铸造Al-50Si合金组织和性能变化
金相组织AlSi相图
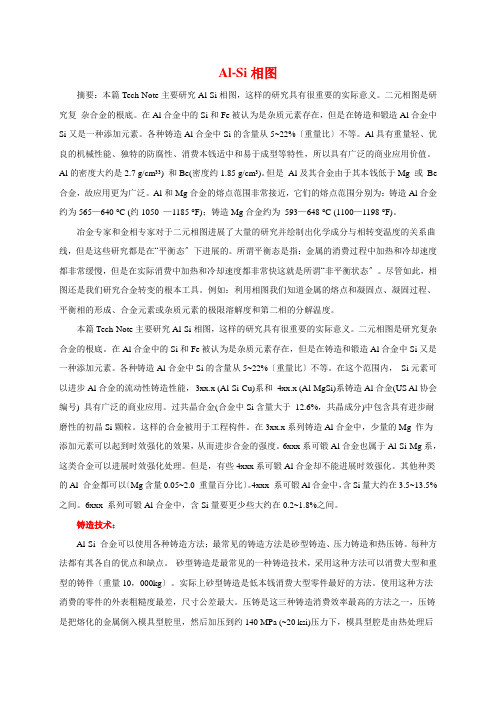
Al-Si相图摘要:本篇Tech-Note主要研究Al-Si相图,这样的研究具有很重要的实际意义。
二元相图是研究复杂合金的根底。
在Al合金中的Si和Fe被认为是杂质元素存在,但是在铸造和锻造Al合金中Si又是一种添加元素。
各种铸造Al合金中Si的含量从5~22%〔重量比〕不等。
Al具有重量轻、优良的机械性能、独特的防腐性、消费本钱适中和易于成型等特性,所以具有广泛的商业应用价值。
Al的密度大约是2.7 g/cm33) 和Be(密度约1.85 g/cm3)。
但是Al及其合金由于其本钱低于Mg 或Be 合金,故应用更为广泛。
Al和Mg合金的熔点范围非常接近,它们的熔点范围分别为:铸造Al合金约为565—640 °C (约1050 —1185 °F);铸造Mg合金约为593—648 °C (1100—1198 °F)。
冶金专家和金相专家对于二元相图进展了大量的研究并绘制出化学成分与相转变温度的关系曲线,但是这些研究都是在“平衡态〞下进展的。
所谓平衡态是指:金属的消费过程中加热和冷却速度都非常缓慢,但是在实际消费中加热和冷却速度都非常快这就是所谓“非平衡状态〞。
尽管如此,相图还是我们研究合金转变的根本工具。
例如:利用相图我们知道金属的熔点和凝固点、凝固过程、平衡相的形成、合金元素或杂质元素的极限溶解度和第二相的分解温度。
本篇Tech-Note主要研究Al-Si相图,这样的研究具有很重要的实际意义。
二元相图是研究复杂合金的根底。
在Al合金中的Si和Fe被认为是杂质元素存在,但是在铸造和锻造Al合金中Si又是一种添加元素。
各种铸造Al合金中Si的含量从5~22%〔重量比〕不等。
在这个范围内,Si元素可以进步Al合金的流动性铸造性能,3xx.x (Al-Si-Cu)系和4xx.x (Al-MgSi)系铸造Al合金(US Al协会编号) 具有广泛的商业应用。
过共晶合金(合金中Si含量大于12.6%,共晶成分)中包含具有进步耐磨性的初晶Si颗粒。
高强韧铸造铝合金材料
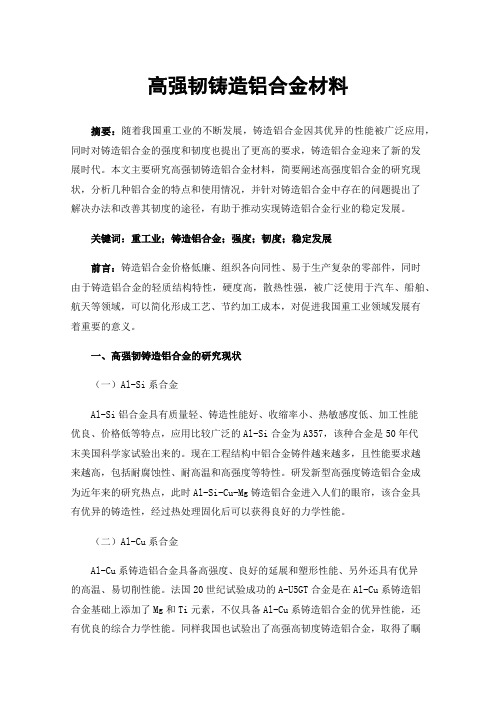
高强韧铸造铝合金材料摘要:随着我国重工业的不断发展,铸造铝合金因其优异的性能被广泛应用,同时对铸造铝合金的强度和韧度也提出了更高的要求,铸造铝合金迎来了新的发展时代。
本文主要研究高强韧铸造铝合金材料,简要阐述高强度铝合金的研究现状,分析几种铝合金的特点和使用情况,并针对铸造铝合金中存在的问题提出了解决办法和改善其韧度的途径,有助于推动实现铸造铝合金行业的稳定发展。
关键词:重工业;铸造铝合金;强度;韧度;稳定发展前言:铸造铝合金价格低廉、组织各向同性、易于生产复杂的零部件,同时由于铸造铝合金的轻质结构特性,硬度高,散热性强,被广泛使用于汽车、船舶、航天等领域,可以简化形成工艺、节约加工成本,对促进我国重工业领域发展有着重要的意义。
一、高强韧铸造铝合金的研究现状(一)Al-Si系合金Al-Si铝合金具有质量轻、铸造性能好、收缩率小、热敏感度低、加工性能优良、价格低等特点,应用比较广泛的Al-Si合金为A357,该种合金是50年代末美国科学家试验出来的。
现在工程结构中铝合金铸件越来越多,且性能要求越来越高,包括耐腐蚀性、耐高温和高强度等特性。
研发新型高强度铸造铝合金成为近年来的研究热点,此时Al-Si-Cu-Mg铸造铝合金进入人们的眼帘,该合金具有优异的铸造性,经过热处理固化后可以获得良好的力学性能。
(二)Al-Cu系合金Al-Cu系铸造铝合金具备高强度、良好的延展和塑形性能、另外还具有优异的高温、易切削性能。
法国20世纪试验成功的A-U5GT合金是在Al-Cu系铸造铝合金基础上添加了Mg和Ti元素,不仅具备Al-Cu系铸造铝合金的优异性能,还有优良的综合力学性能。
同样我国也试验出了高强高韧度铸造铝合金,取得了瞩目的成就。
我国于20世纪70年代末试验出ZL205A合金,该种合金在常态下就有具备良好的抗压和延展性能,是目前世界上强度最高的铸造铝合金,同时具有非常优越的塑形能力、韧性、抗应力腐蚀性和易于焊接等特点,因此该种合金被广泛应用于航空航天领域,用于制造各种零部件,使用效果良好。
工程材料实验指导书(附参考答案)西南交通大学
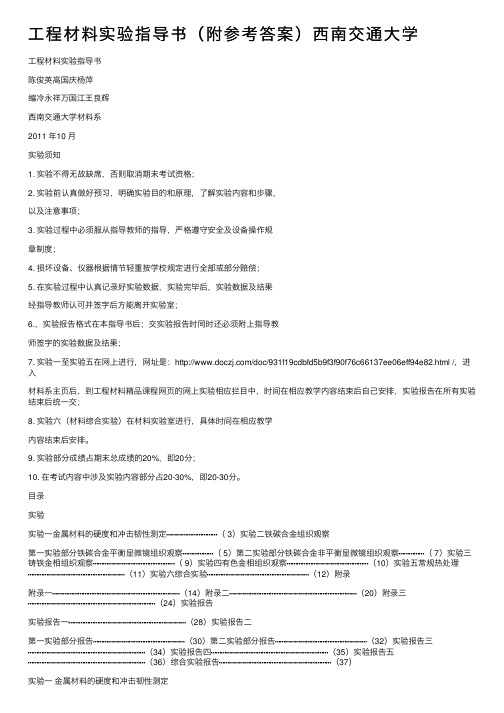
⼯程材料实验指导书(附参考答案)西南交通⼤学⼯程材料实验指导书陈俊英⾼国庆杨萍编冷永祥万国江王良辉西南交通⼤学材料系2011 年10 ⽉实验须知1. 实验不得⽆故缺席,否则取消期未考试资格;2. 实验前认真做好预习,明确实验⽬的和原理,了解实验内容和步骤,以及注意事项;3. 实验过程中必须服从指导教师的指导,严格遵守安全及设备操作规章制度;4. 损坏设备、仪器根据情节轻重按学校规定进⾏全部或部分赔偿;5. 在实验过程中认真记录好实验数据,实验完毕后,实验数据及结果经指导教师认可并签字后⽅能离开实验室;6.,实验报告格式在本指导书后;交实验报告时同时还必须附上指导教师签字的实验数据及结果;7. 实验⼀⾄实验五在⽹上进⾏,⽹址是:/doc/931f19cdbfd5b9f3f90f76c66137ee06eff94e82.html /,进⼊材料系主页后,到⼯程材料精品课程⽹页的⽹上实验相应拦⽬中,时间在相应教学内容结束后⾃⼰安排,实验报告在所有实验结束后统⼀交;8. 实验六(材料综合实验)在材料实验室进⾏,具体时间在相应教学内容结束后安排。
9. 实验部分成绩占期末总成绩的20%,即20分;10. 在考试内容中涉及实验内容部分占20-30%,即20-30分。
⽬录实验实验⼀⾦属材料的硬度和冲击韧性测定┅┅┅┅┅┅┅┅┅┅( 3)实验⼆铁碳合⾦组织观察第⼀实验部分铁碳合⾦平衡显微镜组织观察┅┅┅┅┅┅( 5)第⼆实验部分铁碳合⾦⾮平衡显微镜组织观察┅┅┅┅┅( 7)实验三铸铁⾦相组织观察┅┅┅┅┅┅┅┅┅┅┅┅┅┅┅┅( 9)实验四有⾊⾦相组织观察┅┅┅┅┅┅┅┅┅┅┅┅┅┅┅┅(10)实验五常规热处理┅┅┅┅┅┅┅┅┅┅┅┅┅┅┅┅┅┅┅(11)实验六综合实验┅┅┅┅┅┅┅┅┅┅┅┅┅┅┅┅┅┅┅┅(12)附录附录⼀┅┅┅┅┅┅┅┅┅┅┅┅┅┅┅┅┅┅┅┅┅┅┅┅┅(14)附录⼆┅┅┅┅┅┅┅┅┅┅┅┅┅┅┅┅┅┅┅┅┅┅┅┅┅(20)附录三┅┅┅┅┅┅┅┅┅┅┅┅┅┅┅┅┅┅┅┅┅┅┅┅┅(24)实验报告实验报告⼀┅┅┅┅┅┅┅┅┅┅┅┅┅┅┅┅┅┅┅┅┅┅┅(28)实验报告⼆第⼀实验部分报告┅┅┅┅┅┅┅┅┅┅┅┅┅┅┅┅┅┅(30)第⼆实验部分报告┅┅┅┅┅┅┅┅┅┅┅┅┅┅┅┅┅┅(32)实验报告三┅┅┅┅┅┅┅┅┅┅┅┅┅┅┅┅┅┅┅┅┅┅┅(34)实验报告四┅┅┅┅┅┅┅┅┅┅┅┅┅┅┅┅┅┅┅┅┅┅┅(35)实验报告五┅┅┅┅┅┅┅┅┅┅┅┅┅┅┅┅┅┅┅┅┅┅┅(36)综合实验报告┅┅┅┅┅┅┅┅┅┅┅┅┅┅┅┅┅┅┅┅┅┅(37)实验⼀⾦属材料的硬度和冲击韧性测定⼀、实验⽬的1. 了解材料硬度测定原理及⽅法;2. 了解布⽒和洛⽒硬度的测量范围及其测量步骤和⽅法;3. 了解显微硬度的测量范围及⽅法;4. 了解冲击韧性设备的测定原理、⽅法;5. 了解脆性、韧性材料冲击后的断⼝及冲击值的区别。
铸造铝合金热处理质量缺陷及其消除与预防
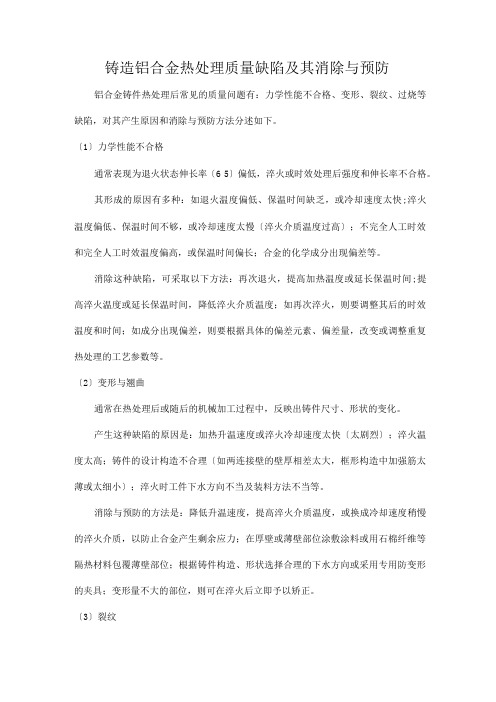
铸造铝合金热处理质量缺陷及其消除与预防铝合金铸件热处理后常见的质量问题有:力学性能不合格、变形、裂纹、过烧等缺陷,对其产生原因和消除与预防方法分述如下。
〔1〕力学性能不合格通常表现为退火状态伸长率〔6 5〕偏低,淬火或时效处理后强度和伸长率不合格。
其形成的原因有多种:如退火温度偏低、保温时间缺乏,或冷却速度太快;淬火温度偏低、保温时间不够,或冷却速度太慢〔淬火介质温度过高〕;不完全人工时效和完全人工时效温度偏高,或保温时间偏长;合金的化学成分出现偏差等。
消除这种缺陷,可采取以下方法:再次退火,提高加热温度或延长保温时间;提高淬火温度或延长保温时间,降低淬火介质温度;如再次淬火,则要调整其后的时效温度和时间;如成分出现偏差,则要根据具体的偏差元素、偏差量,改变或调整重复热处理的工艺参数等。
〔2〕变形与翘曲通常在热处理后或随后的机械加工过程中,反映出铸件尺寸、形状的变化。
产生这种缺陷的原因是:加热升温速度或淬火冷却速度太快〔太剧烈〕;淬火温度太高;铸件的设计构造不合理〔如两连接壁的壁厚相差太大,框形构造中加强筋太薄或太细小〕;淬火时工件下水方向不当及装料方法不当等。
消除与预防的方法是:降低升温速度,提高淬火介质温度,或换成冷却速度稍慢的淬火介质,以防止合金产生剩余应力;在厚壁或薄壁部位涂敷涂料或用石棉纤维等隔热材料包覆薄壁部位;根据铸件构造、形状选择合理的下水方向或采用专用防变形的夹具;变形量不大的部位,则可在淬火后立即予以矫正。
〔3〕裂纹表现为淬火后的铸件外表用肉眼可以看到明显的裂纹,或通过荧光检查肉眼看不见的微细裂纹。
裂纹多曲折不直并呈暗灰色。
产生裂纹的原因是:加热速度太快,淬火时冷却太快〔淬火温度过高或淬火介质温度过低,或淬火介质冷却速度太快〕;铸件构造设计不合理〔两连接壁壁厚差太大,框形件中间的加强筋太薄或太细小〕;装炉方法不当或下水方向不对;炉温不均匀,使铸件温度不均匀等。
消除与预防的方法是:减慢升温速度或采取等温淬火工艺;提高淬火介质温度或换成冷却速度慢的淬火介质;在壁厚或薄壁部位涂敷涂料或在薄壁部位包覆石棉等隔热材料;采用专用防开裂的淬火夹具,并选择正确的下水方向。
铸造铝合金力学性能
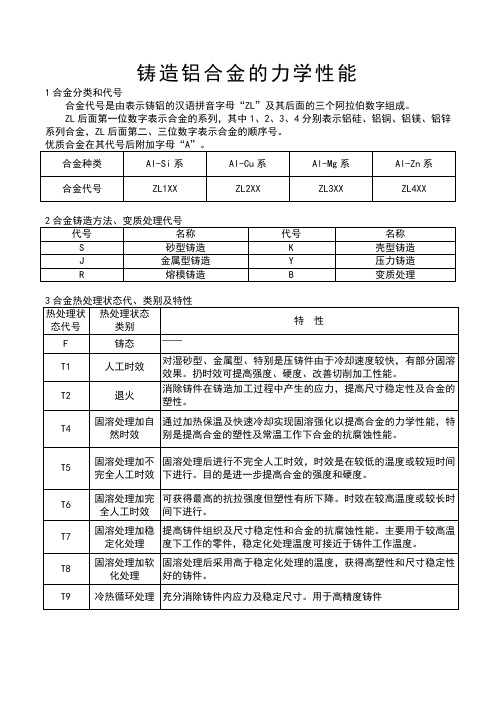
SB
F
165
2
65
SB
T6
245
2
90
J
F
195
2
70
J
T6
275
100
ZAISi12Cu2Mgl
ZL108
J
Tl
195
——
85
J
T6
255
——
90
ZAISi12CulMgINil
ZL109
J
T1
195
90
J
T6
245
——
100
ZAISi5Cu6Mg
ZL110
S
F
125
——
80
J
F
155
——
铸造铝合金的力学性能
1合金分类和代号
合金代号是由表示铸铝的汉语拼音字母“ZL”及其后面的三个阿拉伯数字组成。
ZL后面第一位数字表示合金的系列,其中1、2、3、4分别表示铝硅、铝铜、铝镁、铝锌系列合金,ZL后面第二、三位数字表示合金的顺序号。
优质合金在其代号后附加字母“A”。
合金种类
Al-Si系
Al-Cu系
T2
退火
消除铸件在铸造加工过程中产生的应力,提高尺寸稳定性及合金的塑性。
T4
固溶处理加自然时效
通过加热保温及快速冷却实现固溶强化以提高合金的力学性能,特别是提高合金的塑性及常温工作下合金的抗腐蚀性能。
T5
固溶处理加不完全人工时效
固溶处理后进行不完全人工时效,时效是在较低的温度或较短时间下进行。目的是进一步提高合金的强度和硬度。
T9
冷热循环处理
充分消除铸件内应力及稳定尺寸。用于高精度铸件
Al-Si合金液态模锻工艺研究的开题报告

Al-Si合金液态模锻工艺研究的开题报告一、选题背景随着航空航天、汽车、电子等行业的发展,对于轻量化、高强度、高可靠性材料的需求日益增加。
因此,作为一种轻质、高强度、耐腐蚀性好的材料,Al-Si合金被广泛应用于制造零件和结构件中。
其中,液态模锻技术作为一种高效、精密的成形方法,对于Al-Si合金的制造具有重要的意义。
二、研究目的本研究旨在探究Al-Si合金的液态模锻工艺,包括铸造工艺、熔化工艺、液态模锻过程中的参数控制等方面,为提高Al-Si合金的制造质量和效率提供科学依据。
三、研究内容(1)Al-Si合金熔炼工艺的研究:探讨熔炼条件和工艺对于合金组织和性能的影响,确定最佳的熔炼工艺。
(2)液态模锻工艺参数的研究:通过模锻试验,分析模锻参数对于Al-Si合金组织和性能的影响,确定最佳的液态模锻工艺参数。
(3)模锻试验及组织分析:对于模锻试验的样品进行分析,探究液态模锻工艺对于Al-Si合金组织和性能的影响。
(4)研究工作总结和展望:总结本研究的实验结果,提出进一步研究的展望和设想。
四、研究方法(1)实验研究方法:通过实验研究的方式探究Al-Si合金的液态模锻工艺,包括铸造工艺、熔化工艺、液态模锻过程中的参数控制等方面。
(2)组织分析方法:通过金相显微镜、扫描电镜等方法对Al-Si合金的组织进行分析,并进行定量分析和统计。
五、预期成果通过本研究的实验和分析,预计可以得到以下成果:(1)探讨熔炼工艺和液态模锻参数对Al-Si合金组织和性能的影响。
(2)确定优化的Al-Si合金液态模锻工艺参数。
(3)获得Al-Si合金的优化组织结构,提高合金的性能。
(4)为提高Al-Si合金的制造质量和效率提供科学依据。
六、研究进度安排阶段一:对Al-Si合金液态模锻工艺进行文献调研和理论研究。
阶段二:进行Al-Si合金的熔炼试验和液态模锻试验。
阶段三:对实验结果进行组织分析并进行定量分析和统计。
阶段四:总结研究工作成果并进行展望和设想。
Al-Si系铸造高强度铝合金的制备技术研究 (1)
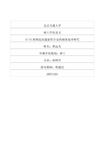
北京交通大学硕士学位论文Al-Si系铸造高强度铝合金的制备技术研究姓名:詹远光申请学位级别:硕士专业:材料学指导教师:韩建民20071201图1.1日本高速列车轻量化试验结果Fig.1.1WeightreductiontestresultsofJapanesehigh-speedtrain铝合金的比强度与合金结构钢相当,某些铝合金的强度甚至高于普通结构钢,并已生产出抗拉强度超过600MPa的超高强度高韧铝合金材料。
虽然某些铝合金在200℃~260℃温度下仍然能保持良好的强度.但在高温下,多数铝合金的强度呈大幅下降趋势,然而在摄氏零度以下,随着温度降低,铝及铝合金材料的强度反而会增加,因而能够作为优良的低温金属材料。
铝合金具有很高的抗腐蚀性,且北京交通人学坝I.学位论文2试验内容及Ⅳf究方法图2.14.Skw电阻炉及真空调压设备Ftga.14.Skwelectricalresistancefurnaceandvagunmadjustablecastingequipment2.1.4合金的熔炼工艺合金的熔炼过程按如下步骤进行:(1)烘烤吸管。
先将吸管用耐火材料压紧,然后放入吸管烘烤炉中进行预热。
以备铝料熔化后吸铸用。
(2)升温化料。
在吸管加热半个小时后,将铝料放入预先刷好涂料的坩埚内,将坩埚抽真空,在真空环境下把铝料加热至浇注温度。
(3)搅拌除气和扒渣。
在熔体温度达到浇注温度后,要定期用铌制搅拌杆进行搅拌除气,同时抽真空。
在浇注之前要扒渣数次。
除去氧化皮和杂质.2.2合金液态质量控制合金的熔体质量一般包含三方面的内容:熔体温度、熔体成分和熔体结构,它们对金属和合金的凝固组织与性能有重要的影响。
本试验采用实验室自主开发的一套搅拌系统来进行适当搅拌,使各种合金元素尽量均匀的分布于铝液中,保证熔体成分的均匀性,并促进熔体合金中的气体析出。
(1)合金熔体温度对合金凝固过程中组织形成及各类缺陷的控制有重要的影响。
的Al-Si合金表面张力及组织的研究

毕业设计(论文)含Si3%-8%的Al-Si合金表面张力及组织的研究学生姓名王培鑫学号 08070220专业班级冶金(2)班指导教师张胜全提交日期2012-6兰州理工大学技术工程学院摘要表面张力是液体表面层由于分子引力不均衡而产生的沿表面作用于任一界面上的张力。
液态合金表面张力是液态金属的重要参数之一,它是晶体生长、凝固过程模拟和铸造合金品质预测的关键因素,高温熔体表面现象在冶金、化工、熔盐和材料科学等领域十分普遍。
目前测量表面张力的方法有静态法和动态法。
根据实验条件和各种方法的特点,应用静滴法原理采用封闭管式炉两端通入氮气保护和金不被氧化的办法测出铝硅合金的表面张力,结合合金相图及硬度综合分析得出:铋即能细化铝合金组织又能够降低合金表面张力。
硅对合金组织细化和表面张力降低效果不明显。
组织细化和硅含量增加使铝合金硬度增加。
关键字:表面张力,铝硅合金,组织性能IABSTRACTThe surface tension is the tension of the liquid surface layer along the surface of molecular attraction is not balanced in any of the interface. The surface tension of the liquid alloy is one of the important parameters of the liquid metal, it is crystal growth, solidification simulation and a key factor in casting alloy quality forecast, high-temperature melt surface phenomenon is very common in areas such as metallurgy, chemicals, molten salt, and materials science. Static method and dynamic method for measuring surface tension. Under the experimental conditions and the characteristics of a variety of methods, application of intravenous infusion of the principle of the closed tube furnace at both ends into the protection of nitrogen and gold is not oxidized way to measure the surface tension of Al-Si alloy, combined with the alloy phase diagram and hardness analysis obtained: bismuth can refine aluminum alloy to reduce the surface tension. Silicon alloy microstructure refinement and surface tension reducing effect is not obvious. Microstructure refinement and silicon content aluminum alloy hardness increase.KEY WORDS:Surface tension,Al-Si alloys, microstructure and properties目录摘要 (Ⅰ)ABSTRATE (Ⅱ)1文献综述 (1)1.1引言 (1)1.2表面张力概述 (1)1.3测定高温熔体常用方法 (2)1.3.1动态法 (2)1.3.2静态法 (2)1.3.2.1最大气泡法 (2)1.3.2.2拉筒法 (3)1.3.2.3滴外形法 (5)1.3.2.4毛细管上升法 (7)1.4表面张力在各领域中的重要性 (8)1.4.1表面张力在铸造中的作用 (8)1.4.2表面张力在熔盐中的体现 (8)1.4.3表面张力在高温熔体中的体现 (8)1.4.4表面张力在高表面能固体中的体现 (8)1.4.5表面张力在液态金属中的体现 (9)1.5国内外研究现状 (9)1.5.1国内动态 (9)1.5.2国外动态 (10)1.6铝合金的分类﹑性能及用途 (10)1.6.1铝合金的分类与标示 (10)1.6.2铝合金的用途 (11)1.6.3铝合金的用途 (11)1.7金属元素对合金组织性能的影响 (11)1.7.1铋对铝硅合金稳定性的影响 (11)1.7.2铋对铝硅合金耐磨性的影响 (12)1.7.3铋对铝硅合金组织的影响 (12)1.7.4铋对锌铝合金性能的影响 (12)1.8展望 (12)2实验过程 (13)2.1实验准备 (13)2.1.1实验仪器 (13)2.1.2主要设备简介 (13)2.1.3实验材料 (14)2.2实验流程 (14)2.2.1实验流程 (14)2.2.2实验装置图 (14)2.2.3实验过程介绍 (14)3实验结果及讨论 (16)3.1实验原理 (16)3.2实验照片的处理方法 (16)3.2.1绘制与铝合金熔滴外形一致的椭圆曲线 (16)3.2.2铝合金熔滴水平截面特征数值和体积的获取 (17)3.2.3电脑处理铝合金熔滴照片实况记录 (18)3.3实验结果及分析 (19)3.3.1铝合金金相分析 (19)3.3.2铝合金硬度分析 (22)3.3.3铝合金表面张力分析 (23)4结论 (25)参考文献 (26)外文文献 (28)中文翻译 (42)致谢 (57)1文献综述1.1引言表面张力就是界面张力,通常将物体表面单位长度作用的力称为表面张力,它是合金熔化、熔体处理、凝固等过程中气相和固相、气相和液相、固相和液相的重要物理性质参数之一,是液体表面层由于分子引力不均衡而产生的沿表面作用于任一界线上的张力。
- 1、下载文档前请自行甄别文档内容的完整性,平台不提供额外的编辑、内容补充、找答案等附加服务。
- 2、"仅部分预览"的文档,不可在线预览部分如存在完整性等问题,可反馈申请退款(可完整预览的文档不适用该条件!)。
- 3、如文档侵犯您的权益,请联系客服反馈,我们会尽快为您处理(人工客服工作时间:9:00-18:30)。
Evolution of microstructure and mechanical propertiesof as-cast Al-50Si alloy due to heat treatment and P modifiercontentFuyang Cao a ,Yandong Jia a ,b ,Konda Gokuldoss Prashanth b ,Pan Ma a ,b ,Jingshun Liu a ,c ,Sergio Scudino b ,Feng Huang a ,d ,Jürgen Eckert b ,e ,Jianfei Sun a ,⇑aSchool of Materials Science and Engineering,Harbin Institute of Technology,Harbin 150001,China bIFW Dresden,Institute for Complex Materials,P.O.Box 270116,D-01171Dresden,Germany cSchool of Materials Science and Engineering,Inner Mongolia University of Technology,Hohhot 010051,China dHubei Key Laboratory of Advanced Technology of Automobile Parts,School of Automotive Engineering,Wuhan University of Technology,430070,China eTU Dresden,Institut für Werkstoffwissenschaft,D-01062Dresden,Germanya r t i c l e i n f o Article history:Received 12September 2014Revised 4March 2015Accepted 7March 2015Available online 9March 2015Keywords:Al–50Si alloySuperheat treatment MicrostructureMechanical propertya b s t r a c tThe effects of superheat temperature,content of modifier (P)and T6heat treatment on the microstruc-ture and mechanical properties of the Al–50Si alloy have been investigated systematically by scanning electron microscopy (SEM)and differential scanning calorimetry (DSC).The results indicate that the pri-mary Si exhibits a plate-like morphology,with average size decreasing with increasing of the superheat temperature for the unmodified alloy.The morphology of primary Si changes to small blocky shape at an optimal P content of 0.5wt.%,and the nucleation temperature increases for the alloy with 1.3wt.%P because of the ease of formation of the AlP phase.The nucleation temperature is lower for 0.5wt.%P due to lack of P atoms at relatively higher temperature.The ultimate tensile strength was enhanced by the addition of P followed by the T6heat treatment,and the maximum ultimate tensile strength ($160MPa)was observed for the sample containing 0.5wt.%P.Ó2015Elsevier Ltd.All rights reserved.1.IntroductionElectronic packaging materials are required to protect the elec-tronic components from physical damage,mechanical forces,atmospheric chemical contamination,etc.[1].As the electronic packaging requires increasingly smaller size,lighter weight and higher integration,new packaging materials have to be developed to improve the performance of electronic components.However,the properties of traditional packaging materials can no longer sat-isfy the practical requirements [2–4].Hypereutectic Al–Si alloys with high Si content (50–70wt.%)are one of the ideal candidates for electronic packaging application as a result of the positive combination of properties,such as relatively low coefficient of thermal expansion (CTE),which closely matches that of GaAs or Si semiconductor materials,high thermal conductivity,low density and superior strength [5].However,the main limitation of this type of material is the presence of the coarse,irregular,and brittle primary Si phase that can act as soft spots for premature crack initiation,deteriorating the overall mechanical properties of these materials [6,7].Therefore,it is essential to modify the microstructure of hypereutectic Al–Si alloy to optimize the mor-phology and distribution of the primary and eutectic Si [8,9].Efforts have been made to modify the microstructure of hypereutectic Al–Si cast alloys in order to achieve a refined Si phase with beneficial shape and distribution [10–12].For example,Liu et al.[13]have investigated the modification of hypereutectic Al–24%Si alloys with Si–P,which leads to the formation of primary Si with size of 19l m.Choi et al.[14]have reported that the mor-phology of primary Si in hypereutectic Al–20%Si alloy can be modi-fied from star-like to the polygon or blocky shape by the addition of c -Al 2O 3nanoparticles.Moreover,the spray forming technology was also used to prepare the Al–35%Si alloy with size of the Si phase less than10l m [15].However,only little attention has been paid to the modification of Al–Si alloys with high Si contents (e.g.50wt.%).The present study analyzes this mentioned above aspect by examining the potential of different superheat temperatures and phosphorus contents as modifying agents for simultaneous refine-ment of both the size and morphology of primary Si phase in the as-cast Al–50Si alloy.Additionally,the work investigates the effect of the induced microstructural modifications on the mechanical properties of the material./10.1016/j.matdes.2015.03.0080261-3069/Ó2015Elsevier Ltd.All rights reserved.⇑Corresponding author.E-mail address:jfsun@ (J.Sun).F.Cao et al./Materials and Design74(2015)150–1561512.Experimental details equipped with an energy dispersive X-ray(EDX)setup.Differential scanning calorimetry(DSC)measurements wereof Al–50Si alloy processed using different superheat temperatures(a)100,(b)200,(c)300,(d)400and(e)500K,(f)temperature.the nucleation and growth of primary Si [17].A higher superheat temperature is necessary to promote the dissolution and to Table 1The O,N,H contents in the samples fabricated at different superheat temperatures SEM images of Al–50Si with different concentration of the modifier:(a)0,(b)0.1,(c)0.3,(d)0.5,(e)0.7and (f)1.3152 F.Cao et al./Materials and Design 74(2015)150–156of300K is used in order to evaluate the effects of P on the microstructure and properties of the Al–50Si alloy.The microstructures of the Al–50Si alloy with different P con-tents are shown in Fig.2.The micrographs indicate substantial microstructural differences in the morphology,size and distribution of the primary Si with respect to the un-refined material(Fig.2(a)). The morphology of primary Si is still plate-like,but its average size is reduced to62.2±1.5l m for the sample with0.1wt.%P(Fig.2(b)) compared to the unmodified alloy(117±2.5l m).With increasing the P content to0.5wt.%,the average size of the primary Si decreases further to38.0±2.1l m.In addition,the morphology is no longer plate-like but blocky in shape as displayed in Fig.2(d). The average size of the primary Si grows as the P content increases to0.7wt.%and1.3wt.%,reaching values of about39.4±1.3l m and 41.2±2.3l m,respectively(Fig.2(e)and(f));yet,the size of the pri-mary Si is smaller than the unmodified alloy.AlP particles can be accordingly formedthe Al–P master alloy into the hypereutecticuseful for refining the primary Si phase.the fact that both the crystal structure andAlP(cubic structure,a=5.45Å)is similar toture,a=5.431Å),and hence,the AlP particlesnucleation sites for primary Si[20–22].Theand changes the morphology from particle shapewhen the P addition is1.3wt.%(Fig.2(f)).Thistribution and morphology of AlP wouldnucleation sites in the melt for the primarythen cause the increasing size of primaryA typical backscattered electron image ofP is shown in Fig.3along with the correspondingmaps.It can be also observed that the particlemary Si phase is rich in Al and P,which canAlP according to the elemental scanning measurementsin Fig.3(b)–(d).Fig.4shows the cooling curves of the Al–50Siof the P concentration.The nucleation temperature of the eutectic Si at about843K does not change significantly with the addition of P.On the other hand,the primary Si nucleation temperature changes with increasing P content.The nucleation temperature of primary Si is about1334K for the unmodified alloy,and the tem-perature increases by$22K with the addition of1.3wt.%P.It is reported that dissolved Si atoms may aggregate on the surface of the AlP substrates to nucleate with the cube–cube orientation relationship to have similar lattice parameters[22].During solid-ification,AlP can nucleate easily owing to a sufficient amount of P atoms in the melt with1.3wt.%P.The Si crystals tend to grow by the aggregation of Si atoms on the pre-formed primary Si crystal surfaces in accordance with the twinning plane re-entrant edge (TPRE)mechanism during the growth stage[23].Compared to the smooth surfaces of thefirstly-formed primary Si[24],the AlP parti-cles possess additional twinning planes[25].Thus the Si atoms are more inclined to aggregate on the surface of AlP rather than on the firstly-formed primary Si[26].SEM image of the primary Si in the alloy modified with0.5wt.%P and corresponding their EDX images for(b)Al,(c) Fig.4.DSC curves of the Al–50Si alloy as a function of P addition.The nucleation temperature of the primary Si decreases by about 55K in the sample with0.5wt.%P compared with the unmodified alloy.Due to the smaller amount of P atoms in the melt with 0.5wt.%P,it is difficult for the AlP phase to nucleate and a larger degree of supercooling is needed.As a result of the similar electronic structure between the Si and P atoms,the nucleation of primary Si is restricted.Therefore,primary Si nucleates after the nucleation of the AlP phase nucleation at a relatively lower temperature.In order to understand the influence of the heat treatment on the microstructure,the alloy with0.5wt.%P with ST=300K is used for the following investigation.The microstructures of Al–50Si alloy before and after heat treatment are shown in Fig.5. Compared with the as-cast alloy(Fig.5(a)),the size of primary Si increases slightly(about42.2±1.5l m)inrial,as shown in Fig.5(c).However,comparingFig.5(d)reveals that after heat treatment theSi are round and smooth,whereas large amountssolves into the Al matrix and the eutectic Sishape.The morphology variation in both primarymay have led to reduction of stress concentrationmay be beneficial for enhancing the mechanicalalloy.3.2.Mechanical propertiesThe ultimate tensile strength(UTS)ofdifferent P contents is presented in Fig.6.alloys is higher compared to the unmodifiedproperties of the hypereutectic Al–Si castlargely dependent on the primary Si characteristics morphology and distribution)[13].Accordingly,the P addition may positively influence the tensile properties of the as-cast alloys due to the refinement and modification of the primary Si.The ulti-mate tensile strength of the alloys increases with increasing the P content,reaching131MPa for the sample with0.5wt.%P,which is four times higher than that of the unmodified alloy.After T6heat treatment,the UTS of the alloy with0.5wt.%P reaches160MPa,which is$22%higher than the same alloy without heat treatment and is only32MPa less than the same alloy fabricated by rapid solidification[29].This result is consistent with the microstructural evolution reported in Fig.5showing that the Al–Si alloy can be precipitation-strengthened after T6heat treat-ment.Consequently,the T6heat treatment plays a crucial role during the fragmentation and spheroidization process of eutectic Si[30].It has been reported[31]that coarse and elongated Si par-ticles tend to fracture more frequently than spherical particles,asFig.5.SEM microstructures of Al–50Si alloy(a)and(b)before,and(c)and(d)after heat treatment.Fig. 6.Ultimate tensile strength for the Al–50Si alloys processed at differentconditions.coarse Si particles can induce high levels of stress concentration and consequently result in the reduction of UTS.Fig.7shows the morphology of the fracture surface of the unmodified,modified and heat-treated samples after room tem-perature tensile tests.The fracture surface of the unmodified alloy exhibits a brittle morphology along with a large number of sec-ondary cracks(Fig.7(a)).Figs.7(b)and(c)display the fracture sur-face of the alloy with0.5wt.%P addition and after T6heat treatment.It can be also seen that the specimen presents the typical brittle fracture,but the amount of secondary cracks decreases as compared with the unmodified alloy,and some dimples indicative of ductile fracture are also observed.4.ConclusionsThe microstructural evolution and the tensile properties of the Al–50Si alloy has been investigated in detail and the following con-clusions can be drawn as follows:(1)Primary Si phase exhibits a plate-like morphology,and itsaverage size decreases from171l m(for ST=100K)to about 103l m(for ST=500K).However,the optimal ST is observed to be300K.(2)Due to the formation of AlP particles,the primary Si phasevaries from plate-like morphology to blocky shape and a minimum size of38l m is obtained with the addition of0.5wt.%P.(3)The primary Si nucleation temperature decreases by about55K with the addition of0.5wt.%P,with further increase of the P content to 1.3wt.%,the nucleation temperature increases by about22K compared with the unmodified Al–50Si alloy.(4)The edges of the primary Si becomes round and smooth,whereas the eutectic Si transforms to spherical shape after the proper heat-treatment.(5)Both the addition of P modifier and T6heat treatment arebeneficial for the enhancement of the mechanical property of the Al–50Si alloy.The largest UTS of160MPa is obtained with the addition of0.5wt.%P and after T6heat treatment. AcknowledgmentsThis project was supported by National Natural Science Foundation of China(Grant No.51375110),the National973Plan Project of China(Grant No.2010CB631205),the Fundamental Research Funds for the Central Universities of China(WUT:2013-IV-076),and Jingshun Liu acknowledges the support from Scientific Research Foundation of Inner Mongolia University of Technology of China(Grant No.ZD201405).References[1]Q.Zhang,G.H.Wu,L.T.Jiang,G.Q.Chen,Thermal expansion and dimensionalstability of Al–Si matrix composite reinforced with high content SiC,Mater.Chem.Phys.82(2003)780–785.[2]S.C.Hogg,mbourne,A.Ogilvy,P.S.Grant,Microstructural characterisationof spray formed Si–30Al for thermal management applications,Scripta Mater.55(2006)111–114.[3]K.Yu,C.Li,R.C.Wang,J.Yang,Production and properties of a spray formed70%Si–Al alloy for electronic packaging applications,Mater.Trans.49(2008) 685–687.[4]Q.J.Jia,J.Y.Liu,Y.X.Li,W.S.Wang,Microstructure and properties of electronicpackaging box with high silicon aluminum-base alloy by semi-solid thixoforming,Trans.Nonferr.Metal.Soc.23(2013)80–85.[5]F.Wang,B.Q.Xiong,Y.A.Zhang,B.H.Zhu,H.W.Liu,Y.G.We,Microstructure,thermo-physical and mechanical properties of spray-deposited Si–30Al alloy for electronic packaging application,Mater.Charact.59(2008)1455–1457. [6]Q.L.Li,T.D.Xia,n,W.J.Zhao,L.Fan,P.F.Li,Effect of in situ c-Al2O3particles on the microstructure of hypereutectic Al–20%Si alloy,J.Alloys Compd.577(2013)232–236.[7]Y.D.Jia,F.Y.Cao,S.Scudino,P.Ma,H.C.Li,L.Yu,et al.,Microstructure andthermal expansion behavior of spray-deposited Al–50Si,Mater.Des.57(2014) 585–591.[8]Y.J.Sun,Q.L.Wang,H.R.Geng,Effects of complex modificating technique onmicrostructure and mechanical properties of hypereutectic Al–Si alloys,J.Mater.Sci.47(2012)2104–2109.Fig.7.Fracture morphology of the Al–50Si alloy in(a)unmodified,and(b)and(c)0.5wt.%P+T6heat treatment.[9]Z.J.Wei,P.Ma,H.W.Wang,C.M.Zou,S.Scudino,K.K.Song,et al.,The thermalexpansion behaviour of SiC p/Al–20Si composites solidified under high pressures,Mater.Des.65(2015)387–394.[10]L.Z.Zhao,M.J.Zhao,L.J.Song,J.Mazumder,Ultra-fine Al–Si hypereutectic alloyfabricated by direct metal deposition,Mater.Des.56(2014)542–548. [11]S.Tomida,K.Nakata,S.Shibata,I.Zenkouji,Saji,Improvement in wearresistance of hyper-eutectic Al–Si cast alloy by laser surface remelting,Surf.Coat.Technol.169–170(2003)468–471.[12]L.Zhang,G.S.Gan,B.Yang,Microstructure and property measurements onin situ TiB2/70Si–Al composite for electronic packaging applications,Mater.Des.36(2010)177–181.[13]Y.P.Wu,S.J.Wang,H.Li,X.F.Liu,A new technique to modify hypereutectic Al–24%Si alloys by a Si–P master alloy,J.Alloys Compd.477(2009)139–144. [14]H.S.Choi,H.Konishi,X.C.Li,Al2O3nanoparticles induced simultaneousrefinement and modification of primary and eutectic Si particles in hypereutectic Al–20Si alloy,Mater.Sci.Eng.A541(2012)159–165.[15]C.Cui,A.Schulz,K.Schimanski,H.W.Zoch,Spray forming of hypereutectic Al–Si alloys,J.Mater.Process.Technol.209(2009)5220–5228.[16]F.C.Robles Hernandez,W.B.Djurdjevic,W.T.Kierkus,J.H.Sokolowski,Calculation of the liquidus temperature for hypo and hypereutectic aluminum silicon alloys,Mater.Sci.Eng.A396(2005)271–276.[17]Z.W.Chen,W.Q.Jie,R.J.Zhang,Superheat treatment of Al–7Si–0.55Mg alloymelt,Mater.Lett.59(2005)2183–2185.[18]W.M.Wang,X.F.Bian,J.Y.Qin,S.I.Syliusarenko,The atomic-structure changesin Al-16pct Si alloy above the liquidus,Metall.Mater.Trans.A31(2000) 2163–2168.[19]S.Z.Du,C.C.Li,S.Y.Pang,J.F.Leng,H.R.Geng,Influences of melt superheattreatment on glass forming ability and properties of Al84Ni10La6alloy,Mater.Des.47(2013)358–364.[20]Q.Zhang,X.F.Liu,H.S.Dai,Re-formation of AlP compound in Al–Si melt,J.Alloys Compd.480(2009)376–381.[21]J.Y.Chang,I.Moon, C.S.Choi,Refinement of cast microstructure ofhypereutectic Al–Si alloys through the addition of rare earth metals,J.Mater.Sci.33(1998)5015–5023.[22]M.Zuo,D.G.Zhao,X.Y.Teng,H.R.Geng,Z.S.Zhang,Effect of P and Sr complexmodification on Si phase in hypereutectic Al–30Si alloys,Mater.Des.47(2013) 857–864.[23]K.F.Kobayashi,L.M.Hogan,The crystal growth of silicon in Al–Si alloys,J.Mater.Sci.20(1985)1961–1975.[24]C.L.Xu,H.Y.Wang,C.Liu,Q.C.Jiang,Growth of octahedral primary silicon incast hypereutectic Al–Si alloys,J.Cryst.Growth291(2006)540–547.[25]R.Y.Wang,W.H.Lu,L.M.Hogan,Growth morphology of primary silicon in castAl–Si alloys and the mechanism of concentric growth,J.Cryst.Growth207 (1999)43–54.[26]D.K.Li,M.Zuo,Q.Zhang,X.F.Liu,The investigation of continuous nucleationand refinement of primary Si in Al–30Si mushy zone,J.Alloys Compd.502 (2010)304–309.[27]M.F.Kilicaslan,W.R.Lee,T.H.Lee,Y.Sohn,S.J.Hong,Effect of Sc addition on themicrostructure and mechanical properties of as-atomized and extruded Al–20Si alloys,Mater.Lett.71(2012)164–167.[28]M.F.Kilicaslan,Effect of V addition on the nano-size spherical particlesgrowing on the Fe-bearing intermetallics and silicon phases of gas atomized hypereutectic Al–20Si–5Fe alloys,J.Alloys Compd.606(2014)86–91.[29]Sandvik Materials Technology,CE Alloy Products–Alloys and Properties,CE11</en/products/ce-alloys/product-properties/#tab-mechanical-properties>.(accessed09.09.14).[30]B.Li,H.W.Wang,J.C.Jie,Z.J.Wei,Effect of yttrium and heat treatment on themicrostructure and tensile properties of Al–7.5Si–0.5Mg alloy,Mater.Des.32 (2011)1617–1622.[31]S.J.Hong,C.Suryanarayana,Mechanical properties and fracture behavior of anultrafine-grained Al–20wt pct Si alloy,Metall.Mater.Trans.A36(2005)715–723.156 F.Cao et al./Materials and Design74(2015)150–156。