010).STRAIN-COMPENSATED QUANTUM WELL STRUCTUREIt is extremely difficult to develop a narrow band-gap material that can maintain lattice matching with Ge orGaAs unless dilute nitrogen-based materials mentioned earlier are used.As shown in Fig.2,the conventionally used material InGaAs has a narrower band gap and a larger lattice constant than GaAs.Therefore,it is difficult to grow InGaAs with a thickness larger than the critical film thickness on GaAs without causing lattice relaxation.However,the total film thickness of InGaAs can be increased as an InGaAs/GaAsP strain-compensated multi-layer structure by laminating InGaAs with a thickness less than the critical film thickness in combination with GaAsP that is based on GaAs as well,but has a small lattice constant,and bringing the average strain close to zero (Fig.3.).This InGaAs/GaAsP strain-compensated multilayer structure will form a quantum well-type potential as shown in Fig.5.The narrow band-gap InGaAs layer absorbs the long-wavelength photons to generate electron–hole pairs.When these electron–hole pairs go over the potential bar-rier of the GaAsP layer due to thermal excitation,the electrons and holes are separated by a built-in electricfieldFig.2Relationship between band gaps and lattice constants of III–V-based and IV-based crystalsto generate photocurrent.There is a high probability of recombination of electron–hole pairs that remain in the well.To avoid this recombination,it is necessary to take out the electron–hole pairs efficiently from the well and transfer them to n-type and p-type regions without allowing them to be recaptured into the well.Designing thequantumFig.3Materials and structures of narrow band-gap middle cells being researched by thisteamFig.4Spectral quantum efficiency of GaAs single-junction cell using GaNAs bulk crystal layer (inserted with InAs dots)as the absorption layer:Since the InAs dot layer and the GaNAs bulk layer are stacked alternately,the total thickness of GaNAs layers increases as the number of stacked InAs dot layers is increased.The solid line in the graph indicates the data of a reference cell that uses GaAs for its absorption layer (Oshima et al.2010)well structure suited for this purpose is essential for improving conversion efficiency.The high-quality crystal growth by means of the metal-organic vapor phase epitaxy (MOVPE)method with excellent ability for mass production has already been applied for InGaAs and GaAsP layers in semiconductor optical device applications.Therefore,it is technologically quite possible to incorporate the InGaAs/GaAsP quantum well structure into multi-junction solar cells that are man-ufactured at present,only if highly accurate strain com-pensation can be achieved.As the most basic approach related to quantum well structure design,we are working on fabrication of super-lattice cells with the aim of achieving higher efficiency by making the GaAsP barrier layer as thin as possible,and enabling carriers to move among wells by means of the tunnel effect.Figure 6shows the spectral quantum effi-ciency of a superlattice cell.In this example,the thickness of the GaAsP barrier layer is 5nm,which is not thin enough for proper demonstration of the tunnel effect.When the quantum efficiency in the wavelength range (860–960nm)that corresponds to absorption of the quan-tum well is compared between a cell,which has a con-ventionally used barrier layer and a thickness of 10nm or more,and a superlattice cell,which has the same total layer thickness of InGaAs,the superlattice cell demonstrates double or higher quantum efficiency.This result indicates that carrier mobility across quantum wells is promoted by even the partial use of the tunnel effect.By increasing the P composition in the GaAsP layer,the thickness of well (or the In composition)can be increased,and the barrier layer thickness can be reduced while strain compensation is maintained.A cell with higher quantum efficiency can befabricated while extending the absorption edge to the long-wavelength side (Wang et al.2010,2012).GROWTH TECHNIQUE FOR STRAIN-COMPENSATED QUANTUM WELLTo reduce the strain accumulated in the InGaAs/GaAsP multilayer structure as close to zero as possible,it is nec-essary to control the thickness and atomic content of each layer with high accuracy.The In composition and thickness of the InGaAs layer has a direct effect on the absorption edge wavelength and the GaAsP layer must be thinned to a satisfactory extent to demonstrate fully the tunnel effect of the barrier layer.Therefore,it is desirable that the average strain of the entire structure is adjusted mainly by the P composition of the GaAsP layer.Meanwhile,for MOVPE,there exists a nonlinear rela-tionship between the P composition of the crystal layer and the P ratio [P/(P ?As)]in the vapor phase precursors,which arises from different absorption and desorption phenomena on the surface.As a result,it is not easy to control the P composition of the crystal layer.To break through such a difficulty and promote efficient optimiza-tion of crystal growth conditions,we have applied a mechanism to evaluate the strain of the crystal layer during growth in real time by sequentially measuring the curvature of wafers during growth with an incident laser beam from the observation window of the reactor.As shown in Fig.7,the wafer curvature during the growth of an InGaAs/GaAsP multilayer structure indicates a periodic behavior.Based on a simple mechanical model,it has become clear that the time changes ofwaferFig.5Distribution of potential formed by the InGaAs/GaAsP strain-compensated multilayer structure:the narrow band-gap InGaAs layer is sandwiched between wide band-gap GaAsP layers and,as a result,it as quantum well-type potential distribution.In the well,electron–hole pairs are formed by absorption of long-wavelength photons and at the same time,recombination of electrons and holes takes place.The team from Solar Quest is focusing on developing a superlattice structure with the thinnest GaAsP barrier layercurvature are proportionate to the strain of the crystal layer relative to a substrate during the growing process.One vibration cycle of the curvature is same as the growth time of an InGaAs and GaAsP pair (Sugiyama et al.2011).Therefore,the observed vibration of the wafer curvature reflects the accumulation of the compression strain that occurs during InGaAs growth and the release of the strain that occurs during GaAsP growth.When the strain is completely compensated,the growth of the InGaAs/GaAsP pair will cause this strain to return to the initial value and the wafer curvature will vibrate with the horizontal line as the center.As shown in Fig.7,strain can be compensated almost completely by adjusting the layer structure.Only by conducting a limited number of test runs,the use of such real-time observation technology of the growth layer enables setting the growth conditions for fabricating the layer structure for which strain has been compensated with highaccuracy.Fig.6Spectral quantum efficiency of GaAs single-junction cell using InGaAs/GaAsP superlattice as theabsorption layer:This structure consists of 60layers of InGaAs quantum wells.The graph also shows data of a reference cell that uses GaAs for its absorption layer (Wang et al.2010,2012)Fig.7Changes in wafer curvature over time during growth of the InGaAs/GaAsP multilayer structure.This graph indicates the measurement result and the simulation result of the curvature based on the layer structure(composition ?thickness)obtained by X-ray diffraction.Since compressive strain is applied during InGaAs growth,the curvature decreases as time passes.On the other hand,since tensile strain is applied during GaAsP growth,the curvature changes in the oppositedirection (Sugiyama et al.2011)FUTURE DIRECTIONSIn order to improve the conversion efficiency by enhancing the current matching of multi-junction solar cells using III–V compound semiconductors,there is an urgent need to create semiconductor materials or structures that can maintain lattice matching with Ge or GaAs,and have a band gap of1.2eV.As for InGaNAs,which consists of InGaAs with several percent of nitrogen added,we have the prospect of extending the band edge to1.0eV while retaining sufficient carrier mobility for solar cells by means of atomic hydrogen irradiation and application of a small quantity of Sb during the growth process.In addition,as for GaNAs bulk crystal containing InAs dots,we were able to extend the band edge to1.2eV and produce a high-quality crystal with enoughfilm thickness to achieve the quantum efficiency equivalent to that of GaAs.These crystals are grown by means of MBE. Therefore,measures that can be used to apply these crys-tals for mass production,such as migration to MOVPE, will be investigated after demonstrating their high effi-ciency by embedding these crystals into multi-junction cells.As for the InGaAs/GaAsP strain-compensated quantum well that can be grown using MOVPE,we are working on the development of a thinner barrier layer while compen-sating for the strain with high accuracy by real-time observation of the wafer curvature.We have had the prospect of achieving a quantum efficiency that will sur-pass existing quantum well solar cells by promoting the carrier transfer within the multilayer quantum well struc-ture using the tunnel effect.As this technology can be transferred quite easily to the existing multi-junction solar cell fabrication process,we strongly believe that this technology can significantly contribute to the efficiency improvement of the latest multi-junction solar cells. REFERENCESOshima,R.,A.Takata,Y.Shoji,K.Akahane,and Y.Okada.2010.InAs/GaNAs strain-compensated quantum dots stacked up to50 layers for use in high-efficiency solar cell.Physica E42: 2757–2760.Sugiyama,M.,K.Sugita,Y.Wang,and Y.Nakano.2011.In situ curvature monitoring for metalorganic vapor phase epitaxy of strain-balanced stacks of InGaAs/GaAsP multiple quantum wells.Journal of Crystal Growth315:1–4.Wang,Y.,Y.Wen,K.Watanabe,M.Sugiyama,and Y.Nakano.2010.InGaAs/GaAsP strain-compensated superlattice solar cell for enhanced spectral response.In Proceedings35th IEEE photovoltaic specialists conference,3383–3385.Wang,Y.P.,S.Ma,M.Sugiyama,and Y.Nakano.2012.Management of highly-strained heterointerface in InGaAs/GaAsP strain-balanced superlattice for photovoltaic application.Journal of Crystal Growth.doi:10.1016/j.jcrysgro.2011.12.049. AUTHOR BIOGRAPHYYoshiaki Nakano(&)is Professor and Director General of Research Center for Advanced Science and Technology,the University of Tokyo.His research interests include physics and fabrication tech-nologies of semiconductor distributed feedback lasers,semiconductor optical modulators/switches,monolithically integrated photonic cir-cuits,and high-efficiency heterostructure solar cells.Address:Research Center for Advanced Science and Technology, The University of Tokyo,4-6-1Komaba,Meguro-ku,Tokyo153-8904,Japan.e-mail:nakano@rcast.u-tokyo.ac.jp。