永磁同步电机无位置传感器
《永磁同步电机全速度范围无位置传感器控制技术的研究与实现》范文
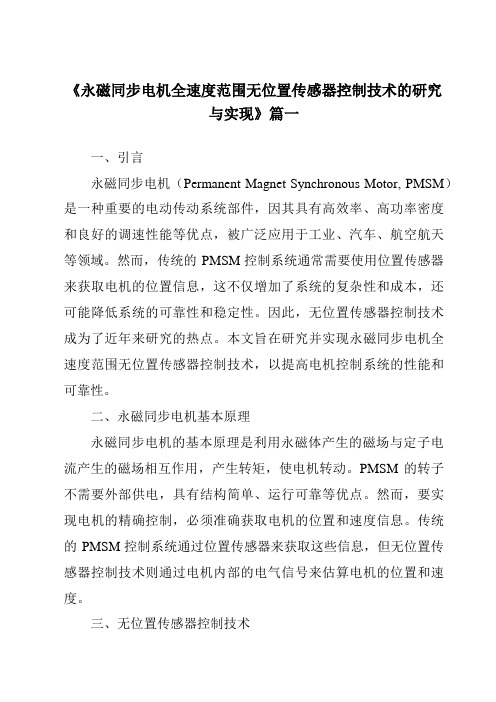
《永磁同步电机全速度范围无位置传感器控制技术的研究与实现》篇一一、引言永磁同步电机(Permanent Magnet Synchronous Motor, PMSM)是一种重要的电动传动系统部件,因其具有高效率、高功率密度和良好的调速性能等优点,被广泛应用于工业、汽车、航空航天等领域。
然而,传统的PMSM控制系统通常需要使用位置传感器来获取电机的位置信息,这不仅增加了系统的复杂性和成本,还可能降低系统的可靠性和稳定性。
因此,无位置传感器控制技术成为了近年来研究的热点。
本文旨在研究并实现永磁同步电机全速度范围无位置传感器控制技术,以提高电机控制系统的性能和可靠性。
二、永磁同步电机基本原理永磁同步电机的基本原理是利用永磁体产生的磁场与定子电流产生的磁场相互作用,产生转矩,使电机转动。
PMSM的转子不需要外部供电,具有结构简单、运行可靠等优点。
然而,要实现电机的精确控制,必须准确获取电机的位置和速度信息。
传统的PMSM控制系统通过位置传感器来获取这些信息,但无位置传感器控制技术则通过电机内部的电气信号来估算电机的位置和速度。
三、无位置传感器控制技术无位置传感器控制技术主要通过电机内部的电气信号来估算电机的位置和速度。
常见的无位置传感器控制技术包括基于反电动势法、模型参考自适应法、滑模观测器法等。
本文采用基于反电动势法的无位置传感器控制技术,通过检测电机的反电动势来估算电机的位置和速度。
四、全速度范围无位置传感器控制策略为了实现永磁同步电机全速度范围的无位置传感器控制,需要采用合适的控制策略。
本文采用基于矢量控制的策略,通过实时调整电机的电压和电流来控制电机的位置和速度。
在低速阶段,采用初始位置估算和误差补偿技术来提高位置的估算精度;在高速阶段,则采用反电动势法来准确估算电机的位置和速度。
此外,还采用了自适应控制技术来应对电机参数变化和外部干扰的影响。
五、实验与结果分析为了验证本文所提出的无位置传感器控制技术的有效性,进行了实验验证。
基于滑模观测器的无位置传感器永磁同步电机研究资料
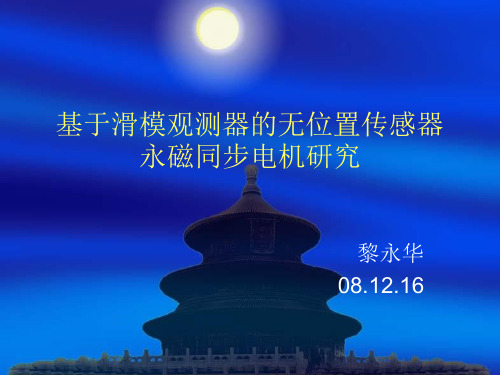
s cx1 x2
c>0
直线s=0是切换线,在这个切换线上,u是不连续的。
设t=0时,状态x在s>0一侧,在u=u+作用下,在某个时限后到达s=0, 并进入s<0一侧,u=u-,又往s=0控制。……
当系统在滑模状态时,s 0, s 0
滑模观测器在无位置观测器系统中的应用
PMSM在 坐标系下的数学模型
u R 1 i e i L L L u R 1 i i e L L L
e 0e sin e e 0e cos e
假设 e 0 即转速变化很慢时,电机的反电动势模型:
e e
2. 国内外研究现状
电力电子器件的发展为电机调速奠定了物质基础。高速数字信号处理器 (DsP)的 高速处理能力使无位置传感器电机控制技术的复杂算法得到实现,近10年来, 各国学者致力于无位置传感器控制系统的研究,并且提出了几种切实可行的方 法,目前,适用于永磁同步电机位置估算的主要策略有: (1)利用定子端电压和电流直接计算ω和θ (2)基于观测器基础上的估算 (3)模型参考自适应 (4)人工智能理论基础上的估算
基于滑模观测器的无位置传感器 永磁同步电机研究
黎永华 08.12.16
1. 研究意义
永磁同步电机调速系统是以永磁同步电机为控制对象,采用变压变频技术对电机 进行调速的控制系统。因其具有能耗低、可靠性高、控制精确等优点,在许多领 域得到广泛的应用。PMSM控制系统稳定运行是建立在闭环控制基础之上的,如何 获取转子位置和速度信号是整个系统中相当重要的一个环节。当前,在大多数调速 驱动系统中,最常用的方法是在转子轴上安装位置传感器,不过传感器增加了系统 的成本,降低了系统的可靠性和耐用性。因此,无位置传感器的控制系统有着广泛 的应用前景。它通过测量电动机的电流、电压等可测量的物理量,通过特定的观测 器策略估算转子位置,提取永磁转子的位置和速度信息,完成闭环控制。 主要优点: 1、减少成本 2、减小电机体积 3、提高系统可靠性 4、减少系统维护量
永磁同步电机无位置传感器控制技术研究综述
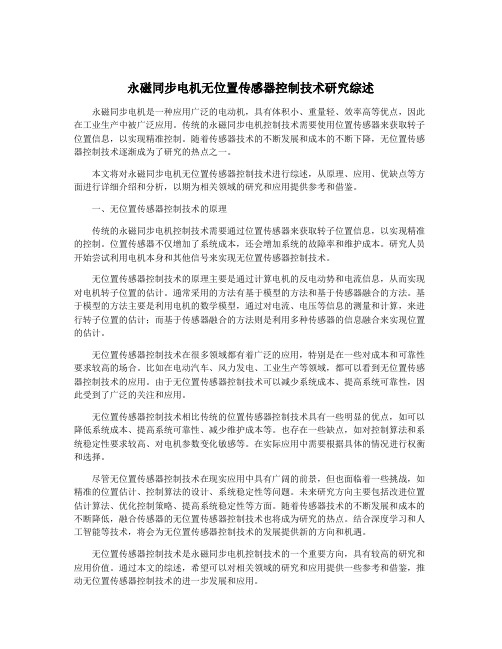
永磁同步电机无位置传感器控制技术研究综述永磁同步电机是一种应用广泛的电动机,具有体积小、重量轻、效率高等优点,因此在工业生产中被广泛应用。
传统的永磁同步电机控制技术需要使用位置传感器来获取转子位置信息,以实现精准控制。
随着传感器技术的不断发展和成本的不断下降,无位置传感器控制技术逐渐成为了研究的热点之一。
本文将对永磁同步电机无位置传感器控制技术进行综述,从原理、应用、优缺点等方面进行详细介绍和分析,以期为相关领域的研究和应用提供参考和借鉴。
一、无位置传感器控制技术的原理传统的永磁同步电机控制技术需要通过位置传感器来获取转子位置信息,以实现精准的控制。
位置传感器不仅增加了系统成本,还会增加系统的故障率和维护成本。
研究人员开始尝试利用电机本身和其他信号来实现无位置传感器控制技术。
无位置传感器控制技术的原理主要是通过计算电机的反电动势和电流信息,从而实现对电机转子位置的估计。
通常采用的方法有基于模型的方法和基于传感器融合的方法。
基于模型的方法主要是利用电机的数学模型,通过对电流、电压等信息的测量和计算,来进行转子位置的估计;而基于传感器融合的方法则是利用多种传感器的信息融合来实现位置的估计。
无位置传感器控制技术在很多领域都有着广泛的应用,特别是在一些对成本和可靠性要求较高的场合。
比如在电动汽车、风力发电、工业生产等领域,都可以看到无位置传感器控制技术的应用。
由于无位置传感器控制技术可以减少系统成本、提高系统可靠性,因此受到了广泛的关注和应用。
无位置传感器控制技术相比传统的位置传感器控制技术具有一些明显的优点,如可以降低系统成本、提高系统可靠性、减少维护成本等。
也存在一些缺点,如对控制算法和系统稳定性要求较高、对电机参数变化敏感等。
在实际应用中需要根据具体的情况进行权衡和选择。
尽管无位置传感器控制技术在现实应用中具有广阔的前景,但也面临着一些挑战,如精准的位置估计、控制算法的设计、系统稳定性等问题。
未来研究方向主要包括改进位置估计算法、优化控制策略、提高系统稳定性等方面。
永磁同步电动机无位置传感器矢量控制仿真
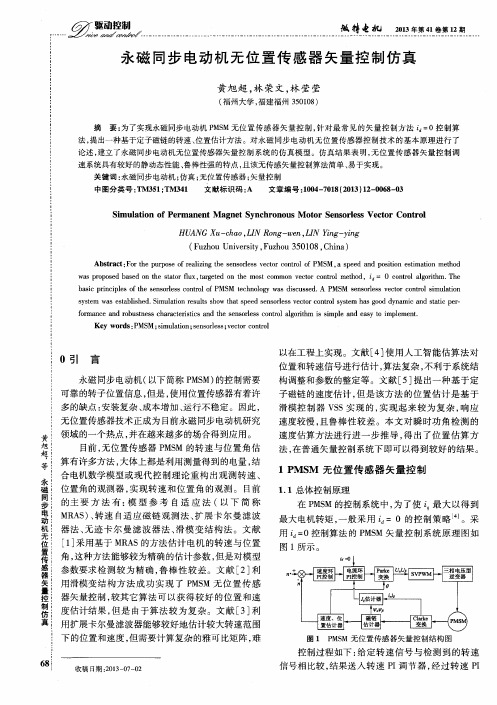
可靠的转子位置信息, 但是, 使用位置传感器有着许
多的缺 点 : 安装 复 杂 、 成本 增加 、 运行不 稳定 。因此 ,
无 位置 传感器 技术 正成 为 目前永 磁 同步 电动机研 究 领 域 的一个热 点 , 并 在越来 越多 的场 合得 到应用 。
中 图分 类 号 : T M3 5 1 ; T M3 4 1 文献 标 识 码 : A 文章编号 : 1 0 0 4 — 7 0 1 8 ( 2 00 3
S i mul a t i o n o f Pe r ma n e n t Ma gn e t S y nc hr o n o us Mo t o r Se n s or l e s s Ve c t o r Co nt r o l HU ANG Xu— c h a o. Ll N Ro n g-we n。 L I N Y i n g- y i n g
( F u z h o u U n i v e r s i t y , F u z h o u 3 5 0 1 0 8 , C h i n a )
Ab s t r a c t : F o r t h e p u r p o s e o f r e a l i z i n g t h e s e n s o r l e s s v e c t o r c o n t r o l o f P MS M, a s p e e d a n d p o s i t i o n e s t i ma t i o n me t h o d w a s p r o p o s e d b a s e d o n t h e s t a t o r l f u x, t a r g e t e d o n t h e mo s t c o mmo n v e c t o r c o n t r o l me t h o d,i d= 0 c o n t r o l lg a o r i t h m. T h e b a s i c p r i n c i p l e s o f t h e s e n s o r l e s s c o n t r o l o f P MS M t e c h n o l o g y w a s d i s c u s s e d . A P MS M s e n s o r l e s s v e c t o r c o n t r o l s i mu l a t i o n s y s t e m w a s e s t a b l i s h e d . S i mu l a t i o n r e s u h s s h o w t h a t s p e e d s e n s o r l e s s v e c t o r c o n t r o l s y s t e m h a s g o o d d y n a mi c a n d s t a t i c p e r — f o r ma n c e a n d r o b u s t n e s s c h a r a c t e is r t i c s a n d t h e s e n s o r l e s s c o n t r o l lg a o r i t h m i s s i mp l e a n d e a s y t o i mp l e me n t .
永磁同步电机无位置传感器控制技术研究综述
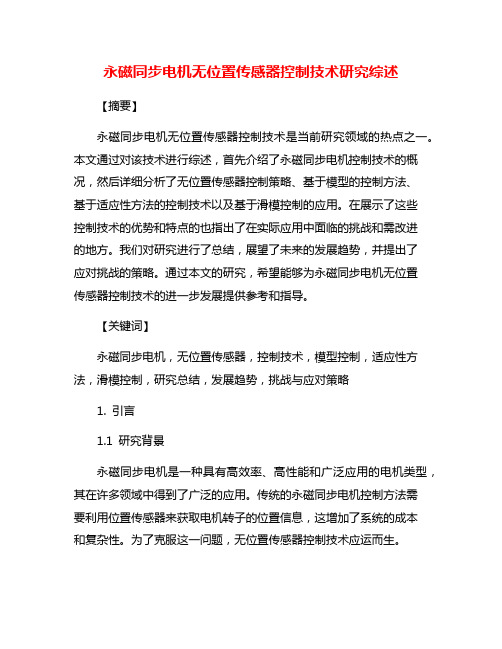
永磁同步电机无位置传感器控制技术研究综述【摘要】永磁同步电机无位置传感器控制技术是当前研究领域的热点之一。
本文通过对该技术进行综述,首先介绍了永磁同步电机控制技术的概况,然后详细分析了无位置传感器控制策略、基于模型的控制方法、基于适应性方法的控制技术以及基于滑模控制的应用。
在展示了这些控制技术的优势和特点的也指出了在实际应用中面临的挑战和需改进的地方。
我们对研究进行了总结,展望了未来的发展趋势,并提出了应对挑战的策略。
通过本文的研究,希望能够为永磁同步电机无位置传感器控制技术的进一步发展提供参考和指导。
【关键词】永磁同步电机,无位置传感器,控制技术,模型控制,适应性方法,滑模控制,研究总结,发展趋势,挑战与应对策略1. 引言1.1 研究背景永磁同步电机是一种具有高效率、高性能和广泛应用的电机类型,其在许多领域中得到了广泛的应用。
传统的永磁同步电机控制方法需要利用位置传感器来获取电机转子的位置信息,这增加了系统的成本和复杂性。
为了克服这一问题,无位置传感器控制技术应运而生。
无位置传感器控制技术通过利用电流和电压的反馈信息,结合适当的控制策略,实现对永磁同步电机的精准控制。
这种技术不仅可以降低系统成本,还可以提高系统的鲁棒性和稳定性。
研究永磁同步电机无位置传感器控制技术具有重要的理论和实际意义。
本文旨在对永磁同步电机无位置传感器控制技术进行综述和总结,系统地介绍这一领域的研究现状和发展趋势,为相关领域的研究人员提供参考和借鉴。
通过对相关文献和案例的分析和总结,为进一步推动永磁同步电机无位置传感器控制技术的发展提供理论支持和实践指导。
1.2 研究目的永磁同步电机无位置传感器控制技术的研究目的是为了探索在没有位置传感器的情况下,如何实现对永磁同步电机的精准控制。
通过研究不依赖位置传感器的控制策略和技术,可以降低系统的成本和复杂度,提高系统的稳定性和可靠性。
研究无位置传感器控制技术还可以拓展永磁同步电机在各种应用中的适用范围,推动新能源车辆、工业制造等领域的发展。
永磁同步电机无位置控制正反转原理

永磁同步电机无位置控制正反转原理下载温馨提示:该文档是我店铺精心编制而成,希望大家下载以后,能够帮助大家解决实际的问题。
文档下载后可定制随意修改,请根据实际需要进行相应的调整和使用,谢谢!并且,本店铺为大家提供各种各样类型的实用资料,如教育随笔、日记赏析、句子摘抄、古诗大全、经典美文、话题作文、工作总结、词语解析、文案摘录、其他资料等等,如想了解不同资料格式和写法,敬请关注!Download tips: This document is carefully compiled by the editor. I hope that after you download them, they can help you solve practical problems. The document can be customized and modified after downloading, please adjust and use it according to actual needs, thank you!In addition, our shop provides you with various types of practical materials, such as educational essays, diary appreciation, sentence excerpts, ancient poems, classic articles, topic composition, work summary, word parsing, copy excerpts, other materials and so on, want to know different data formats and writing methods, please pay attention!永磁同步电机是一种广泛应用于工业和家用领域的电机,其高效率和稳定性受到了广泛关注。
永磁同步电机无位置传感器矢量控制PPT.
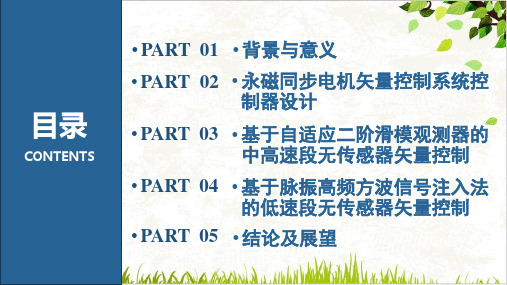
转速阶跃给定时dq 轴电流瞬态波形
随着转速的升高,交直轴电流的 动态过渡过程越来越长。
02 电流环控制器设计
电流环+ 等效控C s制 器U为s : Gs
Y s
+
- Y s*
Y s*
U s C s
Gs Y s
- - F s =F结PI s论 +F:dec表s明= qRs轴s +0上 L的s 电Gˆ s流Rs s 0+波 L动s +对d0轴sr L电s 流无0sr L影s 响,实现GFˆ 了ss dq轴电流的
Rs
i i
+2r
L信波Lcsois器号n22来,rr 实需LL现要csoins信使22号rr用 分ii多 离个。r滤f
f sinr cosr
01 主要工作
转速环
电流环
r*
控制器
PI
iq*s
控制器
PI
d , q u
②
r
在中高速段,研究id*s了 0
u
④ 针对P全I 速范围 内
一种具有电机参数在 的无位置传感器
i
uq +
-a b c
ib 态耦合关系。
1
iq
-
r f
Rs sLs
PMSM
PMSM矢量控制框图
02 电流环控制器设计
为 了 观 察 电 机 d-q 轴 电 流 的 耦 合
影响,进行了仿真分析。图所示 的工况为初始转速为零,在0.2s 时 刻 转 速 阶 跃 给 定 为 0-300rad/s , 在0.4s时刻给定 300-600 rad/s 的 转 速 阶 跃 , 在 0.6s 时 刻 给 定 600900 rad/s转速阶跃。
永磁同步电机无位置传感器控制技术研究综述
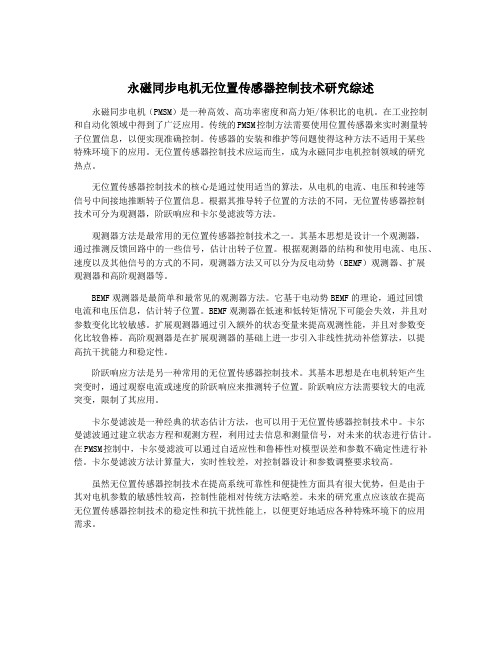
永磁同步电机无位置传感器控制技术研究综述永磁同步电机(PMSM)是一种高效、高功率密度和高力矩/体积比的电机。
在工业控制和自动化领域中得到了广泛应用。
传统的PMSM控制方法需要使用位置传感器来实时测量转子位置信息,以便实现准确控制。
传感器的安装和维护等问题使得这种方法不适用于某些特殊环境下的应用。
无位置传感器控制技术应运而生,成为永磁同步电机控制领域的研究热点。
无位置传感器控制技术的核心是通过使用适当的算法,从电机的电流、电压和转速等信号中间接地推断转子位置信息。
根据其推导转子位置的方法的不同,无位置传感器控制技术可分为观测器,阶跃响应和卡尔曼滤波等方法。
观测器方法是最常用的无位置传感器控制技术之一。
其基本思想是设计一个观测器,通过推测反馈回路中的一些信号,估计出转子位置。
根据观测器的结构和使用电流、电压、速度以及其他信号的方式的不同,观测器方法又可以分为反电动势(BEMF)观测器、扩展观测器和高阶观测器等。
BEMF观测器是最简单和最常见的观测器方法。
它基于电动势BEMF的理论,通过回馈电流和电压信息,估计转子位置。
BEMF观测器在低速和低转矩情况下可能会失效,并且对参数变化比较敏感。
扩展观测器通过引入额外的状态变量来提高观测性能,并且对参数变化比较鲁棒。
高阶观测器是在扩展观测器的基础上进一步引入非线性扰动补偿算法,以提高抗干扰能力和稳定性。
阶跃响应方法是另一种常用的无位置传感器控制技术。
其基本思想是在电机转矩产生突变时,通过观察电流或速度的阶跃响应来推测转子位置。
阶跃响应方法需要较大的电流突变,限制了其应用。
卡尔曼滤波是一种经典的状态估计方法,也可以用于无位置传感器控制技术中。
卡尔曼滤波通过建立状态方程和观测方程,利用过去信息和测量信号,对未来的状态进行估计。
在PMSM控制中,卡尔曼滤波可以通过自适应性和鲁棒性对模型误差和参数不确定性进行补偿。
卡尔曼滤波方法计算量大,实时性较差,对控制器设计和参数调整要求较高。
- 1、下载文档前请自行甄别文档内容的完整性,平台不提供额外的编辑、内容补充、找答案等附加服务。
- 2、"仅部分预览"的文档,不可在线预览部分如存在完整性等问题,可反馈申请退款(可完整预览的文档不适用该条件!)。
- 3、如文档侵犯您的权益,请联系客服反馈,我们会尽快为您处理(人工客服工作时间:9:00-18:30)。
Performance Comparison of Permanent Magnet Synchronous Motors and Controlled Induction Motors in Washing Machine Applications using Sensorless Field Oriented ControlAengus Murray, Marco Palma and Ali HusainEnergy Saving Products DivisionInternational RectifierEl Segundo, CA 90245Abstract—This paper describes two alternative variable speed motor drive systems for washing machine applications. Three phase induction motors with tachometer feedback and direct drive permanent magnet synchronous motors with hall sensor feedback are two drive systems commonly used in North American washers today. Appliance manufacturers are now evaluating sensorless drive systems because of the low reliability and high cost of the speed and position feedback sensors. A Field Oriented Control Algorithm with an embedded rotor flux and position estimation algorithm enables sensorless control of both permanent magnet synchronous motors and induction motors. The estimator derives rotor shaft position and speed from rotor flux estimates obtained from measured stator currents and the applied voltages. Sampling of currents in the dc link shunt simplifies stator current measurement and minimizes cost. Field oriented control algorithm allows good dynamic control of torque and enables an extended speed range through field weakening. The digital control algorithm runs on a unique hardware engine that allows algorithms to be designed using graphical tools. A common hardware platform can run either the PMSM or IM using sensorless field oriented control in a front loading washer application. Test results are presented for both drives in standard wash cycles.Keywords-component; Advanced Control; Field Oriented Control Algorithm;, Appliance control architecture;I.I NTRODUCTIONAccurate control of drum speed is required in both horizontal and vertical axis washer machines [1]. In front loading horizontal axis washers, the drum speed determines the washing action. There is a critical drum RPM, depending on the drum radius, above which the clothes stick to the inside edge of the drum. At this speed, the centrifugal force due to rotation balances the weight of the wet clothes. At speeds below this, the clothes will stick to the side of the drum until the component of the weight acting along the radius is greater than the centrifugal force. Once this angle is reached, the clothes fall back down into the base of the drum. The speed of the drum determines how vigorously the clothes are washed and allows a gentle wash cycle to be selected for delicate items. In the spin mode, the water is drained and the drum speed is increased well beyond the critical speed and the water forced out of the cloths by the centrifugal force. In traditional top loading vertical axis machines, the agitation action is produced mechanically using a gearbox and clutch. However, the introduction of speed control systems not only simplifies the mechanical system but also allows for wash cycle control. The control of the speed and angle of stroke allows the system designer to better manage the washing action and so develop wash cycles that use less water.European front-loading washers have used variable speed control for many years and typically use a universal ‘brush type’ motor. However, the American washer uses a larger drum size, which requires a motor with a power range beyond that of the universal motor solution. The front-loading drive solutions on the market today include direct drive permanent magnet synchronous motor drives or a belt drive using an induction motor. Appliance manufacturers are now evaluating these two drive types in top-loading machine to reduce cost and improve performance. However, both these drive systems use shaft feedbacks sensors. The direct drive PMSM typically uses a Hall Effect sensor for position feedback while the induction motor drive typically uses an analog or digital tachometer for speed feedback. The ideal universal drive can run either a PMSM or an induction motor without shaft feedback sensors. However, a single hardware platform can efficiently run either a PMSM or an induction motor using sensorless field oriented control algorithm. In both cases, speed and position estimates derive from motor terminal voltages and currents.Induction motors were initially preferred for washing machine drives because of the ease of running in high speed field weakening mode even with simple scalar control methods. However, the PMSM is now becoming a viable solution because field oriented control approach enables high speed field weakening. In an induction motor, the torque producing current flows in both the rotor and stator windings while the air gap field generation needs additional field current. Therefore, in washing mode, the total copper losses are more than doublethe PM motor losses since all the PM motor stator current can generate torque. However, in spin mode the PM motor has a disadvantage since field weakening consumes a large fraction of the stator current. In the induction motor, the magnetizing current is almost zero in field weakening mode. This paper compares the performance of two similarly rated motors operating in both parts of the wash cycle. It also examines specific advantages for each of these drives in the washer application.II.F IELD ORIENTED CONTROL OF PERMANENT MAGNET SYNCHRONOUS MOTORS AND INDUCTION MOTORSField oriented control (FOC) in three phase motors is a well know technique that decouples the ac stator currents into quasi dc field and torque components to simplify the control system design [2]. The control architecture, shown in Fig. 1, is based around rotating reference frame models that were developed many years ago to analyze the operation of ac induction and synchronous machines. In the control model, stationary windings carrying ac currents are represented by equivalent rotating windings carrying dc current. The well known Clarke and Park vector transformations translate three phase stator currents into d-axis and q-axis current components in a reference frame synchronized to the angle of the rotor flux. In the rotating frame reference system, we define the d-axis as being aligned with the rotor field and the q-axis as being in quadrature with the rotor field. In both the synchronous motor and the induction motor, the rotor flux is a function of the d-axis current and the motor torque is proportional to the product of the rotor flux and the q-axis current. Thus, the d-axis current is equivalent to the field current in a dc motor while the q-axis current is equivalent to the dc motor armature current. Two independent current loops vary the applied stator winding voltages in order to deliver the desired motor torque and flux. The use of the above transforms reduces the problem of controlling the magnitude and frequency of three ac currents to a problem of controlling the magnitude of two dc currents. A further advantage is that the stator winding impedance, which is time varying in the stationary ac reference frame, becomes a fixed inductance in the rotating reference frame. This means that the current control loop dynamics become independent of the stator frequency improving the robustness of the system robustness. The input to the q-axis current controller is the torque reference, which is derived from the velocity or position control loop. The input to the d-axis controller is derived froma field current control function depending on the motor type.Figure 1. Field Oriented Control ArchitectureSince the rotor flux in a PMSM is generated by a permanent magnet, the field reference is normally set to zero. In the case of the induction motor, d-axis current is required to magnetize the core just like in a field wound dc motor. Since there is cross coupling between the q-axis and d-axis windings, the d-axis current controller must apply voltage even to maintain zero d-axis current. At some speed, known as the base speed, the required q- axis and d-axis voltage exceeds the inverter voltage capacity. Above base speed, the controller operates in field weakening mode and reduces the rotor flux to lower q-axis voltage generated by the motor windings. In the induction motor, the rotor flux is reduced by lowering the d-axis current. However, in the case of the PMSM, a negative d-axis current is required to oppose the permanent magnet field in order to weaken the rotor flux. The PMSM is more efficient than an induction motor at low speeds because it does not require magnetizing current but the induction motor draws lower current at high speeds.III. R OTOR F LUX ANGLE ESTIMATIONPermanent magnet synchronous motors with rotor position sensors have been used in high end industrial drives for many years. In sensorless drive systems, a motor circuit model estimates the rotor flux λr from the measured stator currents and applied voltages. The two-phase equivalent circuit model for a PMSM yields two equations (1) that are sine and cosine functions of the rotor flux angle θr . Integration of the voltage equations yields flux estimates (2) that are sine and cosine functions of the rotor angle. The flux estimates drive a phase locked loop that extracts the rotor angle and velocity. The rotor angle feedback enables field oriented control of stator currents while the rotor velocity feedback enables closure of the outer velocity loop.()()()()inductanceand resistance stator the are currents and tages stator vol phase two the are ,,,where,sin ...cos ...s s r r d di S S r r dt d dt di S S ,L r i i v v L i r v L i r v βαβαββααθλθλβα++=++= (1)()()()()βββαααθλθλi L dt i r v i L dt i r v ssrr ssr r ...sin ....cos .∫∫−−=−−= (2) Field oriented control of a PMSM is easily implemented because the rotor flux angle is locked to the rotor position. The rotor flux angle is not easily measured in an induction motor because it is not synchronized to the rotor shaft angle or speed. However, the above rotor flux angle estimation technique applies equally well to an induction machine [3]. In this case, the winding inductance term L s ’, in the motor circuit model also includes contributions from the rotor circuit (3).inductanceself rotor the is inductance g magnetizin the is inductance self stator the is where,2'r m S rms sL L L L L L L −= (3) This flux angle estimate enables direct field oriented control of an induction motor where the motor torque is proportional to the product of the rotor flux magnitude λr and the q-axis stator current i qs . The velocity estimate cannot be directly used to close the outer velocity loop because of rotor slip is required for the motor to deliver torque. However, the motor shaft velocity can be calculated under field orientation since there is a linear relationship (4) between the motor slip s and the q-axis stator current i qs .resistancerotor the is where,...r rqsm r r e r i L L r s λω=(4)The control schematic of the rotor flux estimator and the phase locked loop (PLL) is shown in Fig. 2. A vector rotation function calculates the error between the flux angle θr and the estimated angle θest . The integrator in the PLL feedback loop ensures that it also calculates both the rotor angle and velocity. Digital implementations support precision integration without drift however, special care is still required to avoid flux integrator saturation due to dc offsets in the current sampling circuits. The actual implementation includes low frequency cut off in the flux integrator functions with a matching low frequency correction function in the PLL. Good performance can be achieved down to about 5% of motor rated speed but theangle and velocity estimates are not reliable at lower speeds.Figure 2. Flux estimator and angle and frequency PLLIV. PMSM AND I NDUCTION MOTOR STARTINGStarting the PMSM is a challenge with sensorless control because the flux estimator does not provide information at zero speed. Starting the induction motor is easier but starting torque is low with open loop volts per hertz control. The approach tooptimized starting of both motors is similar and so a common starting controller is used. There is a three stage starting sequence: dc injection, open loop velocity ramp and closed loop operation. The startup controller, shown in Fig. 3, manages the operation of the PLL switching it from open loopto closed loop modes based on the flux magnitude output |λr | of the vector rotator. It also selects the reference inputs d-axis andq-axis current loops as a function of the operating mode.Figure 3. PLL starting controlThe dc injection phase, also know as parking, aligns the PMSM rotor to a known angle. The starting controller sets the initial value of the angle integrator to the parking angle θinit and the d-axis reference current i d * to the parking current i park . The flux integrator is also primed with initial rotor flux values λinit based on the parking angle. When the controller applies the parking current, the PM rotor aligns with the d-axis stator flux. The magnitude and duration of the parking current pulse is adjusted to match the shaft load characteristics. The parking phase maximizes the starting torque after the controller switches to open loop velocity ramp control and so improves starting reliability. In the case of the induction motor, the dc injection phase magnetizes the rotor core before starting. The parking current value is set to the motor magnetizing current while the parking time is matched to the rotor time constant. In the second phase, the q-axis reference current i q * is set at a fixed value i start in order to deliver a constant accelerating torque. The starting controller drives the integrator in the PI compensator with a fixed value matching the acceleration rate αinit set by the starting torque. The starting controller switches the PLL into closed loop mode when the motor reaches the minimum speed at which the flux integrator can reliably operate. The controller tests for start failure by comparing the rotor flux magnitude |λr | with predicted values.In ideal conditions with zero static torque, the dc injection current will park the PM rotor at an angle where the motor torque is zero. However, when there is non-zero static load, there will be a parking angle error. Fig. 4 helps demonstrate that the drive can tolerate relatively high static starting torque. The parking torque T park is a sine function of the angular distance from the parking position while the starting torque T park is a cosine function of the error in the parking angle. In the case where the static torque is equal to 50% of the maximum available parking torque the parking error εpark will be 30o (=sin -1(0.5)) which only reduces starting torque to 87% (=cos(30o )) of its maximum value. For most initial rotor angles,the parking torque will move the rotor towards the parking angle until the parking torque matches the static load torque. However, there is a dead zone around ±180o for initial rotor angles where the static torque will prevent any movement towards the parking position causing the initial starting torque to be in the reverse direction. A two stage parking scheme with a second parking angle advanced by 60o from the first positionwill avoid this problem.Figure 4. Parking error and the initial starting torqueIn a perfectly tuned system, the rotor angle will track the PLL angle in the open loop velocity ramp phase. The open loop starting acceleration is predicted from the starting current, the motor torque constant and the load inertia. However, the load inertia is difficult to characterize and real application loads have static friction. The starting process is tolerant to errors in the starting acceleration prediction as long as it is lower than the correct value. In this case, the initial acceleration will cause the rotor angle to move ahead of the angle predicted by the PLL resulting in a reduction in the generated torque. The system reaches equilibrium when the angle advances to the point at which the resultant acceleration falls to the predicted value. When the PLL switches to closed loop mode it will correct any angle offset angle generated during the open loop velocity ramp phase. V.S ENSORLESS AC MOTOR C ONTROL USING THE M OTIONC ONTROL E NGINEThe sensor-less field oriented control algorithms for both motor types are implemented on new digital control architecture described in Fig. 5 [4]. The ICs Motion Control Engine (MCE) includes digital control ASIC modules to implement the algorithm control calculations. MCE sequencer instructions stored in RAM enable flexibility while dedicated ASIC implementations of motor control functions significantly speed up algorithm execution. The MCE control modules are connected to hardware interface blocks that include a three-phase space vector PWM unit and an analog subsystem to measure the motor currents. The PWM timing unit generates the power inverter gating signals and the A/D sample timing signals required to reconstruct the motor winding current from the dc link current. The flux angle estimator, as described previously, calculates the rotor flux angle from the applied v α and v β voltages and measured i α and i β currents. This angledrives the vector rotation blocks that allow the current loop tobe closed in the rotating reference frame.Figure 5. Digital Control ICThe control schematics for the PMSM controller and the induction motor controller are shown in Fig. 6 and Fig. 7. The field oriented current control loops are identical in structure in both systems. The outer velocity loop is also identical for both drives; but for the induction motor, the slip frequency is subtracted from the rotor flux frequency to calculate the motor speed. The PMSM does not require field current so the d-axis current reference can be set to zero. However, a negative d-axis current enables extended speed range operation under so called “field weakening control”. The opposite is the case for the induction motor. The d-axis reference current is set to the magnetizing current at low speeds but is reduced at higher speeds to enable field weakening control. The field weakening controller determines the d-axis current that will optimize the use of the dc bus based on the outputs of the currentcompensators.Figure 6. Sensorless PMSM drive algorithmFigure 7. Sesorless IM drive algorithmGraphical design tools supporting the IRMCK341 digital control IC enables easy modification of the outer velocity loop and field control functions. These tools have also been used by washing machine manufacturers to add special functions to support load out of balance detection before entering the spin cycle. A hardware reference design demonstrates recommended circuit layout for the dc link current signal. Thisis the most critical part of the circuit design because this signalis the only source of information on motor current, rotor flux position and velocity.VI.WASHING MACHINE M OTOR P ERFORMANCECOMPARISONThis section describes results for a PMSM and an induction motor evaluated for use in a front loader washer. Both motors met the washer application requirements including torque speed range and speed accuracy using sensorless control. Fig. 8 shows an example of the variation in the PMSM motor current during a wash cycle. The current varies significantly during the cycle as the drum lifts the cloths then lets them fall to the bottom of the drum. The current variation is quite unpredictable during the cycle depending on how the cloths become lumped together. The test data presented here compares starting performance and efficiency during washing. Both motors run the washer drum via a 15:1 belt drive where the typical washing speed is 47 RPM corresponding to a motor speed of approximately 700 RPM. The maximum spin speed of 1100 RPM requires a motor shaft speed of 16,500 RPM which iswell over 3 times base speed.Figure 8. Motor currents during wash cycleDynamometer test data is presented to allow a comparison in drive performance during washing. Fig. 9 and Fig. 10 show the starting performance of the PMSM and IM with a target speed of 470 RPM. The PMSM achieves a staring torque of 2.7 Nm, which exceeds the application requirements. The maximum motor starting current is 6 Arms and the total starting time was 2 seconds. The induction motor achieves a higher starting torque of 3.4 Nm with a maximum starting current of 5.6 Arms and a starting time of less than 1 second. Both motors met the application specification but the inductionmotor performed better.Figure 9. PMSM startup test with 2.7 Nm loadFigure 10. Induction motor starting with 3.4 Nm loadFig. 11 and Fig. 12 show the motor efficiencies at washing speed over the application torque range. The induction motor efficiency drops to less than 40% at the maximum torque range while the PMSM efficiency is still above 70% at this point. The inverter efficiency is almost 90% at the maximum torque range for both motors. This is expected since the rated currents for both motors are almost same.Figure 11. PMSM efficiency at 700 RPMFigure 12. Induction motor efficiency at 700 RPM The motor efficiency is a minor contributor to washing machine energy efficiency rating since the energy consumed by the motor is still significantly less than the energy consumed in heating the hot water used. However, since the PMSM is more efficient it can be made smaller and therefore may cost less to build than the induction motor. The PMSM efficiency is a little lower at spin speeds (16500 RPM) because field weakening consumes most of the current but this is still higher than the induction motor. It was not possible to take dynamometer efficiency measurements for the induction motor at spin speeds but the drive operates in this mode for a relatively short time.VII.W ASHING M ACHINE C ONTROL S YSTEM While the motor control function is a key part of the washing control system, the washing machine application software adds most the appliance product features. The IRMCF341 washing machine control IC integrates an independent 8-bit microcontroller for the application layer functions. This solution preserves all the advantages of having an independent application layer processor that can control the relatively slow washing process without having to compete for system resources required by the faster motor algorithm. The 8-bit microcontroller loads the control loop parameters into the dual port RAM and special control registers in the ASIC control modules. It also loads sequencing code into the MCE program memory to define the motor control algorithm. This makes is possible switch between induction motor control and PMSM control algorithms without changing the drive hardware. The 8-bit microcontroller can also access the internal algorithm variables such as speed and torque current required to implement washing machine functions such as wash load detection or out of balance measurements. In the case of the PMSM drive, it is possible to optimize starting parameters to match different wash load conditions and so minimize starting currents.VIII.C ONCLUSIONThe paper presented two implementations of field oriented control using a common control platform. Both the induction motor and the PMSM meet the washer application requirements. The induction motor has a higher starting torque than the PMSM but has a much lower efficiency in washing mode. The use of sensorless field oriented control using dc link current feedback enables the use of a single drive platform to control either motor.R EFERENCES[1]Bianchi, A. and Buti, L. “Three-Phase A.C. Motor Drive and Controllerfor Clothes Washer” Appliance Magazine, June 2003[2]Krause, P.K., Wasynczuk, O. and Sudhoff, S.D. “Analysis of ElectricMachinary and Drive Systems” 2nd Edition, IEEE Press 2002[3]Novotny, D.W. and Lipo, T. A. “Vector Control and Dynamics of ACdrives” Oxford University Press 1996 pp 257-282[4]Murray, A. and Ho, E. “New Motion Control Architecture SimplifiesWashing Machine Control System Development” IAS 2006 Conferencer Record pp 1229-1234。