土木工程专业英语课文原文及对照翻译
完整版土木工程专业英语课文原文及对照翻译
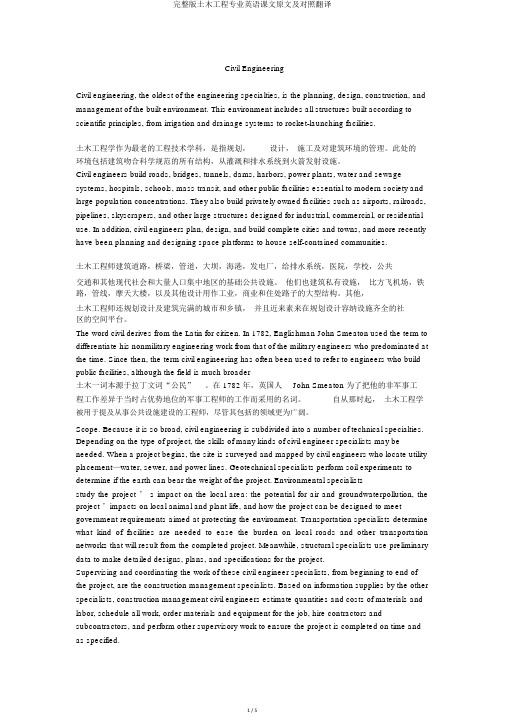
Civil EngineeringCivil engineering, the oldest of the engineering specialties, is the planning, design, construction, and management of the built environment. This environment includes all structures built according to scientific principles, from irrigation and drainage systems to rocket-launching facilities.土木工程学作为最老的工程技术学科,是指规划,设计,施工及对建筑环境的管理。
此处的环境包括建筑吻合科学规范的所有结构,从灌溉和排水系统到火箭发射设施。
Civil engineers build roads, bridges, tunnels, dams, harbors, power plants, water and sewage systems, hospitals, schools, mass transit, and other public facilities essential to modern society and large population concentrations. They also build privately owned facilities such as airports, railroads, pipelines, skyscrapers, and other large structures designed for industrial, commercial, or residential use. In addition, civil engineers plan, design, and build complete cities and towns, and more recently have been planning and designing space platforms to house self-contained communities.土木工程师建筑道路,桥梁,管道,大坝,海港,发电厂,给排水系统,医院,学校,公共交通和其他现代社会和大量人口集中地区的基础公共设施。
土木工程专业英语(带翻译)

State-of-the-art report of bridge health monitoring AbstractThe damage diagnosis and healthmonitoring of bridge structures are active areas of research in recent years. Comparing with the aerospace engineering and mechanical engineering, civil engineering has the specialities of its own in practice. For example, because bridges, as well as most civil engineering structures, are large in size, and have quite lownatural frequencies and vibration levels, at low amplitudes, the dynamic responses of bridge structure are substantially affected by the nonstructural components, unforeseen environmental conditions, and changes in these components can easily to be confused with structural damage.All these give the damage assessment of complex structures such as bridges a still challenging task for bridge engineers. This paper firstly presents the definition of structural healthmonitoring system and its components. Then, the focus of the discussion is placed on the following sections:①the laboratory and field testing research on the damage assessment;②analytical developments of damage detectionmethods, including (a) signature analysis and pattern recognition approaches, (b) model updating and system identification approaches, (c) neural networks approaches; and③sensors and their optimum placements. The predominance and shortcomings of each method are compared and analyzed. Recent examples of implementation of structural health monitoring and damage identification are summarized in this paper. The key problem of bridge healthmonitoring is damage automatic detection and diagnosis, and it is the most difficult problem. Lastly, research and development needs are addressed.1 IntroductionDue to a wide variety of unforeseen conditions and circumstance, it will never be possible or practical to design and build a structure that has a zero percent probability of failure. Structural aging, environmental conditions, and reuse are examples of circumstances that could affect the reliability and thelife of a structure. There are needs of periodic inspections to detect deterioration resulting from normal operation and environmental attack or inspections following extreme events, such as strong-motion earthquakes or hurricanes. To quantify these system performance measures requires some means to monitor and evaluate the integrity of civil structureswhile in service. Since the Aloha Boeing 737 accident that occurred on April 28, 1988, such interest has fostered research in the areas of structural health monitoring and non-destructive damage detection in recent years.According to Housner, et al. (1997), structural healthmonitoring is defined as“the use ofin-situ,non-destructive sensing and analysis of structural characteristics, including the structural response, for detecting changes that may indicate damage or degradation”[1]. This definition also identifies the weakness. While researchers have attempted the integration of NDEwith healthmonitoring, the focus has been on data collection, not evaluation. What is needed is an efficient method to collect data from a structure in-service and process the data to evaluate key performance measures, such as serviceability, reliability, and durability. So, the definition byHousner, et al.(1997)should be modified and the structural health monitoring may be defined as“the use ofin-situ,nondestructive sensing and analysis of structural characteristics, including the structural response, for the purpose of identifying if damage has occurred, determining the location of damage, estimatingthe severityof damage and evaluatingthe consequences of damage on the structures”(Fig.1). In general, a structural health monitoring system has the potential to provide both damage detection and condition assessment of a structure.Assessing the structural conditionwithout removingthe individual structural components is known as nondestructive evaluation (NDE) or nondestructive inspection. NDE techniques include those involving acoustics, dye penetrating,eddy current, emission spectroscopy, fiber-optic sensors, fiber-scope, hardness testing, isotope, leak testing, optics, magnetic particles, magnetic perturbation, X-ray, noise measurements, pattern recognition, pulse-echo, ra-diography, and visual inspection, etc. Mostof thesetechniques have been used successfullyto detect location of certain elements, cracks orweld defects, corrosion/erosion, and so on. The FederalHighwayAdministration(FHWA, USA)was sponsoring a large program of research and development in new technologies for the nondestructive evaluation of highway bridges. One of the two main objectives of the program is to develop newtools and techniques to solve specific problems. The other is to develop technologies for the quantitative assessment of the condition of bridges in support of bridge management and to investigate howbest to incorporate quantitative condition information into bridge management systems. They hoped to develop technologies to quickly, efficiently, and quantitatively measure global bridge parameters, such as flexibility and load-carrying capacity. Obviously, a combination of several NDE techniques may be used to help assess the condition of the system. They are very important to obtain the data-base for the bridge evaluation.But it is beyond the scope of this review report to get into details of local NDE.Health monitoring techniques may be classified as global and local. Global attempts to simultaneously assess the condition of the whole structure whereas local methods focus NDE tools on specific structural components. Clearly, two approaches are complementaryto eachother. All such available informationmaybe combined and analyzed by experts to assess the damage or safety state of the structure.Structural health monitoring research can be categorized into the following four levels: (I) detecting the existence of damage, (II) findingthe location of damage, (III) estimatingthe extentof damage, and (IV) predictingthe remaining fatigue life. The performance of tasks of Level (III) requires refined structural models and analyses, local physical examination, and/or traditional NDE techniques. To performtasks ofLevel (IV) requires material constitutive information on a local level, materials aging studies, damage mechanics, and high-performance computing. With improved instrumentation and understanding of dynamics of complex structures, health monitoring and damage assessment of civil engineering structures has become more practical in systematic inspection andevaluation of these structures during the past two decades.Most structural health monitoringmethods under current investigation focus on using dynamic responses to detect and locate damage because they are global methods that can provide rapid inspection of large structural systems.These dynamics-based methods can be divided into fourgroups:①spatial-domain methods,②modal-domain methods,③time-domain methods, and④frequency- domain methods. Spatial-domain methods use changes of mass, damping, and stiffness matrices to detect and locate damage. Modal-domain methods use changes of natural frequencies, modal damping ratios, andmode shapesto detect damage. In the frequency domain method, modal quantities such as natural frequencies, damping ratio, and model shapes are identified.The reverse dynamic systemof spectral analysis and the generalized frequency response function estimated fromthe nonlinear auto-regressive moving average (NARMA) model were applied in nonlinear system identification. In time domainmethod, systemparameterswere determined fromthe observational data sampled in time. It is necessaryto identifythe time variation of systemdynamic characteristics fromtime domain approach if the properties of structural system changewith time under the external loading condition. Moreover, one can use model-independent methods or model-referenced methods to perform damage detection using dynamic responses presented in any of the four domains. Literature shows that model independent methods can detect the existence of damage without much computational efforts, butthey are not accurate in locating damage. On the otherhand, model-referencedmethods are generally more accurate in locating damage and require fewer sensors than model-independent techniques, but they require appropriate structural models and significant computational efforts. Although time-domain methods use original time-domain datameasured using conventional vibrationmeasurement equipment, theyrequire certain structural information and massive computation and are case sensitive. Furthermore, frequency- and modal-domain methods use transformed data,which contain errors and noise due totransformation.Moreover, themodeling and updatingofmass and stiffnessmatrices in spatial-domain methods are problematic and difficult to be accurate. There are strong developmenttrends that two or three methods are combined together to detect and assess structural damages.For example, several researchers combined data of static and modal tests to assess damages. The combination could remove the weakness of each method and check each other. It suits the complexity of damage detection.Structural health monitoring is also an active area of research in aerospace engineering, but there are significant differences among the aerospace engineering, mechanical engineering, and civil engineering in practice. For example,because bridges, as well as most civil engineering structures, are large in size, and have quite lownatural frequencies and vibration levels, at lowamplitudes, the dynamic responses of bridge structure are substantially affected by the non-structural components, and changes in these components can easily to be confused with structural damage. Moreover,the level of modeling uncertainties in reinforced concrete bridges can be much greater than the single beam or a space truss. All these give the damage assessment of complex structures such as bridges a still challenging task for bridge engineers. Recent examples of research and implementation of structural health monitoring and damage assessment are summarized in the following sections.2 Laboratory and field testing researchIn general, there are two kinds of bridge testing methods, static testing and dynamic testing. The dynamic testing includes ambient vibration testing and forced vibration testing. In ambient vibration testing, the input excitation is not under the control. The loading could be either micro-tremors, wind, waves, vehicle or pedestrian traffic or any other service loading. The increasing popularity of this method is probably due to the convenience of measuring the vibrationresponse while the bridge is under in-service and also due to the increasing availability of robust data acquisition and storage systems. Since the input is unknown, certain assumptions have to be made. Forced vibration testing involves application of input excitation of known force level at known frequencies. The excitation manners include electro-hydraulic vibrators, forcehammers, vehicle impact, etc. The static testing in the laboratory may be conducted by actuators, and by standard vehicles in the field-testing.we can distinguish that①the models in the laboratory are mainly beams, columns, truss and/or frame structures, and the location and severity of damage in the models are determined in advance;②the testing has demonstrated lots of performances of damage structures;③the field-testing and damage assessmentof real bridges are more complicated than the models in the laboratory;④the correlation between the damage indicator and damage type,location, and extentwill still be improved.3 Analytical developmentThe bridge damage diagnosis and health monitoring are both concerned with two fundamental criteria of the bridges, namely, the physical condition and the structural function. In terms of mechanics or dynamics, these fundamental criteria can be treated as mathematical models, such as response models, modal models and physical models.Instead of taking measurements directly to assess bridge condition, the bridge damage diagnosis and monitoring systemevaluate these conditions indirectly by using mathematical models. The damage diagnosis and health monitoring are active areas of research in recentyears. For example, numerous papers on these topics appear in the proceedings of Inter-national Modal Analysis Conferences (IMAC) each year, in the proceedings of International Workshop on Structural HealthMonitoring (once of two year, at Standford University), in the proceedings of European Conference on Smart materials and Structures and European Conference on Structural Damage AssessmentUsing Advanced Signal Processing Procedures, in the proceedings ofWorld Conferences of Earthquake Engineering, and in the proceedings of International Workshop on Structural Control, etc.. There are several review papers to be referenced, for examples,Housner, et al. (1997)provided an extensive summary of the state of the art in control and health monitoring of civil engineering structures[1].Salawu (1997)discussed and reviewed the use of natural frequency as a diagnostic parameter in structural assessment procedures using vibrationmonitoring.Doebling, Farrar, et al. (1998)presented a through review of the damage detection methods by examining changes in dynamic properties.Zou, TongandSteven (2000)summarized the methods of vibration-based damage and health monitoring for composite structures, especially in delamination modeling techniques and delamination detection.4 Sensors and optimum placementOne of the problems facing structural health monitoring is that very little is known about the actual stress and strains in a structure under external excitations. For example, the standard earthquake recordings are made ofmotions of the floors of the structure and no recordings are made of the actual stresses and strains in structural members. There is a need for special sensors to determine the actual performance of structural members. Structural health monitoring requires integrated sensor functionality to measure changes in external environmental conditions, signal processing functionality to acquire, process, and combine multi-sensor and multi-measured information. Individual sensors and instrumented sensor systems are then required to provide such multiplexed information.FuandMoosa (2000)proposed probabilistic advancing cross-diagnosis method to diagnosis-decision making for structural health monitoring. It was experimented in the laboratory respectively using a coherent laser radar system and a CCD high-resolution camera. Results showed that this method was promising for field application. Another new idea is thatneural networktechniques are used to place sensors. For example,WordenandBurrows (2001)used the neural network and methods of combinatorial optimization to locate and classify faults.The static and dynamic data are collected from all kinds of sensorswhich are installed on the measured structures.And these datawill be processed and usable informationwill be extracted. So the sensitivity, accuracy, and locations,etc. of sensors are very important for the damage detections. The more information are obtained, the damage identification will be conducted more easily, but the price should be considered. That’s why the sensors are determinedin an optimal ornearoptimal distribution. In aword, the theory and validation ofoptimumsensor locationswill still being developed.5 Examples of health monitoring implementationIn order for the technology to advance sufficiently to become an operational system for the maintenance and safety of civil structures, it is of paramount importance that new analytical developments are ultimately verified with appropriate data obtained frommonitoring systems, which have been implemented on civil structures, such as bridges.Mufti (2001)summarized the applications of SHM of Canadian bridge engineering, including fibre-reinforced polymers sensors, remote monitoring, intelligent processing, practical applications in bridge engineering, and technology utilization. Further study and applications are still being conducted now.FujinoandAbe(2001)introduced the research and development of SHMsystems at the Bridge and Structural Lab of the University of Tokyo. They also presented the ambient vibration based approaches forLaser DopplerVibrometer (LDV) and the applications in the long-span suspension bridges.The extraction of the measured data is very hard work because it is hard to separate changes in vibration signature duo to damage form changes, normal usage, changes in boundary conditions, or the release of the connection joints.Newbridges offer opportunities for developing complete structural health monitoring systems for bridge inspection and condition evaluation from“cradle to grave”of the bridges. Existing bridges provide challenges for applying state-of-the-art in structural health monitoring technologies to determine the current conditions of the structural element,connections and systems, to formulate model for estimating the rate of degradation, and to predict the existing and the future capacities of the structural components and systems. Advanced health monitoring systems may lead to better understanding of structural behavior and significant improvements of design, as well as the reduction of the structural inspection requirements. Great benefits due to the introduction of SHM are being accepted by owners, managers, bridge engineers,etc..6 Research and development needsMost damage detection theories and practices are formulated based on the following assumption: that failure or deterioration would primarily affect the stiffness and therefore affect the modal characteristics of the dynamic response of the structure. This is seldom true in practice, because①Traditional modal parameters (natural frequency, damping ratio and mode shapes, etc.) are not sensitive enough to identify and locate damage. The estimation methods usually assume that structures are linear and proportional damping systems.②Most currently used damage indices depend on the severity of the damage, which is impractical in the field. Most civil engineering structures, such as highway bridges, have redundancy in design and large in size with low natural frequencies. Any damage index should consider these factors.③Scaledmodelingtechniques are used in currentbridge damage detection. Asingle beam/girder models cannot simulate the true behavior of a real bridge. Similitude laws for dynamic simulation and testing should be considered.④Manymethods usually use the undamaged structural modal parameters as the baseline comparedwith the damaged information. This will result in the need of a large data storage capacity for complex structures. But in practice,there are majority of existing structures for which baseline modal responses are not available. Only one developed method(StubbsandKim (1996)), which tried to quantify damagewithout using a baseline, may be a solution to this difficulty. There is a lot of researchwork to do in this direction.⑤Seldommethods have the ability to distinguish the type of damages on bridge structures. To establish the direct relationship between the various damage patterns and the changes of vibrational signatures is not a simple work.Health monitoring requires clearly defined performance criteria, a set of corresponding condition indicators and global and local damage and deterioration indices, which should help diagnose reasons for changes in condition indicators. It is implausible to expect that damage can be reliably detected or tracked byusing a single damage index. We note that many additional localized damage indiceswhich relate to highly localized properties ofmaterials or the circumstances may indicate a susceptibility of deterioration such as the presence of corrosive environments around reinforcing steel in concrete, should be also integrated into the health monitoring systems.There is now a considerable research and development effort in academia, industry, and management department regarding global healthmonitoring for civil engineering structures. Several commercial structural monitoring systems currently exist, but further development is needed in commercialization of the technology. We must realize that damage detection and health monitoring for bridge structures by means of vibration signature analysis is a very difficult task. Itcontains several necessary steps, including defining indicators on variations of structural physical condition, dynamic testing to extract such indication parameters, defining the type of damages and remaining capacity or life of the structure, relating the parameters to the defined damage/aging. Unfortunately, to date, no one has accomplished the above steps. There is a lot of work to do in future.桥梁健康监测应用与研究现状摘要桥梁损伤诊断与健康监测是近年来国际上的研究热点,在实践方面,土木工程和航空航天工程、机械工程有明显的差别,比如桥梁结构以及其他大多数土木结构,尺寸大、质量重,具有较低的自然频率和振动水平,桥梁结构的动力响应极容易受到不可预见的环境状态、非结构构件等的影响,这些变化往往被误解为结构的损伤,这使得桥梁这类复杂结构的损伤评估具有极大的挑战性.本文首先给出了结构健康监测系统的定义和基本构成,然后集中回顾和分析了如下几个方面的问题:①损伤评估的室内实验和现场测试;②损伤检测方法的发展,包括:(a)动力指纹分析和模式识别方法, (b)模型修正和系统识别方法, (c)神经网络方法;③传感器及其优化布置等,并比较和分析了各自方法的优点和不足.文中还总结了健康监测和损伤识别在桥梁工程中的应用,指出桥梁健康监测的关键问题在于损伤的自动检测和诊断,这也是困难的问题;最后展望了桥梁健康监测系统的研究和发展方向.关键词:健康监测系统;损伤检测;状态评估;模型修正;系统识别;传感器优化布置;神经网络方法;桥梁结构1概述由于不可预见的各种条件和情况下,设计和建造一个结构将永远不可能或无实践操作性,它有一个失败的概率百分之零。
土木工程概论-中英对照

土木工程概论—中英对照对土木工程的发展起关键作用的,首先是作为工程物质基础的土木建筑材料,其次是随之发展起来的设计理论和施工技术。
每当出现新的优良的建筑材料时,土木工程就会有飞跃式的发展。
人们在早期只能依靠泥土、木料及其它天然材料从事营造活动,后来出现了砖和瓦这种人工建筑材料,使人类第一次冲破了天然建筑材料的束缚。
中国在公元前十一世纪的西周初期制造出瓦。
最早的砖出现在公元前五世纪至公元前三世纪战国时的墓室中。
砖和瓦具有比土更优越的力学性能,可以就地取材,而又易于加工制作。
砖和瓦的出现使人们开始广泛地、大量地修建房屋和城防工程等。
由此土木工程技术得到了飞速的发展。
直至18~19世纪,在长达两千多年时间里,砖和瓦一直是土木工程的重要建筑材料,为人类文明作出了伟大的贡献,甚至在目前还被广泛采用。
钢材的大量应用是土木工程的第二次飞跃。
十七世纪70年代开始使用生铁、十九世纪初开始使用熟铁建造桥梁和房屋,这是钢结构出现的前奏。
从十九世纪中叶开始,冶金业冶炼并轧制出抗拉和抗压强度都很高、延性好、质量均匀的建筑钢材,随后又生产出高强度钢丝、钢索。
于是适应发展需要的钢结构得到蓬勃发展。
除应用原有的粱、拱结构外,新兴的桁架、框架、网架结构、悬索结构逐渐推广,出现了结构形式百花争艳的局面。
建筑物跨径从砖结构、石结构、木结构的几米、几十米发展到钢结构的百米、几百米,直到现代的千米以上。
于是在大江、海峡上架起大桥,在地面上建造起摩天大楼和高耸铁塔,甚至在地面下铺设铁路,创造出前所未有的奇迹。
为适应钢结构工程发展的需要,在牛顿力学的基础上,材料力学、结构力学、工程结构设计理论等就应运而生。
施工机械、施工技术和施工组织设计的理论也随之发展,土木工程从经验上升成为科学,在工程实践和基础理论方面都面貌一新,从而促成了土木工程更迅速的发展。
十九世纪20年代,波特兰水泥制成后,混凝土问世了。
混凝土骨料可以就地取材,混凝土构件易于成型,但混凝土的抗拉强度很小,用途受到限制。
土木工程专业英语(带翻译)

State-of-the-art report of bridge health monitoring AbstractThe damage diagnosis and healthmonitoring of bridge structures are active areas of research in recent years. Comparing with the aerospace engineering and mechanical engineering, civil engineering has the specialities of its own in practice. For example, because bridges, as well as most civil engineering structures, are large in size, and have quite lownatural frequencies and vibration levels, at low amplitudes, the dynamic responses of bridge structure are substantially affected by the nonstructural components, unforeseen environmental conditions, and changes in these components can easily to be confused with structural damage.All these give the damage assessment of complex structures such as bridges a still challenging task for bridge engineers. This paper firstly presents the definition of structural healthmonitoring system and its components. Then, the focus of the discussion is placed on the following sections:①the laboratory and field testing research on the damage assessment;②analytical developments of damage detectionmethods, including (a) signature analysis and pattern recognition approaches, (b) model updating and system identification approaches, (c) neural networks approaches; and③sensors and their optimum placements. The predominance and shortcomings of each method are compared and analyzed. Recent examples of implementation of structural health monitoring and damage identification are summarized in this paper. The key problem of bridge healthmonitoring is damage automatic detection and diagnosis, and it is the most difficult problem. Lastly, research and development needs are addressed.1 IntroductionDue to a wide variety of unforeseen conditions and circumstance, it will never be possible or practical to design and build a structure that has a zero percent probability of failure. Structural aging, environmental conditions, and reuse are examples of circumstances that could affect the reliability and the life of a structure. There are needs of periodic inspections to detect deterioration resulting from normal operation and environmental attack or inspections following extreme events, such as strong-motion earthquakes or hurricanes. To quantify these system performance measures requires some means to monitor and evaluate the integrity of civil structureswhile in service. Since the Aloha Boeing 737 accident that occurred on April28, 1988, such interest has fostered research in the areas of structural health monitoring and non-destructive damage detection in recent years.According to Housner, et al. (1997), structural healthmonitoring is defined as“the use ofin-situ,non-destructive sensing and analysis of structural characteristics, including the structural response, for detecting changes that may indicate damage or degradation”[1]. This definition also identifies the weakness. While researchers have attempted the integration of NDEwith healthmonitoring, the focus has been on data collection, not evaluation. What is needed is an efficient method to collect data from a structure in-service and process the data to evaluate key performance measures, such as serviceability, reliability, and durability. So, the definition byHousner, et al.(1997)should be modified and the structural health monitoring may be defined as“the use ofin-situ,nondestructive sensing and analysis of structural characteristics, including the structural response, for the purpose of identifying if damage has occurred, determining the location of damage, estimatingthe severityof damage and evaluatingthe consequences of damage on the structures”(Fig.1). In general, a structural health monitoring system has the potential to provide both damage detection and condition assessment of a structure.Assessing the structural conditionwithout removingthe individual structural components is known as nondestructive evaluation (NDE) or nondestructive inspection. NDE techniques include those involving acoustics, dye penetrating,eddy current, emission spectroscopy, fiber-optic sensors, fiber-scope, hardness testing, isotope, leak testing, optics, magnetic particles, magnetic perturbation, X-ray, noise measurements, pattern recognition, pulse-echo, ra-diography, and visual inspection, etc. Mostof these techniques have been used successfullyto detect location of certain elements, cracks orweld defects, corrosion/erosion, and so on. The FederalHighwayAdministration(FHWA, USA)was sponsoring a large program of research and development in new technologies for the nondestructive evaluation of highway bridges. One of the two main objectives of the program is to develop newtools and techniques to solve specific problems. The other is to develop technologies for the quantitative assessment of the condition of bridges in support of bridge management and to investigate howbest to incorporate quantitative condition information into bridge management systems. They hoped to develop technologies to quickly, efficiently, and quantitatively measure global bridge parameters, such as flexibility and load-carrying capacity. Obviously, a combination of several NDEtechniques may be used to help assess the condition of the system. They are very important to obtain the data-base for the bridge evaluation.But it is beyond the scope of this review report to get into details of local NDE.Health monitoring techniques may be classified as global and local. Global attempts to simultaneously assess the condition of the whole structure whereas local methods focus NDE tools on specific structural components. Clearly, two approaches are complementaryto eachother. All such available informationmaybe combined and analyzed by experts to assess the damage or safety state of the structure.Structural health monitoring research can be categorized into the following four levels: (I) detecting the existence of damage, (II) findingthe location of damage, (III) estimatingthe extentof damage, and (IV) predictingthe remaining fatigue life. The performance of tasks of Level (III) requires refined structural models and analyses, local physical examination, and/or traditional NDE techniques. To performtasks ofLevel (IV) requires material constitutive information on a local level, materials aging studies, damage mechanics, and high-performance computing. With improved instrumentation and understanding of dynamics of complex structures, health monitoring and damage assessment of civil engineering structures has become more practical in systematic inspection and evaluation of these structures during the past two decades.Most structural health monitoringmethods under current investigation focus on using dynamic responses to detect and locate damage because they are global methods that can provide rapid inspection of large structural systems.These dynamics-based methods can be divided into fourgroups:①spatial-domain methods,②modal-domain methods,③time-domain methods, and④frequency- domain methods. Spatial-domain methods use changes of mass, damping, and stiffness matrices to detect and locate damage. Modal-domain methods use changes of natural frequencies, modal damping ratios, andmode shapesto detect damage. In the frequency domain method, modal quantities such as natural frequencies, damping ratio, and model shapes are identified.The reverse dynamic systemof spectral analysis and the generalized frequency response function estimated fromthe nonlinear auto-regressive moving average (NARMA) model were applied in nonlinear system identification. In time domainmethod, systemparameterswere determined fromthe observational data sampled in time. It is necessaryto identifythe time variation of systemdynamic characteristics fromtime domain approach if the properties of structural systemchangewith time under the external loading condition. Moreover, one can use model-independent methods or model-referenced methods to perform damage detection using dynamic responses presented in any of the four domains. Literature shows that model independent methods can detect the existence of damage without much computational efforts, butthey are not accurate in locating damage. On the otherhand, model-referencedmethods are generally more accurate in locating damage and require fewer sensors than model-independent techniques, but they require appropriate structural models and significant computational efforts. Although time-domain methods use original time-domain datameasured using conventional vibrationmeasurement equipment, theyrequire certain structural information and massive computation and are case sensitive. Furthermore, frequency- and modal-domain methods use transformed data,which contain errors and noise due totransformation.Moreover, themodeling and updatingofmass and stiffnessmatrices in spatial-domain methods are problematic and difficult to be accurate. There are strong developmenttrends that two or three methods are combined together to detect and assess structural damages.For example, several researchers combined data of static and modal tests to assess damages. The combination could remove the weakness of each method and check each other. It suits the complexity of damage detection.Structural health monitoring is also an active area of research in aerospace engineering, but there are significant differences among the aerospace engineering, mechanical engineering, and civil engineering in practice. For example,because bridges, as well as most civil engineering structures, are large in size, and have quite lownatural frequencies and vibration levels, at lowamplitudes, the dynamic responses of bridge structure are substantially affected by the non-structural components, and changes in these components can easily to be confused with structural damage. Moreover,the level of modeling uncertainties in reinforced concrete bridges can be much greater than the single beam or a space truss. All these give the damage assessment of complex structures such as bridges a still challenging task for bridge engineers. Recent examples of research and implementation of structural health monitoring and damage assessment are summarized in the following sections.2 Laboratory and field testing researchIn general, there are two kinds of bridge testing methods, static testing and dynamic testing. The dynamic testing includes ambient vibration testing and forcedvibration testing. In ambient vibration testing, the input excitation is not under the control. The loading could be either micro-tremors, wind, waves, vehicle or pedestrian traffic or any other service loading. The increasing popularity of this method is probably due to the convenience of measuring the vibrationresponse while the bridge is under in-service and also due to the increasing availability of robust data acquisition and storage systems. Since the input is unknown, certain assumptions have to be made. Forced vibration testing involves application of input excitation of known force level at known frequencies. The excitation manners include electro-hydraulic vibrators, force hammers, vehicle impact, etc. The static testing in the laboratory may be conducted by actuators, and by standard vehicles in the field-testing.we can distinguish that①the models in the laboratory are mainly beams, columns, truss and/or frame structures, and the location and severity of damage in the models are determined in advance;②the testing has demonstrated lots of performances of damage structures;③the field-testing and damage assessmentof real bridges are more complicated than the models in the laboratory;④the correlation between the damage indicator and damage type,location, and extentwill still be improved.3Analytical developmentThe bridge damage diagnosis and health monitoring are both concerned with two fundamental criteria of the bridges, namely, the physical condition and the structural function. In terms of mechanics or dynamics, these fundamental criteria can be treated as mathematical models, such as response models, modal models and physical models.Instead of taking measurements directly to assess bridge condition, the bridge damage diagnosis and monitoring systemevaluate these conditions indirectly by using mathematical models. The damage diagnosis and health monitoring are active areas of research in recentyears. For example, numerous papers on these topics appear in the proceedings of Inter-national Modal Analysis Conferences (IMAC) each year, in the proceedings of International Workshop on Structural HealthMonitoring (once of two year, at Standford University), in the proceedings of European Conference on Smart materials and Structures and European Conference on Structural Damage AssessmentUsing Advanced Signal Processing Procedures, in the proceedings ofWorld Conferences of Earthquake Engineering, and in the proceedings of International Workshop on Structural Control, etc.. There are several review papers to be referenced, for examples,Housner, et al. (1997)provided an extensive summary ofthe state of the art in control and health monitoring of civil engineering structures[1].Salawu (1997)discussed and reviewed the use of natural frequency as a diagnostic parameter in structural assessment procedures using vibration monitoring.Doebling, Farrar, et al. (1998)presented a through review of the damage detection methods by examining changes in dynamic properties.Zou, TongandSteven (2000)summarized the methods of vibration-based damage and health monitoring for composite structures, especially in delamination modeling techniques and delamination detection.4Sensors and optimum placementOne of the problems facing structural health monitoring is that very little is known about the actual stress and strains in a structure under external excitations. For example, the standard earthquake recordings are made ofmotions of the floors of the structure and no recordings are made of the actual stresses and strains in structural members. There is a need for special sensors to determine the actual performance of structural members. Structural health monitoring requires integrated sensor functionality to measure changes in external environmental conditions, signal processing functionality to acquire, process, and combine multi-sensor and multi-measured information. Individual sensors and instrumented sensor systems are then required to provide such multiplexed information.FuandMoosa (2000)proposed probabilistic advancing cross-diagnosis method to diagnosis-decision making for structural health monitoring. It was experimented in the laboratory respectively using a coherent laser radar system and a CCD high-resolution camera. Results showed that this method was promising for field application. Another new idea is thatneural networktechniques are used to place sensors. For example,WordenandBurrows (2001)used the neural network and methods of combinatorial optimization to locate and classify faults.The static and dynamic data are collected from all kinds of sensorswhich are installed on the measured structures.And these datawill be processed and usable informationwill be extracted. So the sensitivity, accuracy, and locations,etc. of sensors are very important for the damage detections. The more information are obtained, the damage identification will be conducted more easily, but the price should be considered. That’s why the sensors are determined in an optimal ornearoptimal distribution. In aword, the theory and validation ofoptimumsensor locationswill still being developed.5 Examples of health monitoring implementationIn order for the technology to advance sufficiently to become an operational system for the maintenance and safety of civil structures, it is of paramount importance that new analytical developments are ultimately verified with appropriate data obtained frommonitoring systems, which have been implemented on civil structures, such as bridges.Mufti (2001)summarized the applications of SHM of Canadian bridge engineering, including fibre-reinforced polymers sensors, remote monitoring, intelligent processing, practical applications in bridge engineering, and technology utilization. Further study and applications are still being conducted now.FujinoandAbe(2001)introduced the research and development of SHMsystems at the Bridge and Structural Lab of the University of Tokyo. They also presented the ambient vibration based approaches forLaser DopplerVibrometer (LDV) and the applications in the long-span suspension bridges.The extraction of the measured data is very hard work because it is hard to separate changes in vibration signature duo to damage form changes, normal usage, changes in boundary conditions, or the release of the connection joints.Newbridges offer opportunities for developing complete structural health monitoring systems for bridge inspection and co ndition evaluation from“cradle to grave”of the bridges. Existing bridges provide challenges for applying state-of-the-art in structural health monitoring technologies to determine the current conditions of the structural element,connections and systems, to formulate model for estimating the rate of degradation, and to predict the existing and the future capacities of the structural components and systems. Advanced health monitoring systems may lead to better understanding of structural behavior and significant improvements of design, as well as the reduction of the structural inspection requirements. Great benefits due to the introduction of SHM are being accepted by owners, managers, bridge engineers, etc..6 Research and development needsMost damage detection theories and practices are formulated based on the following assumption: that failure or deterioration would primarily affect the stiffness and therefore affect the modal characteristics of the dynamic response of the structure. This is seldom true in practice, because①Traditional modal parameters (natural frequency, damping ratio and mode shapes, etc.) are not sensitive enough to identifyand locate damage. The estimation methods usually assume that structures are linear and proportional damping systems.②Most currently used damage indices depend on the severity of the damage, which is impractical in the field. Most civil engineering structures, such as highway bridges, have redundancy in design and large in size with low natural frequencies. Any damage index should consider these factors.③Scaledmodelingtechniques are used in currentbridge damage detection. Asingle beam/girder models cannot simulate the true behavior of a real bridge. Similitude laws for dynamic simulation and testing should be considered.④Manymethods usually use the undamaged structural modal parameters as the baseline comparedwith the damaged information. This will result in the need of a large data storage capacity for complex structures. But in practice,there are majority of existing structures for which baseline modal responses are not available. Only one developed method(StubbsandKim (1996)), which tried to quantify damagewithout using a baseline, may be a solution to this difficulty. There is a lot of researchwork to do in this direction.⑤Seldommethods have the ability to distinguish the type of damages on bridge structures. To establish the direct relationship between the various damage patterns and the changes of vibrational signatures is not a simple work.Health monitoring requires clearly defined performance criteria, a set of corresponding condition indicators and global and local damage and deterioration indices, which should help diagnose reasons for changes in condition indicators. It is implausible to expect that damage can be reliably detected or tracked by using a single damage index. We note that many additional localized damage indiceswhich relate to highly localized properties ofmaterials or the circumstances may indicate a susceptibility of deterioration such as the presence of corrosive environments around reinforcing steel in concrete, should be also integrated into the health monitoring systems.There is now a considerable research and development effort in academia, industry, and management department regarding global healthmonitoring for civil engineering structures. Several commercial structural monitoring systems currently exist, but further development is needed in commercialization of the technology. We must realize that damage detection and health monitoring for bridge structures by means of vibration signature analysis is a very difficult task. Itcontains several necessary steps, including defining indicators on variations of structural physical condition, dynamic testing to extract such indication parameters,defining the type of damages and remaining capacity or life of the structure, relating the parameters to the defined damage/aging. Unfortunately, to date, no one has accomplished the above steps. There is a lot of work to do in future.桥梁健康监测应用与研究现状摘要桥梁损伤诊断与健康监测是近年来国际上的研究热点,在实践方面,土木工程和航空航天工程、机械工程有明显的差别,比如桥梁结构以及其他大多数土木结构,尺寸大、质量重,具有较低的自然频率和振动水平,桥梁结构的动力响应极容易受到不可预见的环境状态、非结构构件等的影响,这些变化往往被误解为结构的损伤,这使得桥梁这类复杂结构的损伤评估具有极大的挑战性.本文首先给出了结构健康监测系统的定义和基本构成,然后集中回顾和分析了如下几个方面的问题:①损伤评估的室内实验和现场测试;②损伤检测方法的发展,包括:(a)动力指纹分析和模式识别方法, (b)模型修正和系统识别方法, (c)神经网络方法;③传感器及其优化布置等,并比较和分析了各自方法的优点和不足.文中还总结了健康监测和损伤识别在桥梁工程中的应用,指出桥梁健康监测的关键问题在于损伤的自动检测和诊断,这也是困难的问题;最后展望了桥梁健康监测系统的研究和发展方向.关键词:健康监测系统;损伤检测;状态评估;模型修正;系统识别;传感器优化布置;神经网络方法;桥梁结构1概述由于不可预见的各种条件和情况下,设计和建造一个结构将永远不可能或无实践操作性,它有一个失败的概率百分之零。
土木工程英文文献及翻译
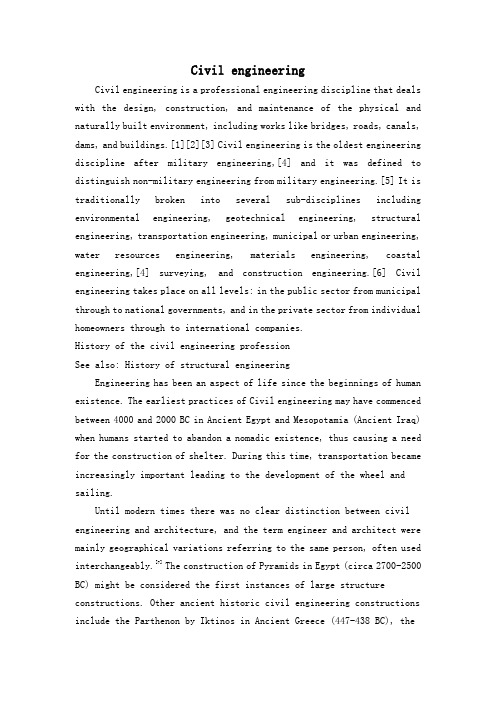
Civil engineeringCivil engineering is a professional engineering discipline that deals with the design, construction, and maintenance of the physical and naturally built environment, including works like bridges, roads, canals, dams, and buildings.[1][2][3] Civil engineering is the oldest engineering discipline after military engineering,[4] and it was defined to distinguish non-military engineering from military engineering.[5] It is traditionally broken into several sub-disciplines including environmental engineering, geotechnical engineering, structural engineering, transportation engineering, municipal or urban engineering, water resources engineering, materials engineering, coastal engineering,[4] surveying, and construction engineering.[6] Civil engineering takes place on all levels: in the public sector from municipal through to national governments, and in the private sector from individual homeowners through to international companies.History of the civil engineering professionSee also: History of structural engineeringEngineering has been an aspect of life since the beginnings of human existence. The earliest practices of Civil engineering may have commenced between 4000 and 2000 BC in Ancient Egypt and Mesopotamia (Ancient Iraq) when humans started to abandon a nomadic existence, thus causing a need for the construction of shelter. During this time, transportation became increasingly important leading to the development of the wheel and sailing.Until modern times there was no clear distinction between civil engineering and architecture, and the term engineer and architect were mainly geographical variations referring to the same person, often used interchangeably.[7]The construction of Pyramids in Egypt (circa 2700-2500 BC) might be considered the first instances of large structure constructions. Other ancient historic civil engineering constructions include the Parthenon by Iktinos in Ancient Greece (447-438 BC), theAppian Way by Roman engineers (c. 312 BC), the Great Wall of China by General Meng T'ien under orders from Ch'in Emperor Shih Huang Ti (c. 220 BC)[6] and the stupas constructed in ancient Sri Lanka like the Jetavanaramaya and the extensive irrigation works in Anuradhapura. The Romans developed civil structures throughout their empire, including especially aqueducts, insulae, harbours, bridges, dams and roads.In the 18th century, the term civil engineering was coined to incorporate all things civilian as opposed to military engineering.[5]The first self-proclaimed civil engineer was John Smeaton who constructed the Eddystone Lighthouse.[4][6]In 1771 Smeaton and some of his colleagues formed the Smeatonian Society of Civil Engineers, a group of leaders of the profession who met informally over dinner. Though there was evidence of some technical meetings, it was little more than a social society.In 1818 the Institution of Civil Engineers was founded in London, and in 1820 the eminent engineer Thomas Telford became its first president. The institution received a Royal Charter in 1828, formally recognising civil engineering as a profession. Its charter defined civil engineering as:the art of directing the great sources of power in nature for the use and convenience of man, as the means of production and of traffic in states, both for external and internal trade, as applied in the construction of roads, bridges, aqueducts, canals, river navigation and docks for internal intercourse and exchange, and in the construction of ports, harbours, moles, breakwaters and lighthouses, and in the art of navigation by artificial power for the purposes of commerce, and in the construction and application of machinery, and in the drainage of cities and towns.[8] The first private college to teach Civil Engineering in the United States was Norwich University founded in 1819 by Captain Alden Partridge.[9] The first degree in Civil Engineering in the United States was awarded by Rensselaer Polytechnic Institute in 1835.[10] The first such degree to be awarded to a woman was granted by Cornell University to Nora Stanton Blatchin 1905.History of civil engineeringCivil engineering is the application of physical and scientific principles, and its history is intricately linked to advances in understanding of physics and mathematics throughout history. Because civil engineering is a wide ranging profession, including several separate specialized sub-disciplines, its history is linked to knowledge of structures, materials science, geography, geology, soils, hydrology, environment, mechanics and other fields.Throughout ancient and medieval history most architectural design and construction was carried out by artisans, such as stone masons and carpenters, rising to the role of master builder. Knowledge was retained in guilds and seldom supplanted by advances. Structures, roads and infrastructure that existed were repetitive, and increases in scale were incremental.[12]One of the earliest examples of a scientific approach to physical and mathematical problems applicable to civil engineering is the work of Archimedes in the 3rd century BC, including Archimedes Principle, which underpins our understanding of buoyancy, and practical solutions such as Archimedes' screw. Brahmagupta, an Indian mathematician, used arithmetic in the 7th century AD, based on Hindu-Arabic numerals, for excavation (volume) computations.[13]Civil engineers typically possess an academic degree with a major in civil engineering. The length of study for such a degree is usually three to five years and the completed degree is usually designated as a Bachelor of Engineering, though some universities designate the degree as a Bachelor of Science. The degree generally includes units covering physics, mathematics, project management, design and specific topics in civil engineering. Initially such topics cover most, if not all, of thesub-disciplines of civil engineering. Students then choose to specialize in one or more sub-disciplines towards the end of the degree.[14]While anUndergraduate (BEng/BSc) Degree will normally provide successful students with industry accredited qualification, some universities offer postgraduate engineering awards (MEng/MSc) which allow students to further specialize in their particular area of interest within engineering.[15]In most countries, a Bachelor's degree in engineering represents the first step towards professional certification and the degree program itself is certified by a professional body. After completing a certified degree program the engineer must satisfy a range of requirements (including work experience and exam requirements) before being certified. Once certified, the engineer is designated the title of Professional Engineer (in the United States, Canada and South Africa), Chartered Engineer (in most Commonwealth countries), Chartered Professional Engineer (in Australia and New Zealand), or European Engineer (in much of the European Union). There are international engineering agreements between relevant professional bodies which are designed to allow engineers to practice across international borders.The advantages of certification vary depending upon location. For example, in the United States and Canada "only a licensed engineer may prepare, sign and seal, and submit engineering plans and drawings to a public authority for approval, or seal engineering work for public and private clients.".[16]This requirement is enforced by state and provincial legislation such as Quebec's Engineers Act.[17]In other countries, no such legislation exists. In Australia, state licensing of engineers is limited to the state of Queensland. Practically all certifying bodies maintain a code of ethics that they expect all members to abide by or risk expulsion.[18] In this way, these organizations play an important role in maintaining ethical standards for the profession. Even in jurisdictions where certification has little or no legal bearing on work, engineers are subject to contract law. In cases where an engineer's work fails he or she may be subject to the tort of negligence and, in extreme cases, thecharge of criminal negligence.[citation needed] An engineer's work must also comply with numerous other rules and regulations such as building codes and legislation pertaining to environmental law.CareersThere is no one typical career path for civil engineers. Most people who graduate with civil engineering degrees start with jobs that require a low level of responsibility, and as the new engineers prove their competence, they are trusted with tasks that have larger consequences and require a higher level of responsibility. However, within each branch of civil engineering career path options vary. In some fields and firms, entry-level engineers are put to work primarily monitoring construction in the field, serving as the "eyes and ears" of senior design engineers; while in other areas, entry-level engineers perform the more routine tasks of analysis or design and interpretation. Experienced engineers generally do more complex analysis or design work, or management of more complex design projects, or management of other engineers, or into specialized consulting, including forensic engineering.In general, civil engineering is concerned with the overall interface of human created fixed projects with the greater world. General civil engineers work closely with surveyors and specialized civil engineers to fit and serve fixed projects within their given site, community and terrain by designing grading, drainage, pavement, water supply, sewer service, electric and communications supply, and land divisions. General engineers spend much of their time visiting project sites, developing community consensus, and preparing construction plans. General civil engineering is also referred to as site engineering, a branch of civil engineering that primarily focuses on converting a tract of land from one usage to another. Civil engineers typically apply the principles of geotechnical engineering, structural engineering, environmental engineering, transportation engineering and construction engineering toresidential, commercial, industrial and public works projects of all sizes and levels of construction翻译:土木工程土木工程是一个专业的工程学科,包括设计,施工和维护与环境的改造,涉及了像桥梁,道路,河渠,堤坝和建筑物工程交易土木工程是最古老的军事工程后,工程学科,它被定义为区分军事工程非军事工程的学科它传统分解成若干子学科包括环境工程,岩土工程,结构工程,交通工程,市或城市工程,水资源工程,材料工程,海岸工程,勘测和施工工程等土木工程的范围涉及所有层次:从市政府到国家,从私人部门到国际公司。
土木工程专业外语,课文翻译
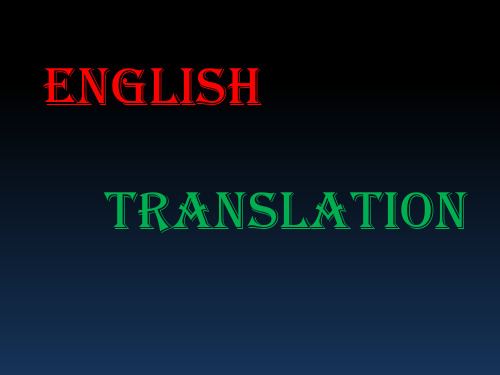
If one looks at technical on structural engineering ,one will find that the meaning of the space frame has been very diverse or even confusing.
A latticed structure is a structure system in the form of a network of elements (as opposed to a continuous surface).
一个网架结构是一个网络元素形成的一 种结构系统(而不是一个连续的表面) 与…相对的
空间架构是一种由线性元素组装安排的结 构系统,以促使其以三维的方式运行
In some cases, the constituent element may be two-dimensional. Macroscopically a space frame often takes the form of a flat or curved surface.
However, in a more restricted sense, space frame means some type of special structure action in three dimensions.
然而,在一个更受限制的观念中, 三维空 间中,空间框架意味着某种类型的特殊 结构功能。
在某些情况下, 组成元素可能是二维的。 宏观上空间框架通常都用平面或曲面。
It should be noted that virtually the same structure defined as a space frame here is referred to as structure.
土木工程专业英语课文翻译陶燕王文萱第九单元
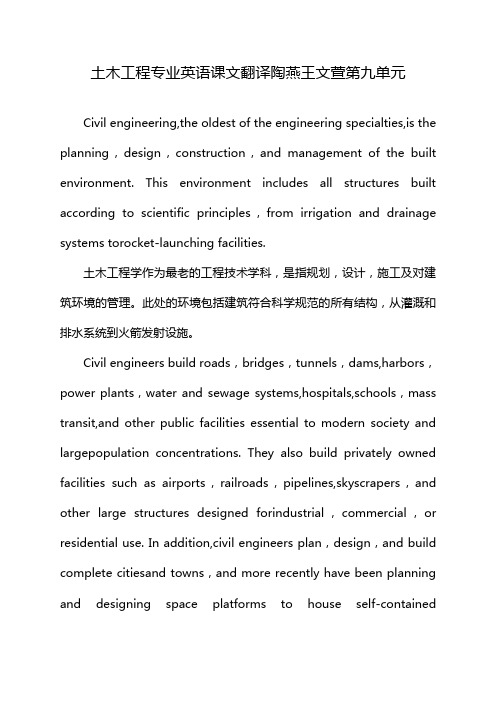
土木工程专业英语课文翻译陶燕王文萱第九单元Civil engineering,the oldest of the engineering specialties,is the planning,design,construction,and management of the built environment. This environment includes all structures built according to scientific principles,from irrigation and drainage systems torocket-launching facilities.土木工程学作为最老的工程技术学科,是指规划,设计,施工及对建筑环境的管理。
此处的环境包括建筑符合科学规范的所有结构,从灌溉和排水系统到火箭发射设施。
Civil engineers build roads,bridges,tunnels,dams,harbors,power plants,water and sewage systems,hospitals,schools,mass transit,and other public facilities essential to modern society and largepopulation concentrations. They also build privately owned facilities such as airports,railroads,pipelines,skyscrapers,and other large structures designed forindustrial,commercial,or residential use. In addition,civil engineers plan,design,and build complete citiesand towns,and more recently have been planning and designing space platforms to house self-containedcommunities.土木工程师建造道路,桥梁,管道,大坝,海港,发电厂,给排水系统,医院,学校,公共交通和其他现代社会和大量人口集中地区的基础公共设施。
土木工程类专业英文文献与翻译

PAVEMENT PROBLEMS CAUSEDBY COLLAPSIBLE SUBGRADESBy Sandra L. Houston,1 Associate Member, ASCE(Reviewed by the Highway Division)ABSTRACT: Problem subgrade materials consisting of collapsible soils are -mon in arid environments, which have climatic conditions and depositional and weathering processes favorable to their formation. Included herein is a discussion of predictive techniques that use commonly available laboratory equipment and testing methods for obtaining reliable estimates of the volume change for these problem soils. A method for predicting relevant stresses and corresponding collapse strains for typical pavement subgrades is presented. Relatively simple methods ofevaluating potential volume change, based on results of familiar laboratory tests, are used.INTRODUCTIONWhen a soil is given free access to water, it may decrease in volume,increase in volume, or do nothing. A soil that increases in volume is calleda swelling or expansive soil, and a soil that decreases in volume is called a collapsible soil. The amount of volume change that occurs depends on thesoil type and structure, the initial soil density, the imposed stress state, and the degree and extent of wetting. Subgrade materials comprised of soils that change volume upon wetting have caused distress to highways since the be- ginning of the professional practice and have cost many millions of dollarsin roadway repairs. The prediction of the volume changes that may occur inthe field is the first step in making an economic decision for dealing with these problem subgrade materials.Each project will have different design considerations, economic con-straints, and risk factors that will have to be taken into account. However, with a reliable method for making volume change predictions, the best design relative to the subgrade soils becomes a matter of economic comparison, anda much more rational design approach may be made. For example, typical techniques for dealing with expansive clays include: (1) In situ treatments with substances such as lime, cement, or fly-ash; (2) seepage barriers and/or drainage systems; or (3) a computing of the serviceability loss and a mod- ification of the design to "accept" the anticipated expansion. In order to make the most economical decision, the amount of volume change (especially non- uniform volume change) must be accurately estimated, and the degree of road roughness evaluated from these data. Similarly, alternative design techniques are available for any roadway problem.The emphasis here will be placed on presenting economical and simplemethods for: (1) Determining whether the subgrade materials are collapsible; and (2) estimating the amount of volume change that is likely to occur in the 'Asst. Prof., Ctr. for Advanced Res. in Transp., Arizona State Univ., Tempe, AZ 85287.Note. Discussion open until April 1, 1989. To extend the closing date one month, a written request must be filed with the ASCE Manager of Journals. The manuscript for this paper was submitted for review and possible publication on February 3, 1988.This paper is part of the Journal of Transportation.Engineering, Vol. 114, No. 6,November, 1988. ASCE, ISSN 0733-947X/88/0006-0673/$1.00 + $.15 per page. Paper No. 22902.673field for the collapsible soils. Then this information will place the engineer in a position to make a rational design decision. Collapsible soils are fre- quently encountered in an arid climate. The depositional process and for- mation of these soils, and methods for identification and evaluation of the amount of volume change that may occur, will be discussed in the following sections.COLLAPSIBLE SOILSFormation of Collapsible SoilsCollapsible soils have high void ratios and low densities and are typically cohesionless or only slightly cohesive. In an arid climate, evaporation greatly exceeds rainfall. Consequently, only the near-surface soils become wettedfrom normal rainfall. It is the combination of the depositional process andthe climate conditions that leads to the formation of the collapsible soil. Although collapsible soils exist in nondesert regions, the dry environment in which evaporation exceeds precipitation is very favorable for the formationof the collapsible structure.As the soil dries by evaporation, capillary tension causes the remainingwater to withdraw into the soil grain interfaces, bringing with it soluble salts, clay, and silt particles. As the soil continues to dry, these salts, clays, and silts come out of solution, and "tack-weld" the larger grains together. This leads to a soil structure that has high apparent strength at its low, natural water content. However, collapse of the "cemented" structure may occurupon wetting because the bonding material weakens and softens, and the soilis unstable at any stress level that exceeds that at which the soil had been previously wetted. Thus, if the amount of water made available to the soilis increased above that which naturally exists, collapse can occur at fairly low levels of stress, equivalent only to overburden soil pressure. Additional loads, such as traffic loading or the presence of a bridge structure, add to the collapse, especially of shallow collapsible soil. The triggering mechanism for collapse, however, is the addition of water.Highway Problems Resulting from Collapsible SoilsNonuniform collapse can result from either a nonhomogeneous subgradedeposit in which differing degrees of collapse potential exist and/or fromnonuniform wetting of subgrade materials. When differential collapse of subgrade soils occurs, the result is a rough, wavy surface, and potentially many miles of extensively damaged highway. There have been several re-ported cases for which differential collapse has been cited as the cause of roadway or highway bridge distress. A few of these in the Arizona and New Mexico region include sections of 1-10 near Benson, Arizona, and sectionsof 1-25 in the vicinity of Algadonas, New Mexico (Lovelace et al. 1982; Russman 1987). In addition to the excessive waviness of the roadway sur-face, bridge foundations failures, such as the Steins Pass Highway bridge,1-10, in Arizona, have frequently been identified with collapse of foundation soils.Identification of Collapsible SoilsThere have been many techniques proposed for identifying a collapsiblesoil problem. These methods range from qualitative index tests conducted on674disturbed samples, to response to wetting tests conducted on relatively un- disturbed samples, to in situ meausrement techniques. In all cases, the en- gineer must first know if the soils may become wetted to a water contentabove their natural moisture state, and if so, what the extent of the potential wetted zone will be. Most methods for identifying collapsible soils are only qualitative in nature, providing no information on the magnitude of the col- lapse strain potential. These qualitative methods are based on various func- tions of dry density, moisture content, void ratio, specific gravity, and At- terberg limits.In situ measurement methods appear promising in some cases, in that many researchers feel that sample disturbance is greatly reduced, and that a more nearly quantitative measure of collapse potential is obtainable. However,in situ test methods for collapsible soils typically suffer from the deficien- cy of an unknown extent and degree of wetting during the field test. This makes a quantitative measurement difficult because the zone of materialbeing influenced is not well-known, and, therefore, the actual strains, in- duced by the addition of stress and water, are not well-known. In addition,the degree of saturation achieved in the field test is variable and usually unknown.Based on recently conducted research, it appears that the most reliablemethod for identifying a collapsible soil problem is to obtain the best quality undisturbed sample possible and to subject this sample to a response to wet- ting test in the laboratory. The results of a simple oedometer test will indicate whether the soil is collapsible and, at the same time, give a direct measureof the amount of collapse strain potential that may occur in the field. Potential problems associated with the direct sampling method include sample distur-bance and the possibility that the degree of saturation achieved in the field will be less than that achieved in the laboratory test.The quality of an undisturbed sample is related most strongly to the arearatio of the tube that is used for sample collection. The area ratio is a measure of the ratio of the cross-sectional area of the sample collected to the cross- sectional area of the sample tube. A thin-walled tube sampler by definitionhas an area ratio of about 10-15%. Although undisturbed samples are best obtained through the use of thin-walled tube samplers, it frequently occursthat these stiff, cemented collapsible soils, especially those containing gravel, cannot be sampled unless a tube with a much thicker wall is used. Samplers having an area ratio as great as 56% are commonly used for Arizona col-lapsible soils. Further, it may take considerable hammering of the tube todrive the sample. The result is, of course, some degree of sample distur- bance, broken.bonds, densification, and a correspondingly reduced collapse measured upon laboratory testing. However, for collapsible soils, which are compressive by definition, the insertion of the sample tube leads to local shear failure at the base of the cutting edge, and, therefore, there is less sample disturbance than would be expected for soils that exhibit general shear failure (i.e., saturated clays or dilative soils). Results of an ongoing study of sample disturbance for collapsible soils indicate that block samples some- times exhibit somewhat higher collapse strains compared to thick-walled tube samples. Block samples are usually assumed to be the very best obtainable undisturbed samples, although they are frequently difficult-to-impossible to obtain, especially at substantial depths. The overall effect of sample distur- bance is a slight underestimate of the collapse potential for the soil.675译文:湿陷性地基引起的路面问题...摘要:在干旱环境中,湿陷性土壤组成的路基材料是很常见的,干旱环境中的气候条件、沉积以与风化作用都有利于湿陷性土的形成。
- 1、下载文档前请自行甄别文档内容的完整性,平台不提供额外的编辑、内容补充、找答案等附加服务。
- 2、"仅部分预览"的文档,不可在线预览部分如存在完整性等问题,可反馈申请退款(可完整预览的文档不适用该条件!)。
- 3、如文档侵犯您的权益,请联系客服反馈,我们会尽快为您处理(人工客服工作时间:9:00-18:30)。
土木工程专业英语课文原文及对照翻译Newly compiled on November 23, 2020Civil EngineeringCivil engineering, the oldest of the engineering specialties, is the planning, design, construction, and management of the built environment. This environment includes all structures built according to scientific principles, from irrigation and drainage systems to rocket-launching facilities.土木工程学作为最老的工程技术学科,是指规划,设计,施工及对建筑环境的管理。
此处的环境包括建筑符合科学规范的所有结构,从灌溉和排水系统到火箭发射设施。
Civil engineers build roads, bridges, tunnels, dams, harbors, power plants, water and sewage systems, hospitals, schools, mass transit, and other public facilities essential to modern society and large population concentrations. They also build privately owned facilities such as airports, railroads, pipelines, skyscrapers, and other large structures designed for industrial, commercial, or residential use. In addition, civil engineers plan, design, and build complete cities and towns, and more recently have been planning and designing space platforms to house self-contained communities.土木工程师建造道路,桥梁,管道,大坝,海港,发电厂,给排水系统,医院,学校,公共交通和其他现代社会和大量人口集中地区的基础公共设施。
他们也建造私有设施,比如飞机场,铁路,管线,摩天大楼,以及其他设计用作工业,商业和住宅途径的大型结构。
此外,土木工程师还规划设计及建造完整的城市和乡镇,并且最近一直在规划设计容纳设施齐全的社区的空间平台。
The word civil derives from the Latin for citizen. In 1782, Englishman John Smeaton used the term to differentiate his nonmilitary engineering work from that of the military engineers who predominated at the time. Since then, the term civil engineering has often been used to refer to engineers who build public facilities, although the field is much broader土木一词来源于拉丁文词“公民”。
在1782年,英国人John Smeaton为了把他的非军事工程工作区别于当时占优势地位的军事工程师的工作而采用的名词。
自从那时起,土木工程学被用于提及从事公共设施建设的工程师,尽管其包含的领域更为广阔。
Scope. Because it is so broad, civil engineering is subdivided into a number of technical specialties. Depending on the type of project, the skills of many kinds of civil engineer specialists may be needed. When a project begins, the site is surveyed and mapped by civil engineers who locate utility placement—water, sewer, and power lines. Geotechnical specialists perform soil experiments to determine if the earth can bear the weight of the project. Environmental specialists study the project’s impact on the local area: the potential for air andgroundwater pollution, the project’s impact on local animal and plant life, and how the project can be designed to meet government requirements aimed at protecting the environment. Transportation specialists determine what kind of facilities are needed to ease the burden on local roads and other transportation networks that will result from the completed project. Meanwhile, structural specialists use preliminary data to make detailed designs, plans, and specifications for the project. Supervising and coordinating the work of these civil engineer specialists, from beginning to end of the project, are the construction management specialists. Based on information supplies by the other specialists, construction management civil engineers estimate quantities and costs of materials and labor, schedule all work, order materials and equipment for the job, hire contractors and subcontractors, and perform other supervisory work to ensure the project is completed on time and as specified.领域。
因为包含范围太广,土木工程学又被细分为大量的技术专业。
不同类型的工程需要多种不同土木工程专业技术。
一个项目开始的时候,土木工程师要对场地进行测绘,定位有用的布置,如地下水水位,下水道,和电力线。
岩土工程专家则进行土力学试验以确定土壤能否承受工程荷载。
环境工程专家研究工程对当地的影响,包括对空气和地下水的可能污染,对当地动植物生活的影响,以及如何让工程设计满足政府针对环境保护的需要。
交通工程专家确定必需的不同种类设施以减轻由整个工程造成的对当地公路和其他交通网络的负担。
同时,结构工程专家利用初步数据对工程作详细规划,设计和说明。
从项目开始到结束,对这些土木工程专家的工作进行监督和调配的则是施工管理专家。
根据其他专家所提供的信息,施工管理专家计算材料和人工的数量和花费,所有工作的进度表,订购工作所需要的材料和设备,雇佣承包商和分包商,还要做些额外的监督工作以确保工程能按时按质完成。
Throughout any given project, civil engineers make extensive use of computers. Computers are used to design the project’s various elements (computer-aided design, or CAD) and to manage it. Computers are necessity for the modern civil engineer because they permit the engineer to efficiently handle the large quantities of data needed in determining the best way to construct a project.贯穿任何给定项目,土木工程师都需要大量使用计算机。
计算机用于设计工程中使用的多数元件(即计算机辅助设计,或者CAD)并对其进行管理。
计算机成为了现代土木工程师的必备品,因为它使得工程师能有效地掌控所需的大量数据从而确定建造一项工程的最佳方法。
Structural engineering. In this specialty, civil engineers plan and design structures of all types, including bridge, dams, power plants, supports for equipment, special structures for offshore projects, the United States space program, transmission towers, giant astronomical and radio telescopes, and many other kinds of projects. Using computers, structural engineers determine the forces a structure must resist: its own weight, wind and hurricane forces, temperature changes that expand or contract construction materials, and earthquakes. They also determine the combination of appropriate materials: steel, concrete, plastic, asphalt, brick, aluminum, or other construction materials.结构工程学。