Enhanced ethanol sensing properties of Zn-doped SnO2 porous hollow microspheres
氧化亚铜的文献

Brush-Like Hierarchical ZnO Nanostructures:Synthesis,Photoluminescence and Gas Sensor PropertiesYuan Zhang,†,‡Jiaqiang Xu,*,†,§Qun Xiang,†Hui Li,†,‡Qingyi Pan,†and Pengcheng Xu §Department of Chemistry,College of Science,Shanghai Uni V ersity,Shanghai 200444,China,Department of Physics,College of Science,Shanghai Uni V ersity,Shanghai 200444,China,and State Key Laboratory of Transducer Technology,Shanghai Institute of Microsystem and Information Technology,Chinese Academy of Sciences,Shanghai 200050,ChinaRecei V ed:September 12,2008;Re V ised Manuscript Recei V ed:December 28,2008Brush-like hierarchical ZnO nanostructures assembled from initial 1D ZnO nanostructures were prepared from sequential nucleation and growth following a hydrothermal process.The morphology,structure,and optical property of hierarchical ZnO nanostructures were characterized by X-ray diffraction (XRD),field-emission scanning electron microscopy (FE-SEM),and photoluminescence (PL)studies.The FE-SEM images showed that the brush-like hierarchical ZnO nanostructures are composed of 6-fold nanorod-arrays grown on the side surface of core pared with ZnO nanowires,brush-like hierarchical ZnO nanostructures easily fabricated satisfactory ethanol sensors.The main advantages of these sensors are featured in excellent selectivity,fast response (less than 10s),high response (sensitivity),and low detection limit (with detectable ethanol concentration in ppm).1.IntroductionNanoscale materials have stimulated great interest in current materials science due to their importance in such basic scientific researches and potential technology applications as microelec-tronic devices,1chemical and biological sensors,2light-emitting displays,3catalysis,4and energy conversion and storage devices.5Previous studies indicated that the shape of nanoscale materials have a profound influence on their properties.6This has led to intensive investigation on quantum dots,7nanowires,8nano-tubes,9and self-assembled monolayers (SAMs).10In recent years,much attention has been paid to three-dimensional and hierarchical architectures that derive from the above-mentioned low dimensional nanostructures as building blocks,due to various novel applications.For example,the Lieber group has reported that core/shell coaxial silicon nanowires architectures can be employed as solar cells.1Wang reported that a novel hierarchical nanostructure based on Kevlar fibers coated with ZnO nanowires could serve as nanogenerators.11Though tremendous progress has been made in this significant field,there are still great demands on the synthesis of alternative three-dimensional and hierarchical architectures with novel or po-tential applications.Hierarchical ZnO architectures have been extensively pro-duced with gas-phase approaches.12-14With these synthetic methods,nanowire arrays,15nanohelitics,16nanopropeller,17and tower-like nanocolumns 18have been successfully prepared.However,these protocols often require high temperature and induce impurities in the final products when catalysts and templates are introduced to the reaction system.In practice,this made it difficult to obtain organic/inorganic hybrid hierarchical architectures.In addition,although the solution-based synthetic strategies are simple and effective in the production of thebuilding blocks of hierarchical nanostructures such as nanopar-ticles,19nanowires,20and nanorods,21achievement of hierarchical architectures from such techniques remains a challenge.Herein,we developed a simple nucleation and growth strategy to synthesize brush-like hierarchical ZnO nanostructures.The two step seeded-growth approach allows stepwise control and optimization of experimental conditions and provides an op-portunity for rational design and synthesis of controlled architectures in nanostructures.22-24We also investigated the effect of morphology and structure of brush-like hierarchical ZnO nanostructures on its gas sensing responses.The results show that ZnO hierarchical nanostructures display better ethanol sensing property than that of ZnO nanowires.2.Experimental SectionAll reagents employed were analytically pure and used as received from Shanghai Chemical Industrial Co.Ltd.(Shanghai,China)unless otherwise mentioned.2.1.Synthesis.The strategies to fabricate the brush-like hierarchical ZnO nanostructures are summarized as follows.First,the ZnO nanowires with a uniform shape were synthesized as described elsewhere,25which was used as the initial material to grow hierarchical ZnO nanostructures.Subsequently,a saturated solution of Zn(OH)42-was prepared by dissolving excess ZnO in 10mL of NaOH solution (5mol/L)for growing hierarchical ZnO nanostructures.Third,the ZnO nanowires (0.05g)were uniformly suspended in deionized water (37mL)in an ultrasonic bath.The suspension was mixed with the Zn(OH)42-saturated solution (3mL).After the mixture was transferred into a Teflon-lined autoclave (50mL),it was kept at 100°C for 10h.Finally,upon the hydrothermal treatment a white precipitate was formed,which was washed thoroughly with ethanol and distilled water in sequence,and dried at 60°C under vacuum for 6h.2.2.Characterization.The initial ZnO seeds were character-ized by transmission electron microscopy (TEM,JEM-200CX,using an accelerating voltage of 160kV).The morphology of*Corresponding author.Tel:+862166134728.Fax:+862166134725.E-mail:xujiaqiang@.†Department of Chemistry,Shanghai University.‡Department of Physics,Shanghai University.§Chinese Academy of Sciences.J.Phys.Chem.C 2009,113,3430–3435343010.1021/jp8092258CCC:$40.75 2009American Chemical SocietyPublished on Web 02/06/2009hierarchical ZnO nanostructures was observed by field emission scanning electron microscopy (FE-SEM,JSM-6700F).The crystal phase of as-synthesized products was identified by powder X-ray diffraction (XRD)analysis using a D/max 2550V diffractometer with Cu K R radiation (λ) 1.54056Å)(Rigaku,Tokyo,Japan),and the XRD data were collected at a scanning rate of 0.02deg s -1for 2θin a range from 10°to 70°.2.3.Photoluminescence (PL)Measurement.Room tem-perature PL measurements were performed on a Hitachi RF-5301PC spectrofluophotometer using the 350nm Xe laser line as the excitation source.2.4.Gas Sensor Fabrication and Response Test.The ZnO powder was mixed with Terpineol and ground in an agate mortar to form a paste.The resulting paste was coated on an alumina tube-like substrate on which a pair of Au electrodes had been printed previously,followed by drying at 100°C for about 2h and subsequent annealing at 600°C for about 2h.Finally,a small Ni -Cr alloy coil was inserted into the tube as a heater,which provided the working temperature of the gas sensor.The schematic drawing of the as-fabricated gas sensor is shown in Figure 1.In order to improve the long-term stability,the sensors were kept at the working temperature for several days.A stationary state gas distribution method was used for testing gas response (Air humidity:47%).In the measurement of electric circuit for gas sensors (Figure 2),a load resistor (Load resistor value:470k Ω)was connected in series with a gas sensor.The circuit voltage was set at 10V,and the output voltage (V out )was the terminal voltage of the load resistor.The working temperature of a sensor was adjusted through varying the heating voltage.The resistance of a sensor in air or test gas was measured by monitoring V out .The test was operated in a measuring system of HW-30A (Hanwei Electronics Co.Ltd.,P.R.China).Detect-ing gases,such as C 2H 5OH,were injected into a test chamber and mixed with air.The gas response of the sensor in this paper was defined as S )R a /R g (reducing gases)or S )R g /R a (oxidizing gases),where R a and R g were the resistance in air and test gas,respectively.The response or recovery time was expressed as the time taken for the sensor output to reach 90%of its saturation after applying or switching off the gas in a step function.3.Results and Discussion3.1.Structure and Morphology.In the XRD pattern of the as-synthesized products (Figure 3),all of the peaks were well indexed to hexagonal wurtzite ZnO (JCPDS No.36-1451,a )0.3249nm,c )0.5205nm)with high crystallization.No characteristic peaks were observed for impurities.The FE-SEM images of hierarchical ZnO nanostructures (Figure 4)were observed at low and medium magnifications,respectively.From the low magnification image (Figure 4a),the secondary nanorods self-organized into very regular arrays,which mimic brush-like hierarchical nanostructures.These nanorod arrays that grew onto one common central nucleus could also be revealed from the protrudent brush-like structures (Figure 4a).The medium magnification (Figure 4b)clearly indicated that these secondary nanorod arrays were brush-like 6-fold symmetry.MorecarefulFigure 1.Sketch of the gassensor.Figure 2.Measuring electric circuit of the gassensor.Figure 3.XRD pattern of the brush-like hierarchical ZnOnanostructures.Figure 4.FE-SEM images of the brush-like hierarchical ZnO nanostructures:(a)at low magnification and (b)at medium magnification.Brush-Like Hierarchical ZnO Nanostructures J.Phys.Chem.C,Vol.113,No.9,20093431observation of the morphology of hierarchical nanostructures indicated that the nanorod arrays grew on the side surface of core nanowire.The reason for nanorod arrays to grow into 6-fold symmetry may arise from the hexagonal symmetry of the major core.26The central stems provide its six prismatic crystal planes/facets as growth platforms for branching of multipod units.The synthesis of 6-fold ZnO nanostructures have been reported;17,26-28however,the solution-based approach to this structure is rarely disclosed.Under hydrothermal conditions,heteronucleation can take place,and the interfacial energy between crystal nuclei and substrates is usually smaller than that between crystal nuclei and solutions.22Therefore,the secondary rod-like branches can grow on the wire-like ZnO central core.3.2.Impact of the Reaction Conditions on the Growth of ZnO Hierarchical Nanostructures.Our studies suggest that the morphology of ZnO seeds and the concentration of OH -ion in the reaction system are the key factors for the formation of ZnO hierarchical nanostructures.First,ZnO nanostructures with different morphology were used as seeds for the nucleation and growth process.In the synthesis of brush-like ZnO hierarchical nanostructures,high aspect ratio (height/width)ZnO nanowires were used as seeds.These ZnO nanowires have typical diameters of 80-100nm and lengths up to 10µm (Figure 5).In order to study the effect of the seeds morphology on the growth of brush-like ZnO hierarchical nanostructures,a lower aspect ratio of ZnO nanorods (200nm diameter,aspect ratio <5,Figure 6a)was used as substitute seeds in this seeding growth method.When nanorods were used as seeds for further crystal growth,shorter and thicker rod-like ZnO nanostructures were obtained (Figure 6b).Second,the brush-like ZnO hierarchical nanostructures could only be obtained under an optimized concentration (5M)of OH -ion.The morphologies of the as-obtained products varied remarkably with changes in the OH -ion concentration.When the OH -ion concentration dropped to 3M,only a few disorder secondary nanorods grew on each common central nucleus (Figure 7a).As the OH -ion concentration increased to 15M,the secondary rod-like branches structures and the brush-like hierarchical nanostructures became shorter than those at lower concentrations because of the dissolve effect of NaOH (Figure 7b).When the OH -ion concentration increased to 20M,the amount of sub-branch nanorods also increased,and chrysan-themum-like microstructures could be obtained (Figure 7c).It is worth mentioning that these secondary rod-like crystals have a relatively planar bottom at high OH -ion concentration.We noted that the concentration of NaOH (i.e.,the concentra-tion of OH -)was crucial to the formation of Zn(OH)42-precursor.The growth of ZnO did not proceed at low concentra-tion of OH -because no Zn(OH)2was formed in the solution.Accordingly,only a few disordered sub-branch nanorods grew on each common central nucleus.With an increase of OH -concentration,the nucleation of ZnO was accelerated leading to more nanorods growing on the central nucleus.When the concentration of OH -was further increased,the growth of sub-branch nanorods was impeded,29and the average aspect ratio of ZnO nanorods was decreased.However,when excess NaOH was employed,it became not only a source of hydroxyl ions to form ZnO,but a capping agent.30ZnO crystal is a polar solid with a positive polar plane (0001)rich in Zn and a negativepolar plane (0001j )rich in O.31At a high OH -concentration,OH -ions are preferably adsorbed on the (0001)plane of ZnO,29,32and the growth of the ZnO nanocrystallite along the c axis is partially suppressed.It suggests that the OH -ion could act as a surface termination reagent,thus impeding the growth of crystal face (0001).29Accordingly,the secondary rod-like crystals have a flat face.Previous studies indicated that surfactants could control the morphology in the solution phase process.33-36We synthesized the ZnO hierarchical nanostructures V ia a surfactant-free route.But,do surfactants have a positive effect on the morphology of the products?Taking a typical surfactant CTAB (cetyltrimethy-lammonium bromide)as example,when CTAB was introduced into the reaction system,the morphology of the as-obtained products was ZnO microsphere consisting of nanorods(FigureFigure 5.TEM image of the ZnO nanowireseeds.Figure 6.TEM images of the ZnO samples:(a)the initial ZnO nanorods seeds and (b)the secondary crystals from the initial ZnO nanorods.3432J.Phys.Chem.C,Vol.113,No.9,2009Zhang et al.7d).The ability of CTAB to form diversified shapes of microparticles has been exploited extensively.37-41Due to the coulomb force action between [Zn(OH)42-]and CTAB,they interact to form complexes,which are adsorbed on the circum-ference of the ZnO nuclei.42Upon the formation of globules,the zinc complex dissociated to form rod-like nanostructures with the assistance of CTAB,43,44leading to the nanorod-assembled microsphere.In addition,the hydrothermal temperature (100-120°C)and hydrothermal time (10-15h)made no obvious difference in the morphology of ZnO hierarchical nanostructures.3.3.Optical Properties Measurement.The photolumines-cence spectrum of the secondary brush-shaped nanostructures was measured using Xe laser (350nm)as excitation source.We also measured the PL spectrum of the initial nanowires for comparison (Figure 8).Strong emission at ∼389nm was observed for both structures.In addition,we observed that the green emission intensity at ∼558nm for the brush-shaped nanostructures is stronger than that of the initial nanowires.The green emission peak is commonly referred to singly ionized oxygen vacancy in ZnO resulting from the radiative recombina-tion of a photogenerated hole with an electron occupying theoxygen vacancy.15,45The stronger green light emission intensity suggests that there is a greater fraction of oxygen vacancies in the brush-shaped nanostructures.Defects at metal oxide surfaces are believed to significantly influence a variety of surface properties,including chemical adsorption reactivity,46,47such as heterogeneous catalysis,cor-rosion inhibition and gas sensing.The influence of the intrinsic defects on the ZnO surface chemistry and the effects of chemisorption have been addressed by theoretical calculation and experimental data.47-50Moreover,the mechanism of the oxygen vacancies induced gas sensing enhancement properties of ZnO has been explained.51A large quantity of oxygen vacancy results in high adsorptions of oxygen,and in turn enhances the chance of interaction with testing gases.3.4.Gas Sensing Properties.The gas sensing properties of low dimensional ZnO nanostructures (nanoparticles,nanowires,nanorods)have been widely investigated by many research groups;52-55however,the influence of hierarchical ZnO mor-phology on their gas sensing performance has scarcely been investigated.In this paper,we also studied the ethanol gas-sensing properties of ZnO hierarchical nanostructures,and discussed the effect of morphology on the gas sensing responses.Eight typical reducing gases (CH 4,H 2,NH 3,i -C 4H 10,HCHO,CO,C 6H 6,and C 2H 5OH)and two typical oxidizing gases (NO 2and Cl 2)were selected as target gases to investigate the gas response at an optimal operating temperature of 265°C,where the concentration of all the tested gases was 50ppm.The gas sensor based on the ZnO hierarchical nanostructures (Figure 9)showed good selectivity to ethanol with little interference against other gases.ZnO is a very common ethanol sensing material.56-58The ethanol sensing mechanism of the ZnO sensors has also been reported in our previous studies.59However,only few studies indicate that ZnO has higher gas response to ethanol than other commonly used gases.58Most metal oxide semiconductor gas sensors are based on the conductance change arising fromtheFigure 7.The FE-SEM images of the secondary ZnO nanostructures obtained under different conditions a)3M NaOH,b)15M NaOH,c)20M NaOH,d)1gCTAB.Figure 8.Photoluminescence spectra of different ZnO samples (a)brush-like hierarchical nanostructures and (b)nanowires.Brush-Like Hierarchical ZnO Nanostructures J.Phys.Chem.C,Vol.113,No.9,20093433reactions between the oxygen ions and the detecting gas molecules adsorbed on the active surface.60,61We found that the electron donating effect of the testing gas molecule is an important factor in the explanation of this phenomenon (In the several forms of oxygen adsorbates,the O -is more active form of adsorbed oxygen.).For example,the typical testing gas sensing reactions are expressed as follows:CO +O -f CO 2+e -(1)H 2+O -f H 2O +e -(2)HCHO +2O -f H 2O +CO 2+2e -(3)Catalytic oxidation of ethanol gas is known through two different routes depending on the acid or base properties of catalyst surface,i.e.,a dehydrogenation route through CH 3CHO intermediate (on the basic surface)and a dehydration route through a C 2H 4intermediate (on the acidic surface).61,62Since ZnO is a basic oxide,dehydrogenation is favored,providing CH 3CHO as the major which undergoes subsequent oxidation to form CO 2and H 2O.C 2H 5OH f CH 3CHO +H 2(4)CH 3CHO(ad)+5O -(ad)f 2CO 2+2H 2O +5e -(5)According to these equations,the electron donating effect of ethanol is stronger than that of the other gases.Therefore,the gas response of ethanol is higher than that of the other gases at the equivalent concentration.However,owing to the complicated reactivity of some gas molecules on the ZnO surface,the electron donating effect is not the only influential factor on gas response.Other factors (such as reaction of testing gases and sensing material,humidity,temperature,and some additional external fluctuating factor)may exist and compete against each other.Interestingly,the response of brush-like hierarchical nano-structures (Figure 9)is greater than that of initial ZnO nanowires.This observation is general to all the gases examined herein.The gas sensing response enhancement can be attributed to more active centers that are obtained from the enhanced oxygen vacancy defects on the brush-shaped nanostructures.Besides,the formation of initial nanorod/secondary nanorods junctions may be an additional reason for the response enhancement.These junctions are considered as the active sites that canenhance the response of the gas sensors.63Moreover,the nanorod arrays could increase the numbers of the gas channels leading to more effective surface areas (defined as the areas which can contact with the gas).The enhancement in gas response of the brush-like hierarchi-cal nanostructures with the increase of ethanol concentration was observed (Figure 10)in a range of 5-50ppm.The gas response of brush-like hierarchical nanostructures was so high that even if the concentration of ethanol decreased to 5ppm,the gas response increased by 3.0times.In addition,the brush-like hierarchical ZnO nanostructures had very high responses to ethanol,showing a promise as ethanol-sensing materials.The dynamic response of brush-like hierarchical ZnO nanostructures to 10,30,and 50ppm ethanol (Figure 11)displayed impressive response and recovery properties for the brush-like hierarchical ZnO nanostructure-based sensors.All the response time and the recovery time of the sensors at the ethanol concentration of 10,30,and 50ppm were less than 10s.The SEM image (Figure 12)of brush-like hierarchical ZnO nanostructure after sintering at 600°C for 2h suggested that the brush-like hierarchical nanostructures were kept well.The heat treatment did not affect the morphology and sizes of these hierarchical nanostructures,which demonstrated a high stability of the brush-like hierarchical ZnO nanostructures.The structural stability may be in favor of the long-term stability of sensors suitable for practical applications.4.ConclusionIn this paper,we developed a simple hydrothermal route to synthesize brush-like hierarchical ZnO nanostructures under mild conditions.Our results showed that a soft chemical route is promising for rational and structural design of the nanoscale materials.The photoluminescence and gas sensing properties of brush-like hierarchical ZnO nanostructures were studied.The photoluminescence spectrum suggested higher oxygen vacancy defects on the brush-shaped nanostructures thannanowires,Figure 9.Responses of the brush-like hierarchical ZnO nanostructures and the ZnO nanowires to various gases (The concentration of all gases was 50ppm).Figure 10.Relationship between gas response of the brush-like hierarchical ZnO nanostructures and ethanolconcentration.Figure 11.Response of sensor based on brush-like hierarchical ZnO nanostructures to 10,30,and 50ppm ethanol.3434J.Phys.Chem.C,Vol.113,No.9,2009Zhang et al.which combined with special ZnO morphology were able to generate more active centers so as to enhance the gas response.The gas sensing measurements showed that the brush-like hierarchical ZnO nanostructures could serve excellent ethanol sensors.Acknowledgment.We appreciate the financial support of Shanghai NSF (No.07ER14039)and Leading Academic Discipline Project of Shanghai Municipal Education Commis-sion (No.J50102).We also thank the Analysis and Research Center at Shanghai University for sample characterization.References and Notes(1)Lauhon,L.J.;Gudiksen,M.S.;Wang,C.L.;Lieber,C.M.Nature 2002,420,57.(2)Modi,A.;Koratkar,N.;Lass,E.;Wei,B.Q.;Ajayan,P.M.Nature 2003,424,171.(3)Banerjee,D.;Jo,S.H.;Ren,Z.F.Ad V .Mater.2004,16,2028.(4)Bansal,V.;Jani,H.;Du Plessis,J.;Coloe,P.J.;Bhargava,S.K.Ad V .Mater.2008,20,717.(5)Arico, A.S.;Bruce,P.;Scrosati, B.;Tarascon,J.M.;Van Schalkwijk,W.Nat.Mater.2005,4,366.(6)Jun,Y.W.;Choi,J.S.;Cheon,J.Angew.Chem.,Int.Ed.2006,45,3414.(7)Nesbitt,D.J.Nature 2007,450,1172.(8)Greene,L.E.;Law,M.;Tan,D.H.;Montano,M.;Goldberger,J.;Somorjai,G.;Yang,P.D.Nano Lett.2005,5,1231.(9)Li,S.;Liu,N.Y.;Chan-Park,M.B.;Yan,Y.A.;Zhang,Q.Nanotechnology 2007,18,455302.(10)Zuo,G.M.;Li,X.X.;Zhang,Z.X.;Yang,T.T.;Wang,Y.L.;Cheng,Z.X.;Feng,S.L.Nanotechnology 2007,18,255501.(11)Qin,Y.;Wang,X.D.;Wang,Z.L.Nature 2008,451,809.(12)Sun,S.H.;Meng,G.W.;Zhang,G.X.;Masse,J.P.;Zhang,L.Chem.Eur.J.2007,13,9087.(13)Kuang,Q.;Jiang,Z.Y.;Xie,Z.X.;Lin,S.C.;Lin,Z.W.;Xie,S.Y.;Huang,R.B.;Zheng,L.S.J.Am.Chem.Soc.2005,127,11777.(14)Yang,Y.H.;Wang,B.;Yang,G.W.Cryst.Growth Des.2007,7,1242.(15)Yang,P.D.;Yan,H.Q.;Mao,S.;Russo,R.;Johnson,J.;Saykally,R.;Morris,N.;Pham,J.;He,R.R.;Choi,H.J.Ad V .Funct.Mater.2002,12,323.(16)Moore,D.;Ding,Y.;Wang,Z.L.Angew.Chem.,Int.Ed.2006,45,5150.(17)Gao,P.X.;Wang,Z.L.Appl.Phys.Lett.2004,84,2883.(18)Tian,Z.R.R.;Voigt,J.A.;Liu,J.;McKenzie,B.;McDermott,M.J.;Rodriguez,M.A.;Konishi,H.;Xu,H.F.Nat.Mater.2003,2,821.(19)Xu,R.;Xie,T.;Zhao,Y.G.;Li,Y.D.Nanotechnology 2007,18,055602.(20)Wang,J.M.;Gao,L.J.Mater.Chem.2003,13,2551.(21)Han,X.H.;Wang,G.Z.;Zhou,L.;Hou,mun.2006,212.(22)Gao,X.P.;Zheng,Z.F.;Zhu,H.Y.;Pan,G.L.;Bao,J.L.;Wu,F.;Song,mun.2004,1428.(23)Sounart,T.L.;Liu,J.;Voigt,J.A.;Hsu,J.W.P.;Spoerke,E.D.;Tian,Z.;Jiang,Y.B.Ad V .Funct.Mater.2006,16,335.(24)Zhang,T.R.;Dong,W.J.;Keeter-Brewer,M.;Konar,S.;Njabon,R.N.;Tian,Z.R.J.Am.Chem.Soc.2006,128,10960.(25)Xiang,Q.;Pan,Q.Y.;Xu,J.Q.;Shi,L.Y.;Xu,P.C.;Liu,R.L.Chinese J.Inorg.Chem.2007,23,369.(26)Lao,J.Y.;Wen,J.G.;Ren,Z.F.Nano Lett.2002,2,1287.(27)Wen,J.G.;Lao,J.Y.;Wang,D.Z.;Kyaw,T.M.;Foo,Y.L.;Ren,Z.F.Chem.Phys.Lett.2003,372,717.(28)Liu,B.;Zeng,H.C.Chem.Mater.2007,19,5824.(29)Cao,B.Q.;Cai,W.P.J.Phys.Chem.C 2008,112,680.(30)Viswanatha,R.;Amenitsch,H.;Sarma,D.D.J.Am.Chem.Soc.2007,129,4470.(31)Li,W.J.;Shi,E.W.;Zhong,W.Z.;Yin,Z.W.J.Cryst.Growth 1999,203,186.(32)Hegemann,I.;Schwaebe,A.;Fink,put.Chem.2008,29,2302.(33)Kuo,C.L.;Kuo,T.J.;Huang,M.H.J.Phys.Chem.B 2005,109,20115.(34)Li,Z.Q.;Xiong,Y.J.;Xie,Y.Inorg.Chem.2003,42,8105.(35)Liang,J.B.;Liu,J.W.;Xie,Q.;Bai,S.;Yu,W.C.;Qian,Y.T.J.Phys.Chem.B 2005,109,9463.(36)Sun,G.B.;Cao,M.H.;Wang,Y.H.;Hu,C.W.;Liu,Y.C.;Ren,L.;Pu,Z.F.Mater.Lett.2006,60,2777.(37)Cao,M.H.;He,X.Y.;Chen,J.;Hu,C.W.Cryst.Growth Des.2007,7,170.(38)Chen,S.H.;Fan,Z.Y.;Carroll,D.L.J.Phys.Chem.B 2002,106,10777.(39)Du,J.M.;Liu,Z.M.;Huang,Y.;Gao,Y.N.;Han,B.X.;Li,W.J.;Yang,G.Y.J.Cryst.Growth 2005,280,126.(40)Kuang,D.B.;Xu,A.W.;Fang,Y.P.;Liu,H.Q.;Frommen,C.;Fenske,D.Ad V .Mater.2003,15,1747.(41)Luo,Y.S.;Li,S.Q.;Ren,Q.F.;Liu,J.P.;Xing,L.L.;Wang,Y.;Yu,Y.;Jia,Z.J.;Li,J.L.Cryst.Growth Des.2007,7,87.(42)Zhang,H.;Yang,D.;Ji,Y.J.;Ma,X.Y.;Xu,J.;Que,D.L.J.Phys.Chem.B 2004,108,3955.(43)Cao,M.H.;Hu,C.W.;Wang,Y.H.;Guo,Y.H.;Guo,C.X.;Wang,mun.2003,1884.(44)Ni,Y.H.;Wei,X.W.;Ma,X.;Hong,J.M.J.Cryst.Growth 2005,283,48.(45)Huang,M.H.;Wu,Y.Y.;Feick,H.;Tran,N.;Weber,E.;Yang,P.D.Ad V .Mater.2001,13,113.(46)Gutierrez-Sosa,G.A.;Baraldi,A.;Larciprete,R.;Lizzit,S.J.Am.Chem.Soc.2002,124,7117.(47)Lindsay,R.;Michelangeli,E.;Daniels,B.G.;Ashworth,T.V.;Limb,A.J.;Thornton Geistlinger,H.J.Appl.Phys.1996,80,1370.(48)Go ¨pel,W.J.Vac.Sci.Technol.1978,15,1298.(49)An,W.;Wu,X.Q.;Zeng,X.C.J.Phys.Chem.C 2008,112,5747.(50)Polarz,S.;Roy, A.;Lehmann,M.;Driess,M.;Kruis, F. E.;Hoffmann,A.;Zimmer,P.Ad V .Funct.Mater.2007,17,1385.(51)Liao,L.;Lu,H.B.;Li,J.C.;He,H.;Wang,D.F.;Fu,D.J.;Liu,C.;Zhang,W.F.J.Phys.Chem.C 2007,111,1900.(52)Seiyama,T.;Kato,A.;Fujiishi,K.;Nagatani,M.Anal.Chem.1962,34,1502.(53)Xu,J.Q.;Pan,Q.Y.;Shun,Y.A.;Tian,Z.Z.Sens.Actuators B 2000,66,277.(54)Liao,L.;Lu,H.B.;Li,J.C.;He,H.;Wang,D.F.;Fu,D.J.;Liu,C.;Zhang,W.F.J.Phys.Chem.C 2007,111,1900.(55)Kwak,G.;Yong,K.J.J.Phys.Chem.C 2008,112,3036.(56)Liu,Y.;Dong,J.;Hesketh,P.J.;Liu,M.L.J.Mater.Chem.2005,15,2316.(57)Wan,Q.;Li,Q.H.;Chen,Y.J.;Wang,T.H.;He,X.L.;Li,J.P.;Lin,C.L.Appl.Phys.Lett.2004,84,3654.(58)Cheng,X.L.;Zhao,H.;Huo,L.H.;Gao,S.;Zhao,J.G.Sens.Actuators B 2004,102,248.(59)Xu,J.Q.;Han,J.J.;Zhang,Y.;Sun,Y.A.;Xie,B.Sens.Actuators B 2008,132,334.(60)Morrison,S.R.Sens.Actuators B 1987,12,425.(61)Yamazoe,N.;Sakai,G.;Shimanoe,K.Catal.Sur V .Asia 2003,7,63.(62)Jinkawa,T.;Sakai,G.;Tamaki,J.;Miura,N.;Yamazoe,N.J.Mol.Catal.A 2000,155,193.(63)Zhang,D.H.;Liu,Z.Q.;Li,C.;Tang,T.;Liu,X.L.;Han,S.;Lei,B.;Zhou,C.W.Nano Lett.2004,4,1919.JP8092258Figure 12.SEM image of the brush-like hierarchical ZnO nanostruc-ture after sintering at 600°C for about 2h.Brush-Like Hierarchical ZnO Nanostructures J.Phys.Chem.C,Vol.113,No.9,20093435。
材料化工专业英语生词本
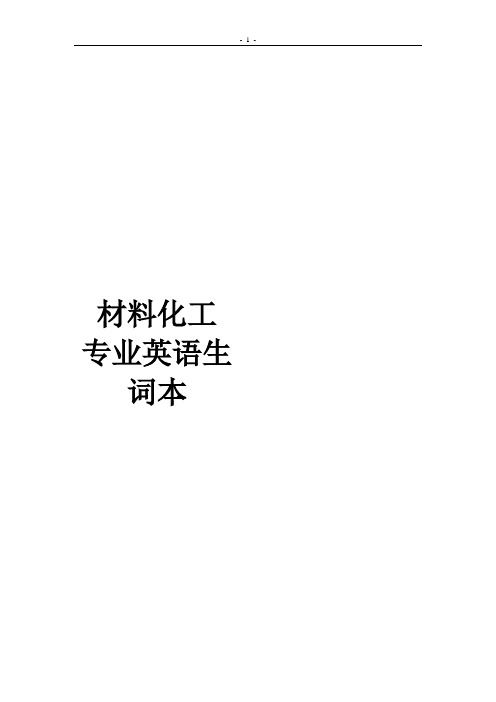
材料化工专业英语生词本Synthesis 合成Properties 性质Anatase 锐钛矿rutile 金红石brookite板钛矿Crystalline 结晶的nanometer 纳米nanorods/wires纳米棒/线nanocrystals 纳米晶体nanocarriers 纳米载体nanoparticles (NPs)纳米颗粒nanocomposite纳米复合Hierarchical Nanostructures 分层纳米材料titanium dioxide TiO2 polymorphs of titania 多晶型 TiO2 amorphous 非晶的Three-dimensional 3Dfacile and controlled 容易控制hydrothermal 热液的annealing 退火investigate 调查,研究radially 放射状地petal 花瓣thin 薄的thick 厚的morphology 形态The surface area 表面积adsorption-desorption 吸附-解析(ads)orption isotherms 吸附等温线the Brunauer-Emmett-Teller BET 比表面积测试法specific surface areas 比表面积sensitivity 灵敏、灵敏性ethanol 乙醇、酒精ethylene glycol 乙二醇EG化学式C2H6O2分子式:HOC2H4OHsensor 传感器、感应器solar cells太阳能电池biosensors 生物传感器catalyst 催化剂Catalysis 催化photo-catalytic 光催化的inorganic 无机的objective 目标optimize 使完善、使优化optical 光学的magnetic 磁的application 应用bandgap 带隙transition metal oxides 过渡金属氧化物paint 油漆、颜料gas sensor 气敏元件、气敏传感器Li-ion battery 锂离子电池Electrochromic 电致变色的Photochromism 光致变色macro/mesoporous materials 宏/介孔材料CVD(Chemical Vapor Deposition, 化学气相沉积)Anodic 阳极的hydrothermal method 水热法Template 样板、模板oriented attachment 定向附着primary nanoparticle 初级纳米粒子anisotropic非等方性的、各向异性的capping agents 盖髓剂kirkendall effect柯肯达尔效应tetragonal structure 四方结构photovoltaic cells 光伏电池smart surface coatings 智能表面涂层single-phase 单相precursor 先驱、前导Herein 在此处、鉴于、如此 Nanoflakes 纳米片metal-enhanced fluorescence 金属增强荧光fluorophores 荧光团The Royal Society of Chemistry 英国皇家化学学会ESI (Electronic Supplementary Material) 电子补充材料 Innovative 创新的 Polymer 聚合物 Chemical 化学品 Silica 硅 FITC (fluorescein isothiocyanate )荧光异硫氰酸酯EiTC ( Eosin isothiocyanate ) 异硫氰酸曙红Fluorescence spectra 荧光光谱 control sample 对照样品 Dissolve 溶解Characterization 表征 analytical grade 分析纯 ethanol 乙醇ethylene glycol 乙二醇 ammonia aqueous solution (28 wt %)氨水溶液(100公斤里含28公斤) acetone 丙酮分子式:C3H6O 简式:CH3COCH3EtoH 乙醇 ( PS :Et 代表乙基CH3CH2- Me 代表甲基CH3-)TEOS (tetraethyl orthosilicate ) 原硅酸四乙酯the TEOS concentration TEOS 浓度 CTAB (hexadecyltrimethylammonium bromide ) 十六甲基溴化铵The CTAB surfactant CATB 表面活性剂Sinopharm Chemical Reagent Co. 国药集团化学试剂有限公司Polyvinylpyrrolidone (PVP, Mw = 55000) 聚乙烯吡咯烷酮(PVP ,MW = 55000=兆瓦,百万瓦特(megawatt))Rhodamine B (Rh B) 玫瑰精,若丹明B poly(allylamine hydrochloride) (PAH, Mw = 56000) 聚(烯丙胺盐酸盐) Deionized water 去离子水PAH ( polycyclic aromatic hydrocarbon )多环芳族烃 Via 经由、通过the three-neck flask 三颈烧瓶 oil bath 油浴precipitate 沉淀centrifugation 离心分离 rpm 每分钟转数 core-shell 核-壳a surfactant-templating sol-gel approach 表面活性剂模板溶胶 - 凝胶法homo-dispersed solution 均聚物分散夜agitate 搅拌ultrasonically and mechanically 超声波地、机械地solvent extraction method 溶剂萃取法reflux 回流an impregnation method 浸渍方法 vial 小瓶 dilute 稀释composite 合成物、复合物TEM (Transmission electron microscopy )透射电子显微镜copper grids 铜网carbon films 碳膜SEM(Scanning electron microscopy)扫描电子显微镜Spray 喷FESEM(Field-emission scanning el ectron microscopy)场发射扫描电子显微镜LCSM(Laser confocal scanning microscopy )激光共聚焦扫描显微镜X-ray diffraction (XRD) X 射线衍射X-ray diffractometer X射线衍射仪Nitrogen 氮Micromeritcs n. 微晶(粒)学,粉末工艺学;粉体学degas除去瓦斯vacuum 真空BET(The Brunauer-Emmett-Teller) pore volume 孔体积spectrofluorometer 荧光分光剂spectrophotometer分光光度计bandpass 带通PMT voltage (Photomultiplier Tube)光电倍增管电压Confocal luminescence images共聚焦荧光图像Silver 银silica spacer 硅垫片fabricate制造; 伪造; 组装; 杜撰the metal-enhancedMEF(the metal-enhanced fluorescence )金属增强荧光Fluorescence quenching 荧光猝灭FRET (Fo¨rs ter resonance energy transfer )福斯特共振能量转移Optimization 最佳化; 最优化excited-state 激发态plasmon 等离子基元quantum yields 量子产率quantum dots 量子点resonance n.共振,共鸣, 反响, 回声donor–acceptor pairs 给体- 受体对proximity 接近efficiency 效率the transfer distances 传输距离deposite 被沉淀,存放plastic planar substrate塑料平面基板photoluminescence (PL)光致发光luminescent 发光的single nanoparticle sensing单一纳米粒子传感dielectric电介质; 绝缘体adj.非传导性的RE complexes稀土复合Polyelectrolytes聚合高分子电解质Electrolyte电解质Multilayer 多层Concentric 同中心的functionalized organic molecules 官能有机分子conjugation 结合,配合tedious and fussy繁琐和挑剔obstacle n.障碍, 阻碍, 妨害物controlled release,控释detection and probe applications 检测和探头应用general一般的; 综合的; 普通的universal普遍的, 通用的, 全体的Inspired 启发Possess 拥有Pore 孔drugs and macro-molecules 药物和大分子herein在此处, 鉴于, 如此Ag@SiO2@mSiO2(Ag-core@silica-spacer@mesoporo us silica )The preparation procedure编制程序Water-soluble可溶于水的; 水溶性的,微溶于水A high-temperature solvothermal method一种高温溶剂热法Solvent 溶剂Esolution 分辨率twinned structures 联动结构,孪生结构concentration 浓度tune 调节is ascribed to 归因于dilute稀释spherical morphology 球形形态type-IV curves IV型曲线polyelectrolytesodium chloride食盐; 氯化钠plasmonic absorption电浆吸收an intuitive way 以直观的方式unambiguous 不含糊的, 明白的demonstrate 证明antibody 抗体NSF(National Sanitation Foundation)美国国家卫生基金会PRC(The People's Republic of China)中华人民共和国Shanghai Municipality上海市Shanghai Leading Academic Discipline Project上海重点学科建设项目Tri-functional hierarchical三官能分层DSSCs(dye-sensitized solar cells)染料敏化太阳能电池DOI(Digital Object Unique Identifier)是一种数字对象标识体系acid thermal method 酸热法titanium n-butoxid正丁醇钛acetic acid乙酸、醋酸kinetic 动能light-scattering 光散射photoelectrodes 光电极opto-electronic 光电的calcine煅烧short-circuit photocurrent density短路光电流密度open-circuit voltage开路电压compared to 相比,把什么比作什么electron 电子recombination rates 重组率oxide 氧化物inorganic 无机的sub-microspheres 亚微球beads珠子To date 迄今a ruthenium complex light-harvester钌络合物的光收割机volatile 挥发性的photoanode光阳极superior 好的,卓越的photons 光子photovoltaic performance光伏性能In addition to 除。
英文 Ce掺杂In2O3纳米纤维的制备及其三乙胺气敏性能
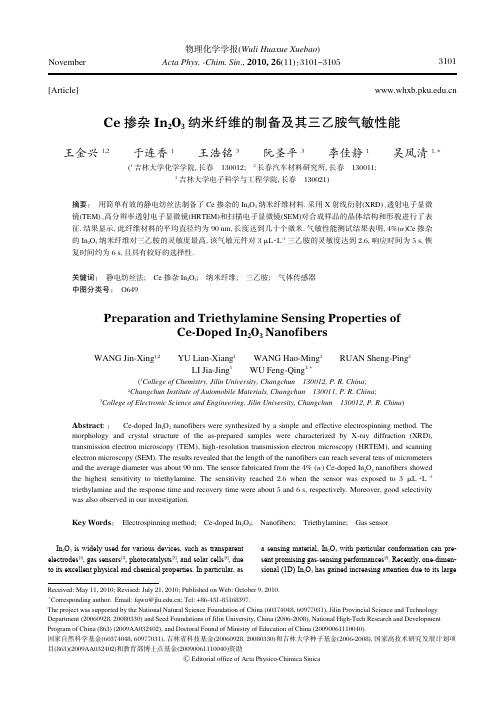
物理化学学报(Wuli Huaxue Xuebao )Acta Phys.鄄Chim.Sin .,2010,26(11):3101-3105Received:May 11,2010;Revised:July 21,2010;Published on Web:October 9,2010.∗Corresponding author.Email:fqwu@;Tel:+86⁃431⁃85168397.The project was supported by the National Natural Science Foundation of China (60374048,60977031),Jilin Provincial Science and Technology Department (20060928,20080330)and Seed Foundations of Jilin University,China (2006⁃2008),National High ⁃Tech Research and Development Program of China (863)(2009AA032402),and Doctoral Found of Ministry of Education of China (20090061110040).国家自然科学基金(60374048,60977031),吉林省科技基金(20060928,20080330)和吉林大学种子基金(2006⁃2008),国家高技术研究发展计划项目(863)(2009AA032402)和教育部博士点基金(20090061110040)资助ⒸEditorial office of Acta Physico ⁃Chimica Sinica[Article]November Ce 掺杂In 2O 3纳米纤维的制备及其三乙胺气敏性能王金兴1,2于连香1王浩铭3阮圣平3李佳静1吴凤清1,∗(1吉林大学化学学院,长春130012;2长春汽车材料研究所,长春130011;3吉林大学电子科学与工程学院,长春130021)摘要:用简单有效的静电纺丝法制备了Ce 掺杂的In 2O 3纳米纤维材料.采用X 射线衍射(XRD)、透射电子显微镜(TEM)、高分辨率透射电子显微镜(HRTEM)和扫描电子显微镜(SEM)对合成样品的晶体结构和形貌进行了表征.结果显示,此纤维材料的平均直径约为90nm,长度达到几十个微米.气敏性能测试结果表明,4%(w )Ce 掺杂的In 2O 3纳米纤维对三乙胺的灵敏度最高,该气敏元件对3μL ·L -1三乙胺的灵敏度达到2.6,响应时间为5s,恢复时间约为6s,且具有较好的选择性.关键词:静电纺丝法;Ce 掺杂In 2O 3;纳米纤维;三乙胺;气体传感器中图分类号:O649Preparation and Triethylamine Sensing Properties of Ce 鄄Doped In 2O 3NanofibersWANG Jin ⁃Xing 1,2YU Lian ⁃Xiang 1WANG Hao ⁃Ming 3RUAN Sheng ⁃Ping 3LI Jia ⁃Jing 1WU Feng ⁃Qing 1,∗(1College of Chemistry,Jilin University,Changchun 130012,P.R.China ;2Changchun Institute of Automobile Materials,Changchun 130011,P.R.China ;3College of Electronic Science and Engineering,Jilin University,Changchun 130012,P.R.China )Abstract ::Ce ⁃doped In 2O 3nanofibers were synthesized by a simple and effective electrospinning method.The morphology and crystal structure of the as ⁃prepared samples were characterized by X ⁃ray diffraction (XRD),transmission electron microscopy (TEM),high ⁃resolution transmission electron microscopy (HRTEM),and scanning electron microscopy (SEM).The results revealed that the length of the nanofibers can reach several tens of micrometers and the average diameter was about 90nm.The sensor fabricated from the 4%(w )Ce ⁃doped In 2O 3nanofibers showed the highest sensitivity to triethylamine.The sensitivity reached 2.6when the sensor was exposed to 3μL ·L -1triethylamine and the response time and recovery time were about 5and 6s,respectively.Moreover,good selectivity was also observed in our investigation.Key Words :Electrospinning method;Ce ⁃doped In 2O 3;Nanofibers;Triethylamine;Gas sensorIn 2O 3is widely used for various devices,such as transparent electrodes [1],gas sensors [2],photocatalysts [3],and solar cells [4],due to its excellent physical and chemical properties.In particular,asa sensing material,In 2O 3with particular conformation can pre-sent promising gas ⁃sensing performances [5].Recently,one ⁃dimen-sional (1D)In 2O 3has gained increasing attention due to its large3101Acta Phys.鄄Chim.Sin.,2010Vol.26surface and high length⁃diameter ratio[6].For gas sensor applica-tion,these characteristics may provide more absorption sites fortarget gas molecules and make the electron transfer more effec-tive,which results in the high sensitivity and fast reaction speedof these1D In2O3sensing materials[7-8].Triethylamine(TEA)is one of the most important organic ami-nes,which is produced in the process of metabolism by animalorgans or proteins.It is also one of the toxic gases in the biologyfield and food stuff industry[9].It was reported that during the pro-cess of fishes deterioration and after the death of seashells,somegaseous species,such as TEA,dimethylamine(DMA),trimethy-lamine(TMA),and ammonia(NH3),are given off[9].And the con-centrations and ratios of these gases are markedly dependent on the fish kind and freshness.Thus the sensors to these gases,es-pecially with high sensitivity and good selectivity,will be useful in our daily life.In this paper,we report the TEA sensing properties of Ce⁃doped pared with the pure In2O3nan-ofibers,Ce⁃doped In2O3nanofibers exhibit improved and excel-lent TEA sensing properties,which indicate the potential appli-cation of Ce⁃doped In2O3nanofiber in fabricating high perfor-mance TEA sensors.1Experimental1.1Material preparationAll the chemicals(analytical grade reagents)were purchased from Beijing Chemicals Co.Ltd.and used as received without further purification.To prepare the pure and Ce⁃doped In2O3 nanofibers,a certain amount of In(NO3)3·4.5H2O and Ce(NO3)3·6H2O powders were added to8.8g mixed solvent containing N, N⁃dimethylformamide(DMF)/EtOH with the mass ratio of1:1 and stirred for2h.Then0.8g polyvinylpyrrolidone(PVP)was added to the above solution with further stirring for6h.The ob-tained solution was then loaded into a plastic syringe and con-nected to a high⁃voltage power supply.20kV was provided be-tween the cathode(a flat aluminum foil)and the anode(syringe) at a distance of25cm.Finally,the In2O3nanofibers doped with different mass fractions of cerium(0%,2%,4%,and6%(w, same as below)were obtained by calcining the as⁃spun compos-ite fibers in air at600℃for4h.1.2CharacterizationThe samples were characterized by X⁃ray diffractometer (XRD)(Shimadzu XD⁃3AX),scanning electron microscopy (SEM SHIMADZU SSX⁃550,Kyoto,Japan),transmission elec-tron microscopy(TEM,HITACHI H⁃8100)with an acceleration voltage of200kV,and high⁃resolution transmission electron mi-croscopy(HRTEM JEM⁃3010).The gas sensing properties of the sensor were measured by a RQ2intelligent test meter(Qingdao,China).The structure and fabrication of the sensor are presented in our previous report in detail[10].The sensitivity(S)is defined as S=R a/R g,where R g and R a denote the sensor′s resistance in the presence and in the ab-sence of the target gases.1.3Sensor fabricationThe as⁃synthesized sample was mixed with deionized water in a mass ratio of100∶25to form a paste.Then the paste was coat-ed on a ceramic tube on which a pair of Au electrodes were pre-viously printed.Pt lead wires attaching to these electrodes were used as electrical contacts.After the ceramic tube was sintered at300℃for2h,a small Ni⁃Cr alloy wire(about33Ω)was placed through the tube as a heater,which provided the operat-ing temperature.The thickness of the Ce⁃doped In2O3fiber film is about250μm.The structure of the sensor is shown in Fig.1.2Results and discussion2.1Material characterizationFig.2(a)shows the SEM image of the as⁃elecrospun PVP/ In(NO3)3·4.5H2O composite nanofibers.The nanofibers are ran-domly distributed to form a fibrous nonwoven,and have smooth and uniform surface.After calcination at600℃for4h(Fig.2(b)), the surface of these nanofibers shrinks and becomes bend and rough,indicating the formation of In2O3nanofibers.The length of the nanofibers can reach several tens of micrometers,and the average diameter is about90nm.A typical TEM image of4% Ce⁃doped In2O3nanofiber in Fig.2(c)shows that the as⁃prepared nanofibers are composed of many interconnected grains of around5nm in size.High⁃resolution TEM(Fig.2(d))demon-strates the insight into the detailed atomic structure of Ce⁃doped In2O3nanofibers.The interplaner spacings(d)of0.29and0.41nm correspond to the[222]and[211]planes of cubic In2O3,respec-tively.Fig.3shows the XRD patterns of the pure and Ce⁃doped In2O3 nanofibers.For pure In2O3,2%and4%Ce⁃doped In2O3nan-ofibers,all the observed diffraction peaks can be indexed to cu-bic indium oxide(JCPDS file No.06⁃0416).For6%Ce⁃doped In2O3nanofibers,a peak corresponding to CeO2is observed at 28.5°.This suggests that some crystal CeO2may form on the surface of In2O3nanofibers.The similar phenomena have also been found in other paper[11].2.2Gas sensitive properties of the samplesThe sensitivities of the pure and Ce⁃doped In2O3nanofibers (n⁃type)to10μL·L-1TEA as a function of operating tempera-ture are shown in Fig.4.For each sample,the sensitivity is found to increase with the increasing operating temperature,which at-tains the maximum at a certain value,and then decreases witha Fig.1Schematic picture of sensor structure3102No.11WANG Jin ⁃Xing et al .:Preparation and Triethylamine Sensing Properties of Ce ⁃Doped In 2O 3Nanofibers further rise of the operating temperature.Among all the samples,4%Ce ⁃doped In 2O 3nanofibers exhibit the highest sensitivity of 13.2at 130℃,which is almost 4times larger than that of the pure In 2O 3nanofibers.The 4%Ce ⁃doped In 2O 3nanofibers also exhibit very high sensitivity and quick response/recovery speed to TEA as shown in Fig.5.As can be seen,we exposed the sen-sor to different concentrations of TEA continuously at 130℃.The sensitivities are about 2.6,5.5,13.2,28.7,39.4,56.8,61.9,and 72.2to 3,6,10,30,50,100,200,and 300μL ·L -1TEA,re-spectively.And the response time and recovery time are about 5and 6s,respectively.The sensitivities of the 4%Ce ⁃doped In 2O 3nanofibers to dif-ferent concentrations of TEA at 130℃are shown in Fig.6.In the low concentrations (3-30μL ·L -1),the sensitivityincreasesFig.2SEM images of electrospun PVP/In(NO 3)3·4.5H 2O composite nanofibers before (a)and after (b)calcination inair at 600℃,a typical TEM image of 4%(w )Ce ⁃doped In 2O 3nanofibers (c)and the high ⁃resolution TEM image of4%(w )Ce ⁃doped In 2O 3nanofibers(d)Fig.3XRD patterns of the pure and Ce ⁃doped In 2O 3nanofibersFig.4Sensitivities (S )of the pure and Ce ⁃doped In 2O 3nanofibers to 10μL ·L -1TEA at different operatingtemperatures Fig.5Sensitivities vs measure time curves of 4%Ce ⁃doped In 2O 3nanofibers to different concentrations (6-300μL ·L -1)of TEA at 130℃3103Acta Phys.⁃Chim.Sin.,2010Vol.26rapidly with increasing the TEA concentrations,and then gradu-ally slow(30-200μL·L-1).Finally the fibers reach saturation atabout300μL·L-1.These results suggest that4%Ce⁃doped In2O3nanofibers can be used to detect TEA at low concentration level.In order to further understand the practicability of our fibers,thesensor was exposed to10μL·L-1TEA,CH3OH,H2S,TMA,C2H5OH,DMA,NH3,and H2O at130益,respectively.The se-lectivity shown in Fig.7indicates that the sample can distinguishTEA from these interferential gases effectively.Thus4%Ce⁃doped In2O3nanofibers can be used to detect TEA at various at-mospheres.The sensing mechanism of In2O3based gas sensors was clari-fied in previous work[12].The most widely accepted model is thatthe resistance change of the In2O3gas sensors is primarily causedby the adsorption and desorption of the gas molecules on thesurface of the In2O3film[5].When In2O3nanofibers are exposed toair,oxygen adsorbs on the exposed surface of the In2O3and isionized O-or O2-,resulting in a decrease of the carrier concen-tration and electron mobility.When exposed to a reducing gassuch as TEA,the reducing gas reacts with the adsorbed oxygenmolecules and releases the trapped electrons back to the conduc-tion band,which increases the carrier concentration and carriermobility of In2O3.Therefore the resistance change of the In2O3sensors can easily be found[7,12].The reaction between surfaceoxygen species and TEA can be simply described as[13]: (C2H5)3N+O-⇌CO2+H2O+NO2+e(1) (C2H5)3N+O2-⇌CO2+H2O+NO2+e(2) The high sensitivity and quick response/recovery speed of thesensor are attributed to the1D structure of these nanofibers.It iswidely accepted that1D nanostructure with high surface⁃to⁃vol-ume ratios(a higher surface area provides more sites for analytemolecules adsorption)can facilitate fast mass transfer of the ana-lyte molecules to and from the interaction region as well as re-quire charge carriers to transverse the barriers introduced bymolecular recognition along the1D nanostructures[14].Ce is widely used to enhance the sensing performances ofZnO,TiO2,as well as In2O3in this case[15-17].Former papers[18-20]re-ported that the existence of Ce in metal⁃oxide⁃semiconductorscan improve the sensitivity prominently.In our case,Ce sup-ports the catalytic conversion of TEA into its oxidation products.This may be due to spill⁃over of activated fragments to the semi-conductor surface to react with the adsorbed oxygen species andis called chemical sensitization[18-20].Another reason for the sens-ing improvement is that Ce may play the same role as La or oth-er rare earth elements in In2O3.They can increase the catalyticactivities of In2O3effectively,which is a base of the high sensi-tivity[21-23].Moreover,Pure In2O3has only one kind of grains ar-ranged uniformly,where in the case of activated films the grainsare of different natures such as CeO2and In2O3.The modifica-tion causes the formation of heterogeneous intergrain boundariesof CeO2⁃In2O3.And the increased barrier heights of the intergran-ular regions of activated In2O3will increase the sensor base resis-tivity,and thereby improve the film sensing properties accord-ingly[24].The sensitivity decreases in the case of6%Ce⁃dopedIn2O3nanofibers,which is based on that too many Ce clusterswill formed on the surface of In2O3at this situation,and the TEAmolecules will be burned on the cluster surface without causingany electrical signal,leading to the decrease of sensitivity[25].3ConclusionsIn summary,the TEA sensing properties of the pure and Ce⁃doped In2O3nanofibers are investigated.Among all the samples,4%Ce⁃doped In2O3nanofibers exhibit the highest sensitivitywith quick response and recovery speed.Especially,thesenanofibers can distinguish TEA from other interferential gaseseffectively.These results make Ce⁃doped In2O3nanofibers goodcandidates for fabricating TEA sensors.References1Koida,T.;Fujiwara,H.;Kondo,M.Solar Energy Materials&Solar Cells,2009,93:8512Prim,A.;Pellicer,E.;Rossinyol,E.;Peiró,F.;Cornet,A.;Morante, J.R.Adv.Funct.Mater.,2007,17:29573Chen,L.Y.;Liang,Y.;Zhang,Z.D.Eur.J.Inorg.Chem.,2009:9034Sharma,R.;Mane,R.S.;Min,S.K.;Han,pd., 2009,479:8405Wang,X.Q.;Zhang,M.F.;Liu,J.Y.;Luo,T.;Qian,Y.T.Sens.Actuators B⁃Chem.,2009,137:103Fig.7Selectivities of4%Ce⁃doped In2O3nanofibers atdifferentatmospheres Fig.6Sensitivities of4%Ce⁃doped In2O3nanofibers todifferent concentrations of TEA at130℃3104No.11WANG Jin⁃Xing et al.:Preparation and Triethylamine Sensing Properties of Ce⁃Doped In2O3Nanofibers6Vomiero,A.;Bianchi,S.;Comini,E.;Faglia,G.;Ferroni,M.;Poli, N.;Sberveglieri,G.Thin Solid Films,2007,515:83567Xu,P.C.;Cheng,Z.X.;Pan,Q.Y.;Xu,J.Q.;Xiang,Q.;Yu,W.J.;Chu,Y.L.Sens.Actuators B⁃Chem.,2008,130:8028Zheng,W.;Lu,X.F.;Wang,W.;Dong,B.;Zhang,H.N.;Wang,Z.J.;Xu,X.R.;Wang,C.J.Am.Ceram.Soc.,2010,93:159Natale,C.D.;Brunink,J.A.J.;Bungaro,F.;Davide,F.;d′Amico,A.;Paolesse,R.;Boschi,T.;Faccio,M.;Ferri,G.Meas.Sci.Technol.,1996,7:110310Wang,J.X.;Zou,B.;Ruan,S.P.;Zhao,J.;Wu,F.Q.Mater.Chem.Phys.,2009,117:48911Ge,C.Q.;Xie,C.S.;Cai,S.Z.Mater.Sci.Eng.B,2007,137:53 12Barsan,N.;Koziej,D.;Weimar,U.Sens.Actuators B⁃Chem., 2007,121:1813Takao,Y.;Nakanishi,M.;Kawaguchi,T.;Shimizu,Y.;Egashira, M.Sens.Actuators B⁃Chem.,1995,24-25:37514Kolmakov,A.;Moskovits,M.Annu.Rev.Mater.Res.,2004,34: 15115Al⁃Kuhaili,M.F.;Durrani,S.M.A.;Bakhtiari,I.A.Appl.Surf.Sci.,2007,255:303316Trinchi,A.;Li,Y.X.;Wlodarski,W.;Kaciulis,S.;Pandolfi,L.;Viticoli,S.;Comini,E.;Sberveglieri,G.Sens.Actuators B⁃Chem., 2003,95:14517Yamazoe,N.;Sakai,G.;Shimanoe,,2003,7: 6318Fang,G.J.;Liu,Z.L.;Liu,C.Q.;Yao,K.L.Sens.Actuators B⁃Chem.,2000,66:4619Siemons,M.;Simon,U.Sens.Actuators B⁃Chem.,2006,120:110 20Ruiz,A.M.;Cornet,A.;Morante,J.R.Sens.Actuators B⁃Chem., 2004,100:25621Kapse,V.D.;Ghosh,S.A.;Chaudhari,G.N.;Raghuwanshi,F.C.;Gulwade,D.D.Vacuum,2009,83:34622Xu,L.;Dong,B.;Wang,Y.;Bai,X.;Chen,J.;Liu,Q.;Song,H.J.Phys.Chem.C,2010,114:908923Niu,X.;Zhong,H.;Wang,X.;Jiang,K.Sens.Actuators B⁃Chem., 2006,115:43424Patil,D.R.;Patil,L.A.;Patil,P.P.Sens.Actuators B⁃Chem., 2007,126:36825Belmonte,J.C.;Manzano,J.;Arbiol,J.;Cirera,A.;Puigcorbé,J.;Vilà,A.;Sabaté,N.;Gràcia,I.;Cané,C.;Morante,J.R.Sens.Actuators B⁃Chem.,2006,114:8813105。
仿生材料论文综述大学生Biomimetic materials
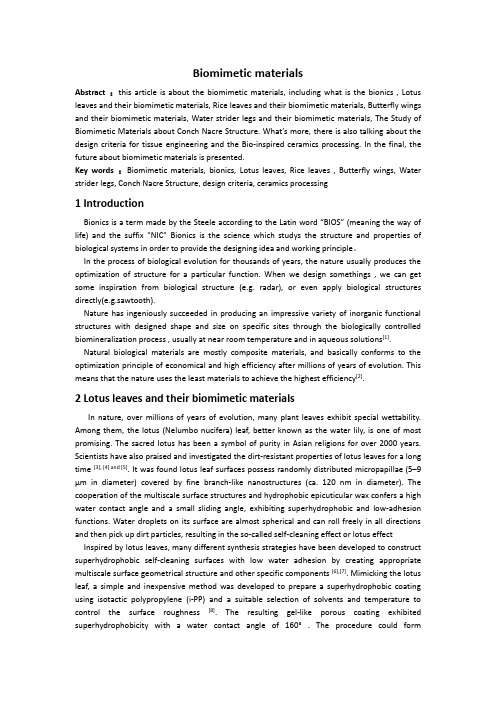
Biomimetic materialsAbstract :this article is about the biomimetic materials, including what is the bionics , Lotus leaves and their biomimetic materials, Rice leaves and their biomimetic materials, Butterfly wings and their biomimetic materials, Water strider legs and their biomimetic materials, The Study of Biomimetic Materials about Conch Nacre Structure. What’s more, there is also talking about the design criteria for tissue engineering and the Bio-inspired ceramics processing. In the final, the future about biomimetic materials is presented.Key words :Biomimetic materials, bionics, Lotus leaves, Rice leaves , Butterfly wings, Water strider legs, Conch Nacre Structure, design criteria, ceramics processing1 IntroductionBionics is a term mad e by the Steele according to the Latin word “BIOS” (meaning the way of life) and the suffix "NIC" Bionics is the science which studys the structure and properties of biological systems in order to provide the designing idea and working principle。
气相检测醋酸灵敏度降低的原因
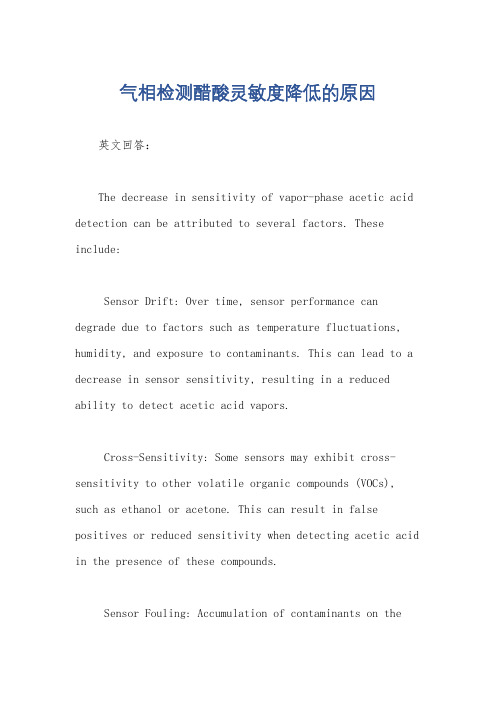
气相检测醋酸灵敏度降低的原因英文回答:The decrease in sensitivity of vapor-phase acetic acid detection can be attributed to several factors. These include:Sensor Drift: Over time, sensor performance can degrade due to factors such as temperature fluctuations, humidity, and exposure to contaminants. This can lead to a decrease in sensor sensitivity, resulting in a reduced ability to detect acetic acid vapors.Cross-Sensitivity: Some sensors may exhibit cross-sensitivity to other volatile organic compounds (VOCs), such as ethanol or acetone. This can result in false positives or reduced sensitivity when detecting acetic acid in the presence of these compounds.Sensor Fouling: Accumulation of contaminants on thesensor surface can block or hinder the interaction of acetic acid molecules with the sensing element. This can lead to a decrease in sensitivity and reduced detection accuracy.Environmental Factors: Ambient temperature and humidity can affect sensor performance. Extreme temperatures or high humidity levels can cause sensor drift or reduced sensitivity, impacting the detection of acetic acid vapors.Sensor Aging: Over time, the sensing element may undergo physical or chemical changes that can affect its performance. This can lead to a gradual decrease in sensitivity and a reduced ability to detect acetic acid vapors.中文回答:醋酸气相检测灵敏度降低的原因有多种,包括:传感器漂移,随着时间的推移,传感器性能会因温度波动、湿度和接触污染物等因素而下降。
球形花状结构氧化锡光催化降解亚甲基蓝
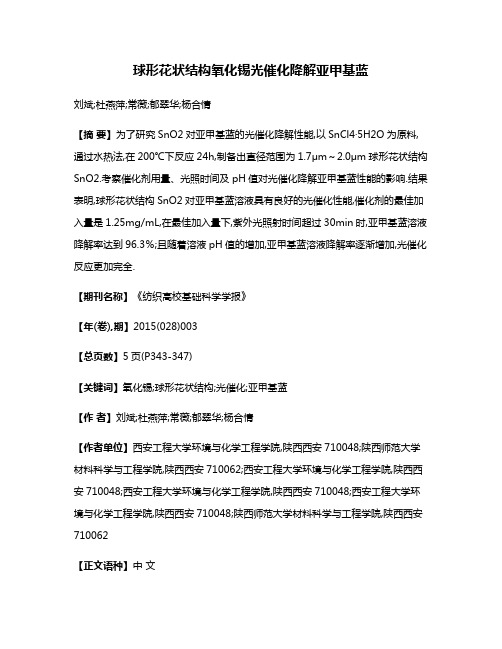
球形花状结构氧化锡光催化降解亚甲基蓝刘斌;杜燕萍;常薇;郁翠华;杨合情【摘要】为了研究SnO2对亚甲基蓝的光催化降解性能,以SnCl4·5H2O为原料,通过水热法,在200℃下反应24h,制备出直径范围为1.7μm~2.0μm球形花状结构SnO2.考察催化剂用量、光照时间及pH值对光催化降解亚甲基蓝性能的影响.结果表明,球形花状结构SnO2对亚甲基蓝溶液具有良好的光催化性能,催化剂的最佳加入量是1.25mg/mL,在最佳加入量下,紫外光照射时间超过30min时,亚甲基蓝溶液降解率达到96.3%;且随着溶液pH值的增加,亚甲基蓝溶液降解率逐渐增加,光催化反应更加完全.【期刊名称】《纺织高校基础科学学报》【年(卷),期】2015(028)003【总页数】5页(P343-347)【关键词】氧化锡;球形花状结构;光催化;亚甲基蓝【作者】刘斌;杜燕萍;常薇;郁翠华;杨合情【作者单位】西安工程大学环境与化学工程学院,陕西西安710048;陕西师范大学材料科学与工程学院,陕西西安710062;西安工程大学环境与化学工程学院,陕西西安710048;西安工程大学环境与化学工程学院,陕西西安710048;西安工程大学环境与化学工程学院,陕西西安710048;陕西师范大学材料科学与工程学院,陕西西安710062【正文语种】中文【中图分类】O643金属氧化物,如二氧化锡(SnO2)、氧化锌(ZnO)、二氧化钛(TiO2)等,由于其独特的物理和化学性能,近年来备受关注[1-2].SnO2是一种重要的n-型半导体材料,其带隙宽度为3.6eV,由于其独特的光学、电学和化学性质,在锂离子电池、染料敏化太阳能电池、气敏传感器、光催化及透明电极等领域具有广泛地应用[3].这些器件的性能在很大程度上取决于SnO2纳米结构单元的尺寸、形貌及其组装形成的纳米结构.因此,不同尺寸和形貌的SnO2的制备成为研究热点.目前为止,研究人员已经通过多种方法,如化学气相沉积、热蒸发法、水热法、微波法和溶胶凝胶法,制备了SnO2纳米粒子、纳米棒、纳米带、纳米片、纳米管、纳米线、纳米花、空心球等纳米结构,并对这些纳米结构的物理及化学性能进行了测试[4-11].SnO2纳米管[8]和空心球[11]已经被应用于制造锂离子电池的负极,测试发现,这些SnO2纳米结构具有较高的储锂容量和良好的电循环性能.此外,SnO2纳米带、纳米线、纳米粒子、纳米棒和纳米管等纳米结构已经被用于构筑各种气体传感器,并被广泛应用于乙醇、一氧化碳、氢气、二氧化氮、2-丙醇等气体和液体的测定[12-16].结果表明,这些具有不同纳米结构的SnO2传感器比SnO2粉末具有更高的灵敏度.文献[17-18]研究了SnO2纳米棒和纳米花对罗丹明B降解的光催化能力,结果发现,SnO2纳米棒[17]和纳米花[18]对罗丹明B降解的催化性能高于SnO2纳米颗粒.然而,有关球形花状纳米结构SnO2光催化降解亚甲基蓝的研究至今未见报道.本文通过水热法制备球形花状纳米结构SnO2光催化剂,以亚甲基蓝溶液为研究对象,讨论催化剂的用量、光照时间及pH值对球形花状纳米SnO2光催化性能的影响.1.1 试剂与仪器(1) 试剂四氯化锡(SnCl4·5H2O,分析纯,天津市科密欧化学试剂有限公司),氢氧化钠(NaOH,分析纯,天津市科密欧化学试剂有限公司),聚乙烯吡咯烷酮(PVP, Mr=30000,分析纯,国药集团化学试剂有限公司),亚甲基蓝(分析纯,北京化工厂),二次蒸馏水.(2)仪器 IKA RO10型磁力搅拌器(广州仪科实验室技术有限公司),DHA-9246A型电热恒温鼓风干燥箱(上海精宏实验设备有限公司),AL-204型电子天平(梅特勒-托利多仪器有限公司),TGL-16G型台式高速离心机(上海安亭科学仪器厂),UV-2450型紫外可见分光光度计(日本岛津公司),BL-GHX-V型光化学反应仪(西安比朗生物科技有限公司).1.2 方法(1) 球形花状结构SnO2的制备根据文献[19],称取0.500g SnCl4·5H2O于50 mL 烧杯中,再向其中依次加入2mL, 6M NaOH,0.5g PVP和13mL H2O,搅拌形成清亮溶液.将该溶液转入具有聚四氟乙烯内衬的反应釜中,密封反应釜,在200℃下反应24h,然后自然冷却至室温,得白色成淀.离心后分别用去离子水和无水乙醇依次洗涤3次,室温下,最终得到白色粉末状产物.(2) 光催化实验实验采用BL-GHX-V型光化学反应仪,光源是300W汞灯.首先,量取20mL,10mg/L的亚甲基蓝溶液于体积为50mL的石英试管中,再称取不同量的SnO2样品分散到亚甲基蓝溶液中,于暗处震荡10min,使亚甲基蓝分子和半导体光催化剂表面建立起吸附脱附平衡,然后置于紫外灯管下,进行测试.由于溶液pH值不同,对亚甲基蓝的结构会产生不同的影响,为了仅考虑SnO2对亚甲基蓝的光催化降解性能,所以在光催化实验方法中没有控制溶液的pH.所有实验均在室温下进行,照射一定时间后取出,高速离心,将光催化剂与溶液进行分离,取上层清液用岛津UV-2450紫外可见分光光度计进行分析测定.(3) 降解率计算先用紫外可见分光光度计对亚甲基蓝进行全波段(190nm~800nm) 扫描,确定亚甲基蓝的最大吸收波长(664 nm),再用紫外可见分光光度计在此波长下测定清液的吸光度.由Lambert-Beer定律可知:A=εbc,式中b为光程,cm;c为质量浓度,g/L;ε为质量吸光系数,L/g·cm,亚甲基蓝溶液的降解率按照下面的公式进行计算:式中:η为亚甲基蓝溶液的降解率,%;C0为含有SnO2样品的亚甲基蓝溶液的初始浓度,g/L;C为含有SnO2样品的亚甲基蓝溶液光照不同时间后的实际浓度,g/L;A0为含有SnO2样品的亚甲基蓝溶液的初始吸光度;A为含有SnO2样品的亚甲基蓝溶液光照不同时间后的吸光度.2.1 SEM和XRD分析制备的球形花状结构SnO2的SEM和XRD图谱如图1所示.从图1(a)可看出,所制备的产物由大量的球形花状结构物质组成,其直径范围为1.7μm~2.0μm.由图1(b)可见,所有衍射峰均指向四方相SnO2(JCPDS卡号:41-1445).此外,其衍射峰比较尖锐,说明该产物结晶性很高.除此之外,没有其他杂质峰出现, 说明所制备的产物为纯的四方相SnO2.2.2 亚甲基蓝溶液的吸收光谱分别测定未经紫外光照射的亚甲基蓝溶液,紫外光照射30min后的亚甲基蓝溶液和含有SnO2球形花状结构并经紫外光照射30min后的亚甲基蓝溶液的吸收光谱,其结果如图2所示.从图2可以看出,亚甲基蓝溶液的最大吸收波长为664nm(曲线Ⅰ),当紫外光照射30min后,其吸光度大约下降了12%(曲线Ⅱ),而含有SnO2球形花状结构的亚甲基蓝溶液,在紫外光照射30min后,其吸光度大约下降了96%(曲线Ⅲ).由此可见,采用球形花状结构SnO2作为光催化剂可有效降解亚甲基蓝溶液.2.3 SnO2用量对降解率的影响在光催化反应中,催化剂的用量是非常重要的影响因素.在20mL,10mg/L的亚甲基蓝溶液中加入光催化剂SnO2,用300W汞灯照射,通过改变SnO2的加入量,探究紫外光照射30min后亚甲基蓝溶液的降解率,其结果如图3所示.由图3可知,随着SnO2用量的增加,亚甲基蓝溶液的降解率逐渐增加.但当加入量至25mg后,亚甲基蓝溶液的降解率增加缓慢,不再有明显的提高.故SnO2最佳用量为25mg.2.4 光照时间对降解率的影响在其他反应条件固定不变的情况下,在20mL亚甲基蓝溶液中加入25mg 光催化剂SnO2,研究不同紫外光照射时间下亚甲基蓝溶液的降解率,其结果如图4所示.由图4 可知,随光照时间的延长,亚甲基蓝溶液的降解率不断升高,但是当光照时间超过30min后,降解率升高至最大值96.3%且增加缓慢,说明亚甲基蓝溶液基本降解完全.2.5 pH值对降解率的影响向7份,20mL亚甲基蓝溶液中分别加入25mg 光催化剂SnO2,用HCl和NaOH调节溶液pH值分别为1,3,5,7,9,11,13,用300W汞灯光照20min后,计算降解率,其结果如图5所示.由图5可见,随着溶液pH值的增加,亚甲基蓝溶液的降解率逐渐增加.由于SnO2受到紫外光照射后,会产生空穴,空穴可以和OH-反应生成羟基自由基(·OH),·OH具有很强的氧化性,可以使亚甲基蓝氧化褪色[20].在酸性pH范围时,亚甲基蓝溶液的降解率增加缓慢,这是由于在酸性溶液中,OH-浓度较低,所产生的·OH的数目相对较小,所以降解率增加缓慢.当溶液由酸性过渡到碱性时,OH-增加很多,产生的·OH数目随之增加,所以在溶液pH=7的前后,亚甲基蓝溶液的降解率大幅增加,当溶液的pH值增加到11以后,亚甲基蓝溶液的降解率达91%,亚甲基蓝溶液基本降解完全.由于光照时间较短只有20min,所以其降解率低于光照30min的降解率.本文通过水热法制备了对亚甲基蓝具有良好光催化性能的球形花状结构SnO2,并考察了催化剂用量、光照时间及溶液pH值对其光催化性能的影响.结果表明,300W汞灯照射30min,SnO2最佳加入量1.25mg/mL;当紫外光照时间超过30 min时,亚甲基蓝溶液降解率达到了96.3%.当溶液pH由酸性变化到碱性时,亚甲基蓝溶液降解率大幅增加,降解更加完全.球形花状SnO2的制备方法操作方便、条件温和、设备简单,有望用于大规模生产,且可能应用于其他氧化物花状结构的制备,预计其在染料废水处理方面有一定的应用价值.当然,这种光催化剂的性能还没有与其他光催化剂进行比较,此外,这种光催化剂对不同染料的降解性能和降解机理还有待于进一步的研究.【相关文献】[1] 余花娃,樊慧庆,王晶,等.Co掺杂ZnO微/纳米纤维的制备及其光催化性能[J].纺织高校基础科学学报,2014,27(2):244-247.YU Huawa,FAN Huiqing,WANG Jing,et al.Preparation and photocatalytic characterization of Co-doped ZnO micro/nanofibers[J].Basic Sciences Journal of TextileUniversities,2014,27(2):244-247.[2] 王文静,郭晓玲,王志刚,等.纳米二氧化钛光催化净化酸性染料废水的研究[J].西安工程大学学报,2011,25(2):216-219.WANG Wenjing,GUO Xiaoling,WANG Zhigang,et al.Study of photocatalysis purification of acid dyeing wastewater with titania[J].Journal of Xi′an PolytechnicUniversity,2011,25(2):216-219.[3] WANG X,HAN X G,XIE S F.Controlled synthesis and enhanced catalytic and gas-sensing properties of tin dioxide nanoparticles with exposed high-energy facets[J].Chem Eur J,2012,18(8):2283-2289.[4] KRISHNAKUMAR T,JAYAPRAKASH R,PARTHIBAVARMAN M.Microwave-assisted synthesis and investigation of SnO2 nanoparticles[J].Mater Lett,2009,63(11):896-898. [5] LUPAN O,CHOW L,CHAI G,et al.Synthesis of one-dimensional SnO2 nanorods via a hydrothermal technique[J].Physica E,2009,41(4):533-536.[6] MA X L,LI Y,ZHU Y L.Growth mode of the SnO2 nanobelts synthesized by rapid oxidation[J].Chem Phys Lett,2003,376(5/6):794-798.[7] KUMAR B,LEE D H,KIM S H,et al.General route to single-crystalline SnO2 nanosheets on arbitrary substrates[J].J Phys Chem C,2010,114(25):11050-11055.[8] LI L M,YIN X M,LIU S,et al.Electrospun porous SnO2 nanotubes as high capacity anode materials for lithium ion batteries[J].Electrochem Commun,2010,12(10):1383-1386.[9] CHEN Z W,JIAO Z,WU M H,et al.Bulk-quantity synthesis and electrical properties of SnO2 nanowires prepared by pulsed delivery[J].Mater Chem Phys,2009,115(2/3):660-663.[10] NING J J,DAI Q Q,JIANG T,et al.Facile synthesis of tin oxide nanoflowers:A potential high-capacity lithium-ion-storage material[J].Langmuir,2009,25(3):1818-1821.[11] LOU X W,WANG Y,YUAN C L,et al.Template-free synthesis of SnO2 hollownanostructures with high lithium storage capacity[J].Adv Mater,2006,18(17):2325-2329.[12] ANDREI P,FIELDS L L,ZHENG J P,et al.Modeling and simulation of single nanobelt SnO2 gas sensors with FET structure[J].Sens Actuators B,2007,128 (1):226-234.[13] WANG B,ZHU L F,YANG Y H,et al.Fabrication of a SnO2 nanowire gas sensor and sensor performance for hydrogen[J].J Phys Chem C,2008,112(17):6643-6647.[14] MATIN B M,MORTAZAVI Y,KHODADADI A A,et al.Alkaline-and template-free hydrothermal synthesis of stable SnO2 nanoparticles and nanorods for CO and ethanol gas sensing[J].Sens Actuators B,2010,151 (1):140-145.[15] WANG D,CHU X F,GONG M L.Gas-sensing properties of sensors based on single-crystalline SnO2 nanorods prepared by a simple molten-salt method[J].Sens Actuators B,2006,117(1):183-187.[16] WANG G X,PARK J S,PARK M S,et al.Synthesis and high gas sensitivity of tin oxide nanotubes[J].Sens Actuators,B,2008,131(1):313-317.[17] CHENG G E,CHEN J Y,KE H Z,et al.Synthesis,characterization and photocatalysis of SnO2 nanorods with large aspect ratios[J].Mater Lett,2011,65(21/22):3327-3329. [18] DAI S D,YAO Z L.Synthesis of flower-like SnO2 single crystals and its enhanced photocatalytic activity[J].Appl Surf Sci,2012,258(15):5703-5706.[19] LIU B,ZHANG L H,ZHAO H,et al.Synthesis and sensing properties of spherical flowerlike architectures assembled with SnO2 submicron rods[J].Sens ActuatorsB,2012,173:643-651.[20] WU S S,CAO H Q,YIN S F,et al.Amino acid-assisted hydrothermal synthesis and photocatalysis of SnO2 nanocrystals[J].J Phys Chem C,2009,113(41):17893-17898.。
色谱中英文对照

色谱中英文对照中英文对照色谱词汇间接检测indirect detection间接荧光检测indirect fluorescence detection间接紫外检测indirect ultraviolet detection检测器detector检测器检测限detector detectability检测器灵敏度detector sensitivity检测器线性范围detector linear range阴离子交换剂anion exchanger阴离子交换色谱法anion exchange chromatography, AEC高速逆流色谱法high speed counter-current chromatography高温凝胶色谱法high temperature gel chromatography高效液相色谱-付里叶变换红外分析法high performance liquid ch…高效液相色谱法high performance liquid chromatography高效柱high performance column高压流通池技术high pressure flow cell technique高压输液泵high pressure pump高压梯度high-pressure gradient高压液相色谱法high pressure liquid chromatography阴离子交换树脂anion exchange resin荧光薄层板fluorescent thin layer plate荧光检测器fluorescence detector荧光色谱法fluorescence chromatography迎头色谱法frontal chromatography迎头色谱法frontal method硬(质)凝胶hard gel有机改进剂organic modifier有机相生物传感器Organic biosensor有效峰数effective peak number EPN有效理论塔板数number of effective theoretical plates有效塔板高度effective plate height有效淌度effective mobility淤浆填充法slurry packing method予柱pre-column在线电堆集on-line electrical stacking在柱电导率检测on-column electrical conductivity detection噪声noise噪信比noise –signal ratio增强紫外-可见吸收检测技术UV-visible absorption enhanced det…窄粒度分布narrow particle size distribution折射率检测器refractive index detector, RID真空脱气装置vacuum degasser阵列毛细管电泳capillary array electrophoresis蒸发光散射检测器evaporative light-scattering detector, ELSD整体性质检测器integral property detector正相高效液相色谱法normal phase high performance liquid chro…正相离子对色谱法normal phase ion-pair chromatography正相毛细管电色谱positive capillary electrokinetic chromatog…直接化学离子化direct chemical ionization GC-MS直接激光在柱吸收检测on-column direct laser detection纸色谱法paper chromatography置换色谱法displacement chromatography制备色谱preparative chromatography制备色谱仪preparative chromatograph制备柱preparation column智能色谱chromatography with artificial intelligence质量色谱mass chromatography质量型检测器mass detector质量型检测器mass flow rate sensitive detector中压液相色谱middle-pressure liquid chromatography重建色谱图reconstructive chromatogram重均分子量weight mean molecular weight轴向扩散longitudinal diffusion轴向吸收池absorption pool of axial direction轴向压缩柱axial compression column柱端电导率检测out-let end detection of electrical conductiv…柱负载能力column loadability柱后衍生化post-column derivatization柱老化condition (aging) of column柱流出物(column) effluent柱流失column bleeding柱内径column internal diameter柱前衍生化pro-column derivatization柱切换技术column switching technique柱清洗column cleaning柱容量column capacity柱入口压力column inlet pressure柱色谱法column chromatography柱上检测on-line detection柱渗透性column permeability柱寿命column life柱头进样column head sampling柱外效应extra-column effect柱温箱column oven柱效column efficiency柱压column pressure柱再生column regeneration柱中衍生化on-column derivatization注射泵syringe pump转化定量法trans-quantitative method紫外-可见光检测器ultraviolet visible detector, UV-Vis紫外吸收检测器ultraviolet absorption detector自动进样器automatic sampler自由溶液毛细管电泳free solution capillary electrophoresis总分离效能指标over-all resolution efficiency总交换容量total exchange capacity总渗透体积total osmotic volume纵向扩散longitudinal diffusion组合式仪器系统building block instrument最佳流速optimum flow rate最佳实际流速optimum practical flow rate最小检测量minimum detectable quantity最小检测浓度minimum detectable concentration萃取色谱法extraction chromatography脱气装置degasser外标法external standard method外梯度outside gradient网状结构reticular structure往复泵reciprocating pump往复式隔膜泵reciprocating diaphragm pump微分型检测器differential detector微孔树脂micro-reticular resin微库仑检测器micro coulometric detector微量进样针micro-syringe微量色谱法micro-chromatography微乳液电动色谱microemulsion electrokinetic chromatography 微生物传感器Microbial sensor微生物显影bioautography微填充柱micro-packed column微吸附检测器micro adsorption detector微型柱micro-column涡流扩散eddy diffusion无机离子交换剂inorganic ion exchanger无胶筛分毛细管电泳non-gel capillary electrophoresis无孔单分散填料non-porous monodisperse packing无脉动色谱泵pulse-free chromatographic pump物理钝化法physical deactivation吸附等温线adsorption isotherm吸附剂adsorbing material吸附剂活性adsorbent activity吸附平衡常数adsorption equilibrium constant吸附溶剂强度参数adsorption solvent strength parameter吸附色谱法adsorption chromatography吸附型PLOT柱adsorption type porous-layer open tubular colum…吸附柱adsorption column吸光度比值法absorbance ratio method洗脱强度eluting power显色器color-developing sprayer限制扩散理论theory of restricted diffusion线速度linear velocity线性梯度linear gradient相比率phase ratio相对保留值relative retention value相对比移值relative Rf value相对挥发度relative volatility相对灵敏度relative sensitivity相对碳(重量)响应因子relative carbon response factor相对响应值relative response相对校正因子relative correction factor相交束激光诱导的热透镜测量heat lens detection of intersect …相似相溶原则rule of similarity响应时间response time响应值response小角激光散射光度计low-angle laser light scattering photomet…小内径毛细管柱Microbore column校正保留体积corrected retention volume校正曲线法calibration curve method校正因子correction factor旋转薄层法rotating thin layer chromatography旋转小室逆流色谱rotational little-chamber counter-current c…选择性检测器selective detector循环色谱法recycling chromatography压电晶体piezoelectric crystal压电免疫传感器Piezoelectric Immunosensor压电转换器piezoelectric transducer压力保护pressure protect压力上限pressure high limit压力梯度校正因子pressure gradient correction factor压力下限pressure low limit衍生化法derivatization method衍生化试剂derivatization reagent阳离子交换剂cation exchanger阳离子交换色谱法cation exchange chromatography, CEC氧化铝色谱法alumina chromatography样品环sample loop样品预处理sample pretreatment液-液分配色谱法liquid-liquid partition chromatography液-液色谱法liquid-liquid chromatography液滴逆流色谱drop counter-current chromatography液固色谱liquid-solid chromatography液晶固定相liquid crystal stationary phase液态离子交换剂liquid ion exchanger液相传质阻力resistance of liquid mass transfer液相色谱-傅里叶变换红外光谱联用liquid chromatography-FTIR 液相色谱-质谱分析法liquid chromatography-mass spectrometry 液相色谱-质谱仪liquid chromatography-mass spectrometer液相色谱法liquid chromatography液相载荷量liquid phase loading溶剂效率solvent efficiency溶解度参数solubility parameter溶液性能检测器solution property detector溶胀swelling溶质性质检测器solute property detector容量因子capacity factor渗透极限分子量permeation limit molecular weight生物色谱biological chromatography生物特异性柱biospecific column生物自显影法bioautography升温速率temperature rate湿法柱填充wet column packing十八烷基键合硅胶octadecyl silane石墨化碳黑graphitized carbon black示差折光检测器differential refraction detector试剂显色法reagent color-developing method手动进样器manual injector手性氨基酸衍生物GC固定相chiral amino acid derivatives stat…手性拆分试剂chiral selectors手性固定相chiral stationary phase手性固定相拆分法chiral solid phase separation手性环糊精衍生物GC固定相chiral cyclodextrin der GC手性金属络合物GC固定相chirametal stationary phase in GC手性流动相chiral mobile phase手性流动相拆分法chiral mobile phase separation手性色谱chiral chromatography手性试剂chiral reagent手性衍生化法chiral derivation method疏溶剂理论solvophobic theory疏溶剂色谱法solvophobic chromatography疏溶剂作用理论solvophobic interaction principle疏水作用色谱hydrophobic interaction chromatography树脂交换容量exchange capacity of resin数均分子量number mean molecular weight双保留机理dual reservation mechanism双活塞往复泵two-piston reciprocating pump双束差分检测器detector of dual-beam difference 双柱色谱法dual column chromatography水凝胶hydragel水系凝胶色谱柱aqua-system gel column死区域dead zone死体积dead volume塔板理论方程plate theory equation碳分子筛carbon molecular sieve特殊选择固定液selective stationary phase梯度洗脱gradient elution梯度洗脱装置gradient elution device梯度液相色谱gradient liquid chromatography体积排斥理论size exclusion theory体积排斥色谱size exclusion chromatography体积色谱法volumetric chromatography填充柱packed column填料packing material停流进样stop-flow injection通用型检测器common detector涂层毛细管coated capillary拖尾峰tailing peak拖尾因子tailing factor流动分离理论separation by flow流动相mobile phase流动相梯度eluent gradient流体动力学进样hydrostatic pressure injection流体力学体积hydrodynamic volume流型扩散dispersion due to flow profile脉冲阻尼器pulse damper酶传感器Enzyme sensor酶联免疫传感器Enzyme linked immunosensor 酶免疫分析enzyme immnunoassay内标法internal standard method内标物internal standard内梯度inside gradient逆流色谱法counter-current chromatography逆流色谱仪counter current chromatograph凝胶过滤色谱gel filtration chromatography凝胶内体积gel inner volume凝胶色谱法gel chromatography凝胶色谱仪gel chromatograph凝胶渗透色谱gel permeation chromatography凝胶外体积gel interstitial volume凝胶柱gel column浓度梯度成像检测器concentration gradient imaging detector浓度型检测器concentration detector排斥极限分子量exclusion limit molecular weight排斥体积exclusion volume排阻薄层色谱法exclusion TLC漂移drift迁移时间migration time迁移时间窗口the window of migration time前延峰leading peak前沿色谱法frontal chromatography强碱性阴离子交换剂strong-base anion exchanger强酸性阳离子交换剂strongly acidic cation exchanger切换时间switching time去离子水deionized water全多孔硅胶macro-reticular silica gel全多孔型填料macro-reticular packing material全二维色谱Comprehensive two-dimensional gas chromatography…全硅烷化去活complete silylanization deactivation溶剂强度solvent strength激光解吸质谱法laser desorption MS, LDMS激光色谱laser chromatography激光诱导光束干涉检测detection of laser-induced light beam I…激光诱导毛细管振动测量laser-reduced capillary vibration det…激光诱导荧光检测器laser-induced fluorescence detector记忆峰memory peak记忆效应memory effect夹层槽sandwich chamber假峰ghost peak间断洗脱色谱法interrupted-elution chromatography间接光度(检测)离子色谱法indirect photometric ion chromato…间接光度(检测)色谱法indirect photometric chromatography减压液相色谱vacuum liquid chromatography键合固定相bonded stationary phase键合型离子交换剂bonded ion exchanger焦耳热joule heating胶束薄层色谱法micellar thin layer chromatography胶束液相色谱法micellar liquid chromatography交联度crosslinking degree阶梯梯度stagewise gradient进样阀injection valve进样量sample size进样器injector聚苯乙烯PSDVB聚硅氧烷高温裂解去活high-temperature pyrolysis deactivation…聚合物基质离子交换剂polymer substrate ion exchanger绝对检测器absolute detector可见光检测器visible light detector可交换离子exchangable ion空间性谱带加宽band broadening in space空穴色谱法vacancy chromatography孔结构pore structure孔径pore diameter孔径分布pore size distribution控制单元control unit快速色谱法high-speed chromatography理论塔板高度height equivalent to a theoretical plate(HETP)理论塔板数number of theoretical plates峰面积peak area峰面积测量法measurement of peak area峰面积校正calibration of peak area峰容量peak capacity固定相stationary phase固定液stationary liquid固定液的相对极性relative polarity of stationary liquid固定液极性stationary liquid polarity固相扩散solid diffusion固相荧光免疫分析solid phase fluorescence immunoassay固有粘度intrinsic viscosity光散射检测器light scattering detector硅胶silica gel硅烷化法silanization硅烷化法silanizing硅烷化载体silanized support过压液相色谱法over pressured liquid chromatography,OPLC恒流泵constant flow pump恒温操作constant temperature method恒压泵constant pressure pump红色载体red support红外检测器infrared detector红外总吸光度重建色谱图total infrared absorbance reconstruct…化合物形成色谱compound-formation chromatography化学发光检测器chemiluminescence detector化学发光检测器Chemiluminescence detector, SCD化学键合固定相bonded stationary phase化学键合相色谱bonded phase chromatography化学色谱法chemi-chromatography环糊精电动色谱cyclodextrin electrokinetic chromatography环形展开比移值circular development Rf value环形展开法circular development缓冲溶液添加剂buffer additives辉光放电检测器glow discharge detector混合床离子交换固定相mixed-bed ion exchange stationary phase 混合床柱mixed bed column活塞泵piston pump活性activation活性硅胶activated silica gel活性氧化铝activated aluminium oxide基流background current or base current基线baseline基线宽度baseline width基质substrate materials基质隔离技术matrix isolation technique电歧视效应the effect of electrical discrimination电迁移进样electrophoretic injection电渗流electroendosmotic flow电渗流标记物electroendosmotic flow marker电渗流淌度electroendosmotic mobility电泳淌度electrophoretic mobility调整保留时间adjusted retention time调整保留体积adjusted retention volume叠加内标法added internal standard method高效毛细管电泳high-performance capillary electrophoresis归一化法normalization method毛细管等电聚焦capillary isoelectric focusing毛细管等速电泳isotachophoresis毛细管电色谱capillary electrochromatography毛细管电泳capillary electrophoresis毛细管电泳电喷雾质谱联用capillary electrophoresis – electr芯片电泳microchip electrophoresis色谱法chromatography色谱峰chromatographic peak色谱峰区域宽度peak width色谱富集过样samt injection of chromatography色谱工作站chromatographic working station色谱图chromatogram色谱仪chromatograph色谱柱chromatographic column色谱柱column色谱柱切换技术switching column technique毛细管超临界流体色谱法capillary supercritical fluid chromat…毛细管电泳基质辅助激光解吸电离质谱离线检测off-line capillar…毛细管电泳离子分析capillary ion analysis毛细管电泳免疫分析immunity analysis of capillary electropho…毛细管胶束电动色谱micellar electrokinetic chromatography毛细管凝胶电泳capillary gel electrophoresis毛细管凝胶柱capillary gel column毛细管亲和电泳affinity capillary electrophoresis毛细管区带电泳capillary zone electrophoresis毛细管有效长度the effective length of capillary electrophor…二极管阵列检测器diode-array detector, DAD二维色谱法two-dimensional chromatography二元溶剂体系dual solvent system反冲洗back wash反吹技术back flushing technique反峰negative peak反离子counter ion反相高效液相色谱法reversed phase hig h performance liquid ch…反相离子对色谱reversed phase ion pair chromatography反相离子对色谱法reversed phase ion-pair chromatography反相毛细管电色谱reverse capillary electrokinetic chromatogr…反相柱reversed phase column反应色谱reaction chromatography反圆心式展开anti-circular development反转电渗流reverse electroendosmotic flow范第姆特方程式van Deemter equation仿生传感器Biomimic electrode放射性检测器radioactivity detector放射自显影autoradiography非极性固定相non-polar stationary phase非极性键合相non-polar bonded phase非水系凝胶色谱柱non-aqua-system gel column非水相色谱nonaqueous phase chromatography非吸附性载体non-adsorptive support非线性分流non-linearity split stream非线性色谱non-linear chromatography非线性吸附等温线non-linear adsorption isotherm酚醛离子交换树脂phenolic ion exchange resin分离-反应-分离展开SRS development分离数separation number分离因子separation factor分离柱separation column分配等温线distribution isotherm分配色谱partition chromatography分配系数partition coefficient分析型色谱仪analytical type chromatograph分子扩散molecular diffusion封尾endcapping峰高peak heightpH梯度动态分离dynamic separation of the pH gradientpH值梯度洗脱pH gradient elutionZata电势Zata potentialZ形池Z-form pool氨基键合相amino-bonded phase氨基酸分析仪amino acid analyzer安培检测器ampere detector白色载体white support半微柱semimicro-column半制备柱semi-preparation column包覆型离子交换剂coated ion exchanger包覆型填料coated packing material保护柱guard column保留间隙retention gap保留时间retention time保留体积retention volume保留温度retention temperature保留值定性法retention qualitative method保留值沸点规律boiling point rule of retention保留值碳数规律carbon number rule of retention保留指数retention index单活塞往复泵single piston reciprocating pump单相色谱仪single phase chromatograph单向阀one-way valve单柱离子色谱法single column ion chromatography等度洗脱isocratic elution等离子体色谱法plasma chromatography等途电泳-毛细管区带电泳耦合进样isotachophoresis injection-c…低负荷柱low load column低容量柱low capacity column低压梯度low-pressure gradient低压液相色谱low-pressure liquid chromatography电导池conductance cell电导检测法conductance detection电荷转移分光光度法charge transfer spectrophotometry电化学检测器electrochemical detector电解抑制器electrolyze suppressor保留指数定性法retention index qualitative method背景电导background conductance苯酚磺酸树脂phenol sulfonic acid resin苯乙烯styrene比保留体积specific retention volume比例阀proportional valve比渗透率specific permeability比移值Rf value便携式色谱仪portable chromatograph标准偏差standard deviation表观电泳淌度apparent electrophoretic mobility表观交换容量apparent exchange capacity表面电位检测器surface potential detector表面多孔硅胶superficially porous silica gel表面多孔填料superficially porous packing material表面多孔型离子交换剂superficially porous ion-exchanger玻璃球载体glass beads support不分流进样splitless sampling参比柱reference column场放大进样electrical field magnified injection场流分离field-flow fractionation场流分离仪field-flow fractionation场效应生物传感器Field effect transistor based Biosensor常压液相色谱法common-pressure liquid chromatography超声波脱气ultrasonic degas程序变流色谱法programmed flow (gas) chromatography程序升温进样programmed temperature sampling程序升温色谱法programmed temperature (gas) chromatography 程序升温蒸发器programmed temperature vaporizer ,PTV程序升压programmed pressure大孔树脂macro-reticular resin大孔填料macro-reticular packing material大内径毛细管柱Megaobore column。
超敏感的ZnO镀覆传感器在室温下对甲醛的影响

超敏感的ZnO镀覆传感器在室温下对甲醛的影响何一聪;罗浩;朱振【摘要】在本工作中,用介孔结构的甲醛传感器在室温下检测了甲醛(十亿分之几)浓度.通过 X-射线衍射(XRD)、Brunauer Emmett Teller(BET)和扫描电子显微镜(SEM)检测介孔氧化锌的形态和结构.测试了甲醛、甲醇、乙醇、丙酮和丙酮的传感特性.气体传感研究表明,介孔氧化锌在室温下对浓度为0.037 mg/m3的甲醛具有极好的敏感性.因此,介孔氧化锌在甲醛传感器领域有着广阔的应用前景.%In the present work, the formaldehyde sensor with mesoporous structure has been used to detect formaldehyde(parts-per-billion(ppb) concentrations) at room temperature. The morphology and structure of mesoporous ZnO were characterized by X-ray diffraction(XRD),Brunauer-Emmett-Teller(BET) and scanning electron microscopy (SEM). The sensing characteristics of formaldehyde, methanol, ethanol, hydrogen and acetone were examined. Gas sensing study revealed that mesoporous ZnO exhibited excellent sensitivity to formaldehyde at concentration as low as 0.037 mg/m3at room temperature. Therefore,mesoporous ZnO was a promising application in the field of formaldehyde sensor.【期刊名称】《广州化工》【年(卷),期】2018(046)005【总页数】3页(P61-63)【关键词】介孔氧化锌;甲醛;室温;气体传感器【作者】何一聪;罗浩;朱振【作者单位】天津理工大学环境科学与安全工程学院,天津 300384;天津理工大学环境科学与安全工程学院,天津 300384;天津理工大学环境科学与安全工程学院,天津 300384【正文语种】中文【中图分类】X851甲醛会造成鼻腔肿瘤、眼睛刺激、恶心、头痛、疲劳、迟钝、口渴、中枢神经损伤,免疫系统紊乱等症状[1]。
- 1、下载文档前请自行甄别文档内容的完整性,平台不提供额外的编辑、内容补充、找答案等附加服务。
- 2、"仅部分预览"的文档,不可在线预览部分如存在完整性等问题,可反馈申请退款(可完整预览的文档不适用该条件!)。
- 3、如文档侵犯您的权益,请联系客服反馈,我们会尽快为您处理(人工客服工作时间:9:00-18:30)。
Applied Surface Science 261 (2012) 890–895Contents lists available at SciVerse ScienceDirectApplied SurfaceSciencej o u r n a l h o m e p a g e :w w w.e l s e v i e r.c o m /l o c a t e /a p s u scEnhanced ethanol sensing properties of Zn-doped SnO 2porous hollow microspheresWenchuang Wang,Yongtao Tian ∗,Xinjian Li,Xinchang Wang,Hao He,Yurui Xu,Chuan HeDepartment of Physics and Laboratory of Material Physics,Zhengzhou University,Zhengzhou 450052,PR Chinaa r t i c l ei n f oArticle history:Received 28February 2012Received in revised form 7August 2012Accepted 29August 2012Available online 4 September 2012Keywords:Zn-doped SnO 2Hollow microspheres C 2H 5OH Gas sensora b s t r a c tZn-doped SnO 2porous hollow microspheres with an average diameter of ∼180nm have been prepared by a direct precipitation method using colloidal carbon sphere as template.The XRD data disclosed that the structure of the Zn-doped SnO 2microspheres was the same as pure SnO 2,while the crystallite size of Zn-doped SnO 2microspheres (10.63nm)was smaller than SnO 2(23.2nm).The sensing measurement showed that the response (R a /R g )increased near linearly with the ethanol gas concentration at the oper-ating temperature of 240◦pared with SnO 2microspheres,Zn-doped SnO 2porous hollow spheres exhibited a significant improvement for the response towards ethanol at 240◦C.The response of Zn-doped SnO 2microspheres was up to 3when the sensor was exposed to 2ppm C 2H 5OH,with the response and recovery times of 7and 4s,respectively.Additionally,the response of Zn-doped SnO 2sensor showed slight variation after 15weeks storage.The results indicated that Zn-doped SnO 2microspheres are of great potential for fabricating C 2H 5OH sensors with high performance.© 2012 Elsevier B.V. All rights reserved.1.IntroductionGas sensor,as one kind of chemical sensors,has got considerable attention driven by its practical applications in air quality monitor-ing and controlling,flammable or toxic gases detection,medical diagnosis,and combustion efficiency optimization [1–4].Based on their high response value,fast response,quick recovery,excellent stability and easy in preparation,metal-oxide semiconductors have been widely chosen as the sensing materials for gas sensors over the last decades [5–9].Among various metal-oxide nanostructures,such as nanowires,nanotubes,porous membrane,nanorods and hollow spheres,porous material has been paid large attention and extensively studied as gas sensing material [10–12].Gas sensor with a porous structure could contribute to improve sensing per-formances because of large active surface area and efficient gas diffusion induced by this unique structure [6,13,14].Tin dioxide (SnO 2)is a typical wide band gap n-type semicon-ductor (3.6eV)with rutile-type crystal structure.Because of its unique optical,catalytic,and electrical properties,SnO 2has been widely investigated,for various potential applications such as sen-sors [15],solar cells [16],lithium batteries [17],catalysis [18]and transparent conductive electrodes [19].In particular,it has been the predominant sensing material since the 1960s,due to its low cost,excellent stability and high response,which ensure superior∗Corresponding author.Fax:+863716776662.E-mail address:tianytao@ (Y.Tian).performance of these devices.Recently,many scientific and tech-nological efforts have been focused on improving the sensing performance of SnO 2gas sensors,such as adding catalysts,doping metals and metal oxides,decreasing grain size,controlling pore and surface defects,etc.Among these methods,doping metals or metal oxides to SnO 2have been proved to be a simple and effi-cient route to enhance the sensing properties.Yu et al.prepared ZnO and CuO-doped SnO 2with high selectivity to CO gas [20].Kol-makov et al.synthesized Pd catalyst particles functionalized SnO 2nanowires and nanobelts with a dramatic improvement in response toward oxygen and hydrogen [21].However,few of reports about Zn-doped SnO 2porous hollow microspheres for gas detection have been found.Here,we synthesized Zn-doped SnO 2porous hollow micro-spheres by using colloidal carbon spheres as template.The as prepared samples were used for the ethanol gas detection.Results indicated that Zn-doped SnO 2microsphere sensor had a better ethanol gas response than pure SnO 2microsphere sensor.2.Experimental2.1.Preparation of colloidal carbon spheresColloidal carbon spheres were fabricated by the hydrothermal approach as reported previously [13].6g glucose was dissolved in 40mL of distilled water under stirring.The aqueous solution was then transferred into a 50mL Teflon-lined stainless steel autoclave and maintained at 180◦C for 8h.The black or puce precipitates0169-4332/$–see front matter © 2012 Elsevier B.V. All rights reserved./10.1016/j.apsusc.2012.08.118W.Wang et al./Applied Surface Science261 (2012) 890–895891Fig.1.(a)The schematic of HW-30A gas sensing test system,(b)the image of prepared sensor,(c)the equivalent circuit of the gas sensing test system.were isolated by centrifugation,washed by three cycles of centrifu-gation/washing/redispersion in water and alcohol alternately,and then oven-dried at80◦C for8h.2.2.Synthesis of Zn-doped SnO2hollow microspheres0.1125mmol of Zn(AC)2and3.6375mmol of SnCl2·2H2O were dissolved in a mixed solvent of60mL of ethanol and8mL of water under stirring,followed by dissolution of30mmol of urea to form a clear solution.Then the as-prepared carbon spheres(30mmol) were added and well dispersed into the above solution with the assistance of sonication for1h.After aged for24h,the mixture was isolated and dried at80◦C for12h.Finally,the sample was calcined in air at550◦C for2h.For comparison,the ZnO and SnO2 hollow microspheres were prepared by3.73mmol of Zn(AC)2and SnCl2·2H2O,respectively,using the same method.2.3.CharacterizationThe phase characterization of the products was done by X-ray powder diffraction(XRD,Philips X)with Cu K␣as the radiation source and operated at40kV and30mA.The Brunauer–Emmett–Teller(BET)surface area of the powders was analyzed by nitrogen physisorption at77K on a Quantachrome NOVA1000e.The morphology and chemical composition of the samples were inspected using afield emission scanning electron microscope(FESEM,JEOL F6700)equipped with an energy-dispersive X-ray spectrum(EDX).Transmission electron microscopy(TEM)images and selected area electron diffraction (SAED)patterns were obtained using TEM,JEOL JEM2100.2.4.Measurement of gas-sensingAs-prepared samples were dispersed into an appropriate amount of ethanol under ultrasonication.Then,the formed sus-pension was coated onto the surface of the alumina ceramic tube and dried in air.There are two Pt wires on each end of alumina tube,and a Ni–Cr alloy coil going through the tube employed as a heatingfilament to control the operating temperature by tuning the heating voltage.The gas sensing measurements were taken on a commercial HW-30A system(Han Wei Electronics Co.,Ltd.,Henan province,China)at a relative humidity of25–33%.HW-30A is a static system using atmospheric air as the interference gas.Test gases with calculated amount are introduced into the test chamber by a microsyringe.Two electric fans installed in the chamber are used to make test gas homogeneous.After test,the chamber was removed for test gases to diffuse away.The schematic and working principle are shown in Fig.1.The gas response is defined as S=R a/R g,where R a and R g are the resistance of the sensor in air and in the target gas,respectively.The time taken by the sensor to achieve90% of the total resistance change is defined as the response time in the case of adsorption or the recovery time in the case of desorption.3.Results and discussionFig.2shows the powder X-ray diffraction(XRD)patterns of SnO2 with and without Zn doping.All of them can be well indexed as tetragonal SnO2with a rutile structure(JCPDS Card No41-1445) and no diffraction peak of ZnO appeared in the XRD pattern(For Sn4+and Zn2+,the ion radius and covalent radius are0.071nm, 0.141nm and0.074nm,0.125nm respectively.The differences are too small to effect the crystal structure when Zn2+replacement Sn4+).The crystallite size of Zn-doped SnO2was calculated to be 10.63nm according to Debye–Scherrer formula,which is smaller than that of SnO2(23.2nm).The specific surface areas of SnO2 and Zn-doped SnO2microspheres,determined by a multipoint BET method using the adsorption data in the relative pressure(P/P0) range of0.05–0.3(Fig.S1),were13.826,and25.963m2/g,respec-tively.Result indicated the doping of Zn decreased the crystallite size,which lead to the increase of the specific surface area.The typical SEM and TEM images of colloidal carbon micro-spheres with diameters of250nm are shown in Fig.3.The colloidal carbon spheres fabricated by hydrothermal approach were hydrophilic with plenty of OH and C O groups on the surface [13].These functional groups enabled the carbon spheres to bind metal cations.The carbon spheres loaded with metal cationsgave Fig.2.The XRD patterns of pure and Zn-doped SnO2microspheres.892W.Wang et al./Applied Surface Science 261 (2012) 890–895Fig.3.(a)SEM and (b)TEM images of colloidal carbon microspheres.rise to hollow metal oxide spheres after the carbon cores sacrificed in the form of CO 2during calcination.The as-prepared Zn-doped SnO 2were exhibited in Fig.4(a),indicating that the morphology of Zn-doped SnO 2is porous hol-low spheres with diameter of ∼180nm.The size of Zn-doped SnO 2sphere is smaller than that of colloidal carbon spheres (∼250nm),suggesting that the Zn-doped SnO 2spheres could collapse slightly and contract in calcinations process [22].Details of shells can be seen in Fig.4(b),revealing that the shells are porous and con-sist of abundant particles.The HRTEM image of thenanoparticlesFig.4.(a)SEM image (the inset is a single Zn-doped SnO 2microsphere),(b)TEM image,(c)HRTEM image and its corresponding SAED (the inset in c)and (d)EDS spectra of Zn-doped SnO 2microspheres.W.Wang et al./Applied Surface Science 261 (2012) 890–895893Fig.5.The transient response of Zn-doped SnO 2sensor to 100ppm C 2H 5OH at various temperatures.in Fig.4(c)shows lattice fringe with spacing of 0.264nm and 0.335nm,which agree well with the (101)and (110)lattice plane of the rutile structure of SnO 2,respectively.Selected area elec-tron diffraction (SAED)patterns from the sample exhibit several bright concentric rings,as shown in inset of Fig.4(c),suggesting that the composite is polycrystalline.The analysis results from EDX (Fig.4(d))shows that the as-fabricated sample are composed of Zn,Sn and O elements with atomic ratio (Zn:Sn:O)of 1.21:37.81:60.99without other impurities,implying that Zn element has been doped into SnO 2.In order to find the optimum operating condition of our samples,the sensors were exposed to 100ppm C 2H 5OH at various operating temperatures (Fig.5).The response was found to increase with the operating temperature,which reached the summit at the operat-ing temperature of 240◦C,and then decrease as the temperature increase.Thus 240◦C can be defined as the optimum operating temperature and applied in all the investigations hereinafter.The transient response of samples (ZnO,SnO 2and Zn-doped SnO 2)at various C 2H 5OH concentrations was shown in Fig.6.It was clear that the response–recovery curves descend or ascend sharply with C 2H 5OH gas in or out.For Zn-doped SnO 2sensor,the fastest response and recovery time were 7s and 4s to 2ppm C 2H 5OH,respectively (The lowest response and recovery time were 20sandFig.6.The transient response of samples (ZnO,SnO 2and Zn-doped SnO 2)at various C 2H 5OH concentrations.22s to 500ppm C 2H 5OH,respectively).Such a rapid response was based on the structures of as-prepared microspheres.The large spe-cific surface of the microspheres made the absorption of C 2H 5OH molecules on the surface of the material easy.The porous struc-tures of the microspheres can facilitate fast mass transfer of the C 2H 5OH molecules to and from the interaction region as well as improve the rate for charge carriers to traverse the barriers.Those advantages lead to significant gain in the fast response/recovery of the as-prepared sensors.The similar results have been widely reported [6,10,14,23].Compared with pure ZnO and SnO 2,the Zn-doped SnO 2microspheres exhibited the highest response,with the value of 100–500ppm C 2H 5OH at the temperature of 240◦C.The response value of ZnO and SnO 2were 27and 61,respectively.Gas sensors based on the ZnO–SnO 2have also been reported.Kim et al.reported the ZnO–SnO 2thin film senor with the response value of 4.69to 100ppm ethanol [24].Song et al.reported the sensor based on two kinds of ZnO–SnO 2nanofibers with the response value of 88and 60–500ppm ethanol,respectively [25,26].Results indicated that the doping of Zn is beneficial to improve the response of the ethanol gas sensor based on SnO 2microspheres.Gas sensors respond to the change of the carrier concentrations,which is usually induced by chemical interaction of gas molecules with the surface of the sensing materials [27].The gas sensing mechanisms are not fully understood and subject to ongoing dis-cussion [28].According to a standard model [7],when Zn-doped SnO 2sensors are exposed to air,the surface of the microspheres will adsorb some oxygen molecules.The adsorbed oxygen will cap-ture electrons from the conductance band of the spheres to become oxygenions (O 2−and O 22−).So the resistance of microspheres in air ambient is higher than that in vacuum.While the microsphere Zn-doped SnO 2sensors are exposed to ethanol gas,ethanol molecules will react with oxygenions on the surface of the spheres.This can be simply described as2C 2H 5OH +O 2−=2CH 3CHO +2H 2O +e(1)The electrons released from the surface reaction transfer back into the conductance band,which increase the conductivity of the Zn-doped SnO 2microspheres.The high response of Zn-doped SnO 2can be explained by the specific surface area and the oxygen vacancies.With the doping of Zn,the grain size decreased leading to a higher specific area.The-oretically,the oxygen vacancies could also be formed when Zn 2+cations substitute Sn 4+cations.It is well known a large specific surface area facilitates the chemisorptions process by increasing the adsorption rates,which induced a large change in the electrical conductivity and eventually a high sensor response [29].In addi-tion,the oxygen vacancies can adsorb more oxygen molecules in the ambient atmosphere without lowering the expansion level of depletion layer on the surface of microspheres,resulting in a fur-ther increase of the sensor response [30].Therefore,the doping of Zn increased the SnO 2microsphere sensor response.The dependence of response on C 2H 5OH concentration at 240◦C was measured and shown in Fig.7.In the detecting concentration range (from 2ppm to 500ppm),the increase in response depended near linearly on the concentration.In fact,the response of the semi-conducting oxide gas sensitive sensor can usually be empirically represented as:S g =A P g N ,where P g is the target gas partial pres-sure,which is indirect proportion to its concentration,and the sensitivity is characterized by the prefactor A and exponent N .N may have some rational fraction value (usually 1or 1/2),depend-ing on the charge of the surface species and the stoichiometry of the elementary reactions on the surface [31].For the Zn-doped SnO 2microspheres,N is 1.The sensitivity of the sensor to per ppm ethanol is 0.2027and the linear related coefficient (r )is 0.988.Such good linearity between the sensitivity and gas concentration894W.Wang et al./Applied Surface Science 261 (2012) 890–895Fig.7.The response of Zn-doped SnO 2microspheres sensor to various concentra-tions of C 2H 5OH at 240◦C.Fig.8.The transient response of Zn-doped SnO 2microspheres sensor to 100ppm of C 2H 5OH at 240◦C after storage for various time periods.provides potential application in quantitative measurement of C 2H 5OH concentration.Additionally,stability is also one of the most important char-acteristics for sensors.The transient response of Zn-doped SnO 2microspheres sensor to 100ppm of C 2H 5OH at 240◦C after stor-ing for 0,2,5,10,15weeks are shown in Fig.8.It was found that very small variations were detected in the response of the sensor,showing that the sensor exhibited good long-term stability after the initial storage duration.4.ConclusionIn summary,Zn-doped SnO 2was obtained through direct precipitation method by using carbon sphere as template and investigated as the C 2H 5OH sensing materials.The results demon-strate that Zn-doped SnO 2microspheres have excellent potential applications for fabrication high performance C 2H 5OH sensors.This method can work as a feasible solution to improve the response of SnO 2-based sensors and accelerate the commercialization of metal oxide nanodevices.AcknowledgmentsThis work was supported by the National Natural Science Foun-dation of China (grant no.10574112and 50602040)and the Science and Technology Project on Key Problems of Henan Province (grant no.082101510007).Appendix A.Supplementary dataSupplementary data associated with this article can be found,in the online version,at /10.1016/j.apsusc.2012.08.118.References[1]M.E.Franke,T.J.Koplin,U.Simon,Metal and metal oxide nanoparticles inchemiresistors:does the nanoscale matter,Small 2(2006)36–50.[2]N.Yamazoe,Toward innovations of gas sensor technology,Sensors and Actua-tors B 108(2005)2–14.[3]S.Neethirajan,D.S.Jayas,S.Sadistap,Carbon dioxide (CO 2)sensors for the agri-food,Food and Bioprocess Technology 2(2009)115–121.[4]D.Kohl,Function and applications of gas sensors,Journal of Physics D:AppliedPhysics 34(2001)125–149.[5]N.Al-Hardan,M.J.Abdullah,A.Abdul Aziz,H.Ahmad,Low operating tempera-ture of oxygen gas sensor based on undoped and Cr-doped ZnO films,Applied Surface Science 256(2010)3468–3471.[6]M.Tiemann,Porous metal oxides as gas sensors,Chemistry–A European Journal13(2007)8376–8388.[7]N.Barsan,U.Weimar,Conduction model of metal oxide gas sensors,Journal ofElectroceramics 7(2001)143–167.[8]J.Janata,M.Josowicz,D.M.DeVaney,Chemical sensors,Analytical Chemistry66(1994)207–228.[9]N.Al-Hardan,M.J.Abdullah,A.Abdul Aziz,Impedance spectroscopy of undopedand Cr-doped ZnO gas sensors under different oxygen concentrations,Applied Surface Science 257(2011)8993–8997.[10]J.Liu,Z.Guo,F.Meng,T.Luo,M.Li,J.Liu,Novel porous single-crystallineZnO nanosheets fabricated by annealing ZnS (en)0.5(en =ethylenediamine)precursor.Application in a gas sensor for indoor air contaminant detection,Nanotechnology 20(2009)125501–125508.[11]J.Zeng,M.Hu,W.Wang,H.Chen,Y.Qin,NO 2-sensing properties of porousWO 3gas sensor based on anodized sputtered tungsten thin film,Sensors and Actuators B 161(2012)447–452.[12]A.Ramizy,Z.Hassan,K.Omar,Porous GaN on Si (111)and its application tohydrogen gas sensor,Sensors and Actuators B 155(2011)699–708.[13]X.M.Sun,J.F.Liu,Y.D.Li,Use of carbonaceous polysaccharide microspheres astemplates for fabricating metal oxide hollow spheres,Chemistry–A European Journal 12(2006)2039–2047.[14]T.Hyodo,K.Sasahara,Y.Shimizu,M.Egashira,Preparation of macroporousSnO 2films using PMMA microspheres and their sensing properties to NO x and H 2,Sensors and Actuators B 106(2005)580–590.[15]Q.Kuang,o,Z.L.Wang,Z.X.Xie,L.S.Zheng,High-sensitivity humiditysensor based on a single SnO 2nanowire,Journal of the American Chemical Society 129(2007)6070–6071.[16]E.Ramasamy,J.Lee,Ordered mesoporous SnO 2based photoanodes for high-performance dye-sensitized solar cells,Journal of Physical Chemistry C 114(2010)22032–22037.[17]X.W.Lou,Y.Wang,C.L.Yuan,J.Y.Lee,L.A.Archer,Template-free synthesisof SnO 2hollow nanostructures with high lithium storage capacity,Advanced Materials 18(2006)2325–2329.[18]Y.L.Du,N.Zhang,C.M.Wang,Photo-catalytic degradation of trifluralin by SnO 2-doped Cu 2O crystals,Catalysis Communications 11(2010)670–674.[19]P.Veluchamy,M.Tsuji,T.Nishio,et al.,A pyrosol process to deposit large-areaSnO 2:F thin films and its use as a transparent conducting substrate for CdTe solar cells,Solar Energy Materials and Solar Cells 67(2001)179–185.[20]J.H.Yu,G.M.Choi,Selective CO gas detection of CuO and ZnO-doped SnO 2gassensor,Sensors and Actuators B 75(2001)56–61.[21]A.Kolmakov,D.O.Klenov,Y.Lilach,S.Stemmer,M.Moskovits,Enhanced gassensing by individual SnO 2nanowires and nanobelts functionalized with Pd catalyst particles,Nano Letters 5(2005)667–673.[22]G.Jia,M.Yang,Y.H.Song,H.P.You,H.J.Zhang,General and facile method toprepare uniform Y 2O 3:Eu hollow microspheres,Crystal Growth and Design 9(2009)301–307.[23]J.Zhang,S.Wang,Y.Wang,Y.Wang,B.Zhu,H.Xia,X.Guo,S.Zhang,W.Huang,S.Wu,NO 2sensing performance of SnO 2hollow sphere sensor,Sensors and Actuators B 135(2009)610–617.[24]K.Kim,P.Cho,S.Kim,J.Lee,C.Kang,J.Kim,S.Yoon,The selective detectionof C 2H 5OH using SnO 2–ZnO thin film gas sensors prepared by combinatorial solution desposition,Sensors and Actuators B 123(2007)318–324.[25]X.Song,Z.Wang,Y.Liu, C.Wang,L.Li,A highly sensitive ethanol sen-sor based on mesoporous ZnO–SnO 2nanofibers,Nanotechnology 20(2009)075501–075505.W.Wang et al./Applied Surface Science261 (2012) 890–895895[26]X.Song,L.Liu,Characterization of electrospun ZnO–SnO2nanofibers for ethanolsensor,Sensors and Actuators A154(2009)175–179.[27]T.Seiyama,A.Kato,K.Fujiishi,M.Nagatani,A new detector for gaseouscomponents using semiconductive thinfilms,Analytical Chemistry34(1962) 1502–1503.[28]A.Gurlo,Interplay between O2and SnO2:oxygen ionosorption and spec-troscopic evidence for adsorbed oxygen,ChemPhysChem7(2006)2041–2052.[29]J.Q.Xu,Y.Q.Pan,Y.A.Shun,Z.Z.Tian,Grain size control and gas sensing prop-erties of ZnO gas sensor,Sensors and Actuators B66(2000)277–279.[30]L.Liao,H.B.Lu,J.C.Li,H.He,D.F.Wang,D.J.Fu,C.Liu,Size dependence ofgas sensitivity of ZnO nanorods,Journal of Physical Chemistry C111(2007) 1900–2190.[31]Q.Wan,Q.H.Li,Y.J.Chen,T.H.Wang,X.L.He,J.P.Li,C.L.Lin,Fabrication andethanol sensing characteristics of ZnO nanowire gas sensors,Applied Physics Letters84(2004)3654–3656.。