HTPB推进剂裂纹起裂J积分研究
HTPB推进剂三点弯曲过程试验与数值模拟
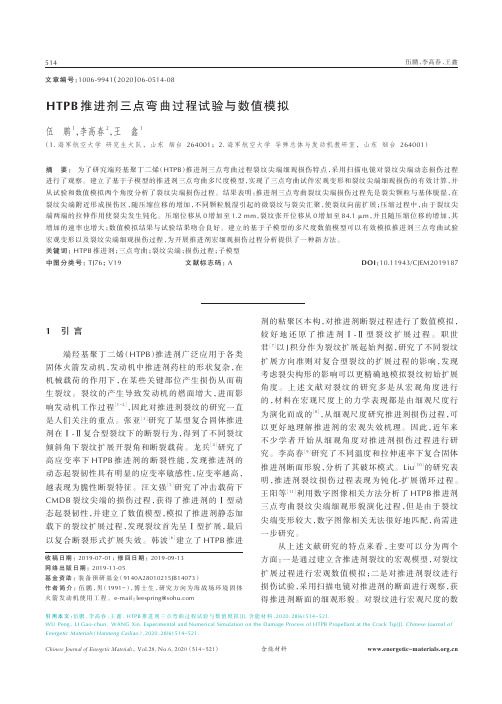
HTPB 推进剂三点弯曲过程试验与数值模拟伍鹏1,李高春2,王鑫1(1.海军航空大学研究生大队,山东烟台264001;2.海军航空大学导弹总体与发动机教研室,山东烟台264001)摘要:为了研究端羟基聚丁二烯(HTPB )推进剂三点弯曲过程裂纹尖端细观损伤特点,采用扫描电镜对裂纹尖端动态损伤过程进行了观察。
建立了基于子模型的推进剂三点弯曲多尺度模型,实现了三点弯曲试件宏观变形和裂纹尖端细观损伤的有效计算,并从试验和数值模拟两个角度分析了裂纹尖端损伤过程。
结果表明:推进剂三点弯曲裂纹尖端损伤过程先是裂尖颗粒与基体脱湿,在裂纹尖端附近形成损伤区,随压缩位移的增加,不同颗粒脱湿引起的微裂纹与裂尖汇聚,使裂纹向前扩展;压缩过程中,由于裂纹尖端两端的拉伸作用使裂尖发生钝化。
压缩位移从0增加至1.2mm ,裂纹张开位移从0增加至84.1μm ,并且随压缩位移的增加,其增加的速率也增大;数值模拟结果与试验结果吻合良好。
建立的基于子模型的多尺度数值模型可以有效模拟推进剂三点弯曲试验宏观变形以及裂纹尖端细观损伤过程,为开展推进剂宏细观损伤过程分析提供了一种新方法。
关键词:HTPB 推进剂;三点弯曲;裂纹尖端;损伤过程;子模型中图分类号:TJ76;V19文献标志码:ADOI :10.11943/CJEM20191871引言端羟基聚丁二烯(HTPB )推进剂广泛应用于各类固体火箭发动机,发动机中推进剂药柱的形状复杂,在机械载荷的作用下,在某些关键部位产生损伤从而萌生裂纹。
裂纹的产生导致发动机的燃面增大,进而影响发动机工作过程[1-2],因此对推进剂裂纹的研究一直是人们关注的重点。
张亚[3]研究了某型复合固体推进剂在Ⅰ‐Ⅱ复合型裂纹下的断裂行为,得到了不同裂纹倾斜角下裂纹扩展开裂角和断裂载荷。
龙兵[4]研究了高应变率下HTPB 推进剂的断裂性能,发现推进剂的动态起裂韧性具有明显的应变率敏感性,应变率越高,越表现为脆性断裂特征。
含TKX-50的HTPB推进剂能量性能研究
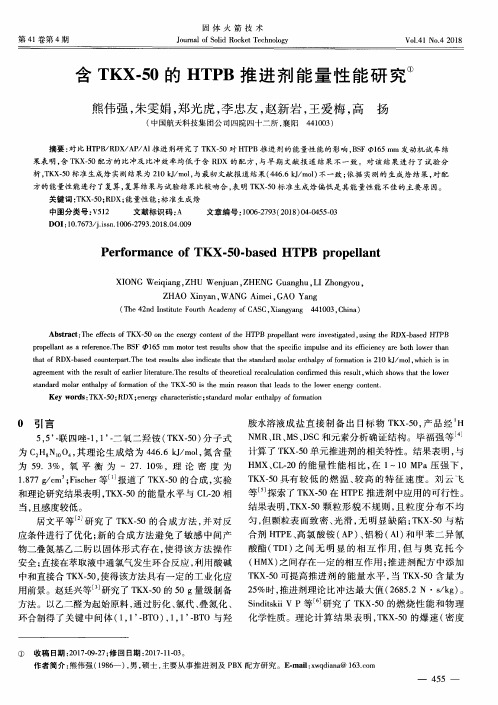
为 c H8N1。O ,其理论生成焓为 446.6 kJ/mol,氮含量 计算了 TKX一50单元推进剂的相关特性。结果表明 ,与
为 59.3% ,氧 平 衡 为 一27.10% ,理 论 密 度 为 HMX、CL.20的 能 量 性 能 相 比 ,在 1~10 MPa压 强 下 ,
1.877 g/cm ;Fischer等 … 报道 了 TKX。50的合 成 ,实 验 TKX一50具 有 较 低 的 燃 温 、较 高 的 特 征 速 度 。刘 云 飞
关 键 词 :TKX一50;RDX;能 量 性 能 ;标 准 生成 焓 中图分类号 :V512 文献标识码 :A 文章编 号 :1006—2793(2018)04—0455—03
DoI:10.7673/i.issn.1006—2793.2018.04.009
Perform ance of TK X--50--based HTPB propellant
XIONG Weiqiang,ZHU Wenjuan,ZHENG Guanghu,LI Zhongyou,
ZHAO Xinyan,W ANG Aimei,GAO Yang (The 42nd Institute Fouah Academy of CASC,Xiangyang 441003,China)
应 条件 进行 了优 化 ;新 的合 成 方 法避 免 了敏 感 中间 产 合 剂 HTPE、高氯 酸 铵 (AP)、铝 粉 (A1)和 甲苯 二 异 氰
物二叠 氮基 乙二 肟 以 固体 形 式存 在 ,使 得 该 方 法 操 作 酸酯 (TDI)之 间 无 明 显 的 相 互 作 用 ,但 与 奥 克 托 今
Abstract:The effects of TKX一50 on the energy content of the H rPB propellant were investigated.using the RDX —based HTPB propellant as a reference.The BSF 165 mm motor test results show that the specif ic impulse and its efficiency are both lower than that of RDX—based counterpart.The test results alSO indicate that the standard molar enthalpy of formation iS 210 kJ/mo1.which is in agreem ent with the result of earlier literature.The results of theoretical recalculation conf irmed this result.which shows that the lower standard molar enthalpy of form ation of the TKX一50 iS the main reason that leads to the lower energy content.
基于分段曲线拟合的HTPB固体推进剂损伤数值模拟
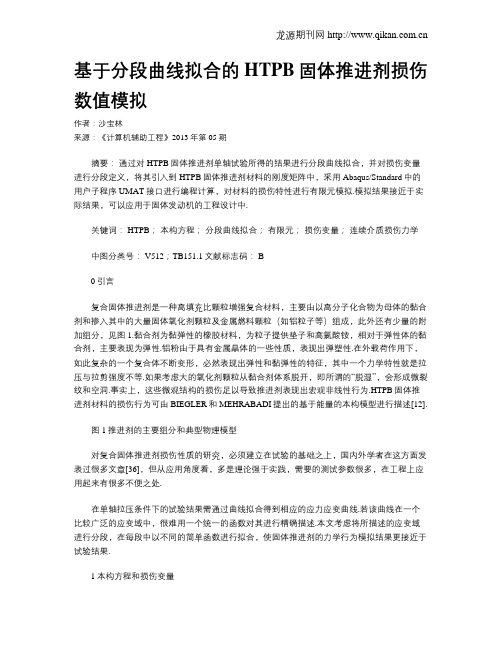
基于分段曲线拟合的HTPB固体推进剂损伤数值模拟作者:沙宝林来源:《计算机辅助工程》2013年第05期摘要:通过对HTPB固体推进剂单轴试验所得的结果进行分段曲线拟合,并对损伤变量进行分段定义,将其引入到HTPB固体推进剂材料的刚度矩阵中,采用Abaqus/Standard中的用户子程序UMAT接口进行编程计算,对材料的损伤特性进行有限元模拟.模拟结果接近于实际结果,可以应用于固体发动机的工程设计中.关键词: HTPB;本构方程;分段曲线拟合;有限元;损伤变量;连续介质损伤力学中图分类号: V512;TB151.1文献标志码: B0引言复合固体推进剂是一种高填充比颗粒增强复合材料,主要由以高分子化合物为母体的黏合剂和掺入其中的大量固体氧化剂颗粒及金属燃料颗粒(如铝粒子等)组成,此外还有少量的附加组分,见图1.黏合剂为黏弹性的橡胶材料,为粒子提供垫子和高氯酸铵,相对于弹性体的黏合剂,主要表现为弹性.铝粉由于具有金属晶体的一些性质,表现出弹塑性.在外载荷作用下,如此复杂的一个复合体不断变形,必然表现出弹性和黏弹性的特征,其中一个力学特性就是拉压与拉剪强度不等.如果考虑大的氧化剂颗粒从黏合剂体系脱开,即所谓的“脱湿”,会形成微裂纹和空洞.事实上,这些微观结构的损伤足以导致推进剂表现出宏观非线性行为.HTPB固体推进剂材料的损伤行为可由BIEGLER和MEHRABADI提出的基于能量的本构模型进行描述[12].图 1推进剂的主要组分和典型物理模型对复合固体推进剂损伤性质的研究,必须建立在试验的基础之上,国内外学者在这方面发表过很多文章[36],但从应用角度看,多是理论强于实践,需要的测试参数很多,在工程上应用起来有很多不便之处.在单轴拉压条件下的试验结果需通过曲线拟合得到相应的应力应变曲线.若该曲线在一个比较广泛的应变域中,很难用一个统一的函数对其进行精确描述.本文考虑将所描述的应变域进行分段,在每段中以不同的简单函数进行拟合,使固体推进剂的力学行为模拟结果更接近于试验结果.1本构方程和损伤变量1.1本构方程描述根据MEHRABADI和COWIN的表述:(1)对于任意弹性对称体,全应力张量和全应变张量可以分解为6个或少于6个的等同形式的特征张量之和;(2)对于任意弹性对称体,每个特征应力张量直接与其等同形式的特征应变张量成正比;(3)总应变能可分解为6项或少于6项之和,每项都是等同形式的特征应力与特征应变的标量积,表示6个互不作用的能量模态.所建立材料模型只适用于平面应力情况.在没有损伤影响下,材料在单向应力状态下应力与应变之间的本构关系是线性的.对于正交各向异性的复合材料,应力与应变之间的弹性本构关系为T=C:E(1)式中:C为2阶刚度张量,其矩阵形式可写为与其他各向异性材料相同,HTPB推进剂表现为拉压和剪切性质的不同,因此式(2)是假定材料为正交各向异性后所得出的沿2个不同方向的初始值.E11和E22可考虑在2个正交方向上取拉伸和压缩试验的平均值,υ12和υ21同样可以通过试验确定.根据文献,要描述复合材料的正交各向异性,应该分解应力张量和应变张量.在平面应力状态下,全应力和全应变分解成对应其特征值的3个特征张量.由上述第二个性质可知,第i个特征值λi,特征应力与特征应变之间的关系可写为Tci=λiEci(3)由第三个性质,可得2ρ=3iTciEci(4)式中:ρ为总应变能密度.对于这些非相互作用的能量模态,可用于建成立失效准则.1.2损伤变量的定义根据连续介质损伤力学可以假定,复合固体推进剂材料的非线性特性完全是由材料的损伤引起的.当损伤变量D=0时,材料是完全弹性的;当D=1时,材料完全损伤.对于线弹性材料,可以证明按材料刚度的改变定义损伤变量与连续介质损伤力学中按横截面损伤的比值定义损伤变量是等价的,因此若损伤之前材料的刚度为C0,损伤后的刚度为C(02应力应变曲线的分段拟合通过对复合固体推进剂材料的试样进行拉伸、剪切或者压缩试验,可以得到固体推进剂材料的力学性质.若能对应力应变试验值进行较简单的拟合,得到一个统一表达的函数,则可以使上述编程过程变得相对简单.但是,在一个较大的应变域中,可能很难找到一个能够描述试验形态的统一函数.因此,可以考虑用分段拟合的方法得到相应的应力应变关系曲线.设第r(r=1,2,…)组试验数据,取线性无关组φ(i)ij(ε)=εi-1ri=1,2,…,m,j=1,2…,n(21)由m次最小二乘拟合,可得多项式σr(ε)=mk=0Crkφrk(ε)=mk=0Crkεk-1r(22)将式(22)代入式(15)中,可以得到在不同区域的范围内,对损伤变量的不同定义为Dr=I-1CroTr(E)E(23)式(23)中需要确定Cro,在增量计算中Cro为分段区域端点上2条拟合曲线所共同具有的切线斜率,因此在分段拟合中,保持2条拟合曲线在端点处满足条件Tr-1(E-0)=Tr(E+0)=k0Tr-1(E)EE=E-0=Tr(E)EE=E+0(24)k0需满足在计算过程中的稳定性要求.将计算所得的Dr代入(21)式,通过修改Abaqus/Standard中UMAT的DDSDDE进行计算.某配方常温下HTPB固体推进剂应力应变曲线见图2.图 2HTPB固体推进剂典型应力应变曲线局部放大后,当ε表 1HTPB推进剂材料性质参数εmaxab数值102.13.2但是,当ε>10%以后,用双曲正切函数并不能很好地拟合试验曲线,因此在此区间,可采用最小二乘法进行拟合,见表2.表 2HTPB固体推进剂的材料性质第二段试验数据应变102555应力/MPa0.410.690.92由表2 拟合出如下函数.σ(ε)=0.187 9ε0.6-0.024 92ε(26)根据式(26)可得出在ε>10%后的损伤变量定义为D2=1-175.40(0.112 7ε-0.6+0.024 92)(27)3有限元模拟和结果讨论推进剂材料使用圆柱型试件,其尺寸为Φ25 mm×50 mm圆柱型裂纹试件,两端采用专用夹具与拉伸机连接.程序开头片段见图3.图 3程序开头片段计算结果均通过von Mises应变εe和应力σe进行分析.试件的整体应力云图见图4;推进剂材料在破坏前的整体应力云图见图5;载荷时间分别为推进剂在1,2.5,7.5和15 s时的剖面应力云图见图6.图 4含夹具试件的整体应力云图图 5推进剂的整体应力云图图 6推进剂在载荷时间分别为1,2.5,7.5和15 s时的剖面应力云图根据应力计算分析的结果,推进剂发生破坏的位置在推进剂材料的中心位置,随着载荷时间的增加,推进剂内部的应力逐渐增大.若要发生破坏,则从推进剂最中心的位置产生,从这个位置扩展,向外层表面延伸,直到试件完全破坏.由图6可知,推进剂表现出明显的非线性黏弹性特征.随着位移的增加,应力呈曲线上升;因为拉伸速率为定值,所以应变为线性发展趋势,这与试验情况一致.材料单轴加载试验表明,在ε4结束语依据试验结果,通过对单轴试验曲线的适当分段拟合,用有限元法进行模拟,可以对HTPB推进剂材料的损伤行为做出较好解释,其模拟结果与试验也有较好的一致性.参考文献:[1]COWIN S C, MEHRABADI M M. The structure of the linear anisotropic elastic symmetries[J]. Mech Phys Solids, 1992, 40(7): 14591471.[2]ARRAMON Y P, MEHRABADI M M, MARTIN D W, et al. A multidimentional anistropic strength criterion based on kelvin modes[J]. Int J Solids & Struct, 2000, 37(21):29152935.[3]沈观林,胡更开. 复合材料力学[M]. 北京:清华大学出版社, 2006: 2944.[4]李灏. 损伤力学基础[M]. 济南:山东科学技术出版社, 1992: 4349.[5]LEMAITRE J. A continuous damage mechanics model for ductile fracture[J]. J Eng Mat & Technol, 1983, 107(1): 8389.[6]LOCKYEAR S A. Mechanical analysis of transversely loaded wood/plastic sections[D]. Pullman: Washington State University, 1999.。
配方对富燃复合推进剂力学性能影响的实验研究
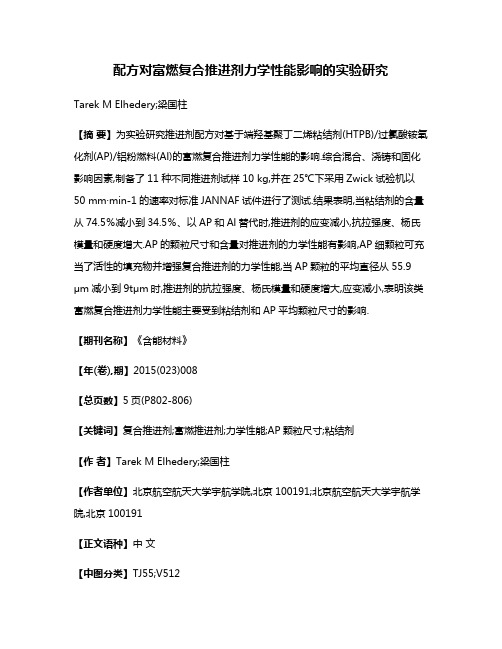
配方对富燃复合推进剂力学性能影响的实验研究Tarek M Elhedery;梁国柱【摘要】为实验研究推进剂配方对基于端羟基聚丁二烯粘结剂(HTPB)/过氯酸铵氧化剂(AP)/铝粉燃料(Al)的富燃复合推进剂力学性能的影响.综合混合、浇铸和固化影响因素,制备了11种不同推进剂试样10 kg,并在25℃下采用Zwick试验机以50 mm·min-1的速率对标准JANNAF试件进行了测试.结果表明,当粘结剂的含量从74.5%减小到34.5%、以AP和Al替代时,推进剂的应变减小,抗拉强度、杨氏模量和硬度增大.AP的颗粒尺寸和含量对推进剂的力学性能有影响,AP细颗粒可充当了活性的填充物并增强复合推进剂的力学性能,当AP颗粒的平均直径从55.9 μm减小到9tμm时,推进剂的抗拉强度、杨氏模量和硬度增大,应变减小,表明该类富燃复合推进剂力学性能主要受到粘结剂和AP平均颗粒尺寸的影响.【期刊名称】《含能材料》【年(卷),期】2015(023)008【总页数】5页(P802-806)【关键词】复合推进剂;富燃推进剂;力学性能;AP颗粒尺寸;粘结剂【作者】Tarek M Elhedery;梁国柱【作者单位】北京航空航天大学宇航学院,北京100191;北京航空航天大学宇航学院,北京100191【正文语种】中文【中图分类】TJ55;V5121 IntroductionFuel-rich propellants are typically used for ramjet-rocket motors[1]. Fuel-rich propellants are classified into metal-loaded compositions with high smoke levels, carbon compositions with moderate smoke levels and hydrocarbon-fueled compositions with low smoke level. The major components of hydrocarbon-fueled compositions are a binder, an oxidizer, and sometimes a hydrocarbon filler[2]. The propellant grain must withstand the stresses and strains imposed on it during handling, ignition, and firing of rocket[3]. The mechanical properties are so essential for the propellant to be measured to ensure its capability for storage, transportation, aging, and operating under the combustion loads[4].For the composite solid rocket propellants, tensile mechanical tests are often applied. With elastic materials, stresses and strains are essentially proportional to each other and independent of time[5]. Temperature variations during storage are found to be the main reason for the propellant strain and stress leading to decreasing quality[6].The variation of performance parameters with variation in formulation cannot yet be presented by mathematical models exactly. Many studies have been reported previously to correlate the individual performance properties with the formulation parameters. Marsh[7] studied the polymer parameters for formulation design and quality control, and a general correlation of mechanical properties. He concluded that there is a correlation of propellant elongation with polymer network parameter,while tensile capacity becomes a useful formulation characteristic. Hill[8] studied the effect of the plasticizer to improve the elastomeric properties of binders and hence the mechanical properties. Nian[9] studied the effect of the functionality group of hydroxyl-terminated polybutadiene pre-polymer (HTPB) on the binder network and its correlation with the mechanical properties. Keizers[10] made studies on ammonium perchlorate AP/HTPB propellants to predict some of the important parameters by developing computer model.According to binder base, composite propellants are characterized by their excellent tensile mechanical properties, strength at maximum in the range of 5-35 kg·cm-2 and strain at maximum in the range of 25%-120% at normal temperature[11].The objective of the present experimental investigation in the paper was to determine the effect of solid fillers, such as oxidizer solid loading and oxidizer particle size distribution, on the mechanical properties of the fuel-rich composite propellant. The mechanical properties (tensile strength, strain, and Young′s modulus) were measured a nd analyzed for different formulations.2 Experimental Method2.1 Propellant formulationsThe formulations studied in this work were formulated on the basis of HTPB as a binder and AP as an oxidizer. Further investigation includes system in which fuel, aluminum (Al), and burning rate accelerator, copper chromite (CC), added at the expense of the binder.The compositions of all tested propellant formulations presented in Table 1. The propellant binder was composed of 85.7% HTPB as a main backbone, 12.3% hexa-methylene di-isocianate (HMDI) as a cross-linking agent and 2.0% methyl aziridinyl phosphine oxide (MAPO) as a bonding agent[12]. All propellant compositions contained 0.5%carbon black (CB) with 10 μm particle size as an opacifier which was found to be ve ry important to apply the black body role[2]. The base line propellant formulation A3 consisted of 45% AP with 9.0 μm particles and the particle size variations were investigated through a bi-modal system with AP (64.0 μm)[13]. The AP average particle size in formulation B1 is 44.5 μm and in formulation B2 is 55.9 μm.Table 1 The fuel-rich formulationsbatchbinder/%AP(9μm)/%AP(64μm)/%Al/%CC/%CB/%A174.525.00.00.00.00.5A264.535.00.00.00.00.5A354.545.00.00.00.00.5B154.530.015.00.00.00.5B254.515.030.00.00.00.5C14 4.545.00.010.00.00.5C234.545.00.020.00.00.5D151.545.00.00.03.00.5D248.545.00.00.06.00.5E141.545.00.010.03.00.5E238.545.00.010.06.00.5Preparation of the propellants for the test program was made by using a heavy-duty mixer (9×10-3 m3 capacity) with weight of 10 kg per formulation to guarantee the slurry homogeneity. Propellant slurry casting was applied in special molds with standard dimensions l5 cm×15 cm×15 cm under vacuum and vibration. The molds are placed in a curing oven at 55 ℃, for a total curing time of 240 h. Curing temperature is selected toaccelerate the cross linking reactions. After curing the molds are cut into sheets with uniform thickness of 1.2 cm.Test specimens were produced according to Joint Army-Navy-NASA-Air Force Propulsion Committee (JANNAF) standard[14].Fig.1 shows the produced specimens which checked for voids (e.g. air bubbles-cracks-porosities-foreign matter) by X-Ray. After that, the accepted specimens are stored at ambient temperature in dry place.Fig.1 X-Ray film for JANNAF specimens2.2 ApparatusesThe Zwick Z050 universal test machine was used in carrying out all the mechanical tests. The machine can do seven different mechanical tests (tensile, compression, bending, peeling, creep, relaxation, and low cycle fatigue tests). The machine is provided with 3 different load cells (2.5 kN,10 kN, and 50 kN) to cover wide ranges of measurements and applications. The machine is supported with a temperature chamber which allow for performing tests under desired temperature.The Shore hardness(A) was measured using the hardness tester Zwick (model 3102) at 25 ℃.2.3 Measurement methodsTensile tests are used to determine a certain number of parameters, as shown in figure 2 that allow for better representation of propellant behavior. These parameters include: Young′s modulus (E),maximum strength (σm),maximum strain (εm) corresponding to maximum strength,break stress,break strain.Young′s modulus is determined from the linear part of the stress- strain curve. JANNAF specimens were tested at tensile rate of 50 mm/min and temperature 25 ℃. The tensile test was carried out for at least five samples for each prepared formulation and then the mean value of the obtained results was recorded.3 Results and Discussion3.1 ResultIn this work the regression line is calculated in the range from begin of Young′s Modulus determination to the end of Young′s Modulus determination (see Fig.2) that indicates the parameters which can be identified from the tensile test.Fig. 2 General tensile behavior of solid propellantThe mechanical properties of the tested propellant formulations were independent on the curative ratio (NCO/OH ratio of HMDI and HTPB) in this program where NCO/OH ratio was 2.0 in all formulations (this high ratio was verified practically by preparation of different binder formulations with ratio from 0.5 to 3 then test them which lead to this value). The results of these mechanical properties with different compositions are given in Table 2.Table 2 Results of mechanical properties at 25 ℃batchbinder/%σm/kg·cm-2εm/%E/kg·cm-2AA174.522.5198.0846.6656.00A264.524.6390.9957.5057.50A354.527.9275.3263.1961.25B154.520.0578.3353.3353.50 B254.515.6485.3044.2450.50C144.531.2270.0167.5264.00C 234.535.0736.0773.5272.25D151.528.9973.2265.2263.00D2 48.530.0170.0966.0163.50E141.532.0148.5468.7766.75E238.533.8237.0071.0568.003.3 Effect of binder content on the mechanical propertiesAccording to Fig.3, Fig.4 and Fig.5 increasing the binder percentage (from 34.5% to 74.5%) in group A, C, D, and E make decreasing of the maximum strength (from 35.07 kg·cm-2 to 22.51 kg·cm2 with a percentage of about 35.8%), Young′s modulus (from 73.52 kg·cm-2 to 46.66 kg·cm-2 with a percentage of about 36.5%) and hardness (A) (from 72 to 56 with a percentage of about 22.2%) respectively. That because of decreasing binder by increasing the fillers percentage leads to increase the filling coefficient in the binder matrix which strengthens it and increasing the maximum strength and hardness. Also increasing the solid loading leads to formation of additional crosslinks between the solid particles and the network chains of the binder matrix.Fig.3 Effect of the binder content on the propellant maximum strengthFig.4 Effect of the binder content on the propellant Young′s modulusFig.5 Effect of the binder content on the propellant Shore hardness(A) Fig. 6 shows the trend of increasing the maximum strain (from 37.07% to98.08% with a percentage of about 164.8%) when increasing the binder percentage (from 34.5% to 74.5%) in group A, C, D, and E. It is observed that although the binder decreased 4% from E2 to C2 and from D2 to C1 but the effect in maximum strain is negligible. It is clear that incorporate 10% of Al instead of 6% of CC has no effect on the maximum strain.It is clear that the maximum strain of the composite propellant samples decreases with decreasing the binder content and increasing the solid loading. This effect may arise from the reduced volume fraction of the binder matrix due to the increasing volume fraction of solid particles. When the sample of composite propellant is subjected to uniaxial tensile stresses, the total deformation is accommodated only by the binder matrix because aluminum and ammonium perchlorate particles are hard and possess very high elastic modulus compared to the binder matrix.Fig.6 Effect of the binder content on the propellant maximum strain Group B has the same binder content as A3 (55%) but with different AP average diameter and this factor has a great effect on the mechanical properties. This will be discussed in the next part separately.3.2 Effect of the oxidizer particle size on the mechanical propertiesFig.7 and Fig.8 present the effect of the oxidizer particle size on the maximum strength and hardness (A) respectively. The larger particle size of AP (9 μm in A3, then 44.5 μm in B1, finally 55.9 μm in B2) without changing the propellant composition, the lower the maximum strength (27.92 kg·cm-2, 20.05 kg·cm-2, 15.64 kg·cm-2) and the lower the hardness (A)(61.25, 53.5, 50.5). This is because the small particle sizes of AP act as active fillers and enhance the mechanical strength of the propellant matrix.Fig.7 Effect of the oxidizer particle size on the propellant maximum strengthFig.8 Effect of the oxidizer particle size on the propellant Shore hardness(A) The polymer system filled with coarse particles starts dewetting at a smaller stress compared to a system containing fine particles. Thus the strength increases with the increasing content of fine particles in the polymer matrix system.According to Fig.9 the same trend is found. Young′s modulus is decreased (63.19 kg·cm-2, 53.33 kg·cm-2, 44.24 kg·cm-2) with increasing the particle size of AP.Fig.9 Effect of the oxidizer particle size on the propellant Young′s modulus Fig. 10 shows the relation between the oxidizer particle size and the maximum strain. The results show that increasing AP particle size within the same binder percentage leads to increase the maximum strain (75.32%, 78.33%, 85.3%) because with increasing the particle size of the oxidizer the apparent volume will be decreased and the distances between the particles will be increased.Fig.10 Effect of the oxidizer particle size on the propellant maximum strainIncreasing volume fraction of fine fillers reduces the elongation capability of the composite propellant. This behavior caused by different dewetting nature of large and small particles. Vacuoles (vacuum-holes) occur between solid particle and binder phase when the propellant sample dewets. If the load on the sample is maintained, vacuoles enlarge and eventually combine with each other. The volume of the propellant increases during tensile testing due to the presence of vacuoles and due to its combine with each other[15]. This means that these vacuoles contribute to the total elongation after dewetting occurs. Formation of vacuoles in the propellants containing a larger quantity of fine AP is less than that in propellants containing coarse particles. Thus, increasing fine AP fraction decreases the maximum strain.4 ConclusionThe mechanical properties are so essential for the propellant to be measured to ensure its capability for storage, transportation, aging, and operating under the combustion loads. In this work the effect of solid loading of the fillers in the fuel-rich composite propellants on the mechanical properties was studied. It is observed that as the solid loading increase and the binder percentage decrease from 74.5% to 34.5% the tensile strength increase from 22.51 kg·cm-2 to 35.07 kg·cm-2 and the strain decrease from 98.08% to 36.07% due to formation of additional crosslinks between the solid particles and the network chains of the binder matrix.The mechanical properties of composite propellants are also stronglyaffected by the particle size of the ammonium perchlorate. With increasing the oxidizer average particle size (9 μm, 44.5 μm and 55.9 μm) the maximum strength decreases (27.92 kg·cm-2, 20.05 kg·cm-2, 15.64 kg·cm-2) and the maximum strain increases (75.32%, 78.33%, 85.30%) because the apparent volume decreased and the distances between the particles increased.Much further work is required in this direction to study the effect of AP particle size variations through tri-model systems on the mechanical properties of the composite propellants.References:[1] Shalom Abraham, Gany Alon. Flammability Limits and Ballistic Properties of Fuel-Rich Propellants [J]. Propellants, Explosives, Pyrotechnics, 1991, 16: 59-64.[2] Davenas Alain. Solid Rocket Propulsion Technology [M]. First English Edition, New Work: Pergamon Press, 1993, Chapter 12.[3] Structural assessment of solid propellant grain [R]. Advisory Group for Aerospace Research and Development, (AGARD). 7 Rue Ancelle, 92200 Neuilly-Sur-Seine, France, Report No. AGARD-AR-350, 1997.[4] Amtower Paul K. Propellant Formulation: US 7011722[P], 2006.[5] GEORGE P Sutton. Rocket Propulsion Elements [M]. Sixth Edition, New York: John Wiley & Sons, Inc., 1992, Chapter 12.[6] N, Rodic V, Jeremic R, et al. Structural Analysis Procedure for a Case Bonded Solid Rocket Propellant Grain [C]//Scientific Technical Review,2 011, 61(1): 3-11.[7] Harold E Marsh Jr. Formulation and Quality Control of Polyurethane Propellants [J]. Journal of Industrial and Engineering Chemistry, 1960, 52(9): 768-771.[8] Hill M E, Coon C L, Ross D L. Structure Property Relationships in Propellant Ingredients [C]∥AIAA Paper 78-119, 16th Aerospace Science Meeting, 1978.[9] Ninan K N, BalagandadharanV P, Katherine K B. Studies on the functionality distribution of htpb and correlation with mechanical properties[J]. Journal of Polymer, 1991, 32(4): 628-635.[10] Keizers H L J , Hordijik AC, Van Vilet LD, et al. Modeling of Composite Propellant Properties[C]∥36th AIAA/ASME/SAE /ASEE Joint Propulsion conference and Exhibit, Huntsville, Alabama, 2000.[11] Timnat Y M. Advanced Chemical Rocket Propulsion [M]. London: Academic Press, 1987.[12] Alain Davenas. Solid Rocket Propulsion Technology [M]. First English Edition, New Work: Pergamon Press, 1993.[13] Makoto Kohga. Burning rate characteristic of AP-based composite propellant using bimodal AP [J]. Journal of Propulsion and Power, 2008,24(3): 499-506.[14] Neviere R. An Extension of the Time-Temperature Superposition Principal to non-linear Viscoelastic Solids [J]. Int Journal of Solids and Structures, 2006, 43:5295-5306.[15] Yilmazer U Farris R J. Mechanical behavior and dilatation of particulate-filled thermosets in the rubbery state [J]. Journal of AppliedPolymer Science, 1983, 28(11): 3369-3386.。
HTPB推进剂粘聚断裂研究
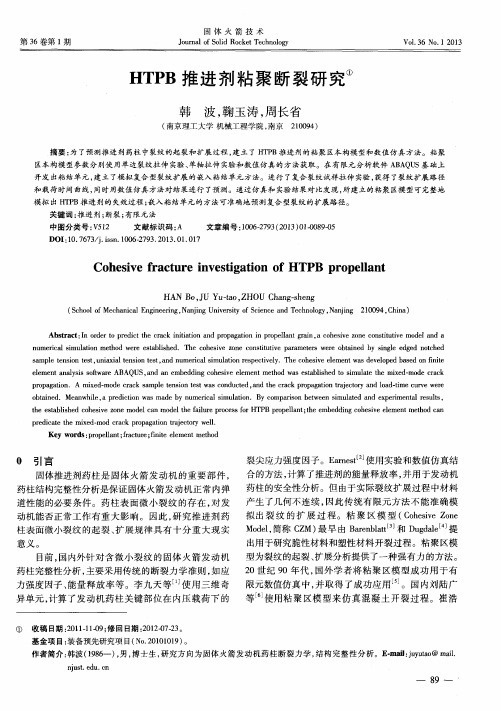
DOI : 1 0 . 7 6 7 3 / i . i s s n . 1 0 0 6 - 2 7 9 3 . 2 0 1 3 . 0 1 . 0 1 7
Co h e s i v e f r a c t u r e i n v e s t i g a t i o n o f H TPB p r o p e l l a n t
摘要 : 为 了预 测推进 剂药柱 中裂纹的起裂和扩展过程 , 建立 了 H T P B推进剂 的粘聚 区本构模 型和数 值仿真 方法。粘聚
区本构模 型参数 分别使 用单边裂纹拉伸 实验 、 单轴拉伸 实验和数值仿真 的方法获取 。在有 限元 分析软件 A B A Q U S 基础 上
开发 出粘 结单 元 , 建立 了模拟 复合 型裂纹扩展 的嵌入粘结单元 方法。进行 了复合 裂纹试样拉 伸 实验 , 获得 了裂纹扩展路径 和栽荷 时间曲线, 同时用数值仿真 方法对结果进行 了预测。通过仿真和 实验 结果 对比发现 , 所建立 的粘聚 区模 型可完整地
固 体 火 箭 技 术 第3 6卷第 1 期
J o u r n a l o f S o l i d Ro c k e t T e c h n o l o g y
固体推进剂药柱表面裂纹动态力学特性研究的开题报告
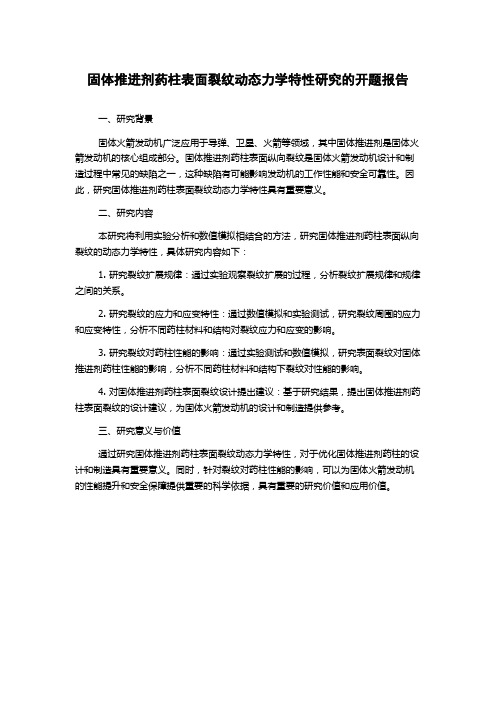
固体推进剂药柱表面裂纹动态力学特性研究的开题报告
一、研究背景
固体火箭发动机广泛应用于导弹、卫星、火箭等领域,其中固体推进剂是固体火箭发动机的核心组成部分。
固体推进剂药柱表面纵向裂纹是固体火箭发动机设计和制造过程中常见的缺陷之一,这种缺陷有可能影响发动机的工作性能和安全可靠性。
因此,研究固体推进剂药柱表面裂纹动态力学特性具有重要意义。
二、研究内容
本研究将利用实验分析和数值模拟相结合的方法,研究固体推进剂药柱表面纵向裂纹的动态力学特性,具体研究内容如下:
1. 研究裂纹扩展规律:通过实验观察裂纹扩展的过程,分析裂纹扩展规律和规律之间的关系。
2. 研究裂纹的应力和应变特性:通过数值模拟和实验测试,研究裂纹周围的应力和应变特性,分析不同药柱材料和结构对裂纹应力和应变的影响。
3. 研究裂纹对药柱性能的影响:通过实验测试和数值模拟,研究表面裂纹对固体推进剂药柱性能的影响,分析不同药柱材料和结构下裂纹对性能的影响。
4. 对固体推进剂药柱表面裂纹设计提出建议:基于研究结果,提出固体推进剂药柱表面裂纹的设计建议,为固体火箭发动机的设计和制造提供参考。
三、研究意义与价值
通过研究固体推进剂药柱表面裂纹动态力学特性,对于优化固体推进剂药柱的设计和制造具有重要意义。
同时,针对裂纹对药柱性能的影响,可以为固体火箭发动机的性能提升和安全保障提供重要的科学依据,具有重要的研究价值和应用价值。
国产HTPB复合固体推进剂Ⅰ-Ⅱ型裂纹断裂性能实验研究
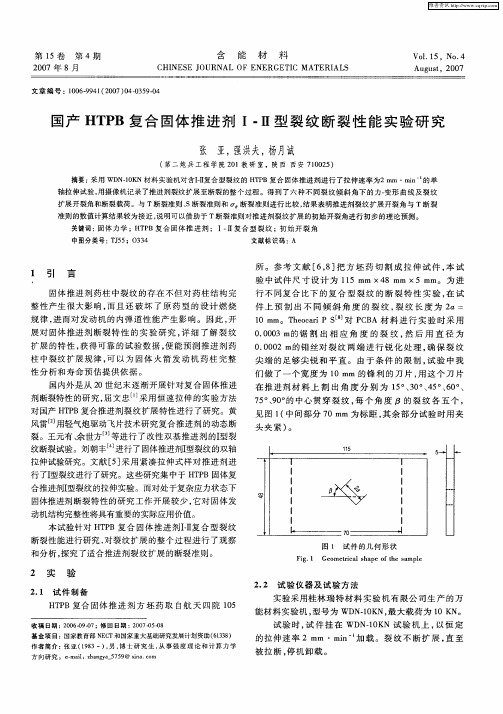
第 1 5卷
第 4期
含 能 材 料
C NE E J RN NERG T C MA ERI S HI S OU AL OF E E I T AL
Vo . 5,No 4 11 . Au u t g s ,20 7 0
20 0 7年 8月
文章 编 号 :1 0 —9 1 2 0 ) 40 5 —4 0 69 4 ( 0 7 0 ,3 9 0
国产 HT B 复 合 固 体 推 进 剂 I一 P Ⅱ型 裂 纹 断 裂 性 能 实 验 研 究
张 亚, 强洪夫, 诚 杨月
( 二 炮 兵 工 程 学 院 2 1教 研 室 ,陕 西 西安 7 0 2 ) 第 0 105 摘要 : 用 WD 一 K 采 N 1 N材料 实 验机 对含I 复合 型裂纹 的 H P 0 一 Ⅱ T B复合 固体 推进 剂 进行 了拉伸 速率 为2m ・ i 的单 m mn 轴拉 伸 试验 , 用摄像 机记 录 了推进 剂裂 纹 扩展 至断 裂的 整个 过 程 。得 到 了 六 种不 同裂 纹 倾 斜 角 下 的力 一 形 曲线 及 裂纹 变 扩展 开 裂角 和断裂 载荷 。与 T断 裂准 则 、 s断裂 准则 和 断裂 准则 进 行 比较 , 结果 表 明推 进剂 裂纹 扩 展开 裂 角 与 T断裂
拉伸试验研究 。文献 [ ] 用紧 凑拉伸式样 对推 进剂进 5采
行了I 型裂纹进行 了研究。这些研究集 中于 H P T B固体复
合推进剂I 型裂纹 的拉伸实验 。而对处于复杂应力状态下
固体推进剂断 裂特性的研 究工作 开展较 少 , 它对 固体发
动机结构完整性将具有重要的实 际应用价值 。 本试 验针对 H P T B复合 固体 推进 剂I 复合 型裂 纹 一 Ⅱ
固体火箭发动机药柱裂纹的J积分分析
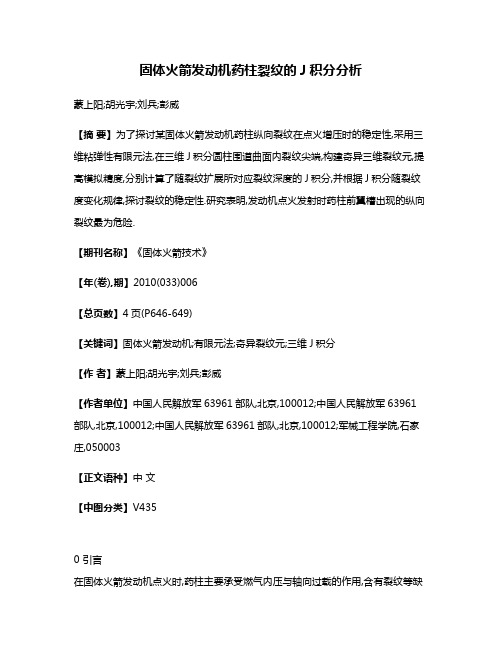
固体火箭发动机药柱裂纹的J积分分析蒙上阳;胡光宇;刘兵;彭威【摘要】为了探讨某固体火箭发动机药柱纵向裂纹在点火增压时的稳定性,采用三维粘弹性有限元法,在三维J积分圆柱围道曲面内裂纹尖端,构建奇异三维裂纹元,提高模拟精度,分别计算了随裂纹扩展所对应裂纹深度的J积分,并根据J积分随裂纹度变化规律,探讨裂纹的稳定性.研究表明,发动机点火发射时药柱前翼槽出现的纵向裂纹最为危险.【期刊名称】《固体火箭技术》【年(卷),期】2010(033)006【总页数】4页(P646-649)【关键词】固体火箭发动机;有限元法;奇异裂纹元;三维J积分【作者】蒙上阳;胡光宇;刘兵;彭威【作者单位】中国人民解放军63961部队,北京,100012;中国人民解放军63961部队,北京,100012;中国人民解放军63961部队,北京,100012;军械工程学院,石家庄,050003【正文语种】中文【中图分类】V4350 引言在固体火箭发动机点火时,药柱主要承受燃气内压与轴向过载的作用,含有裂纹等缺陷的发动机在点火时易出现事故。
因为发动机点火增压时,燃气可能进入裂纹腔内,导致原有裂纹扩展;若裂纹失稳扩展,会导致发动机发生穿火或轰爆等灾难性事故。
因此,获取控制推进剂药柱裂纹开裂的物理参量,判断裂纹的稳定性,一直是固体推进技术领域最为关心的研究课题之一。
在点火增压过程中,发动机药柱始终处于高温、高压的严酷环境,且这一过程难以重现,试图通过点火试验测试发动机药柱裂纹的稳定性十分困难。
随着数值仿真技术的发展,采用数值方法计算推进剂药柱裂纹开裂的物理参量取得了长足进步。
数值方法不受发动机的结构形状、承受载荷种类及药柱裂纹分布等方面限制,可大大减少物理样机试验,节约研发经费。
固体火箭发动机药柱裂纹数值仿真技术发展重要方向是提高计算精度,不仅通过构建平面奇异单元提高计算平面裂纹的应力强度因子的精度[1-2],而且为进一步模拟发动机的真实响应,通过构建发动机的三维有限元模型获取推进剂药柱三维裂纹的断裂参量[3-6]。
- 1、下载文档前请自行甄别文档内容的完整性,平台不提供额外的编辑、内容补充、找答案等附加服务。
- 2、"仅部分预览"的文档,不可在线预览部分如存在完整性等问题,可反馈申请退款(可完整预览的文档不适用该条件!)。
- 3、如文档侵犯您的权益,请联系客服反馈,我们会尽快为您处理(人工客服工作时间:9:00-18:30)。
要 大得 多 。
关键词 : H T P B推进 剂; . , 积分; 几 积分 ; 裂纹构型 因子 ; 裂纹起 裂
me t h o d s e s t bl a i s h e d c a n c a l c u l a t e t h e J - i n t e ra g l a n d i n t e ra g l o f p op r e l l nt a . h e T i n i t i a t i o n J - i n t e ra g l a n d J i n t e g r l a h a v e a n o b v i —
固 体 火 箭 技 术
第 3 8卷 第 3期
J o u na r l o f S o l i d Ro c k e t T e c h n o l o g y Vo 1 . 3 8 No . 3 2 01 5
HT P B 推 进 剂 裂 纹 起 裂 积 分 研 究①
龙
( 1 . 第二炮兵工程 大学 , 西安
兵 , 常新 龙 , 陈
刚 , 马仁利
1 0 0 0 7 4 )
7 1 0 0 2 5 ; 2 . 第二炮兵装备部科研 部驻 航天科技集团一院型号办 , 北京
摘要 : 为研 究 H T P B推进 剂的裂纹起 裂特性 , 建立 了一种 由单试件 计算推进 剂 I , 积分和 凡 积分 的方法 , 开展 了 H T P B
推进剂松弛试验和含 I 型裂纹平板试件 . , 积分试验 , 标定 了试件的裂纹构型 因子 , 得 到 了推进 剂的载荷- 虚位移 曲线以及 裂 纹起裂 J积分和 . , 积分值 。结果表 明, 文 中建立的方 法能 够很好地计 算推进 剂的裂纹起裂 . , 积分和 . , 积分值 , 推进 剂的
D e p a r t me n t i n t h e 1 s t R e s e a r c h I n s t i t u t e o f C A S I C, B e i j i n g 1 0 0 0 7 4 , C h i n a )
Ab s t r a c t : I n o r d e r t o s t u d y t h e c r a c k i n i t i a t i o n p r o p e t r y o f t h e HT P B p r o p e l l a n t ,a wa y c a l c u l a t i n g t h e J - i n t e g r a l a n d J v i n t e - ra g l t h r o u g h s i n g l e s p e c i me n w a s e s t a b l i s h e d. T h e r e l a x a t i o n e x p e i r me n t nd a t h e 1 一 i n t e g r l a e x p e ime r n t o f p r o p e l l a n t s p e c i me n wi t h mo d e I c r a c k we r e c a r r i e d o u t 。t h e c r a c k c o n f i g u r a t i o n f a c t o r o f t h e s p e c i me n w a s c lc a u l a t e d,a n d t h e l o a d p s e u d o e l a s t i c d i s p l a c e — me n t c u r v e s we r e o b t a i n e d . T h e c r a c k ro g w t h i n i t i a t i o n J - i n t e ra g l a n d J v i n t e g r l a o f t h e p r o p e l nt a we l ' e g a i n e d . Re s u l t s s h o w t h a t t h e
Re s e a r c h o f t h e c r a c k i n i t i a t i o n J - i n t e g r a l f o r
HTPB s o l i d p r o p e l l a n t
L ONG Bi n g ,CHANG Xi n. 1 o n g ,CHEN Ga n g 。 MA Re n- l i
中 图分 类 号 : V 5 1 2 文 献标 识 码 : A 文章编号 : 1 0 0 6 — 2 7 9 3 ( 2 0 1 5 ) 0 3 — 0 3 6 7 — 0 5
D oI : 1 0 . 7 6 7 3 / j . i s s n . 1 0 0 6 — 2 7 9 3 . 2 0 1 5 . 0 . T h e S e c o n d A r t i l l e r y E n g i n e e i r n g U n i v e r s i t y , X i " a n 7 1 0 0 2 5 , C h i n a ;
2 . T h e S c i e n t i i f c Re s e a r c h D e p a r t me n t o f t h e S e c o n d Ar ti l l e r y E q u i p me n t