隧道毕业设计应该考虑应力释放的影响-外文翻译
隧道毕业设计
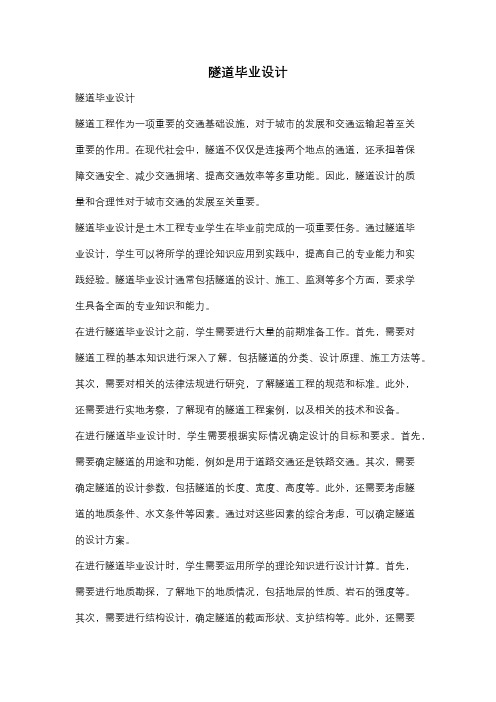
隧道毕业设计隧道毕业设计隧道工程作为一项重要的交通基础设施,对于城市的发展和交通运输起着至关重要的作用。
在现代社会中,隧道不仅仅是连接两个地点的通道,还承担着保障交通安全、减少交通拥堵、提高交通效率等多重功能。
因此,隧道设计的质量和合理性对于城市交通的发展至关重要。
隧道毕业设计是土木工程专业学生在毕业前完成的一项重要任务。
通过隧道毕业设计,学生可以将所学的理论知识应用到实践中,提高自己的专业能力和实践经验。
隧道毕业设计通常包括隧道的设计、施工、监测等多个方面,要求学生具备全面的专业知识和能力。
在进行隧道毕业设计之前,学生需要进行大量的前期准备工作。
首先,需要对隧道工程的基本知识进行深入了解,包括隧道的分类、设计原理、施工方法等。
其次,需要对相关的法律法规进行研究,了解隧道工程的规范和标准。
此外,还需要进行实地考察,了解现有的隧道工程案例,以及相关的技术和设备。
在进行隧道毕业设计时,学生需要根据实际情况确定设计的目标和要求。
首先,需要确定隧道的用途和功能,例如是用于道路交通还是铁路交通。
其次,需要确定隧道的设计参数,包括隧道的长度、宽度、高度等。
此外,还需要考虑隧道的地质条件、水文条件等因素。
通过对这些因素的综合考虑,可以确定隧道的设计方案。
在进行隧道毕业设计时,学生需要运用所学的理论知识进行设计计算。
首先,需要进行地质勘探,了解地下的地质情况,包括地层的性质、岩石的强度等。
其次,需要进行结构设计,确定隧道的截面形状、支护结构等。
此外,还需要进行施工工艺设计,确定隧道的施工方法和工期等。
通过这些设计计算,可以确保隧道的安全性和可行性。
在进行隧道毕业设计时,学生还需要考虑隧道的环境影响和可持续发展。
隧道工程对于周边环境的影响是不可忽视的,包括噪音、震动、空气污染等。
因此,在进行隧道设计时,需要考虑如何减少这些影响,保护周边环境的安全和舒适。
此外,还需要考虑如何提高隧道的可持续性,包括节能减排、资源循环利用等方面。
外文翻译--机器零件的设计
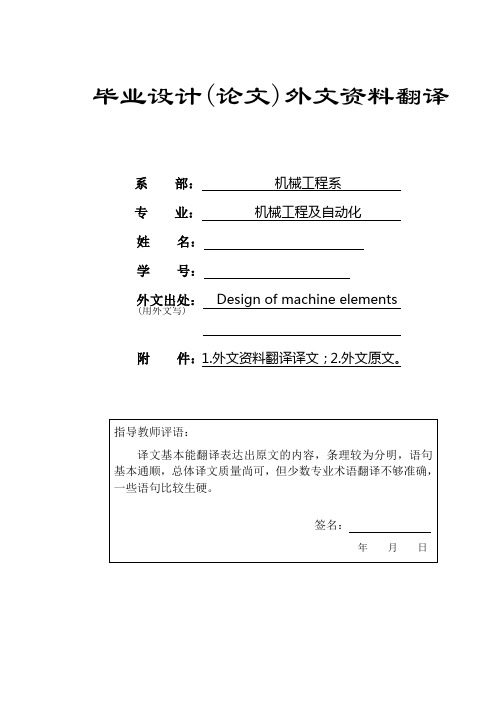
毕业设计(论文)外文资料翻译系部:机械工程系专业:机械工程及自动化姓名:学号:外文出处:Design of machine elements(用外文写)附件:1.外文资料翻译译文;2.外文原文。
附件1:外文资料翻译译文机器零件的设计相同的理论或方程可应用在一个一起的非常小的零件上,也可用在一个复杂的设备的大型相似件上,既然如此,毫无疑问,数学计算是绝对的和最终的。
他们都符合不同的设想,这必须由工程量决定。
有时,一台机器的零件全部计算仅仅是设计的一部分。
零件的结构和尺寸通常根据实际考虑。
另一方面,如果机器和昂贵,或者质量很重要,例如飞机,那么每一个零件都要设计计算。
当然,设计计算的目的是试图预测零件的应力和变形,以保证其安全的带动负载,这是必要的,并且其也许影响到机器的最终寿命。
当然,所有的计算依赖于这些结构材料通过试验测定的物理性能。
国际上的设计方法试图通过从一些相对简单的而基本的实验中得到一些结果,这些试验,例如结构复杂的及现代机械设计到的电压、转矩和疲劳强度。
另外,可以充分证明,一些细节,如表面粗糙度、圆角、开槽、制造公差和热处理都对机械零件的强度及使用寿命有影响。
设计和构建布局要完全详细地说明每一个细节,并且对最终产品进行必要的测试。
综上所述,机械设计是一个非常宽的工程技术领域。
例如,从设计理念到设计分析的每一个阶段,制造,市场,销售。
以下是机械设计的一般领域应考虑的主要方面的清单:①最初的设计理念②受力分析③材料的选择④外形⑤制造⑥安全性⑦环境影响⑧可靠性及寿命在没有破坏的情况下,强度是抵抗引起应力和应变的一种量度。
这些力可能是:①渐变力②瞬时力③冲击力④不断变化的力⑤温差如果一个机器的关键件损坏,整个机器必须关闭,直到修理好为止。
设计一台新机器时,关键件具有足够的抵抗破坏的能力是非常重要的。
设计者应尽可能准确地确定所有的性质、大小、方向及作用点。
机器设计不是这样,但精确的科学是这样,因此很难准确地确定所有力。
日本隧道维修毕业论文外文文献翻译
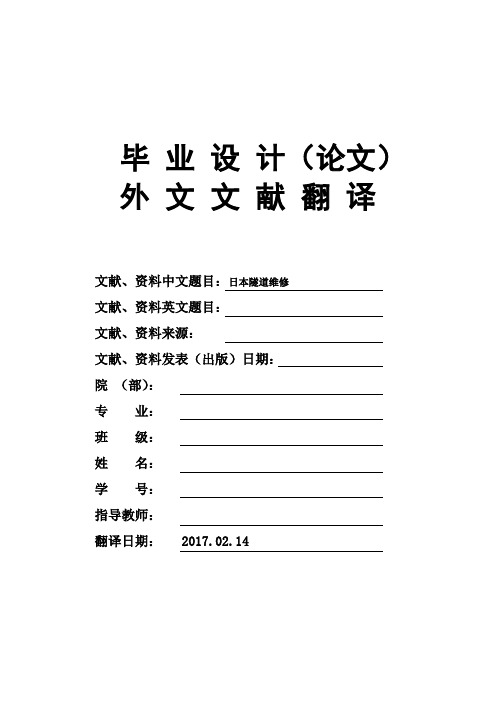
毕业设计(论文)外文文献翻译文献、资料中文题目:日本隧道维修文献、资料英文题目:文献、资料来源:文献、资料发表(出版)日期:院(部):专业:班级:姓名:学号:指导教师:翻译日期: 2017.02.14日本隧道维修Tunnel maintenance in Japan摘要本文论述了日本铁路隧道最近的维修技术和典型的变形情况。
检测隧道衬砌分为初级检查和辅助检查,在可行的检查中本文引进了无损检测的新技术。
修复和加强隧道变形的方法可分为:(一)土压力的对策(二)恶化衬里;(三)防渗漏水和冰霜伤害的应对措施;(4)防止剥落的应对措施。
此外,本文介绍了三个近期典型的日本铁路隧道变形案件;其中之一是Tukayama隧道对塑料的土压力,另一个是关于福冈隧道和Rebunhama隧道由于衬砌剥落造成的事故。
关键词:隧道维修,隧道检查;隧道修复,加固,无损检测1 引言隧道不同于地上建筑物,设计条件(地形,地质,地下水等)依变化情况而定。
因此,它是不容易在各种类型的地形中合理设计和建造,而且它在使用过程中会发生不确定的变形。
由于这些原因,隧道的养护和控制就显得很重要,为保持隧道的良好状态,可采用以下方法:如定期检查,隧道声音的正确判断,以及相应的应对措施。
变形的因素,可分为三组,即:(1)由于地质因素的土压力;(2)劣化的衬里材料;以及(3)漏水和霜冻损害(朝仓等人,1991年)。
1999年,在福冈北九州隧道沿山阳新干线(子弹头列车)和室兰线的Rebunhama隧道发生了混凝土衬砌剥落的的事件。
剥落,在各项建设工程中,已成为一个重要问题。
在本文,对隧道衬砌审查和变形的对策做了调查和评估技术,并介绍了一些典型的例子。
2 检查和诊断方法2.1 隧道的检查和诊断对隧道进行检查和诊断,能及时掌握变形是否影响结构的安全性和耐久性,然后采取适当的应对措施,以确保在评估结果的基础上保持隧道的良好状况。
因此,隧道的诊断和检查,是隧道维护管理最基础的部分。
隧道盾构-毕设论文外文翻译(翻译-原文)
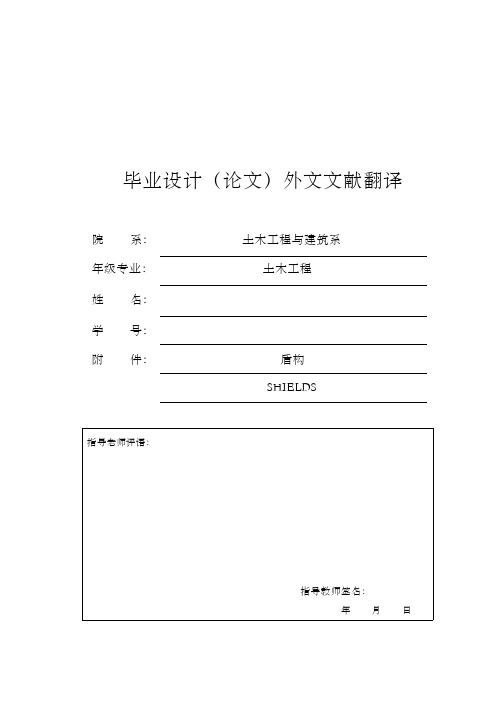
毕业设计(论文)外文文献翻译院系:土木工程与建筑系年级专业:土木工程姓名:学号:附件:盾构SHIELDS指导老师评语:指导教师签名:年月日S HIEL D S【Abstr act】A tunnel shield is a structural system, used during the face excavation process. The paper mainly discusses the form and the structure of the shield. Propulsion for the shield is provided by a series of hydraulic jacks installed in the tail of the shield and the shield is widespread used in the underground environment where can not be in long time stable. The main enemy of the shield is ground pressure. Non-uniform ground pressure caused by the steering may act on the skin tends to force the shield off line and grade. And working decks inside the shield enable the miners to excavate the face, drill and load holes.【Keywor ds】shield hydraulic jacks ground pressure steering working decksA tunnel shield is a structural system, normally constructed of steel, used during the face excavation process. The shield has an outside configuration which matches the tunnel. The shield provides protection for the men and equipment and also furnished initial ground support until structural supports can be installed within the tail section of the shield. The shield also provides a reaction base for the breast-board system used to control face movement. The shield may have either an open or closed bottom. In a closed-bottom shield, the shield structure and skin provide 360-degree ground contact and the weight of the shield rests upon the invert section of the shield skin. The open shield has no bottom section and requires some additional provision is a pair of side drifts driven in advance of shield excavation. Rails or skid tracks are installed within these side drifts to provide bearing support for the shield.Shield length generally varies from1/2 to 3/4 of the tunnel diameter. The front of the shield is generally hooded to so that the top of the shield protrudes forward further than the invert portion which provides additional protection for the men working at the face and also ease pressure on the breast-boards. The steel skin of the shield may varyfrom 1.3 to 10 cm in thickness, depending on the expected ground pressures. The type of steel used in the shield is the subject of many arguments within the tunneling fraternity. Some prefer mild steel in the A36 category because of its ductility and case of welding in the underground environment where precision work is difficult. Others prefer a high-strength steel such as T-1 because of its higher strength/w eight ratio. Shield weight may range from 5 to 500 tons. Most of the heaviest shields are found in the former Sovier Union because of their preference for cast-iron in both structural and skin elements.Propulsion for the shield is provided by a series of hydraulic jacks installed in the tail of the shield that thrust against the last steel set that has been installed. The total required thrust will vary with skin area and ground pressure. Several shields have been constructed with total thrust capabilities in excess of 10000 tons. Hydraulic systems are usually self-contained, air-motor powered, and mounted on the shield. Working pressures in the hydraulic system may range from 20-70 Mpa. To resist the thrust of the shield jacks, a horizontal structure member (collar brace) must be installed opposite each jack location and between the flanges of the steel set. In addition, some structural provision must be made for transferring this thrust load into the tunnel walls. Without this provision the thrust will extend through the collar braces to the tunnel portal.An Englishman, Marc Brunel, is credited with inventing the shield. Brunel supposedly got his idea by studying the action of the Teredo navalis, a highly destructive woodworm, when he was working at the Chatham dock yard. In 1818 Brunel obtained an English patent for his rectangular shield which was subsequently uses to construct the first tunnel under the River Thames in London. In 1869 the first circular shield was devised by Barlow and Great Head in London and is referred to as the Great Head-type shield. Later that same year, Beach in New York City produced similar shield. The first use of the circular shield came during 1869 when Barlow and Great Head employed their device in the construction of the 2.1 in diameter Tower Subway under the River Thames. Despite the name of the tunnel, it was used only for pedestrian traffic. Beach also put his circular shield to work in 1869 to construct a demonstration project for a proposed NewYork City subway system. The project consisted of a 2.4 m diameter tunnel, 90 m long, used to experiment with a subway car propelled by air pressure.Here are some tunnels which were built by shield principle.Soft-ground tunneling Some tunnels are driven wholly or mostly through soft material. In very soft ground, little or no blasting is necessary because the material is easily excavated.At first, forepoling was the only method for building tunnels through very soft ground. Forepoles are heavy planks about 1.5 m long and sharpened to a point. They were inserted over the top horizontal bar of the bracing at the face of the tunnel. The forepoles were driven into the ground of the face with an outward inclination. After all the roof poles were driven for about half of their length, a timber was laid across their exposed ends to counter any strain on the outer ends. The forepoles thus provided an extension of the tunnel support, and the face was extended under them. When the ends of the forepoles were reached, new timbering support was added, and the forepoles were driven into the ground for the next advance of the tunneling.The use of compressed air simplified working in soft ground. An airlock was built, though which men and equipment passed, and sufficient air pressure was maintained at the tunnel face to hold the ground firm during excavation until timbering or other support was erected.Another development was the use of hydraulically powered shields behind which cast-iron or steel plates were placed on the circumference of the tunnels. These plates provided sufficient support for the tunnel while the work proceeded, as well as full working space for men in the tunnel.Under water tunneling The most difficult tunneling is that undertaken at considerable depths below a river or other body of water. In such cases, water seeps through porous material or crevices, subjecting the work in progress to the pressure of the water above the tunneling path. When the tunnel is driven through stiff clay, the flow of water may be small enough to be removed by pumping. In more porous ground,compressed air must be used to exclude water. The amount of air pressure that is needed increases as the depth of the tunnel increases below the surface.A circular shield has proved to be most efficient in resisting the pressure of soft ground, so most shield-driven tunnels are circular. The shield once consisted of steel plates and angle supports, with a heavily braced diaphragm across its face. The diaphragm had a number of openings with doors so that workers could excavate material in front of the shield. In a further development, the shield was shoved forward into the silty material of a riverbed, thereby squeezing displaced material through the doors and into the tunnel, from which the muck was removed. The cylindrical shell of the shield may extend several feet in front of the diaphragm to provide a cutting edge. A rear section, called the tail, extends for several feet behind the body of the shield to protect workers. In large shields, an erector arm is used in the rear side of the shield to place the metal support segments along the circumference of the tunnel.The pressure against the forward motion of a shield may exceed 48.8 Mpa. Hydraulic jacks are used to overcome this pressure and advance the shield, producing a pressure of about 245 Mpa on the outside surface of the shield.Shields can be steered by varying the thrust of the jacks from left side to right side or from top to bottom, thus varying the tunnel direction left or right or up or down. The jacks shove against the tunnel lining for each forward shove. The cycle of operation is forward shove, line, muck, and then another forward shove. The shield used about 1955 on the third tube of the Lincoln Tunnel in New York City was 5.5 m long and 9.6 m in diameter. It was moved about 81.2 cm per shove, permitting the fabrication of a 81.2 cm support ring behind it.Cast-iron segments commonly are used in working behind such a shield. They are erected and bolted together in a short time to provide strength and water tightness. In the third tube of the Lincoln Tunnel each segment is 2 m long, 81.2 cm wide, and 35.5 cm thick, and weighs about 1.5 tons. These sections form a ring of 14 segments that are linked together by bolts. The bolts were tightened by hand and then by machine.Immediately after they were in place, the sections were sealed at the joints to ensure permanent water tightness.Shields are most commonly used in ground condition where adequate stand-up time does not exist. The advantage of the shield in this type of ground, in addition to the protection afforded men and equipment , is the time available to install steel ribs, liner plates, or precast concrete segments under the tail segment of the shield before ground pressure and movement become adverse factors.One of the principle problems associated with shield use is steering. Non-uniform ground pressure acting on the skin tends to force the shield off line and grade. This problem is particularly acute with closed bottom shield that do not ride on rails or skid tracks. Steering is accomplished by varying the hydraulic pressure in individual thrust jacks. If the shied is trying to dive, additional pressure on the invert jacks will resist this tendency. It is not unusual to find shield wandering several feet from the required. Although lasers are frequently used to provide continuous line and grade data to operator, once the shield wanders off its course, its sheer bulk resists efforts to bring it back. Heterogeneous ground conditions, such as clay with random boulders, also presents steering problems.One theoretical disadvantage of the shield is the annular space left between the support system and the ground surface. When the support system is installed within the tail section of the shield, the individual support members are separated from the ground surface by the thickness of the tail skin. When steel ribs are used, the annular space is filled with timber blocking as the forward motion of the shield exposes the individual ribs.A continuous support system presents a different problem. In this case, a filler material, such as pea gravel or grout, is pumped behind the support system to fill the void between it and the ground surface.The main enemy of the shield is ground pressure. As ground pressure begins to build, two things happen, more thrust is required for shield propulsion and stress increases in the structural members of the shield. Shields are designed and function undera preselected ground pressure. Designers will select this pressure as a percentage of the maximum ground pressure contemplated by the permanent tunnel design. In some cases, unfortunately, the shield just gets built without specific consideration of the ground pressures it might encounter. When ground pressure exceeds the design limit, the shield gets “stuck”.The friction component of the ground pressure on the skin becomes greater than the thrust capability of the jacks. Several methods, including pumping bentonite slurry into the skin, ground interface, pushing heavy equipment, and bumping with dynamite, have been applied to stuck shields with occasional success.Because ground pressure tends to increase with time, the cardinal rule of operation is “keeping moving”.This accounts for the fracture activity when a shield has suffered a temporary mechanical failure. As ground pressure continues to build on the nonmoving shield , the load finally exceeds its structural limit and bucking begins. An example of shield destruction occurred in California in 1968 when two shields being used to drive the Carly V.Porter Tunnel were caught by excessive ground pressure and deformed beyond repair. One of the Porter Tunnel shields was brought to a halt in reasonably good ground by water bearing ground fault that required full breast-boards. While the contractor was trying to bring the face under control, skin pressure began to increase. While the face condition finally stabilized, the contractor prepared to resume operations and discovered the shield was stuck. No combination of methods was able to move it, and the increasing ground pressure destroyed the shield.To offset the ground pressure effect, a standard provision in design is a cutting edge radius several inches greater than the main body radius. This allows a certain degree o f ground movement before pressure can come to bear on the shield skin. Another approach, considered in theory but not yet put into practice, is the “w atermelon seed”design. The theory calls for a continuous taper in the shield configuration; maximum radius at the cutting edge and the minimum radius at the trailing edge of the tail. With this configuration, any amount of forward movement would create a drop in skin pressure.Working decks, spaced 2.4 to 3.0 m vertically, are provided inside the shield. These working decks enable the miners to excavate the face, drill and load holes, if necessary, and adjust the breast-board system. The hydraulic jacks for the breast-board are mounted on the underside of the work decks. Blast doors are sometimes installed as an integral part of the work decks if a substantial amount of blasting is expected.Some form of mechanical equipment is provided on the rear end of the working decks to assist the miners in handing and placing the element of the support system. In large tunnels, these individual support elements can weigh several tons and mechanical assistance becomes essential. Sufficient vertical clearance must be provided between the invert and the first working deck to permit access to the face by the loading equipment.盾构【摘要】隧道盾构是一结构系统,通常用于洞室开挖。
预应力混凝土Prestressed-Concrete大学毕业论文外文文献翻译及原文
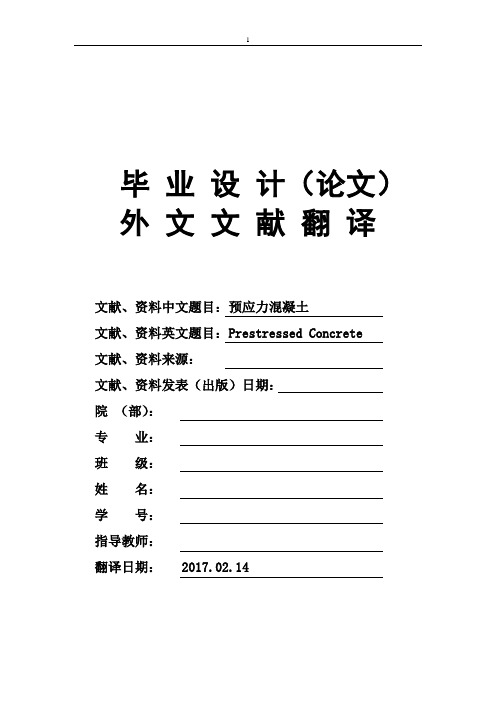
毕业设计(论文)外文文献翻译文献、资料中文题目:预应力混凝土文献、资料英文题目:Prestressed Concrete文献、资料来源:文献、资料发表(出版)日期:院(部):专业:班级:姓名:学号:指导教师:翻译日期: 2017.02.14毕业设计(论文)外文资料翻译外文出处:The Concrete structure附件:1、外文原文;2、外文资料翻译译文。
1、外文资料原文Prestressed ConcreteConcrete is strong in compression, but weak in tension: Its tensile strength varies from 8 to 14 percent of its compressive strength. Due tosuch a Iow tensile capacity, fiexural cracks develop at early stages ofloading. In order to reduce or prevent such cracks from developing, aconcentric or eccentric force is imposed in the longitudinal direction of the structural element. This force prevents the cracks from developing by eliminating or considerably reducing the tensile stresses at thecritical midspan and support sections at service load, thereby raising the bending, shear, and torsional capacities of the sections. The sections are then able to behave elastically, and almost the full capacity of the concrete in compression can be efficiently utilized across the entire depth of the concrete sections when all loads act on the structure.Such an imposed longitudinal force is called a prestressing force,i.e., a compressive force that prestresses the sections along the span ofthe structural elementprior to the application of the transverse gravitydead and live loads or transient horizontal live loads. The type ofprestressing force involved, together with its magnitude, are determined mainly on the basis of the type of system to be constructed and the span length and slenderness desired.~ Since the prestressing force is applied longitudinally along or parallel to the axis of the member, the prestressing principle involved is commonly known as linear prestressing.Circular prestressing, used in liquid containment tanks, pipes,and pressure reactor vessels, essentially follows the same basic principles as does linear prestressing. The circumferential hoop, or "hugging" stress on the cylindrical or spherical structure, neutralizes the tensile stresses at the outer fibers of the curvilinear surface caused by the internal contained pressure.Figure 1.2.1 illustrates, in a basic fashion, the prestressing action in both types of structural systems and the resulting stress response. In(a), the individual concrete blocks act together as a beam due to the large compressive prestressing force P. Although it might appear that the blocks will slip and vertically simulate shear slip failure, in fact they will not because of the longitudinal force P. Similarly, the wooden staves in (c) might appear to be capable of separating as a result of the high internal radial pressure exerted on them. But again, because of the compressive prestress imposed by the metal bands as a form of circular prestressing, they will remain in place.From the preceding discussion, it is plain that permanent stresses in the prestressed structural member are created before the full dead and live loads are applied in order to eliminate or considerably reduce the net tensile stresses caused by these loads. With reinforced concrete,it is assumed that the tensile strength of the concrete is negligible and disregarded. This is because the tensile forces resulting from the bending moments are resisted bythe bond created in the reinforcement process. Cracking and deflection are therefore essentially irrecoverable in reinforced concrete once the member has reached its limit state at service load.The reinforcement in the reinforced concrete member does not exert any force of its own on the member, contrary to the action of prestressing steel. The steel required to produce the prestressing force in the prestressed member actively preloads the member, permitting a relatively high controlled recovery of cracking and deflection. Once the flexural tensile strength of the concrete is exceeded, the prestressed member starts to act like a reinforced concrete element.Prestressed members are shallower in depth than their reinforced concrete counterparts for the same span and loading conditions. In general, the depth of a prestressed concrete member is usually about 65 to 80 percent of the depth of the equivalent reinforced concrete member. Hence, the prestressed member requires less concrete, and,about 20 to 35 percent of the amount of reinforcement. Unfortunately, this saving in material weight is balanced by the higher cost of the higher quality materials needed in prestressing. Also, regardless of the system used, prestressing operations themselves result in an added cost: Formwork is more complex, since the geometry of prestressed sections is usually composed of. flanged sections with thin-webs.In spite of these additional costs, if a large enough number of precast units are manufactured, the difference between at least the initial costs of prestressed and reinforced concrete systems is usually not very large.~ And the indirect long-term savings are quite substantial, because less maintenance is needed; a longer working life is possible due to better quality control of the concrete, and lighter foundations are achieved due to the smaller cumulative weight of the superstructure.Once the beam span of reinforced concrete exceeds 70 to 90 feet (21.3 to 27.4m), the dead weight of the beam becomes excessive, resulting in heavier members and, consequently, greater long-term deflection and cracking. Thus, for larger spans, prestressed concrete becomes mandatory since arches are expensive to construct and do not perform as well due to the severe long-term shrinkage and creep they undergo.~ Very large spans such as segmental bridges or cable-stayed bridges can only be constructed through the use of prestressing.Prestressd concrete is not a new concept, dating back to 1872, when P. H. Jackson, an engineer from California, patented a prestressing system that used a tie rod to construct beams or arches from individual blocks [see Figure 1.2.1 (a)]. After a long lapse of time during which little progress was made because of the unavailability of high-strength steel to overcome prestress losses, R. E. Dill of Alexandria, Nebraska, recognized the effect of the shrinkage and creep (transverse material flow) of concrete on the loss of prestress. He subsequently developed the idea that successive post-tensioning of unbonded rods would compensate for the time-dependent loss of stress in the rods due to the decrease in the length of the member because of creep and shrinkage. In the early 1920s,W. H. Hewett of Minneapolis developed the principles of circular prestressing. He hoop-stressed horizontal reinforcement around walls of concrete tanks through the use of turnbuckles to prevent cracking due to internalliquid pressure, thereby achieving watertightness. Thereafter, prestressing of tanks and pipes developed at an accelerated pace in the United States, with thousands of tanks for water, liquid, and gas storage built and much mileage of prestressed pressure pipe laid in the two to three decades that followed.Linear prestressing continued to develop in Europe and in France, in particular through the ingenuity of Eugene Freyssinet, who proposed in 1926--1928 methods to overcome prestress losses through the use of high-strength and high-ductility steels. In 1940, he introduced thenow well-known and well-accepted Freyssinet system.P. W. Abeles of England introduced and developed the concept of partial prestressing between the 1930s and 1960s. F. Leonhardt of Germany, V. Mikhailov of Russia, and T. Y. Lin of the United States also contributed a great deal to the art and science of the design of prestressed concrete. Lin's load-balancing method deserves particular mention in this regard, as it considerably simplified the design process, particularly in continuous structures. These twentieth-century developments have led to the extensive use of prestressing throughoutthe world, and in the United States in particular.Today, prestressed concrete is used in buildings, underground structures, TV towers, floating storage and offshore structures, power stations, nuclear reactor vessels, and numerous types of bridge systems including segn~ental and cable-stayed bridges, they demonstrate the versatility of the prestressing concept and its all-encompassing application. The success in the development and construction of all these structures has been due in no small measures to the advances in the technology of materials, particularly prestressing steel, and the accumulated knowledge in estimating the short-and long-term losses in the prestressing forces.~2、外文资料翻译译文预应力混凝土混凝土的力学特性是抗压不抗拉:它的抗拉强度是抗压强度的8%一14%。
西安工业大学建工院土木工程毕业设计总说明书外文文献翻译
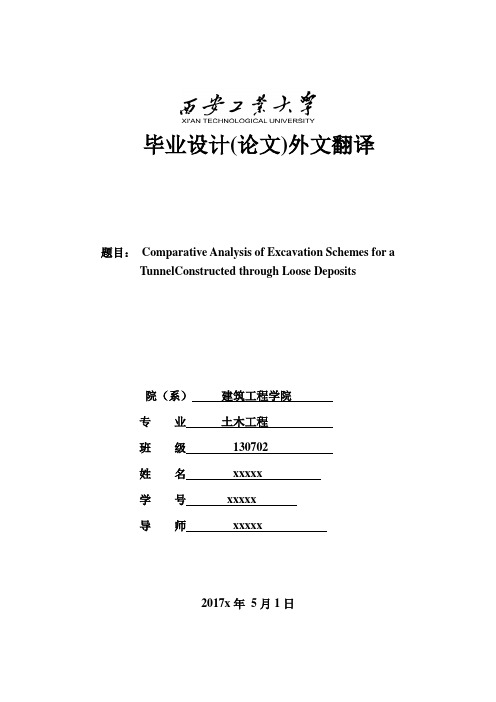
毕业设计(论文)外文翻译题目:Comparative Analysis of Excavation Schemes for a TunnelConstructed through Loose Deposits院(系)建筑工程学院专业土木工程班级130702姓名xxxxx学号xxxxx导师xxxxx2017x年5月1日通过松散堆积物构建了隧道开挖方案的对比分析摘要:由于周围岩石较弱,构造松散沉积物的隧道易于坍塌,二次内衬通常遭受过度变形。
因此,选择适当的挖掘方案是重要的,这将对隧道施工安全和随后的隧道运行产生影响。
本文采用亭子坝隧道,一条浅埋在浅沉积和冲积起源的高速公路隧道为例。
在施工期间,这条隧道经历了很多穹顶倒塌事件和先进的支援破坏。
对重组样品进行各向同性排水(CD)压缩试验,以获得松散沉积物的机械参数。
进行三维建模以模拟三种不同方案开挖后隧道中的应力和变形分布,即上下台阶隧道,三台隧道和单侧方向隧道掘进。
比较分析结果表明,单侧巷道隧道更适合该隧道,既可以减少拱顶沉降,又可以限制塑性区的开发。
对于类似地质环境中的隧道设计和施工,结果应该是重要的。
关键词:松散堆积物;力学参数; 隧道;开挖方案;比较分析。
说明随着中国交通基础设施快速发展,在过去的几十年里,许多新的隧道已经或正在通过具有挑战性的地质条件的地区建设等。
软岩在隧道建设中经常遇到。
软岩的力学特性导致快速变形和各种干扰(Sharifzadeh等人。
2013a;朱某等人。
2013)它能影响地下结构的稳定性。
为此,软岩石已受到很多关于交通隧道建设的关注。
例如,Jeng等人(2002)评价Mushan的变形砂岩和台湾北部对隧道变、形的影响。
Ozsan和Basarr(2003)计算出强风化凝灰岩Urus坝址引水隧洞的支持能力。
李和舒伯特(2008)研究了在软弱围岩中圆形隧道的长度。
Shahrour 等(2010)分析了用软土构建的隧道的地震响应。
外文文献翻译--铁路隧道的安全
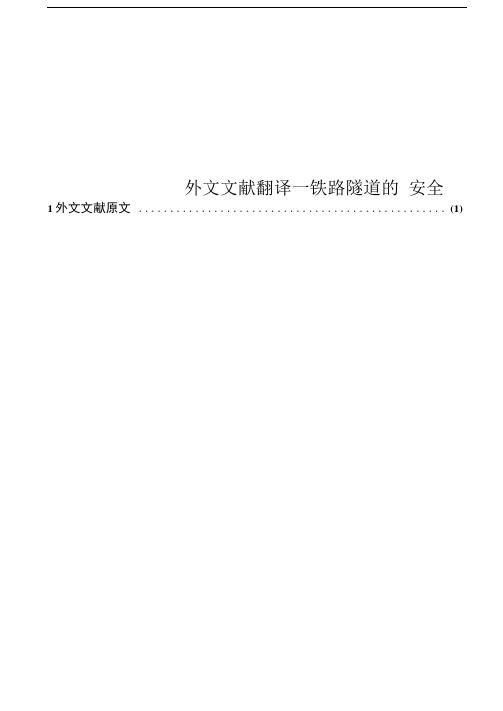
外文文献翻译一铁路隧道的安全1 外文文献原文 (1)2外文文献翻译 (2)1外文文献原文Safety of long railway tunnelsD. Diamantidis"摆,E Zuccarelli b, A. VVestha user *^University of Applied Sciences, Regensburg^ Prufen in ger str. 58y D・93049, Regensburg^GermanybD^Appolonia S.p.A.y Genova^ ItalycBrenner Eisenbahn GmbH, Innsbruck^ AustriaReceived 10 March 1999; accepted 6 September 1999AbstractPlanning and designing railway tunnels with an explicit reference to safety issues is becoming of utmost importance since the combination of high speed, mixed goods-passenger traffic and extreme length of the new tunnels under design or concept evaluation, have sensitively modifled the inherent safety of the railway tunnel. Although the probability of occurrence of accidental events may still be considered rather low, the possible consequences of such events in long tunnels can be catastrophic, therefore raising the overall risk to levels that may be no more acceptable. The scope of this paper is to illustrate the state-of-practice related to risk analysis of long railway tunnels. First, ambitious tunnel projects are briefly reviewed. The applicable risk-analysis procedures are then described and discussed. The problem of risk appraisal is addressed and quantitative target safety levels are proposed. Safety systems for risk reduction are outlined. q2000 Published by Elsevier Science Ltd. All rights reserved.Keywords: Railway tunnels; Risk acceptability; Safety systems; Passenger traffic1.IntroductionThe railway is now moving rapidly toward a modern service transportation industry. High Speed Rail (HSR) systems are already operating in many countries such as Japan, England, France, Italy and Germany. A further development of the whole European HSR network is planned. In order to achieve the design velocity up to 300 km/h, a considerable part of the routes is in tunnels with lengths greater than 10 km and in some cases of the order of 50 km. Table 1 illustrates a list of existing long tunnels worldwide. In this European context, the Commission of the European Communities (CEC) aimed at homogenizing the HSR projects also with respect to the safety issues. However, neither theCEC guidelines nor the existing railway regulations and codes directly address to the problem of quantitatively assessing the safety level for railway systems. This is mostly due to the fact that railway transport is considered by railway operators and perceived by the public as a safe mean of transportation. This approach to safety might be applicable to traditional railway systems, which have proven throughout the years their performance; it is, however, not enough to guarantee the safety of railway systems where innovative and particular conditions are present, or of the existing lines that have to be upgraded to new exercise standards. For example, the combination of high-speed transit, high traffic intensity, combined transport of passengers and dangerous goods and extremely long tunnels, might lead to unacceptable safety levels. Therefore, the designer has to choose a railway system configuration together with the preventive and mitigative measures of accidents that minimize the risk and ultimately should verify bv means of a risk analysis that the obtained safety level is below a predefined target level. The scope of this paper is to illustrate the state-of-practice related to safe tunnel design and associated risk-analysis aspects of long railway tunnels. First, ambitious tunnel projects are briefly reviewed from the safety point of view. The risk-analysis procedures are then described and discussed. The problem of risk appraisal is addressed and quantitative target safety levels are proposed. Finally, safety systems for risk reduction are illustrated.2.Major tunnel projects and the associated riskBasic design aspects in existing or under design and construction tunnels are briefly summarized in this section.Table 1List of existing long tunnels worldwideName Country Length (km) Underground Daischimisu Japan 22.2Simplon II Italv/Switzerland 19.8Appennino Italy 18.6Rokko Japan 16.2Haruna Japan 15.4Gotthard Switzerland 15.0Nakayama Japan 14.8Lolschberg Switzerland 14.5Hokuriku Japan 13.9Prato Tires Italy 13.5Landrucken Germany 10.8 Underwater Seikan Japan 53.9Eurotunnel UK/France 50.0Shin Kanmon Japan 18.7Great Belt Denmark 8.0Severn UK 7.0Mersev UK 4.9Kanmon Japan 3.6 The following tunnels are included:(a)the Channel tunnel between England and France;(b)the Seikan tunnel in Japan;(c)the Gotthard tunnel planned in Switzerland;(d)the Brenner tunnel planned between Italy and Austria;(e)the new Mont Cenis-tunnel planned between Franceand Italy;(f)the tunnel under the Great Belt in Denmark.2.1.The Channel tunnelThe tunnel serves rail traffic and links up the terminals near Folkestone in the south of England and Calais in northern France. The tunnel is some 50 km long and comprises of three parallel tubes, which are located some 25-45 m beneath the sea bed. The trains travel through the twosingle-track running tunnels, each of which has an internal diameter of 7.30 m. Both running tunnels have a continuous escape way in order to enable passengers and train staff to get out of the tunnel quickly in the event of an emergency (see Fig. 1). Two main cross-links connect the two running tunnels so that trains can switch from one tube to the other during maintenance work; these two main cross-links are located in the 37 km long section under the sea bed. Two smaller cross-links are to be found in the vicinity of the tunnel portals. The running tunnels are connected at 250 m intervals by means of 2-00 m diameter pressure-relief tunnels. Through these cross-cuts the pressure that builds up in front of a speeding train can be reduced by diverting the air from one running tunnel into the other. A service tunnel with an internal diameter of 4.50 m is located between the two running tunnels. It is, first and foremost, intended as an escape and access facility in the event of an accident in one of the running tunnels. In addition, this service tunnel provides access to the technical centers, which are distributed along it. The service tunnel and the two running tunnels are connected to each other via a 3.30 in diameter cross-cuts set up at 375 in gaps as escape ways [1].The tunnel is used for the following train services:•the passenger shuttles for cars and buses;•the freight shuttles for lorries as well as;•express and goods trains belonging to the national railway companies.The signaling system incorporating automatic train protection is designed to minimize the risk of any type of collision even during single-line operation when maintenance is being carried out. One of the main criteria for the design of the rolling stock was the requirement that, as far as practicable, in the event of fire, a shuttle is able to continue on its journey out of the tunnel so that fire could be tackled in the open. To achieve this a 30 min fire resistance has been specified for the wagons including the fire doors and shutters in the passenger shuttles. The fire accident that occurred in November 1996 showed that the emergency response procedures required further improvement.c (a)(D(S)° (£) (£)Fig. 2. Investigated tunnel systems: A and B with service tunnel; D without service tunnel.2.2.The Seikan tunnelThe Seikan tunnel was completed in 1988 and constitutesthe longest tunnel worldwide with a total length of 53.9 km.lt is a double-track tunnel with a cross-sectional area of 64 m2. The average traffic is 50 trains per day. The tunnelhas two emergency stations and is thus divided into three sections The middle section is under water with a length of 23 km and has a service tunneL By providing the emergency stations with fire fighting systems, fire can be copedwithin the same manner as conventional tunnel fires. In case of fire, the train must be brought to a stop at the nearest emergency station or must be driven out of the tunneL2.3.The Gotthard Base tunnelThe 57 km long Gotthard Base tunnel is one of the main links for Bahn 2000, the Swiss passenger traffic for the next century, and for the rail corridor of European freight traffic through the Alps [3]. The tunnel route is a part of the Zurich-Lugano line and is intended to carry 150 intercity, passenger and freight trains per day in each direction. Two tracks are needed for these traffic levels and there is a multitude of different tunnel layouts, which can be considered.Possible normal tunnel profiles could consist of:(a)a double-track tunnel with a parallel service tunnel;(b)a pair of single-track tunnel with a service tunnel;(c)three single-track tunnels;(d)a pair of single-track tunnels without a service tunnel^but with frequent interconnections (see Fig. 2).In addition to the traffic tunnels, there is a need for possibly two overtaking stations to allow passenger trains to pass slower freight ones. Natural longitudinal flow in the two tubes will be the basis for the ventilation of the tunnel, which has an overburden of 2000 m or greater, over more than 20 km of itslength.Recently wide-ranging studies have been carried out on the different designs of the Gotthard tunnel. The main parameters that have been thereby investigated are:(a)costs of construction;(b)construction time and method;(c)operational capacity and operability;(d)maintenance;(e)safety for the passengers and the personnel.The performed safety study has shown that the three single-track tunnels and the pair of single-track tunnel with a service tunnel are associated to lower risk and higher operability compared to the double-track tunnel with service tunneL However the associated costs are higher. Based on the evaluation of comprehensive studies the configuration D has been selected, i.e. a pair of single-track tunnels without service tunnel but with interconnections approximatelyevery 325 m. Such interconnections can be used for maintenance purposes and evacuation purposes in case of accidents.2.4.The Brenner tunnelOne of the most striking bottlenecks in passenger and goods transit between Northern Europe andItaly is the north-south connection from Munich via the Brenner Pass to Verona. At present, only one-third of the freight volume can be carried by rail, whilst two-third has to be carried by road over the Brenner Pass. Thus, it is of great importance that the modern railway networks, which either exist or are in the process of being created in the countries of the EuropeanCommunity with their high-speed sections, are welded together via long railway tunnels, which can overcome the Alps as a barrier. If one considers that each year until the turn-of-thecentury, an anticipated trans-goods volume of 150 million tonnes has to be carried over the Brenner Pass 800 m above sea-level, it is thus not surprising that the citizens of the surrounding states have called for the removal of this traffic bottleneck against the background of environmental considerations. The Brenner Base tunnel is urgently required. According to the feasibility study, it consists of a railway tunnel of approximately 55 km length, connecting Innsbruck, Austria and Fortezza, Italy. The rail traffic in the tunnel is similar to that in the Gotthard tunnel and will include approximately 340 trains per day, with 80% of goods trains, of which 10-15% contain dangerous substances. A flnal decision regarding the tunnel configuration has not been taken since the project Is in the feasibility study phase; however, it appears very likely that two single-track tunnels with frequent interconnections as proposed for the Gotthard tunnel would be selected. A safety study has shown that the risk of the tunnel during operation is acceptable if appropriate safety measures are applied [4].Mont Ce'nis tunnel.2.5.The Mont Ce'nis tunnelImproved transport links through the Alps are needed not only because of threatened capacity bottlenecks but also because of the insufficient quality of the existing railway lines through the mountains. The latter, regarded as a technical marvel in the last century, are circuitous with many curves and thus have little chance of competing with the fast Alpine motorways of the present day. In addition to the planned north-south main railway lines through the Alps, the delegates to the World congress for Railway Research in Florence discussed the project for a high-speed east-west rail link taking in Venice, Milan, Turin, Mont Ce'nis, Lyon and Paris. One section of this project is the line between Montme z lian and Turin, catering for mixed passenger and goods traffic, with a base tunnel of 54 km in length beneath Mont d9Ambin.The possible traffic capacities are:•30-40 high-speed trains with a velocity of 220 km/h,•80 goods trains of classical design and combined with a velocity of 100-120 km/h,•50-60 car trains with a velocity of 120-140 km/h.Thus, two single-lane tunnels have been selected as the system configuration (see Fig. 3) with a clearance profile of 43 in2 each [5]. As a result of the topographical conditions and without exceeding a 1.2% gradient for the line, an intermediate point of attack and evacuation point is possible to the north of Modane. Consequently, the project could be executed in the form of two tunnels, each less than 30 km long.2.6.Tunnel under the Great BeltThe tunnel under the Great Belt has a length of ca. 8 km and consists of two single-track tunnels (center distance 25 m) with 30 interconnections every 250 m which serve for evacuation and escape of people in case of an accident [6].2.7.Concluding remarksBased on the aforementioned brief review of existing or planned tunnels, the following conclusions with respect to their design and safety philosophy can be drawn:(a)the design philosophy is somehow different in each of the aforementioned tunnel projects and depends on the national requirements, the tunnel configuration and geometry and the tunnel characteristics (see Table 2);(b)in each case a package of special safety measures is recommended to reduce risk; cost-benefit considerations are usually Implemented to define the optimum package of safety systems;(c)geometries affecting the escape and rescue capabilities vary significantly from case to case (seeTable 2).The basic aspect affecting the tunnel safety is the tunnel configuration. The following tunnel systems are generally considered:(a)one double-track tunnel;(b)one double-track tunnel with service tunnel;(c)two single-track tunnels;(d)two single-track tunnels with service tunnel;(e)three single-track tunnels.Table 2 Comparison of relevant design parameters related to safety in tunnels (TSTT: two single track tunnels; ODTT: one double track tunnel)System Length (km) Distance interconnect, (m) Width of escape-way (m) Traffic itrain/day) Freight trains (%) Velocity Tunnel(km/h)Mont Ce nis TSTT 54 250 > 1.20 160-180 44-50 220Great Belt TSTT 8.0 250 1.20 240 40 10()Eurotunnel TSTT 50 375 1.10 110 45 160Seikan ODTT 53.9 600-1000 0-0.6 40 50 240Gotthard TSTT 57 325 0.75 300 80 200Brenner TSTT 55 250 1.60 340 80 250Fig. 4. Relative risk value for tunnel systems compared to the risk of the double track tunnel.Fig. 4 illustrates the relative risk picture for the aforementioned tunnel systems. The values are based on results from several tunnel risk studies. The final choice of the tunnel system depends not only on safety aspects, but also on other criteria such as costs (construction and maintenance costs), geology and local topography conditions, and operability requirements, etc. In general for tunnels with a length greater than 5 km the configuration of two single-track tunnels is recommended because of the better safety and operability conditions.3.Risk analysis basis3.1.Evaluation of accident statisticsAccident statistics and safety In railway transportation have been discussed in the past and special problems such as the transportation of dangerous materials or fire propagation in tunnels have been analyzed [4,6,7]. The primary causes of accidents can be classified into:•internal causes一mechanical or electrical failures concerning the control guide system as well as the logistic and in service systems;•external causes—arthquakes, floods, avalanches^ etc.;•causes associated to human action― perating faults, errors during maintenance, sabotages,terroristic attacks.Table 3 illustrates the major accidents in railway tunnels during the period 1970-1993. •Based on a critical review of accidental statistics in railway operation, the dominating initiating events and the associated probabilities of occurrence as derived for the Brenner tunnel study are shown in Table 4 for the two basic tunnel configurations, ie one double-track tunnel and two single-track tunnels. The values are based on accident statistics of the Austrian, German and Italian Railways. No relevant accidents have been thereby excluded and approximate correction factors have been considered to account for the safety systems related to the new technology.Table 3 Tunnel accidents in Western Europe with fatalitiesduring the period 1970-1993Date Location Fatalities Initiating event22-7-1971 Simplon (CH)5Derailment16-6-1972 Soissons (F) 108 Hit against an obstacle22-8-1973 S. Sasso (I) 4 Collision23-7-1976 Simplon (CH) 6 Derailment....-4-1980 Sebadell (E) 5 tier21-1-1981 Calabria (I) 5 Hit against an obstacle9-1-1984 El Pais (E) 2 Collision18-4-1984 Spiez (CH) 1 Collision23-12-1984 Bologna (I) 15 Sabotage26-7-1988 Castiglione (I) 1 Fire14-9-1990 Gurtnellen (CH) 1 Derailment31-7-1993 Doniodossola (I) 1 Collision32 Analysis procedureThe analysis of accidents in hazardous scenarios is performed by using event trees. The event tree approach represents a straightforward procedure for describing accidental scenarios and it can include different variables and the notation of time. The probabilities of events in the paths of the event trees are estimated based on the available data, on expert opinion and onengineering Judgement. The complete risk-analysis procedure is shown in Fig. 5. On the basis of the tunnel design and with reference to historical railway accidents, the most important hazardous scenarios are identified. For each selected scenario a probabilisti event tree analysis is performed and the accidental scenario consequences in terms of damages to passengers, Le. facilities are evaluated. The consequence analyses can be based on sophisticated tools that allow to model relevant accidental scenarios in a confined environment. The analysis of the safety measures consists of an evaluation of the actual safety performance of each one of them. Such an evaluation is based, in many cases, on sound engineering Judgement due to the lack of experience with the new safety systems.33. Case studyThe aforementioned procedure has been applied to compute the societal risk in terms of expected fatalities based on the accidental probabilities given in Table 4. The obtained results are illustrated in terms of expected fatalities in Table 5・ A typical application of the results is provided for a 10 km long tunnel in Table 6 for two tunnel systems, i.e. two single-track tunnels and one double-track tunnel. The first system is, as expected, much safer; however, in both cases the obtained societal risk is small. It is noted that the most significant contributor to risk is collision. The acceptability of the risk values is discussedin Section 4.Table 4Input accidental frequencies per one million train kilometers (ODTT: one double track tunnel; TSTT: two single track tunnels)Initiating event TSTT ODTTDerailment0.001Collision0.0002Hit against an obstacle 0.006Fire0.0009 0.001 0.0003 0.006 0.0009Table 5Societal risk, i.e. expected fatalities per 1 million train kilometers (ODTT:one double track tunnel; TSTT: two single track tunnels)Initiating event TSTT ODTT DerailmentCollision 0.025 (46%)0.017 (55%)0.012Hit against an obstacle 0.011 (20%)0.003 (10%)Fire 0.006 (11%)0.006(19%)Total 0.054 (100%)0.031 (100%)4.Risk perception considerations4.1.BackgroundBoth individual risk and societal risk are considered. The acceptable individual risk is a function of the indhdduaPs involvement; different acceptable levels should be defined for activities where the individual voluntarily exposes himself to the hazard with respect to an involuntary participation[8]«For voluntary risk, an upper limit of probability of death per year equal to 1022 has been defined; whereas for the involuntary risk, the following values have been suggested:•P> 10^ 一not acceptable;•10'6< p< ICT1一tolerable;•p < IO"6一acceptable.Table 6Societal risk for the example tunnel (100 trains per day; 10 km long) expressed in expected fatalities per year (ODTT: one double track tunnel; TSTT: two single track tunnels) Initiating event TSTTFor societal risk, the acceptability criteria are based on the definition of an acceptable probabilityrange for events of given consequences. Of course, the severest consequences are associated with the lowest values of the acceptable probability.4.2. Safety standards for other industrial activitiesA brief review of the acceptability risk criteria proposed or adopted by different industrial sectors is provided [9]. Table 7 summarizes the type of approach followed by these industries to define safety targets.42L Road transportRoad accidents have been extensively analyzed and several statistical syntheses have beenpresented. Nevertheless, roadway regulations do no present any quantitative evaluation of the present risk level for the roadway system and do not propose acceptable limits on the occurrence of accidental events.4.2.2. Air transportRisk acceptability criteria have been defined for air transport by some rules and regulations,however, no unique criterion exists yet. At present, one can consider that the acceptable risk level is 1027 accidents with fatalities per hour of flight, corresponding to approximately 2 £ 10210 accidents per kilometer of flight.Table 7 'Risk acceptability criteria for various industrial activitiesQuantitativeRoad transport X XAir transport XChemical XNuclear XOffshore X4.2.3. Chemical industryChemical industries are exposed to hazards that include fires, explosions, toxic releases; riskODTT 0.0039 0.0017 Collision 0.0056Hit agaist an obstacle0.0010 Fire Total0.0103 0.00830.0036 0.0020 0.0020 0.0178analyses in the chemical industry is therefore a strong tradition. Quantitative criteria for the definition of societal acceptable risk levels have been presented [10].4.2.4.Nuclear power plantsSafety is obviously a major concern for nuclear power plants. During design, accidental events with an insignificant probability of occurrence are usually not taken into account. Several studies performed for some plants concluded that the probability of core melt is of the order of 1024-1025 occurrences per year [11].4.2.5.Offshore production platformsSeveral studies have addressed the definition of target safety levels for societal risk for the offshore Industry. In Canada, for example, safety criteria have been defined, based on cost-benefit considerations and comparison to other industrial risks [12], that indicate an annual probability of 1025 for catastrophic consequences, 1023 for severe consequences and 1021 for minor consequences.4.3.Methodological approachThe basic criterion for the definition of a target safet level for a railway system is to assume that the safety inherent in the traditional railways in the past two or three decades is acceptable. The safety target is, therefore derived by analyzing the recent risk history of the railways in terms of the frequency of occurrence of accidents and the extent of their consequences. The procedure generally used to estimate the risk associatedto railway transport is based on the analyses of the frequency of occurrence of given consequences for a given accident; the risk Ri for the ith type of accident is therefore given by:& - RG(1) where pi is the probability of occurrence of the ith type of accident and Ci is the expected consequence of the ith type of accident.Globally, the generic risk Rt is defined as:%=£pg ⑵IThe consequences Ci are generally classified according to three levels of gravity: “medium”, “severe” and “catastrophic”. To each of these classes it has been associated a mean number of victims:•medium consequences: 3 victims;•severe consequences: 30 victims; and•catastrophic consequences: 300 victims.The evaluation of the probability pi can be performed assuming that accidental events occuraccording to a Poisson process; this means thataccidental events are independent [13]. Theprobability of having n accidental events of type/during the time T is given by:P i (n/T} = e-uT (uTy t ln\ (3)where in is the frequency of occurrence of the accidentalevents; whereas the probability of having at least one accidentalevent no in the same time is given by:R (〃O /T )= I -戒 (4)For accidents associated to catastrophic consequencesonly, a few events occurred and therefore statistical data are notsufficient to provide reliable estimates. For these events it istherefore recomniended to use a Bayesian approach.The probability of having at least one accident during the timeTo, having observed n events in a time interval 7\ is given by:p (m ,〃,r )=i-i/[i+7;/Tr +, (5)The aforementioned methodology has been applied ondata of recorded accidents of the Italian, Austrianand German railways. The results are presented in Fig. 6 in a diagram where the consequences, in terms ofexpected victims, are plotted against the annualprobability of having at least one accident that leads to these consequences. Results are considered valid for a first deflnition of an acceptable safety level for Western Europe railway systems and are comparable to the computed values for various tunnel projects.The following can be observed in Fig. 6:10 tolerable and negligibleof riskmatrix:of Fig. 7. Principle classification classification intolerable, undesirable, x.104•events of medium consequences are associated with an annual probability of 10 M (per train-kilometer); •events of severe consequences areassociated with an annual probability of10'10 (per train-kilometer); and•events of catastrophic consequencesRISK CLASSIFICATOI CCR HazardProbeWlVare associated with a probability of 10'11 (per train-kilometer). The curve of Fig. 6, therefore, defines the acceptability conditions for the studiedSuppose, for example, that to a tunnel of approximately 50 km length is associated a daily trafficof 200 trains in both directions, the return periods associated with the accidental events are:■ medium consequences: 100 years;• severe consequences: 1000 years; and• catastrophic consequences: 10 000 years.The return period for “medium consequences” would then result in the same order of magnitudeof the mean life of important infrastructures, such as, for example, a HSR line or a long alpine tunnel.For catastrophic consequences, the return period results are of the same order of magnitude of thataccepted, for example, for offshore production platforms and chemical plants (of the order of 10 000 years) while it results lower than the limit imposed for nuclear plants, which are, however, associated with consequences of significantly higher gravity. As a final remark, it should be noted that the p-C curve proposed in Fig. 6 represents the mean outcome of a probabilistic analysis where several random variables, associated to various uncertainties, have been considered. The acceptability of points falling close to the curve should therefore be critically evaluated also on the basis of cost considerations. Thus, further studies should be aimed at deflning not Just an acceptability curve, but a “desired” region in the p-C diagram which also takes into account cost-benefit considerations.44 Compatibility with rulesNational guidelines regarding the safety of railway tunnels recommend the implementation ofsafety measuresln order to reduce risk. Quantitative risk acceptability criteria are not provided. However, the new EN standards [14] are based on the definition of an acceptable probability range for events of given consequences; to the severest consequences are associated the lowest values of the acceptable probability. For that purpose, the qualitative hazard probability levels suitable for use within railway applications have been defined as:Incredible —xtremely unlikely to occur. It can be assumed that the hazard may not occur;Improbable 一unlikely to occur but possible. It can be assumed the hazard may exceptionally occur; Remote 一likely to occur at sometime in system lifetime. It can be reasonably expectedfor the hazard to occur;Occasional 一likely to occur several times;Probable 一will occur several times. The hazard can be expected to occur often; andFrequent 一likely to occur frequently. The hazard will be continually experienced. Qualitativehazard severity levels have been also defined as follows:Catastrophic 一Fatalities and/or multiple severe injuries;Critical 一Single fatality or severe injury and loss of major system;railway systems, in particular, p-C conditions that fall below the curve are associated to acceptable safety levels.。
Plaxis中常见问题集锦
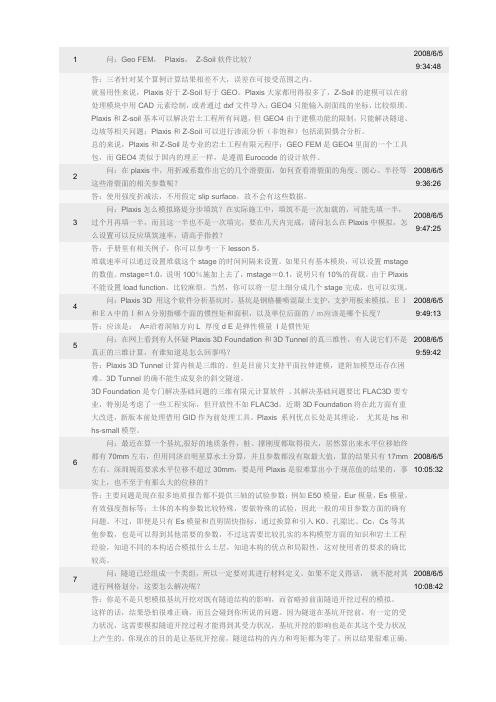
1 问:Geo FEM,Plaxis,Z-Soil软件比较?2008/6/5 9:34:48答:三者针对某个算例计算结果相差不大,误差在可接受范围之内。
就易用性来说,Plaxis好于Z-Soil好于GEO。
Plaxis大家都用得很多了,Z-Soil的建模可以在前处理模块中用CAD元素绘制,或者通过dxf文件导入;GEO4只能输入剖面线的坐标,比较烦琐。
Plaxis和Z-soil基本可以解决岩土工程所有问题,但GEO4由于建模功能的限制,只能解决隧道、边坡等相关问题;Plaxis和Z-Soil可以进行渗流分析(非饱和)包括流固偶合分析。
总的来说,Plaxis和Z-Soil是专业的岩土工程有限元程序;GEO FEM是GEO4里面的一个工具包,而GEO4类似于国内的理正一样,是遵循Eurocode的设计软件。
2问:在plaxis中,用折减系数作出它的几个滑裂面,如何查看滑裂面的角度、圆心、半径等这些滑裂面的相关参数呢?2008/6/59:36:26 答:使用强度折减法,不用假定slip surface,故不会有这些数据。
3问:Plaxis怎么模拟路堤分步填筑?在实际施工中,填筑不是一次加载的,可能先填一半,过个月再填一半,而且这一半也不是一次填完,要在几天内完成,请问怎么在Plaxis中模拟,怎么设置可以反应填筑速率,请高手指教?2008/6/59:47:25 答:手册里有相关例子,你可以参考一下lesson 5。
堆载速率可以通过设置堆载这个stage的时间间隔来设置。
如果只有基本模块,可以设置mstage的数值。
mstage=1.0,说明100%施加上去了,mstage=0.1,说明只有10%的荷载。
由于Plaxis不能设置load function,比较麻烦。
当然,你可以将一层土细分成几个stage完成,也可以实现。
4问:Plaxis 3D 用这个软件分析基坑时,基坑是钢格栅喷混凝土支护,支护用板来模拟,EI和EA中的I和A分别指哪个面的惯性矩和面积,以及单位后面的/m应该是哪个长度?2008/6/59:49:13 答:应该是:A=沿着洞轴方向L×厚度d E是弹性模量I是惯性矩5问:在网上看到有人怀疑Plaxis 3D Foundation和3D Tunnel的真三维性,有人说它们不是真正的三维计算,有谁知道是怎么回事吗?2008/6/59:59:42 答:Plaxis 3D Tunnel计算内核是三维的。
- 1、下载文档前请自行甄别文档内容的完整性,平台不提供额外的编辑、内容补充、找答案等附加服务。
- 2、"仅部分预览"的文档,不可在线预览部分如存在完整性等问题,可反馈申请退款(可完整预览的文档不适用该条件!)。
- 3、如文档侵犯您的权益,请联系客服反馈,我们会尽快为您处理(人工客服工作时间:9:00-18:30)。
隧道设计应该考虑应力释放的影响Van-hung DAO1.水利水电工程学院、河海大学、南京210098,中国2.教师的液压结构,水的资源,越南河内大学摘要:在隧道设计中,支护时间的确定和刚性的支护体系对于维持隧道稳定是非常重要的,该研究所采用的收敛-约束法来确定的隧道的应力和位移,同时考虑到了地基承载力反压力曲线的位移、应力释放的影响以及隧道衬砌和岩石隧道周边洞室的相互作用,这个结论可以确定支护的时间和支护结构的强度和刚度。
这种方法曾适用于越南义安省本卫水力发电厂引水隧道。
结果表明,当位移在0.0865 米到0.0919 米之间时取一个合适的位移值,我们就可以通过架设支护结构来满足隧道的稳定性和经济的要求。
关键词:隧道;支撑结构;稳定性;反压力曲线;应力释放效果1引言岩石在自然环境中,特别是在深部地层当中,常常受到上部地层和重力的影响,由于这些因素的影响在岩体当中二次应力的发展是非常复杂,难以界定的。
隧道开挖过程中,一部分的岩石通常会受到来自隧道洞顶岩石的去除而产生的力-拉应力,有时拉应力会相当高,都会在隧道围岩的周边产生,由于岩石开挖洞周应力的释放会导致周边围岩的变形,从三向应力状态转变为双向应力状态。
在隧道施工过程中,架设支护结构的目的是为了提高和维持岩体的自承能力,以最大程度的发挥岩体的承载能力,并且在岩体内产生有利于发展的内应力场。
1938年,芬纳进行了上部地层和水力结构相互作用方面的研究,并发现了基础的特殊变化曲线和弹塑性介质的解决方案。
1963年,Pacher进行了同样的研究,并取得了同样的结果。
当隧道设计考虑上部地层和水工结构之间的相互作用时,其结果采用新奥法(NATM) 施工和实际结构是比较适合的。
此外,在隧道设计中,隧道衬砌和洞室周围地层之间的相互作用,以及相应的地基反压力曲线,通常被考虑在内(Panet和Guenot1982; Panet1995年)。
在隧道设计中收敛-约束法通常被认为是有效的。
2007年,在越南Nguyen通过研究改变地下水压力载荷,从而影响隧道衬砌的结构,在同一年,Vu和Do也采用收敛-约束的方法对隧道进行设计计算,并假定为初始变形值。
根据Fenne(1938年)和Pacher(1963年)的研究,如果一个刚性支撑结构②(如图1) 架设的比较及时,会因为开挖洞室周围变形不够大而先达到平衡,之后它将会有更大的承载能力。
在曲线外的C点(如图1),岩石性质将变为非线性(塑性)。
当支护结构①安装后产生了一定的位移(A点),则该体系达到与对隧道衬砌较小的均衡负载,之后围岩开始松动,曲线将达到其最低值(如图1中B点),而隧道衬砌压力则增加的非常快。
如果使围岩变形得到适度发展后施做支护结构,则作用在支护结构上的压力将达到最小值,将不会导致隧道的失稳。
图1所示:图1:根据Fenner(1938)and Pacher(1963)得出围岩和支护特征曲线式中:P-支护压力;i-径向应力;Δ r -径向位移;-隧道半径;-为外衬砌的支护抗力;-为外衬砌的支护抗力。
这项研究证实了收敛-约束法在考虑地层反压力曲线、应力释放效应以及隧道衬砌和洞周隧道洞室相互作用条件下,可以确定隧道的应力和位移。
2地层结构模式2.1地基反作用力曲线下的应力计算事实上,静水压力也就是初始应力(侧压力系数为1),洞周开挖的支护半径用表示,如图1所示(Hoek and Brown 1980),塑性区半径的假设条件是依据初始应力场的大小o p、支护压力i p和岩石材料的特性而定。
图2隧道洞周弹塑性应力分布图塑性区内的应力计算公式:2sin 1sin (cot )cot (1)c re i r gH C C r ϕϕσρϕϕ-⎛⎫=+- ⎪⎝⎭其中:-岩石密度g -重力加速度H -洞室埋深C -岩石的内聚力-岩石内摩擦角弹性区内的应力计算公式 (r≥r e ):(2)其中: -径向应力;-切向应力;r -洞周地层任意点离洞室中心的距离;塑性区内的应力计算公式 (r i ≤ r≤r e ):(3)(摩尔应力圆) (塑性区) (弹性区) (1) (2)其中: 隧道的径向变形:(4)其中:G-岩体的剪切模量以及(5)其中:γ -岩石的泊松比;-岩石剪切滑移面的倾角;2.2隧道洞周沿轴线方向上的径向位移和应力释放系数在掌子面和未开挖岩石的影响下,未加固围岩的最大径向位移max u 在距离掌子面只有仅仅的一段距离(通常实验测量结果是1.532i r )。
在现场实测数据的基础上,两位研究人员通过量测半径为的隧道,建立了U r /U rmax 和距离隧道掌子面距离x 之间的关系,如图3中 所示的弹性模型。
1995年Panet 建议采用如下的系数公式,来表示U r /Urmax 和X 之间的关系:(6)其中: -应力释放系数; 公式(6)适用于x 为正值(即前方的掌子面),如图4所绘。
1998年,Chern 在Mingtam 电力隧道工程中,做出了在隧道洞穴附近收敛量测值一览表,根据这些测量的数据绘制在图4所对应的点上,1999年Hock 建议采用如下的经验公式,来最恰当的表示U r /Urmax 和X 之间的关系:(7)图3 表示未支护隧道 的径向位移图4从弹性模型派生的最适合隧道测量的数据曲线2.3支护结构特征曲线特性曲线显示了支护结构的支撑性能(如混凝土、喷射水泥砂浆、岩石锚杆和型钢),它是根据支护压力和径向位移之间的线性关系,并应用到了沿隧道单位长度轴线方向上的支护区域。
假设支护结构的刚度为K ,则支护特性曲线的弹性区域,可以用下列公式计算:(8)混凝土及喷射水泥浆结构的刚度系数为:(9)其中: c E -喷射混凝土的弹性模量;-喷射混凝土的泊松比系数;-衬砌厚度;钢拱支撑的刚度系数表达式:(10)式中: S -钢拱支撑沿洞周轴向的间距(m);-振动棒之间夹角的一半(°);W -夯实块的宽度(m);s A -钢拱支撑横截面的面积(m 2 );s I -钢拱支撑的惯性矩(m 4 );s E -钢拱支撑的弹性模量(Mpa);B t -垫块的厚度(m);B E -垫块的弹性模量(Mpa);一段长为b I 和直径为b d 的机械式锚杆以及黏结式锚杆的支护刚度用如下表达式计算:(11)式中: S c -锚杆的环向间距;S l -锚杆的轴向间距;Q -拉力锚;E b -锚杆的弹性模量;l-螺栓的净长度;当采用组合式支护结构时,组合式支护结构的组件都假设在同一时间被架设,则组合式支护结构刚度可假定为各部件支护刚度的总和:(12)式中: K s1 -第一个支护结构的刚度; (10) (11) (12)K s2-第二个支护结构的刚度;因此,支护结构特征曲线公式如下:(13)式中: u p -塑形区边缘的径向位移量;u0-开挖引起的隧道初始径向位移量(由应力释放效应而定);3案例分析3.1、与设计参数相关的例子对the Ban Ve Hydroelectric 水电厂引水隧洞进行了一次调查,得该隧道的材料参数见表1。
表1 隧道物理力学参数M300喷浆机喷射厚度为10厘米的水泥浆液和直径为20毫米、长度为2米的钢锚杆组合在一起被应用到支护结构上,沿隧道洞周的锚杆轴向间距为 1.5米,Matlab编程语言被用于计算当中。
3.2、计算结果与分析地层压力值=7.3008 MPa.图.5和6分别表示的塑形内和弹性区的应力,从图5可以看出,最大塑性区半径为r emax =1.1451 m,因此在弹塑性边界处压力值为re =4.0195 MPa的压力,这个最大压力值是支撑结构所能够承受的,最大位移u rmax=0.1118米相当于=0(无支护时)的大小,图7表示了在没有支护的情况下,沿隧道轴线方向上的应力释放系数,围岩与支护结构的的初始位移特征曲线如图8所示。
图5塑性区范围内围岩应力图6弹性区范围内围岩应力图7无支护时沿隧道轴线方向上图8围岩和支护结构不同初始洞周的应力释放系数位移特征曲线基于fenner- Pacher理论和Vietnames地下工程设计施工标准(建设部2003),我们通过比较可以得出支护结构的最大压力值(相当于弹塑性边界处的re应力),能承受上述分析结果的压力值。
可以得出如下结论:当支护结构的初始位移u0=0.083米以下时可以得出:隧道开挖后应立即进行加固,支护结构的压力值=6.902MPa>re =4.0195MPa,由于支护结构架设的不及时,则施加支护后围岩将继续发生变形,这将导致围岩的局部失稳。
当支护结构的初始位移u0=0.087米时,将得到以下结论:围岩加固的范围x 为1.7085米,应力释放系数为0.4817。
支护结构的压力值=3.7818MPa<re =4.0195MPa,若围岩发生适度变形,则隧道可保持稳定。
当支护结构的初始位移u0在0.093米时,将得到以下结论:围岩加固的范围x 为 3.74米,应力释放系数为0.7327。
支护结构的压力值=1.9511MPa≤re =4.0195MPa,若围岩发生很大变形,表明隧道将可能失稳。
当支护结构的初始位移u0在0.097米时,将得到以下结论:围岩加固的范围x为 6.8米,应力释放系数=0.868。
支护结构的压力值=0.9632MPa≤re =4.0195MPa,若围岩发生变形过大,将导致隧道洞顶岩石的松动从而增加围岩的压力,则隧道失稳。
因此,在上述情况下,我们可以说当支护结构的位移u0在0.0865和0.0919米之间时,我们可以通过设置支护结构来满足结构的稳定性和经济性的要求。
4、结论总的来说,在这项研究中所描述的初始位移值u0比2007年Vu and Do假设的初始位移值u0更准确、更详细,通过对比,我们可以得到用应力释放效应来确定u0值的大小,比排除应力释放效应提供的曲线方案(Hoek and Brown 1980;Wiliams 1997)更加完整。
上述结果显示,对于确定支护时间收敛-约束法是一种比较有效的设计方法。
这是一种完全不同于传统隧道的设计方法,该方法只适用于早期的围岩巩固和隧道开挖后衬砌及早支护,它仅仅考虑到临时支护结构只承受松动岩石的载荷,而忽略了岩体自身的承载能力。
然而,这个结论仅限于二维弹塑性模型,初始应力场(静力场)和环形隧道横截面。
因此,对于在非静力场的岩石或者非圆形隧道横截面的情况下,例如在非均匀弹塑性模型、粘弹性模型、脆性模型等破坏性模型下,则该收敛-约束法需要在隧道设计中进行更广泛的研究。
5参考文献Anagnostou, G, and Kovari, K.1993.Significant parameters in elastoplastic analysis of underground openings Journal of Geotechnical Engineering, l19(3) 401-419.[doi:10.1061/(ASCE)0733-9410(1993)119:3(401)]Brady, B.H.G, and Brown, E.2004.Rock Mechanics for Underground Mining.Dordrecht: Kluwer Academic PublishersCarranza Torres, C., and Fairhurst, C. 1999. The elasto-plastic response of underground excavations in rock masses that satisfy the Hoek-Brown failure criterion. International Journal of Rock Mechanics and Mining Sciences. 36(6),777—809.Carranza-Torres, C., and Fairhurst, C.2000.Application of the convergence -confinement method of tunnel design to rock masses that satisfy the Hoek-Brown failure criterion.Tunneling and Underground Space Technology,15(2),187-213.[doi: 10.1016/S0886—7798(00)00046—8] Carranza-Torres,C.,Alonso,E.,Alejano,L.R.,Varas,F,and Fdez—Manin,G 2002.Elasto—Plastic analysis of deep tunnels in britile rock using a scaledform of the M ohr-Coulomb failure criterion.Hammah,R.,Bawden,W ,Curran,J.,and Telesnicki,M.,eds.,Proceedings of the 5th North American Rock Mechanics Symposium and the 17th Tunnelling Association of Canada Conference (NARMS—TAC 2002):Mining and Tunnelling Innovation and Opportunity,283—293.Toronto:University of Toronto Carranza—Torres,C.2003.Dimensionless graphical representation of the exactelasto—plastic solution of a circular tunnel in a Mohr-Coulomb material subject to uniform far-field stressess.Rock Mechanics and Rock Engineering,36(3),237—253.[doi:10.1007/s00603—002—0048—7Rock]Chern,J.C.,Shiao,E and Yu,C.W 1998.An empirical safety criterion for tunnel construction.Proceedings of Regional Symposium on Sedimentary Rock Engineering,222—227.Taipei,China.Fairhurst,C.,and Carranza-Torres,C.2002.Closing the circle:Some comments on design procedures for tunnel supports in rock. Labuz, J.E, and Bentler,J.G,eds, Proceedings of the University of Minnesota 50th Annual Geotechnical Conference, 21—84.Minneapolis:University ofMinnesota PressFenner,R.1938.untersuchungen zur erkenntnis des gebirgsdruckes.Gliicka 32,681—695Goodman,R.E.1 980.Introduction to Rock Mechanics.New York:John W iley an d SonsHoek,E.,and Brown,E.T 1980.Underground Excavations in Rock.London:Institute of Mining and Metallurgy.Hoek,E.,Kaiser,P.K.,and Bawden,W.F.1995.Support of Underground Exactions in Hard Rock.Rotterdam:BalkemaHoek,E.1999.Support for very weak rock associated with faults and shear zones.Proceedings of International Symposium on Rock Support and Reinforcement Practice in Mining.KalgoorliMinistry of Construction.2003.The Vietnamese Construction Design Standard for Underground Works.Hanoi:Construction Publisher.(in Vietnamese) Nguyen,R D.2007.Influence of the change in underground water pressure to the load effecting on the tunnel lining.Journal of Road and Bridge,1(2) l1-18 (in Vietnamese)Nguyen,Q.H.,and Nguyen,T H.2004.Design of the Underground Traffic Structure.Hanoi:Traffic Publisher.(in Vietnamese)Pacher,E 1963.Deformations mess ungen in Versuchsstollen als Mittel zur Enforschung des Gebirgsverhaltens undzur Bemessung des Ausbaues.Proceedings of the 14th Symposium of the Austrian Regional Group of the ISRM,149—161.Salzburg二0一三届土木工程专业(岩土与地下结构工程)毕业设计·外文翻译Panet,M.,and Guenot,A.1982.Analysis of convergence behind the face of a tunne1.Proceedings of the International Conference on Tunnelling,197—204.Brighton:The Institution of Mining and MetallurgyPanet, M. 1995. Le Calcul des Tunnels par la Methode Convergence-Confinement.Paris:Presses de l'Ecole Nationale des Ponts et Chaussres.(in French) Tran,B.V,and Bui,T C.2006.The method of convergence—confinement in vault designing.Journal of Road and Bridge,26(2),1 26—1 39.(in Vietnamese) Tran,T G,and Ta,T D.20o3.T he Design of Underground Structures.Hanoi:Construction Publisher.(in Vietnamese)Vu,T T G,and Do,N.2007.Applying the convergence—confinement method in designing the tunne1.Proceeding of the International Workshop Institute of Transport Science and Technology,24—35.Danang:Danang University.(in Vietnamese)Wiliams,O.1997.Engineering and Design-Tunnels and Shafts in Rock. Washington:U. S. Army Corps of Engineers。