酶法生产HT
生物酶法制备淫羊藿素工艺条件的研究
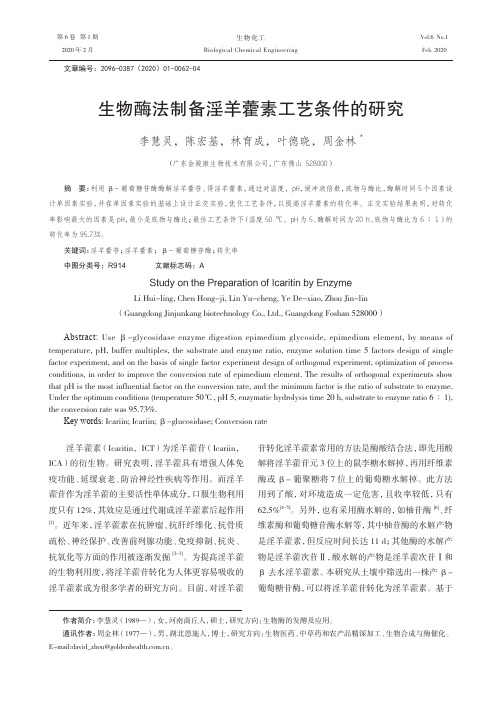
文章编号:2096-0387(2020)01-0062-04
Vol.6 No.1 Feb. 2020
生物酶法制备淫羊藿素工艺条件的研究
李慧灵,陈宏基,林育成,叶德晓,周金林 *
(广东金骏康生物技术有限公司,广东佛山 528000)
摘 要:利用 β- 葡萄糖苷酶酶解淫羊藿苷、得淫羊藿素,通过对温度,pH,缓冲液倍数,底物与酶比,酶解时间 5 个因素设
HT-110X30 水浴摇床,上海赫田科学仪器有限 公司,安捷伦 1100 液相色谱仪,安捷伦科技(中国) 有限公司。 1.2 液相检测条件
色 谱 柱:C18 柱 ODS,250×4.6 mm;流 动 相: 乙 腈(流 动 相 A),0.5% 醋 酸 水 溶 液(为 流 动 相) B;流 速 1 mL/min,波 长 281 nm,柱 温 30 ℃,进 样 量 10μL;洗 脱 程 序 中 流 动 相 A 与 B 的 比 例 按 照 30 ∶ 70(0~10 min)、50 ∶ 50(10~20 min)、 85 ∶ 15(20~25 min)、30 ∶ 70(25~30 min)的 洗 脱条件设置。 1.3 数据分析
·63·
此,通过单因素实验和正交实验优化此种酶的酶解工 艺,以提高淫羊藿素的转化率。
1 材料与方法
1.1 药剂与仪器 98% 淫羊藿苷(西安天本生物工程有限公司),
β- 葡 萄 糖 苷 酶(本 公 司 自 产),淫 羊 藿 苷 标 准 品 (≥ 98%)、淫羊藿素标准品(≥ 98%)均购自 sigma 公司,一水柠檬酸(≥ 99.5%)、十二水合磷酸氢二钠 (≥ 99%)购自广东光华科技股份有限公司。
苷转化淫羊藿素常用的方法是酶酸结合法,即先用酸 解将淫羊藿苷元 3 位上的鼠李糖水解掉,再用纤维素 酶或 β- 葡聚糖将 7 位上的葡萄糖水解掉。此方法 用到了酸,对环境造成一定危害,且收率较低,只有 62.5%[4-5]。另外,也有采用酶水解的,如柚苷酶 [6]、纤 维素酶和葡萄糖苷酶水解等,其中柚苷酶的水解产物 是淫羊藿素,但反应时间长达 11 d;其他酶的水解产 物是淫羊藿次苷Ⅱ,酸水解的产物是淫羊藿次苷Ⅰ和 β 去水淫羊藿素。本研究从土壤中筛选出一株产 β葡萄糖苷酶,可以将淫羊藿苷转化为淫羊藿素。基于
酶法工艺技术

酶法工艺技术酶法工艺技术是一种利用酶催化反应来制取某种化学物质的技术。
酶作为一种高效、特异性的生物催化剂,可以在温和的条件下加速反应的进行,并具有高产率和选择性等优点。
众多的酶法工艺技术在食品、医药、化工和环保等领域中得到了广泛应用。
酶法工艺技术具有独特的优点。
首先,酶是一种天然存在的生物催化剂,可以在中性或弱碱性条件下进行催化反应,而不需要使用高温、高压等极端条件。
相比传统的化学反应,酶法工艺技术能够节约能源,减少生产成本,降低环境污染。
其次,酶具有高效率和选择性。
由于酶对底物具有高度的特异性识别和结合能力,可以将底物转化为特定的产物。
在工业生产中,利用酶法工艺技术可以达到高产率和高选择性的要求,从而提高产品的纯度和质量。
再次,酶法工艺技术具有较好的可控性。
通过调节反应物浓度、温度、pH值和反应时间等参数,可以对酶的反应进行精确控制,从而实现对反应过程的调控。
这种可控性使得酶法工艺技术在研发新产品、合成复杂化合物以及优化传统工艺等方面具有广阔的应用前景。
酶法工艺技术在食品加工中得到了广泛应用。
例如,在奶制品加工中,通过添加酶可以加速牛奶中的乳糖分解,从而产生乳糖不耐受者所需的乳糖酶。
此外,酶法还可以用于葡萄酒、啤酒以及面包等食品的生产中,改善产品的质量和口感。
在医药领域,酶法工艺技术可以用于合成药物中的药物前体或中间体,提高药物的纯度和产率。
目前,已经开发出许多基于酶法的药物制备技术,如酶催化合成抗生素青霉素和血栓溶解酶等。
在化工领域,酶法工艺技术可以用于优化传统的合成工艺。
通过酶的催化反应,可以减少废物的产生,提高化学反应的选择性和产率。
此外,酶还可以用于有机合成中的不对称合成,从而获得具有药理活性的手性产物。
在环保领域,酶法工艺技术被广泛应用于废水处理和废物降解等方面。
酶可以加速有害物质的降解和转化,从而实现废物的资源化利用和减少环境污染。
总而言之,酶法工艺技术是一种高效、可控和环保的化学合成方法。
L-色氨酸的研究进展
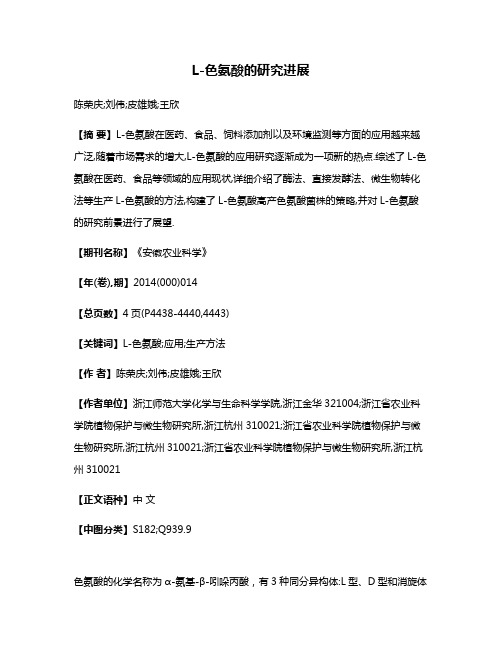
L-色氨酸的研究进展陈荣庆;刘伟;皮雄娥;王欣【摘要】L-色氨酸在医药、食品、饲料添加剂以及环境监测等方面的应用越来越广泛,随着市场需求的增大,L-色氨酸的应用研究逐渐成为一项新的热点.综述了L-色氨酸在医药、食品等领域的应用现状,详细介绍了酶法、直接发酵法、微生物转化法等生产L-色氨酸的方法,构建了L-色氨酸高产色氨酸菌株的策略,并对L-色氨酸的研究前景进行了展望.【期刊名称】《安徽农业科学》【年(卷),期】2014(000)014【总页数】4页(P4438-4440,4443)【关键词】L-色氨酸;应用;生产方法【作者】陈荣庆;刘伟;皮雄娥;王欣【作者单位】浙江师范大学化学与生命科学学院,浙江金华321004;浙江省农业科学院植物保护与微生物研究所,浙江杭州310021;浙江省农业科学院植物保护与微生物研究所,浙江杭州310021;浙江省农业科学院植物保护与微生物研究所,浙江杭州310021【正文语种】中文【中图分类】S182;Q939.9色氨酸的化学名称为α-氨基-β-吲哚丙酸,有3种同分异构体:L型、D型和消旋体DL型[1]。
1825年,色氨酸首次被发现,1902年,由Hokinst首次从酪蛋白中分离获得。
其分子式为C11H12N2O2,分子量为204.23,白色晶体或者微黄色片状晶体或粉末,无味,右旋体或有特殊甜味,溶于水或者热乙醇,不溶于氯仿、乙醚,在稀酸或稀碱中较稳定。
与水一起加热,会产生少量吲哚,在有NaOH、CuSO4存在的条件下加热,则会分解产生大量吲哚。
若迅速加热至210℃时颜色会变黄,290℃时发生分解反应,长时间光照则会发生着色反应。
L-色氨酸结构式见图1。
图1 色氨酸结构式L-色氨酸是人体和动物所必需的8种必需氨基酸之一,不能通过自身合成。
所以,人和动物只能通过食物来摄取L-色氨酸。
D-色氨酸主要存在于植物和微生物之中,动物中含量极少,而且在人体内几乎不发生代谢作用,也无毒性[2]。
酶的生产方法
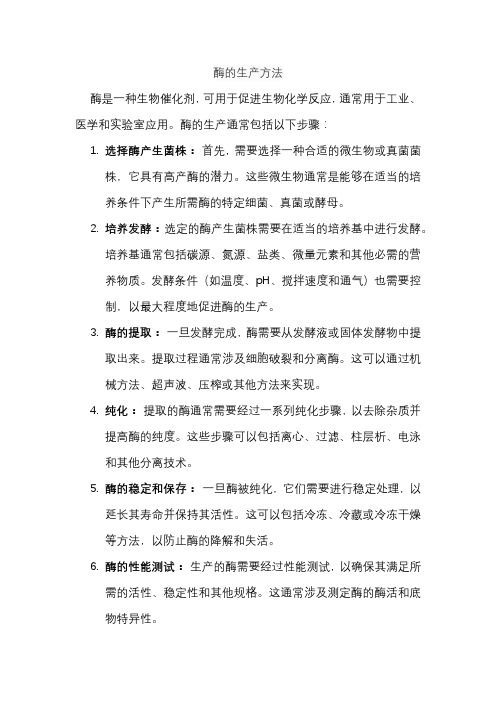
酶的生产方法酶是一种生物催化剂,可用于促进生物化学反应,通常用于工业、医学和实验室应用。
酶的生产通常包括以下步骤:1.选择酶产生菌株:首先,需要选择一种合适的微生物或真菌菌株,它具有高产酶的潜力。
这些微生物通常是能够在适当的培养条件下产生所需酶的特定细菌、真菌或酵母。
2.培养发酵:选定的酶产生菌株需要在适当的培养基中进行发酵。
培养基通常包括碳源、氮源、盐类、微量元素和其他必需的营养物质。
发酵条件(如温度、pH、搅拌速度和通气)也需要控制,以最大程度地促进酶的生产。
3.酶的提取:一旦发酵完成,酶需要从发酵液或固体发酵物中提取出来。
提取过程通常涉及细胞破裂和分离酶。
这可以通过机械方法、超声波、压榨或其他方法来实现。
4.纯化:提取的酶通常需要经过一系列纯化步骤,以去除杂质并提高酶的纯度。
这些步骤可以包括离心、过滤、柱层析、电泳和其他分离技术。
5.酶的稳定和保存:一旦酶被纯化,它们需要进行稳定处理,以延长其寿命并保持其活性。
这可以包括冷冻、冷藏或冷冻干燥等方法,以防止酶的降解和失活。
6.酶的性能测试:生产的酶需要经过性能测试,以确保其满足所需的活性、稳定性和其他规格。
这通常涉及测定酶的酶活和底物特异性。
7.应用和销售:生产的酶可以用于各种应用,如食品加工、制药、生物燃料生产、洗涤剂制造等。
它们可以作为商业产品销售给客户,或用于内部工业生产。
酶的生产是一个复杂的过程,涉及多个步骤和技术,需要仔细控制和监测,以确保高质量的酶产品。
不同类型的酶可能需要不同的生产方法,因此具体的生产过程可能会有所不同。
酶的生产方法介绍
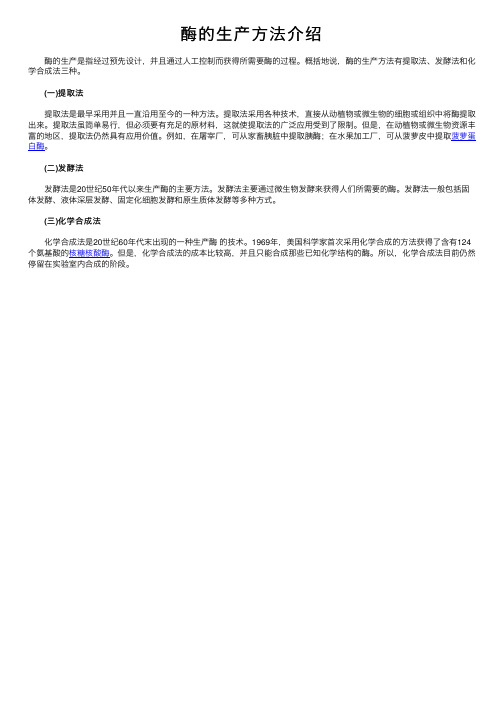
酶的⽣产⽅法介绍
酶的⽣产是指经过预先设计,并且通过⼈⼯控制⽽获得所需要酶的过程。
概括地说,酶的⽣产⽅法有提取法、发酵法和化学合成法三种。
(⼀)提取法
提取法是最早采⽤并且⼀直沿⽤⾄今的⼀种⽅法。
提取法采⽤各种技术,直接从动植物或微⽣物的细胞或组织中将酶提取出来。
提取法虽简单易⾏,但必须要有充⾜的原材料,这就使提取法的⼴泛应⽤受到了限制。
但是,在动植物或微⽣物资源丰富的地区,提取法仍然具有应⽤价值。
例如,在屠宰⼚,可从家畜胰脏中提取胰酶;在⽔果加⼯⼚,可从菠萝⽪中提取菠萝蛋⽩酶。
(⼆)发酵法
发酵法是20世纪50年代以来⽣产酶的主要⽅法。
发酵法主要通过微⽣物发酵来获得⼈们所需要的酶。
发酵法⼀般包括固体发酵、液体深层发酵、固定化细胞发酵和原⽣质体发酵等多种⽅式。
(三)化学合成法
化学合成法是20世纪60年代末出现的⼀种⽣产酶的技术。
1969年,美国科学家⾸次采⽤化学合成的⽅法获得了含有124个氨基酸的核糖核酸酶。
但是,化学合成法的成本⽐较⾼,并且只能合成那些已知化学结构的酶。
所以,化学合成法⽬前仍然停留在实验室内合成的阶段。
酶法生产工艺

酶法生产工艺酶法生产工艺是一种利用酶催化反应来进行生物转化的工艺。
酶作为生物催化剂,具有高效、特异、温和等优点,因此在工业生产中应用广泛。
下面将介绍一种常见的酶法生产工艺。
以酶法生产果糖为例:1. 原料准备:选择含有果糖的寡糖、低聚糖或淀粉等作为原料,将其进行粉碎、过筛等处理,以提高反应效率。
2. 酶制剂配方:根据反应需要,选择适当的酶制剂,比如果糖酶。
将酶制剂与适量的辅助物质如缓冲剂、金属离子等按一定比例混合,制成酶液。
3. 反应装置:选择合适的反应装置,如发酵罐、反应釜等。
根据反应规模和条件要求,进行装置的设计和选型。
4. 反应条件控制:调节反应温度、pH值、酶浓度等反应条件,以最大限度地提高反应效率和产物纯度。
5. 反应操作:将原料添加到反应装置中,加入预先配制好的酶液,通过搅拌等方式促进反应进行。
控制反应时间和反应速率,使反应充分进行。
6. 反应结束:根据反应时间或反应物浓度的变化,确定反应结束时机。
停止反应,通过加热、调节pH值等方式使酶活性失活,停止反应过程。
7. 分离纯化:将反应产物与反应物、副产物等进行分离。
常用的分离纯化方法有过滤、离心、溶剂萃取、蒸馏等。
8. 产品收集:收集分离纯化后的果糖产物,进行检测和分析,判断产品质量和含量。
9. 后续处理:对产品进行进一步的加工和处理,如浓缩、干燥、粉碎等,以得到符合要求的最终产品。
10. 废弃物处理:对反应过程中产生的废弃物进行处理,以减少环境污染和资源浪费。
酶法生产工艺具有操作简便、生产周期短、反应条件温和等优点,广泛应用于食品工业、制药工业、化工工业等领域。
随着生物技术的不断发展,酶法生产工艺在未来将拥有更广阔的应用前景。
ht筛选的原理

HT筛选(High-Throughput Screening)是一种用于高通量药物筛选的技术,其原理主要涉及以下几个步骤:
1. 样本准备:在进行HT筛选之前,需要准备一系列待测试的化合物样本。
这些化合物通常存储在微孔板(microplate)中,每个孔中含有一个化合物。
2. 选择靶标:HT筛选的第一步是确定要测试的药物靶标,这是药物的作用目标,通常是某种疾病的关键蛋白质或生物分子。
3. 测试系统:建立适当的测试系统,这是一种模拟目标蛋白质在体内功能的体外实验系统。
测试系统可以是细胞培养物、酶反应体系、蛋白质结晶等,具体取决于靶标的性质。
4. 筛选过程:将待测试的化合物样本添加到测试系统中,让其与目标蛋白质或生物分子相互作用。
这些化合物会影响靶标的功能,可能抑制或促进其活性,从而对测试系统产生反应。
5. 检测方法:使用高通量检测技术,如荧光探针、酶活性分析、细胞增殖/毒性检测等,来检测化合物样本与靶标的相互作用和对测试系统的影响。
6. 数据分析:收集并记录所有筛选得到的数据。
通常,药物筛选实验会产生大量的数据,因此需要进行有效的数据分析和处理,以确定哪些化合物对靶标具有潜在的生物活性。
7. 确认与优化:在HT筛选结束后,通过进一步的实验验证和优化,确定最有潜力的化合物,使其具备更好的选择性、药物活性和药物性质。
HT筛选技术的优势在于其高效率和高通量性质,能够在相对短的时间内对大量化合物进行测试,从中筛选出有可能成为药物候选的化合物。
这对于新药物研发和药物发现领域具有重要意义。
酶法生物制取饮料的研制
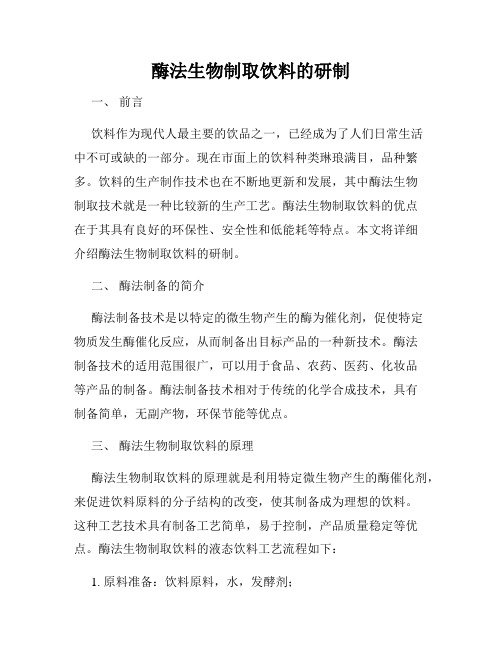
酶法生物制取饮料的研制一、前言饮料作为现代人最主要的饮品之一,已经成为了人们日常生活中不可或缺的一部分。
现在市面上的饮料种类琳琅满目,品种繁多。
饮料的生产制作技术也在不断地更新和发展,其中酶法生物制取技术就是一种比较新的生产工艺。
酶法生物制取饮料的优点在于其具有良好的环保性、安全性和低能耗等特点。
本文将详细介绍酶法生物制取饮料的研制。
二、酶法制备的简介酶法制备技术是以特定的微生物产生的酶为催化剂,促使特定物质发生酶催化反应,从而制备出目标产品的一种新技术。
酶法制备技术的适用范围很广,可以用于食品、农药、医药、化妆品等产品的制备。
酶法制备技术相对于传统的化学合成技术,具有制备简单,无副产物,环保节能等优点。
三、酶法生物制取饮料的原理酶法生物制取饮料的原理就是利用特定微生物产生的酶催化剂,来促进饮料原料的分子结构的改变,使其制备成为理想的饮料。
这种工艺技术具有制备工艺简单,易于控制,产品质量稳定等优点。
酶法生物制取饮料的液态饮料工艺流程如下:1. 原料准备:饮料原料,水,发酵剂;2. 混合原料,得到发酵液;3. 进行微生物发酵,4. 过滤去杂质;5. 消毒,并装瓶、封口;四、酶法生物制取饮料的优点1. 可以用于制备众多的饮料原料,包括绿茶、花果饮料、果蔬汁等;2. 制备时无需添加化学反应剂,避免了污染环境的可能;3. 酶对环境的影响小,制备途中用水较少,可以节约能源;4. 制备的饮料不仅美味,而且更健康。
五、酶法生物制取饮料技术的应用1. 酶法生物制取绿茶饮料。
绿茶中的茶多酚成分对人体有益,酶法生物制取绿茶饮料可以让绿茶中的茶多酚更好的溶解在水中,使得喝下后对人体更有益处,营养价值更高。
2. 酶法生物制取花果饮料。
如草莓、葡萄等水果外皮中的果胶是人体健康的重要营养素,而酶法生物制取可以将果胶完全溶解在果汁中,增强了饮料的口感和健康价值。
3. 酶法生物制取果蔬汁。
酶法生物制取可以让果蔬中无法被人体吸收的营养物质释放出来,使得果蔬汁中营养更足,营养价值更高。
- 1、下载文档前请自行甄别文档内容的完整性,平台不提供额外的编辑、内容补充、找答案等附加服务。
- 2、"仅部分预览"的文档,不可在线预览部分如存在完整性等问题,可反馈申请退款(可完整预览的文档不适用该条件!)。
- 3、如文档侵犯您的权益,请联系客服反馈,我们会尽快为您处理(人工客服工作时间:9:00-18:30)。
Short CommunicationEnzymatic routes for the production of mono-and di-glucosylated derivatives of hydroxytyrosolAntonio Trincone,Eduardo Pagnotta,Annabella Tramice ⇑Istituto di Chimica Biomolecolare,Consiglio Nazionale delle Ricerche,Via Campi Flegrei 34,80072Pozzuoli,Naples,Italya r t i c l e i n f o Article history:Received 30August 2011Received in revised form 20October 2011Accepted 21October 2011Available online 30October 2011Keywords:a -Glucosidase Aplysia fasciata Transglycosylation Hydroxytyrosol Biocatalysisa b s t r a c tIn this work,a new eco-friendly procedure for the synthesis of hydroxytyrosol and tyrosol a -glycosidic derivatives was proposed by using the marine a -glucosidase from Aplysia fasciata ,and a commercial tyrosinase from mushroom for the bioconversion of tyrosol glycosidic derivatives into the corresponding hydroxytyrosol products.New hydroxytyrosol mono-and di-saccharide derivatives were synthesized at final concentrations of 9.35and 10.8g/l of reaction,respectively,and their antioxidant activity was evaluated by DPPH test.The best antioxidant agent resulted the (3,4-dihydroxyphenyl)ethyl-a -D -gluco-pyranoside;it showed a radical scavenging activity similar to that of the hydroxytyrosol,together with an increased hydrosolubility.This molecule could be a good response to many food industry demands,always in search of cheap antioxidants with nutritional properties to improve the nutritional value and the quality of foods.Ó2011Elsevier Ltd.All rights reserved.1.IntroductionOlive biophenols have attracted the attention of food and phar-maceutical industries first of all for their well-acquainted antioxidant activity (Obied et al.,2005).2-(4-Hydroxyphenyl)etha-nol (tyrosol,1)and 3,4-dihydroxyphenyl ethanol (hydroxytyrosol,2)represent the most abundant oil phenols (Vissers et al.,2004).Hydroxytyrosol,as well as being a powerful antioxidant and scavenger of free radicals,reduces,in fact,the risk of coronary heart disease and atherosclerosis (Visioli et al.,1995,2002)and it is involved in a mechanism of protection against oxidative DNA damage (Waterman and Lockwood,2007).Differently,tyrosol shows milder antioxidant properties (Damiani et al.,2003).Never-theless,it exerts a powerful protective effect against oxidative inju-ries in cell systems and improves the intracellular antioxidant defence systems (Mateos et al.,2008).In spite of their potential applications in the nutraceutical and pharmaceutical fields,few methods have been developed for synthesizing tyrosol and hydroxytyrosol glycosidic derivatives.In this paper,we screened the possibility to perform glucosyla-tion reactions of various phenolic compounds,including tyrosol and hydroxytyrosol and their structurally analogous compounds,by using the marine a -glucosidase from Aplysia fasciata .Interesting glucosylations at phenolic sites of some selected acceptors were ob-served,especially considering that phenolic hydroxyls are ineffi-ciently glycosylated by glycosidases (van Rantwijk et al.,1999).Among all molecules tested,tyrosol and hydroxytyrosol glycosyla-tion procedures were more deeply investigated.Tyrosol a -glycosidic derivatives were efficiently produced by direct glucosylation and in a second enzymatic step,these molecules were regioselectively oxi-dized by a commercial mushroom tyrosinase to give the hydroxyty-rosol a -glycosyl derivatives,possessing interesting radical scavenging activities.These results appeared of great interest when compared to enzymatic synthesis of salidroside,monoglucuronides derivatives of hydroxytyrosol,and tyrosol,previously reported in literature (Tong et al.,2004;Khymenets et al.,2006).2.Methods 2.1.GeneralTLC solvent systems:(A)(CH 3CN:H 2O,8:2,v/v);(B)(CH 3CN:H 2O,9:2,v/v).Compounds on TLC plates were visualized under UV light or charring with a -naphthol reagent.Other technical information were reported in Supplementary Section S.1.2.2.Enzyme sourceA clear enzymatic homogenate from A.fasciata visceral mass was prepared as previously described by Andreotti et al.(2006).Since the most abundant hydrolytic enzyme in A.fasciata visceral mass extract was an a -D -glucosidase activity,this enzy-matic solution (8.1mg total protein/ml;1.2U/mg,using p -nitro-0960-8524/$-see front matter Ó2011Elsevier Ltd.All rights reserved.doi:10.1016/j.biortech.2011.10.073Corresponding author.Tel.:+390818675070;fax:+390818041770.E-mail address:atramice@r.it (A.Tramice).phenyl a-D-glucopyranoside as substrate)was considered useful for this work purpose.One unit of a-D-glucosidase activity was defined as that amount of enzyme required to catalyze the release of1.0l mol of p-nitro-phenol per minute.Commercial mushroom tyrosinase(3900U/mg)from Sigma was used without further purification.2.3.Substrate specificity in transglycosylation reactions2-Phenylethanol,tyrosol and its similar compounds such as 2-(2-hydroxyphenyl)ethanol,2-(3-hydroxyphenyl)ethanol,hydro xytyrosol,(±)-1-phenyl-1,2-ethanediol,R(À)-and S(+)-1-phenyl-1,2-ethanediol,4-(2-methoxyethyl)phenol,N-acetyl-L-tyrosine ethyl ester monohydrate were tested as acceptors in analytical-scale transglycosylation reactions.Visceral mass homogenate from A.fasciata(16l l,0.153U of a-D-glucosidase activity)was added to1.5ml of10mM acceptorsolutions in50mM K-acetate buffer at pH 5.5,containing 150mM maltose.Enzymatic reactions were conducted under mag-netic stirring at34°C for48h up to maltose consumption and reg-ularly monitored by TLC analysis(system solvent A).2.4.Optimization of tyrosol and hydroxytyrosol glycosylation reactionsGlycosylation reactions were performed at different maltose (donor)molar excesses with respect to tyrosol(1)or hydroxytyro-sol(2)(acceptors).All reactions were carried out on analytical scale(total volume 1.5ml)in K-acetate buffer(50mM,pH5.5)at34°C,under mag-netic stirring,for48h and in presence of0.72U of a-D-glucosidase from A.fasciata per mmol of maltose,aiming to maintain a con-stant ratio between the enzymatic units and the mmol of initial maltose.Aliquots of200l l of each reaction were withdrawn at different time intervals(from t0to48h),stopped by enzyme denaturation at 100°C for2min and lyophilized.Collected aliquots were dissolved in D2O and analyzed by1H NMR experiments.In afirst set of reactions,acceptor(comp.1or2)concentration wasfixed at5mM and maltose amount was varied according to specific molar excesses with respect to acceptor.In tyrosol exper-iment,maltose:tyrosol molar ratios were 1.5,15,30,60;in hydroxytyrosol experiment,maltose:hydroxytyrosol molar ratios became5,15,30,60.In a second set of reactions,tyrosol was se-lected as sole acceptor and used at increasing concentrations(5, 10,30,60and180mM);transglycosylation reactions were performed at a maltose:tyrosolfixed molar ratio of15:1.2.5.Preparative enzymatic reactionsProduction of tyrosol and hydroxytyrosol glycosylated deriva-tives and their isolation and spectroscopic characterization were described in detail in Supplementary(Sections S.2.1and S.2.2). Tyrosol glycosides were produced starting from a tyrosol solution 180mM containing maltose with a molar excess of15(2.7M) and in presence of 1.94U of a-glucosidase from A.fasciata (0.2ml of enzymatic homogenate).2.6.Oxidation reactionsHydroxytyrosol(2)production was performed using commer-cial mushroom tyrosinase from Sigma,as reported in a previous paper(Espín et al.,2001).Process details were described in Supplementary(Section S.3.1).2-(4-Hydroxyphenyl)ethyl-a-D-glucopyranoside(1a),2-(4-hyd roxyphenyl)ethyl-a-D-maltoside(1b),and2-(4-hydroxyphenyl) ethyl-a-D-isomaltoside(1c),were oxidized to the corresponding hydroxytyrosol derivatives by a synthetic procedure reported in Supplementary Section S.3.2.2.7.Free-radical scavenging activityThe scavenging activity of hydroxytyrosol and its a-glucosyl,a-maltosyl and a-isomaltosyl derivatives was analyzed by using1,1-diphenyl-2-picrylhydrazyl(DPPH)essay.The reaction mixture contained0.75ml of substrate methanolic solutions at differentfi-nal concentrations(16.7,10,5and1.67l M)and1.5ml of50mM DPPH(final concentration33l M).After shaking,reaction systems were kept for10min in the dark,and then the absorbance at 517nm was measured.Experiments were carried out in triplicate and always on freshly made up solutions.Appropriate controls were performed by mixing0.75ml of MeOH(blank solution)with 1.5ml of DPPH solution.EC50of molecules,the amount of antiox-idant needed to decrease by50%the initial radical concentration, were evaluated.All experimental data of absorption decrease showed a linear correlation as to the concentration of the antioxi-dant compounds(straight line resulting from thefit by linear regression,r20.99–0.97);each point was the average of three determinations(SD<0.05).3.Results and discussion3.1.Substrate specificity in transglycosylation reactionsIn thefirst part of this work,the ability of a-D-glucosidase from A.fasciata to glycosylate various naturally occurring phenolic com-pounds with alcoholic and/or phenolic hydroxyls in their molecular skeleton(Section2.3),was analyzed and the regio-and chemo-selectivity in their transglycosylation processes were qualitatively examined.Reaction monitoring was carried out using a TLC system that we succeeded in discriminating between glycosylated prod-ucts(mono-or di-saccharides)in phenolic or alcoholic positions.A different qualitative distribution of products at4,24and48h was recorded.Accumulation of mono-and di-saccharidic deriva-tives was noticed for almost all acceptors up to24h.In many cases, glycosylations were not chemo-selective with the phenolic and alcoholic hydroxyls involvement,but after24h,a prevalent recov-ery of products glycosylated in alcoholic position was observed,as in the case of hydroxytyrosol,tyrosol and its regioisomers with phenolic hydroxyls in2and3aromatic positions.Furthermore,4-(2-methoxyethyl)phenol disaccharidic products were still present at24h,while monoglucosides of2-phenylethanol and N-acetyl-L-tyrosine ethyl ester monohydrate were partially or totally hydro-lyzed,respectively.Racemic1-phenyl-1,2-ethanediol and its pure enantiomers were poly-glycosylated and partially(racemic,R(À)) or totally(S+)hydrolyzed after24h.Results of this qualitative transglycosylation survey encouraged us to more deeply investigate the glycosylation reactions using tyrosol and hydroxytyrosol as acceptors.3.2.Production of tyrosol and hydroxytyrosol glycosydic derivatives: reaction conditions optimizationAiming to identify the best conditions for glycosylating tyrosol (1)and hydroxytyrosol(2),a series of reactions were conducted at different maltose concentrations(from7.5to300mM),constant acceptor concentration(5mM)and afixed enzyme amount.Ali-quots of each reaction were collected over time and analyzed by TLC(system solvent A)and1H NMR experiments.80 A.Trincone et al./Bioresource Technology115(2012)79–83Concerning tyrosol glycosylations,results were reported in Fig.1(a).Reactions B,C and D followed a similar trend up to 7h (al-most 50%of bioconversion).All reactions reached a plateau mostly at 24–31h.Process B was the most synthetically interesting reac-tion:56%of tyrosol glycosylation was achieved after 24h.Subse-quent experiments were conducted in more concentrated media to set up tyrosol glycosylation processes in which an improvement in the production efficiency of products could be obtained (Fig.1(b)).A fixed 15:1maltose:tyrosol molar ratio (B conditions,Fig.1(a)),was used.In particular,at 48h the reaction I (Fig.1(b))furnished a conversion of 70%,corresponding to 126mmol of reacted tyrosol/l of reaction.In these experimental conditions (pro-cess I),a semi-preparative reaction was performed and products were isolated and spectroscopically characterized (Supplementary Sections S.2.1and S.2.3).It was produced:(i)tyrosol monoglucosy-lated at alcoholic and phenolic positions (1a :2-(4-hydroxyphenyl)ethyl-a -D -glucopyranoside and 1b :2-(4-a -D -glucopyranosyloxy-phenyl)ethanol,in molar ratio 6.2:1respectively),with 41%of yield,corresponding to 20g of tyrosol monoglucosydes/l of reaction;(ii)53%of tyrosol diglucosylated derivatives (31g of products/l of reac-tion),of which the 78%were disaccharidic alcoholic derivatives in almost a 1:1mixture,(1c :2-(4-hydroxyphenyl)ethyl-a -D -malto-side,1d :2-(4-hydroxyphenyl)ethyl-a -D -isomaltoside)and the remaining 22%was a complex mixture of disaccharidic phenolic derivatives;(iii)6%of oligosaccharide derivatives.This final compo-sition indicated a prevalent presence of products glycosylated at primary alcoholic position,which represented the thermodynami-cally controlled products.A TLC monitoring of reaction over time showed that phenolic glucosylated product 1b was produced at the beginning of the reaction but was hydrolyzed more quickly than compound 1a .The preferential hydrolysis of phenolic monogluco-side 1b was proved by an hydrolysis experiment on a mixture 1:1of the two monosaccharidic derivatives 1a and 1b ,which was mon-itored by 1H NMR experiments,as described in Supplementary Sec-tion S.2.4.Concerning hydroxytyrosol glycosylation process,as described in Section 2.4,different results were obtained.The best conversion,with the lowest maltose molar excess (EM:30),was of 45%after 24h (data not shown).In these experimental conditions,aiming to isolate and characterize hydroxytyrosol saccharidic derivatives,we performed a semi-preparative reaction (Supplementary Section S.2.2).2.27mmol of reacted hydroxytyrosol (mono-and di-saccha-ridic derivatives)/l of reaction were produced,with a yield lower than 3.35mmol of tyrosol saccharidic derivatives/l of reaction which were obtained by the corresponding process C (67%of con-version,Fig 1(a)).Furthermore,it was discovered that hydroxytyro-sol glycosylation process was not chemo-and regio-selective at all;both alcoholic hydroxyl and two phenolic hydroxyls were involved in glycosylation processes and a mixture of all possible monogly-cosylated derivatives was identified by preparative reaction (55%of total products,2a :(3,4-dihydroxyphenyl)ethyl-a -D -glucopyran-oside,2b (3-(a -D -glucopyranosyloxy)-4-hydroxyphenyl)ethanol,2c (4-(a -D -glucopyranosyloxy)-3-hydroxyphenyl)ethanol,spectro-scopically characterized as described in Supplementary Sectiona102030405060708090100017243148Time ( h )A 1.5B 15C 30D 601020304050607080901000136242948Tyrosol conversion (%)Time ( h )bE 5F 10G 30H 60I 180tyrosol by a -D -glucosidase of Aplysia fasciata in different conditions.In (a)[tyrosol]was fixed at 5mM,working 1.5–60).In (b)maltose molar excess was fixed at 15in all reactions (E–I)conducted at different [tyrosol],calculated by the percentage ratio between the total integration value of all benzylic protonic signals of 2.76–2.68ppm for hydroxytyrosol derivatives)and the sum of integral values of the glycosylated derivatives hydroxytyrosol (2.65ppm).A.Trincone et al./Bioresource Technology 115(2012)79–8381S.2.3),together with a complex assortment of disaccharidic com-pounds.For these reasons,this synthesis was not estimated syn-thetically useful for the production of glycosydic derivative ofhydroxytyrosol.The efficient production of glucosylated hydroxytyrosol deriva-tives was planned by starting from tyrosol and by adopting a com-mercial mushroom tyrosynase as oxidizing agent of glycosylatedderivatives of tyrosol.The employed tyrosynase was used for thesynthesis of hydroxytyrosol from tyrosol,as previously described(Espín et al.,2001);however specificity of the enzyme on deriva-tives of tyrosol was unknown.3.3.Oxidation of tyrosol saccharidic derivativesStarting from glucosylated tyrosol derivatives and by using annew environmental friendly enzymatic approach,the correspond-ing catechol derivatives were pounds1a,1c,1d,re-sulted to be substrates for the adopted commercial tyrosinase andwere efficiently oxidized to2a,2d(3,4-dihydroxyphenyl)ethyl-a-D-maltoside and2e(3,4-dihydroxyphenyl)ethyl-a-D-isomalto-side)derivatives with yields of about50–60%(SupplementarySection S.3.2).Products structures were assigned by comparisonwith reagents NMR spectra.A modification of the multiplicities,chemical shifts and number of aromatic signals in the interval7.3–6.5d in1H NMR spectra proved the o-diphenol structure ofproducts(Supplementary Section S.2.3).3.4.Radical scavenging activityThe radical scavenging activity of hydroxytyrosol derivatives(compounds2a,2d,2e)was DPPH-tested and their activities com-pared with that of hydroxytyrosol(2).(3,4-Dihydroxyphenyl)ethyl-a-D-glucopyranoside(2a)showed similar radical scavenging potency to hydroxytyrosol activity(2):their EC50were10.00and 8.94l M,respectively(Fig.2).Radical scavenging activity of our products decreased upon increasing the carbohydrate chain; hydroxytyrosol a-maltosyl and a-isomaltosyl derivatives(2d,2e) possessed similar EC50value of28.69and25.15l M respectively. Nevertheless,although the antioxidant activity in disaccharidic derivatives was inferior to compound2,it was not trivial and any-way the disaccharidic units made the molecules more water-solu-ble and chemically stable.The best results were furnished by compound2a,in which,together with an increased solubility, the scavenging activity was as good as the aglycone(2).4.ConclusionsAn efficient enzymatic two-step procedure for the synthesis of hydroxytyrosol and tyrosol a-glycosidic derivatives based on a-glucosidase from A.fasciata and a commercial tyrosinase from mushroom,was described.Considering the whole enzymatic process for the formation of glycosidic derivatives of hydroxytyrosol from tyrosol,it was possi-ble to glycosylate regioselectively only the alcoholic primary posi-tion for producing hydroxytyrosol mono-and disaccharidic derivatives,with a reaction total yield which was of20%for each product.AcknowledgementsWe thank D.Melck,E.Castelluccio and A.Esposito(NMR service of Istituto di Chimica Biomolecolare,CNR,Pozzuoli,Italy)for their skilful assistance.Appendix A.Supplementary dataSupplementary data associated with this article can be found,in the online version,at doi:10.1016/j.biortech.2011.10.073. ReferencesAndreotti,G.,Giordano,A.,Tramice,A.,Mollo,E.,Trincone,A.,2006.Hydrolyses and transglycosylations performed by purified alpha-D-glucosidase of the marine mollusc Aplysia fasciata.J.Biotechnol.122(23),274–284.Damiani,E.,Belaid,C.,Carloni,P.,Greci,L.,parison of antioxidant activity between aromatic indolinonic nitroxides and natural and synthetic antioxidants.Free Rad.Res.37,731–741.Espín,J.C.,Soler-Rivas, C.,Cantos, E.,Tomás-Barbern, F.A.,Wichers,H.J.,2001.Synthesis of the antioxidant hydroxytyrosol using tyrosinase as biocatalyst.J.Agri.Food Chem.49,1187–1193.Khymenets,O.,Joglar,J.,Clapés,P.,Parella,T.,Covas,M.-I.,de la Torre,R.,2006.Biocatalyzed synthesis and structural characterization of monoglucuronides of hydroxytyrosol,tyrosol,homovanillic alcohol,and3-(40-hydroxyphenyl)propane l.Adv.Synth.Catal.348,2155–2162.Mateos,R.,Trujillo,M.,Pereira-Caro,G.,Madrona,A.,Cert,A.,Espartero,J.L.,2008.New lipophilic tyrosyl parative antioxidant evaluation with hydroxytyrosyl esters.J.Agri.Food Chem.56,10960–10966.82 A.Trincone et al./Bioresource Technology115(2012)79–83Obied,H.K.,Allen,M.S.,Bedgood,D.R.,Prenzler,P.D.,Robards,K.,Stockmann,R., 2005.Bioactivity and analysis of biophenols recovered from olive mill waste.J.Agri.Food Chem.53,823–837.Tong,A.M.,Lu,W.Y.,Xua,J.H.,Lin,G.Q.,e of apple seed meal as a new source of b-glucosidase for enzymatic glucosylation of4-substituted benzyl alcohols and tyrosol in monophasic aqueous-dioxane medium.Bioorg.Med.Chem.Lett.14,2095–2097.van Rantwijk,F.,Woudenberg-van Oosterom,M.,Sheldon,R.A.,1999.Glycosidase-catalysed synthesis of alkyl glycosides.J.Mol.Catal.B6,511–532.Visioli, F.,Bellomo,G.,Montedoro,G.,Galli, C.,1995.Low density lipoprotein oxidation is inhibited in vitro by olive oil constituents.Atherosclerosis117,25–32.Visioli,F.,Poli,A.,Galli,C.,2002.Antioxidant and other biological activities of phenols from olives and olive oil.Med.Res.Rev.22(1),65–75.Vissers,M.N.,Zock,P.L.,Katan,M.B.,2004.Bioavailability and antioxidant effects of olive oil phenols in humans:a review.Eur.J.Clin.Nutr.58,955–965. Waterman,E.,Lockwood,B.,2007.Active components and clinical applications of olive oil.Thorne Res.Inc.Alt.Med.Rev.12(4),331–342.A.Trincone et al./Bioresource Technology115(2012)79–8383。