cAMP信号转导通路总结
《cAMP信号通路》课件

cAMP的作用
2
的催化水解反应完成。
cAMP主要通过激活蛋白激酶A和转录
因子CREB来发挥其作用。
3
ห้องสมุดไป่ตู้
cAMP与G蛋白偶联受体
cAMP通常与G蛋白偶联受体一起出现, 通过了解G蛋白偶联受体的结构和功 能,有助于我们更好地理解cAMP信 号通路的作用机制。
cAMP信号通路在疾病中的作用
cAMP信号通路的异常可能与多种疾病发生有关,比如肝癌、阿尔茨海默病等。对cAMP信号通路的研 究,对于相关疾病的治疗和预防有着重要意义。
cAMP的作用机制
cAMP主要通过与蛋白激酶 A(PKA)和转录因子 CREB结合,调控多种细 胞功能和生物过程的发生。
cAMP的生理功能
cAMP参与了细胞的能量代 谢、生长发育、细胞增殖 和分化、信号传导等生命 活动。
cAMP信号通路的重要性
cAMP信号通路是人类生命活动中一个重要的信号转导通路,对于多个细胞和组织发挥着重要作用。
1
cAMP信号与心血管系统
2
cAMP通过调节心肌收缩和血管舒缩,
参与调控心血管系统的功能。
3
cAMP信号与代谢调节
4
cAMP通过调节代谢途径和能量平衡, 参与调控胰岛素分泌和脂肪酸氧化等
过程。
cAMP信号与神经系统
cAMP在神经系统中参与调控神经细 胞的兴奋性和突触传递过程。
cAMP信号与免疫系统
cAMP在免疫细胞中发挥着抗炎和调 节免疫应答的重要作用。
《cAMP信号通路》PPT课 件
cAMP信号通路是细胞内的一种重要信号传递系统,通过激活蛋白激酶A和转 录因子CREB,参与多种生命活动的调控。
cAMP信号通路
细胞信号转导通路梳理
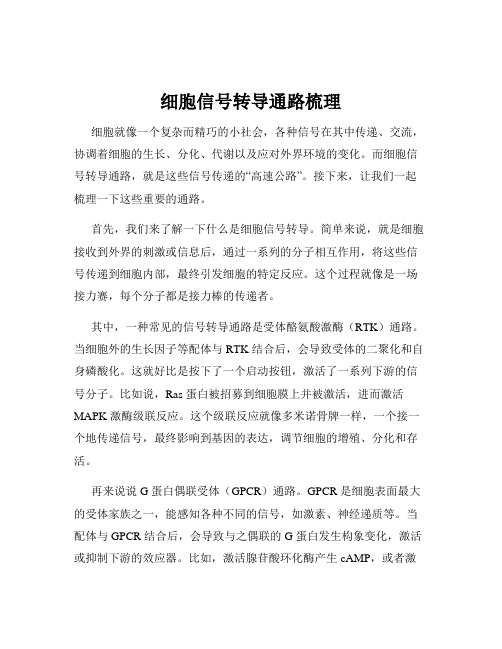
细胞信号转导通路梳理细胞就像一个复杂而精巧的小社会,各种信号在其中传递、交流,协调着细胞的生长、分化、代谢以及应对外界环境的变化。
而细胞信号转导通路,就是这些信号传递的“高速公路”。
接下来,让我们一起梳理一下这些重要的通路。
首先,我们来了解一下什么是细胞信号转导。
简单来说,就是细胞接收到外界的刺激或信息后,通过一系列的分子相互作用,将这些信号传递到细胞内部,最终引发细胞的特定反应。
这个过程就像是一场接力赛,每个分子都是接力棒的传递者。
其中,一种常见的信号转导通路是受体酪氨酸激酶(RTK)通路。
当细胞外的生长因子等配体与 RTK 结合后,会导致受体的二聚化和自身磷酸化。
这就好比是按下了一个启动按钮,激活了一系列下游的信号分子。
比如说,Ras 蛋白被招募到细胞膜上并被激活,进而激活MAPK 激酶级联反应。
这个级联反应就像多米诺骨牌一样,一个接一个地传递信号,最终影响到基因的表达,调节细胞的增殖、分化和存活。
再来说说 G 蛋白偶联受体(GPCR)通路。
GPCR 是细胞表面最大的受体家族之一,能感知各种不同的信号,如激素、神经递质等。
当配体与 GPCR 结合后,会导致与之偶联的 G 蛋白发生构象变化,激活或抑制下游的效应器。
比如,激活腺苷酸环化酶产生 cAMP,或者激活磷脂酶 C 产生 IP3 和 DAG 等第二信使。
这些第二信使进一步激活下游的蛋白激酶,从而调节细胞的各种生理过程。
另一个重要的通路是细胞内的钙离子信号通路。
钙离子在细胞内是一种非常重要的第二信使。
当细胞受到刺激时,细胞内的钙离子浓度会迅速发生变化。
比如,通过细胞膜上的钙通道或内质网中的钙释放通道,使钙离子从细胞外或内质网中释放到细胞质中。
钙离子浓度的升高会激活一系列的钙依赖蛋白激酶,从而调节细胞的收缩、分泌、基因表达等功能。
还有一种叫做核受体信号通路。
这类受体位于细胞内,当它们与配体结合后,会直接进入细胞核内调节基因的表达。
例如,糖皮质激素受体与糖皮质激素结合后,会与特定的 DNA 序列结合,调控相关基因的转录,从而影响细胞的代谢、免疫反应等。
医学细胞生物学 1 G蛋白介导的信号通路

M1 C1
M2 C2
ATP
cAMP
蛋白激酶A(protein kinase A,PKA)
cAMP分子
激酶活 性
RC RC
R
C
+
R
C
调节亚单位 催化亚单位
cAMP-调节 亚单位复合体
活化的催 化亚单位
PKA的作用
(1)调节预存蛋白的活性 (2)调节基因表达活性
PKA对预存蛋白活性的调节
DAG:在磷脂酰丝氨酸和Ca2+协同下激 活PKC
Ca2+ /钙调素(Ca2+ -CaM)
Ca2+-CaM可以激活蛋白激酶或磷酸酶,从而磷酸化相 应的底物蛋白,实现对细胞代谢活动的调节。如,环核苷酸 磷酸二脂酶(PDE)。
2. Ca2+-钙调蛋白依赖性蛋白激酶途径
激素 受体 G蛋白 PLC
IP3
Ca2+
CaM
CaM激酶
酶或功能蛋白磷酸化
生物学效应
磷脂酰肌醇信号途径:
信号分子
G蛋白偶联受体
G蛋白 磷脂酶C
PIP2 (PLC)
组胺释放 平滑肌收缩等
IP3 / Ca 2+ IP3 胞内Ca 2+↑
DG 活化PKC
DG/PKC
糖原分解 微管解体
激活蛋白激酶 或磷酸酶
活化钙调蛋白 (CaM)
底物蛋白磷酸化
分泌、肌肉收缩、
▲
细胞增殖、分化
(一)G蛋白偶联受体介导的信号转导通路 ※1.cAMP信号通路 ※2.磷脂酰肌醇信号通路
(二)催化型受体介导的信号转导通路 1.受体酪氨酸蛋白激酶信号通路
(三
磷酸化的作用
细胞内cAMP信号传导途径与心血管健康关系研究

细胞内cAMP信号传导途径与心血管健康关系研究近年来,心血管疾病已成为全球范围内最主要的健康问题之一。
细胞内cAMP信号传导途径在心血管健康中的作用备受关注。
本文旨在探讨细胞内cAMP信号传导途径与心血管健康的关系,并分析其可能的作用机制。
细胞内cAMP信号传导途径是一种重要的细胞信号传导机制,通过调节多个重要的生物学过程,对细胞的生理功能发挥着重要的影响。
在心血管系统中,细胞内cAMP信号传导途径在调控心脏收缩、松弛、心肌细胞增殖、血管平滑肌细胞舒张等方面起到关键作用。
首先,细胞内cAMP信号传导途径通过激活腺苷酸环化酶(adenylyl cyclase,AC),将AMP转化为cAMP。
cAMP通过激活蛋白激酶A(protein kinase A,PKA)进而调控多种下游效应器蛋白。
在心血管系统中,cAMP通过激活PKA调节心脏收缩和松弛。
具体来说,cAMP通过维持心肌肌浆网中的钙离子浓度平衡,促进心肌肌间盘收缩,从而增加心肌收缩力。
同时,cAMP也能够促进心肌细胞的松弛,从而有助于心脏舒张。
除了对心脏功能的调节外,cAMP信号传导途径也影响血管平滑肌细胞的舒张。
在血管平滑肌细胞中,cAMP通过激活蛋白激酶A,进而磷酸化肌球蛋白轻链,从而使血管平滑肌细胞松弛,促进血管舒张。
这一过程对于维持正常的血压以及预防动脉粥样硬化等心血管疾病的发生发展具有重要意义。
除了心脏功能和血管舒张外,细胞内cAMP信号传导途径还参与调节心肌细胞的增殖。
以心肌梗死为例,心肌细胞的损伤和死亡会导致心脏组织的重构和瘢痕形成。
cAMP信号传导途径的激活被证实能够促进心肌细胞的增殖,从而有助于心脏组织的修复。
然而,细胞内cAMP信号传导途径在心血管健康中的具体作用机制仍然需要进一步研究。
一方面,心血管疾病的发生发展是一个复杂的过程,涉及多个信号通路和因素的相互作用。
cAMP信号传导途径仅是其中之一。
另一方面,cAMP信号传导途径涉及多个下游效应器蛋白,其互作和调控关系也十分复杂。
cAMP—PKA信号转导通路在调节胎盘滋养细胞分化中的作用

激 酶 A( p r o t e i n k i n a s e A, P K A)活 性 , 然 后 磷 酸 化 c A MP反 应 元 件 结 合 蛋 白 ( c A MP r e s p o n s e e l e m e n t —
5 一环式 结 构 。胞 内 c A M P浓 度 一 般 为 1 0 ~m o l / L , 增加 细胞 内 c A MP浓 度 可 以激 活 c A MP依 赖 性 蛋 白
剂) 模 拟 HC G作 用 时 , 发现 P K A抑 制 剂 ( H一8 9 ) 可 以抑 制 8 B r —c A MP及 HC G对 缝 隙连 接 蛋 白 的刺 激 作用 E s ] 。D e l i d a k i 等 利 用 F o r s k o l i n ( c A MP激 动 剂 ) 诱导 建 立 合体 滋 养 细胞 模 型 , 发现 H C G分 泌增 强 膜 融合 基 因表达 , 从 而促 进 滋 养 细 胞 分化 融 合 , 其 中腺
酸腺 苷 ( c y c l i c a d e n o s i n e mo n o p h o s p h a t e , c A MP ) 浓度
内c A M P水平 及 P K A催化 亚 基 蛋 白的表 达 。体 外 诱 导 细胞 滋养层 细胞 分 化 融 合 为绒 毛合 体 滋 养层 细 胞 时, 起 主要作用 的是 细胞 间 的缝 隙连接及 细胞 膜之 间 的融 合 。激光 扫描 共 聚焦显微 镜 的光漂 白恢 复 ( l f u o —
分化 、 生长、 代 谢及 凋亡 过程 发生 影 响 。
一
、
c A MP— P K A 的 生 物 学 特 点
主要的信号转导途径

第三节主要的信号转导途径一、膜受体介导的信号传导(一)cAMP-蛋白激酶A途径述:该途径以靶细胞内cAMP浓度改变和激活蛋白激酶A(PKA)为主要特征,是激素调节物质代谢的主要途径。
1.cAMP的合成与分解⑴引起cAMP水平增高的胞外信号分子:胰高血糖素、肾上腺素、促肾上腺皮质激素、促甲状腺素、甲状旁腺素和加压素等。
α-GDP-βγ(Gs蛋白)激素+受体→激素-受体→↓α-GTP + βγ↓AC激活↓ATP →cAMP述:当信号分子(胰高血糖素、肾上腺素和促肾上腺皮质激素)与靶细胞质膜上的特异性受体结合,形成激素一受体复合物而激活受体。
活化的受体可催化Gs的GDP与GTP交换,导致Gs的α亚基与βγ解离,蛋白释放出αs-GTP。
αs-GTP能激活腺苷酸环化酶,催化ATP转化成cAMP,使细胞内cAMP浓度增高。
过去认为G蛋白中只有α亚基发挥作用,现知βγ复合体也可独立地作用于相应的效应物,与α亚基拮抗。
腺苷酸环化酶分布广泛,除成熟红细胞外,几乎存在于所有组织的细胞质膜上。
cAMP经磷酸二酯酶(PDE)降解成5'-AMP而失活。
cAMP是分布广泛而重要的第二信使。
⑵AC活性的抑制与cAMP浓度降低◇Gα-GTP结合AC并使之激活后,同时激活自身的GTP酶活性,Gα-GTP→Gα-GDP,Gs、AC均失活。
从而在细胞对cAMP浓度升高作出应答后AC活性迅速逆转。
⑶少数激素,如生长激素抑制素、胰岛素和抗血管紧张素II等,它们活化受体后可催化抑制性G蛋白解离,导致细胞内AC活性下降,从而降低细胞内cAMP水平。
⑷正常细胞内cAMP的平均浓度为10-6mol/L。
cAMP在细胞中的浓度除与腺苷酸环化酶活性有关外,还与磷酸二酯酶的活性有关。
举例如下:①一些激素如胰岛素,能激活磷酸二酯酶,加速cAMP降解;②某些药物如茶碱,则抑制磷酸二酯酶,促使细胞内cAMP浓度升高。
2.cAMP的作用机制――cAMP激活PKA(幻灯64)⑴cAMP对细胞的调节作用是通过激活cAMP依赖性蛋白激酶或称蛋白激酶A (PKA)系统来实现的。
细胞信号转导知识点总结归纳

细胞信号转导知识点总结归纳
第二信使(细胞内小分子信使)
如钙离子、环腺苷酸(cAMP)、环鸟苷酸(cGMP)、环腺苷二磷酸核糖、甘油二酯(DAG)、肌醇-1,4,5-三磷酸(IP3),花生四烯酸、神经酰胺、一氧化氮、一氧化碳
cAMP和cGMP的上游信号转导分子分别为腺苷酸环化酶(AC)和鸟苷酸环化酶(GC),下游分子分别为蛋白激酶A(PKA)和蛋白激酶G(PKG)。
磷脂酰肌醇激酶(PI-K)催化磷脂酰肌醇(PI)的磷酸化。
磷脂酰肌醇特异性激酶C(PLC)可将磷脂酰肌醇-4,5-二磷酸(PIP2)分解成DAG和IP3。
钙离子的下游信号转导分子为钙调蛋白,钙调蛋白本身无活性,形成Ca2+/CAM复合物后可调节钙调蛋白依赖性蛋白激酶的活性。
钙离子还可以结合PKC、AC和cAMP-PDE等多种信号转导分子。
NO可通过激活鸟苷酸环化酶、ADP-核糖转移酶和环氧化酶等传递信号。
细胞内一氧化氮合酶可催化精氨酸分解产生瓜氨酸和NO。
camp试验的原理和应用
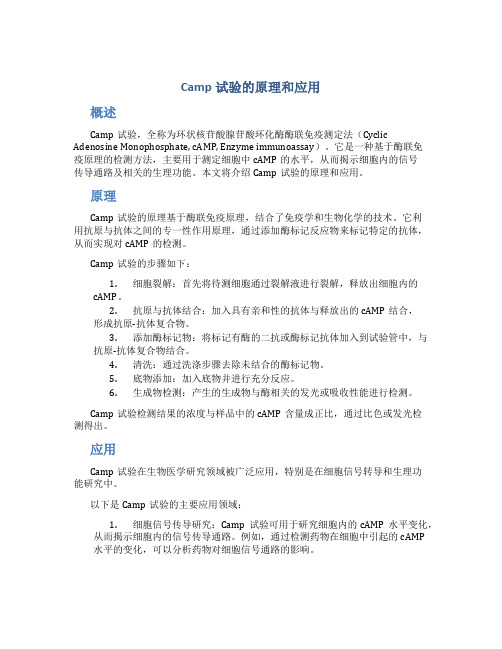
Camp试验的原理和应用概述Camp试验,全称为环状核苷酸腺苷酸环化酶酶联免疫测定法(Cyclic Adenosine Monophosphate, cAMP, Enzyme immunoassay)。
它是一种基于酶联免疫原理的检测方法,主要用于测定细胞中cAMP的水平,从而揭示细胞内的信号传导通路及相关的生理功能。
本文将介绍Camp试验的原理和应用。
原理Camp试验的原理基于酶联免疫原理,结合了免疫学和生物化学的技术。
它利用抗原与抗体之间的专一性作用原理,通过添加酶标记反应物来标记特定的抗体,从而实现对cAMP的检测。
Camp试验的步骤如下:1.细胞裂解:首先将待测细胞通过裂解液进行裂解,释放出细胞内的cAMP。
2.抗原与抗体结合:加入具有亲和性的抗体与释放出的cAMP结合,形成抗原-抗体复合物。
3.添加酶标记物:将标记有酶的二抗或酶标记抗体加入到试验管中,与抗原-抗体复合物结合。
4.清洗:通过洗涤步骤去除未结合的酶标记物。
5.底物添加:加入底物并进行充分反应。
6.生成物检测:产生的生成物与酶相关的发光或吸收性能进行检测。
Camp试验检测结果的浓度与样品中的cAMP含量成正比,通过比色或发光检测得出。
应用Camp试验在生物医学研究领域被广泛应用,特别是在细胞信号转导和生理功能研究中。
以下是Camp试验的主要应用领域:1.细胞信号传导研究:Camp试验可用于研究细胞内的cAMP水平变化,从而揭示细胞内的信号传导通路。
例如,通过检测药物在细胞中引起的cAMP 水平的变化,可以分析药物对细胞信号通路的影响。
2.G蛋白偶联受体研究:Camp试验可用于研究G蛋白偶联受体的活性和功能。
通过添加特定的激动剂或抑制剂,可以调控细胞内的cAMP水平,从而进一步研究G蛋白偶联受体的信号传导机制。
3.药物研发:Camp试验可用于评估潜在药物对细胞内cAMP水平的影响。
这有助于药物研发人员筛选出对特定信号通路有影响的药物,从而指导药物研发过程。
- 1、下载文档前请自行甄别文档内容的完整性,平台不提供额外的编辑、内容补充、找答案等附加服务。
- 2、"仅部分预览"的文档,不可在线预览部分如存在完整性等问题,可反馈申请退款(可完整预览的文档不适用该条件!)。
- 3、如文档侵犯您的权益,请联系客服反馈,我们会尽快为您处理(人工客服工作时间:9:00-18:30)。
cAMP (Cyclic Adenosine 3',5'-monophosphate) is the first identified second messenger, which has a fundamental role in the cellular response to many extracellular stimuli. The cAMP signaling pathway controls a diverse range of cellular processes. Indeed, not only did cAMP provide the paradigm for the second messenger concept, but also provided the paradigm for signaling compartmentalization. The different receptors, chiefly the GPCRs (G-Protein Coupled Receptors), Alpha andBeta-ADRs (Adrenergic Receptors), Growth Factor receptors, CRHR (Corticotropin Releasing Hormone Receptor), GcgR (Glucagon Receptor), DCC (Deleted in Colorectal Carcinoma), etc are responsible for cAMP accumulation in cells that cause different physiological outcomes, and changes in cAMP levels effects the selective activation of PKA (cAMP dependent Protein Kinase-A) isoforms. The chief source of cAMP is from ATP (Adenosine Triphosphate). In mammals, the conversion of ATP to cAMP is mediated by members of the Class-III AC (Adenylyl Cyclase)/ADCY (Adenylate Cyclase) family (E.C 4.6.1.1), which in humans comprises nine trans-membrane AC enzymes or tmACs and one soluble AC or sAC (Ref.1 & 2). The sAC predominantly occurs in mature spermatozoa. tmACs are regulated by heterotrimeric G-proteins in response to the stimulation of various types of GPCRs and therefore play a key role in the cellular response to extracellular signals. sAC, in contrast, is insensitive to G-proteins. Instead, sAC is directly activated by Ca2+ (Calcium Ions) and the metabolite HCO3- (Bicarbonate Ions), rendering the enzyme an intracellular metabolic sensor. Together, tmACs and sAC regulate a diverse set of essential biological processes, such as differentiation and gene transcription, and this makes cAMP signaling, an important mediator of intra- and extracellular signals in organisms from prokaryotes to higher eukaryotes (Ref.2 & 3).The extracellular stimuli like neurotransmitters, hormones, inflammatory stimuli, stress, epinephrine, norepinephrine, etc activate the G-proteins through receptors like GPCRs and ADR-Alpha/Beta. The major G-proteins that regulate activation of ACs are the GN-AlphaS (GN-AlphaS Complex Locus), GN-AlphaQ (Guanine Nucleotide-Binding Protein-Alpha-Q) andGN-AlphaI (Guanine Nucleotide Binding Protein-Alpha Inhibiting Activity Polypeptide). Upon activation these subunits are separated from GN-Beta (Guanine Nucleotide-Binding Protein-Beta) and GN-Gamma (Guanine Nucleotide-BindingProtein-Gamma) subunits and are converted to their GTP bound states that exhibit distinctive regulatory features on the nine tmACs in order to regulate intracellular cAMP levels (Ref.1 & 4). Other ligands like Gcg (Glucagon), Ucns (Urocortins) and Ntn1 (Netrin-1), etc either directly regulate activity of ACs or via G-protein activation through their respective receptors like GcgR, CRHR and DCC. GN-AlphaS and GN-AlphaQ activate ACs to increase intracellular cAMP levels, where as, GN-AlphaI decrease intracellular cAMP levels by inhibiting ACs. GN-Beta and GN-Gamma subunits act synergistically with GN-AlphaS and GN-AlphaQ only to activate ACII, IV and VII. However the Beta and Gamma-subunits along with GN-AlphaI inhibit the activity of ACI, V and VI. ACI, III, V, VI and IX isoforms play a vital role in spinal pain transmission and are up-regulated by chronic Opoids, for which they are often inhibited by Ca2+ and other proteins acting downstream to cAMP signaling. GN-AlphaI activity is counteracted by RGS (Regulator of G-Protein Signaling) proteins (Ref.5, 6 & 7). However, Beta-Arrestins desensitize many GPCRs and ADRs and this blocks interaction between receptors and their cellular effectors, thereby inhibiting GPCR activity. Beta-Arrestins contol intracellular cAMP levels by switching of signaling from GN-AlphaS to GN-AlphaI by recruiting PDE4D (cAMP Specific Phosphodiesterase-4D) isoforms (Ref.8). Growth factors and PI3K (Phosphatidylinositde-3 Kinase) crosstalk with cAMP signaling by activating Akt (v-Akt Murine Thymoma Viral Oncogene Homolog), which further activates PDE (Phosphodiesterase) to facilitate the conversion of cAMP to AMP through Akt Signaling. This modulates cardiac contractility and release of metabolic energy. G-proteins indirectly influence cAMP signaling by activating PI3K and PLC (Phospholipase-C). PLC cleaves PIP2 (Phosphatidylinositol 4,5-bisphosphate) to generate DAG (Diacylglycerol) and IP3 (Inositol 1,4,5-trisphosphate). DAG in turn activates PKC (Protein Kinase-C). IP3 modulates proteins upstream to cAMP signaling with the release of Ca2+ through IP3R (IP3 Receptor) facilitating activation of Src (v-Src Avian Sacroma(Schmidt-Ruppin A-2)Viral Oncogene) along with PYK2 (Proline-Rich Tyrosine Kinase-2). Activation of Src by PI3K and growth factors enhance activation of Raf1 (v-Raf1 Murine Leukemia Viral Oncogene Homolog-1) through Ras. Raf1 facilitate MEK1 (MAPK/ERK Kinase-1) and MEK2 activation, which in turn activates ERKs (Extracellular Signal-Regulated Kinases) and this ultimately leads to induction of transcription regulator Elk1 (ETS-domain protein Elk1) mediated gene expression. PKCmodulate cAMP signaling by activation of Raf1, PYK2 and ACs like ACI, II, III, V and VII, but inhibits ACVI (Ref.9).Once active, the tmACs and sAC produces the second messenger cAMP in response to a wide range of signal transduction pathways. Three main targets of cAMP are PKA, the GTP-exchange protein, EPACs (Exchange Protein Activated by cAMP) and the CNG (Cyclic-Nucleotide Gated Ion Channel). CNG activation by cAMP provides passage to Ca2+ influx. cAMP activate Rap1A (Ras-Related Protein-1A) and Rap1B (Ras-Related Protein Rap1B) through the PKA-independent and EPAC (Exchange Protein Activated by cAMP)-dependent pathway. cAMP activates cAMP-GEFI (cAMP-Regulated Guanine Nucleotide Exchange Factor-I)/EPAC1 and cAMP-GEFII (cAMP-Regulated Guanine Nucleotide Exchange Factor-II)/EPAC2 that in turn activate Rap1A and Rap1B, respectively. Rap1A and Rap1B then forms an active complex with BRaf (v-Raf Murine Sarcoma Viral Oncogene Homolog-B1) for MEK1/2 activation finally resulting in Elk1 activation. Rap1A and Rap1B further stimulate Rap1 and Rap2 pathways that are vital for cell survival (Ref.10 & 11). Apart from CNG, PKC, and EPACs, other direct targets of cAMP includes, PDE, mTOR (Mammalian Target of Rapamycin), p70S6K/RPS6KB1 (Ribosomal Protein-S6kinase-70kDa-Polypeptide-1), PLA2 (Phospholipase-A2). cAMP-activated mTOR and p70S6K promote cell growth via the mTOR and p70S6K signaling route, whereas, PLA2 facilitates release of stored energy by enhancing the Fatty acid metabolism processes. The Urocortin-cAMP mediated induction of PKC and p38 results in Apoptosis and Cytokine production (like that of IL-6 (Interleukin-6)), downstream to the Urocortin-cAMP pathway. Although cAMP directly regulates the activities of some molecules, PKA appears to be the major 'read-out' for cAMP and is the predominant cellular effector of cAMP (Ref.12). PKA is tethered to specific cellular locations by a growing class of proteins called AKAPs (A-Kinase Anchor Proteins). Targeting of PKA isozymes by AKAPs is important for an increasing number of physiological processes such as cAMP regulation of ion channels in the nervous system, regulation of the cell cycle which involves microtubule dynamics, chromatin condensation and decondensation, nuclear envelope disassembly and reassembly, Steroidogenesis, reproductive function, immune responses and numerous intracellular transport mechanisms (Ref.13).In the following sections, the role of localized pools of PKA in the context of some selected physiological processes where regulation by cAMP plays a major role has been analyzed. The ADR-Alpha/Beta stimulation of cAMP-PKA phosphorylates several proteins related to excitation-contraction coupling like activation of L-Type CaCn (Calcium Channels), KCn (Potassium Channel), SCn (Sodium Channels), ClCn (Chloride Channels), RyR (Ryanodine Receptor), Pln (Phospholamban), CRP(C-Reactive Protein) but inhibts TnnI (Troponin-I). PKA phosphorylates Pln that regulates the activity of SERCA2 (Sarcoplasmic Reticulum Ca2+-ATPase-2). It leads to increased reuptake of Ca2+, Cl- (Chloride Ions), K+ (Potassium Ions), Na+ (Sodium Ions) and this process is affected in failing hearts. Ca2+ uptake activates Caln (Calcineurin), which further facilitates NFAT (Nuclear Factor of Activated T-Cells) translocation to the nucleus, a process that is quite essential for axonal growth. cAMP plays a vital role in regulation of cardiovascular function by controlling the process of myocardial contraction. Increase in higher concentration of Ca2+ and PKA activation enhances eNOS (Endothelial NOS) enzyme activity by phosphorylation of Serine residue (Ser635) in order to stimulate eNOS signaling, which is essential to maintain cardiovascular homeostasis (Ref.13). In mammals, Ca2+ and HCO3- play a critical role in the regulation of sperm function, most likely by regulation of cAMP levels. sAC is active in human spermatozoa and is a sensor for both HCO3- and Ca2+. Ca2+ release by CaCn and CNG, activate Calm (Calmodulin) and CamKs (Calcium/Calmodulin-Dependent Protein Kinases). Calm further activate Caln, CamK2 (Calcium/Calmodulin-Dependent Protein Kinase-II) and CamK4. These in turn modulate cAMP production by regulating the activity of ACs and PDEs. The CamKs along with Caln inhibit PDE and ACIX, whereas CamK2 and CamK4 inhibit the function of ACIX and ACI, respectively (Ref.2). ACIX is also inhibited by PKC thus controlling cAMP signaling in the hippocampal neurons. cAMP activated PKA represses ERK activation by the formation of an inactive Rap1/Raf1 that interferes with ERK activation through seizure of MEK1,2 activation by sequestering Raf1 activity. 14-3-3 like the PKA also aids the process of Raf1 inactivation. Therefore, the effect of cAMP on ERK differs depending on the balance of the Raf1, BRaf and PKA isoforms occurring inside a cell (Ref.10). Long PDE4A (cAMP Specific Phosphodiesterase-4A) isoforms are activated bycAMP, PKA and Akt kinases. The PDE sequester cAMP activity by converting it back to AMP. This negative-feedback loop terminates the cAMP signal locally. cAMP further represses the activity PDE1 (Phosphodiesterase-1) to enhance the duration and intensity of cAMP signaling (Ref.14).cAMP follows a distinct route and activates a single PKA-AKAP complex close to the substrate to mediate a distinct biological effect. Accordingly, each substrate appears to have its own, private anchored pool of PKA and its own local gradient of cAMP. PKA inhibit the interaction of 14-3-3 proteins with BAD (through 14-3-3/BAD signaling) and NFAT to promote cell survival. PKA inhibits Adducin action by limiting its role during assembly of Spectrin-Actin network in erythrocytes, thereby reducing the chances of Erythroleukemia. It activates KDELR (KDEL (Lys-Asp-Glu-Leu) Endoplasmic Reticulum Protein Retention Receptor) to promote retrieval of proteins (protein retention) from golgi complex to endoplasmic reticulum thereby maintaining steady state of the cell. Increased cAMP levels promote survival of neuronal cells by inactivating GSK3Alpha (Glycogen Synthase Kinase-3-Alpha) and GSK3Beta (Glycogen Synthase Kinase-3-Beta) via a PKA dependent mechanism and thus prevents Oncogenesis and neurodegeneration (Ref.13 & 15). PKA interferes at different levels with other signaling pathways. Inactivation of PTP (Protein Tyrosine Phosphatase) results in dissociation from and consequent activation of ERKs. Inactivation of PCTK1 (PCTAIRE Protein Kinase-1) and APC (Anaphase-Promoting Complex) helps to maintain control cell proliferation and anaphase initiation and late mitotic events, respectively, thereby checking the degradation cell cycle regulators. PKA activation by cAMP enhances release of stored energy in cells by phosphorylation of HSL (Hormone-Sensitive Lipase) in white adipose tissue, which leads to the hydrolysis of triglycerides (vital intermediates of Tricaylglycerol Metabolism). Hydrolysis of triglycerides by HSL generates free fatty acids, the major gateway for the release of stored energy, and this process is termed as Lipolysis. Gcg binds to on the surface of liver cells and triggers an increase in cAMP production leading to an increased rate of Glycogenolysis by activating PHK (Phosphorylase Kinase) via the PKA-mediated cascade. PHK further activate PYG (Glycogen Phosphorylase), which converts Glycogen to Glucose-1-Phosphate. Phosphoglucomutase then transfers phosphate to C-6 of Glucose-1-Phosphate generating Glucose-1,6-phosphate as an intermediate. The phosphate on C-1 is then transferred to the enzyme regenerating it and Glucose-6-Phospahte is the released product that enters Glycolysis. This is the same response hepatocytes have to Epinephrine release through the ADR-Alpha/Beta. PKA further inhibits GYS (Glycogen Synthase) leading to seizure of energy consuming process like Glycogen Synthesis (Ref.13 & 16).Upon stimulation from hormones, cAMP increases phosphorylation of RhoA that inactivates Rho Kinase. Rho Kinase regulates Myosin-II and cell contraction by catalyzing phosphorylation of the regulatory subunit of Myosin phosphatase, PPtase1 (Protein Phosphatase-1), by inhibiting its catalytic activity, which results in an indirect increase in RLC (Regulatory Light Chain of Myosin) phosphorylation. Inactivation of Rho Kinase also directly increases RLC phosphorylation. Such increased intracellular cAMP and PKA activation on RLC phosphorylation decreases Thrombin-induced isometric tension development in endothelial cells and this decrease the development of Edema, a hallmark of acute and chronic inflammation (Ref.17). Interestingly, PKA controls phosphatase activity by phosphorylation of specific PPtase1 inhibitors, such as DARPP32 (Dopamine-andcAMP-Regulated Phosphoprotein). Neurotransmitters enhance DARPP32 interaction via GPCRs, which leads to suppression of PPtase1 activity, when DARPP32 is phosphorylated at Thr-34 (Threonine-34) position. Phosphorylation is a crucial event in transcriptional activation by CREB (cAMP Response Element-Binding Protein), CREM (cAMP Response Element Modulator) and ATF1 (Activating Transcription Factor-1), because it allows interaction with the transcriptional co-activators CBP(CREB-Binding Protein) and p300. CREM gene encodes many different isoforms, some of which have repressive functions. Particularly the repressor ICER (Inducible cAMP Early Repressor), participates in the downregulation of cAMP-induced transcription by competing with the binding of CREB and CREM activators to their DNA binding sites. PPtase1 checks the phosphorylation events in order to inactivate the formation of repressor isoforms like ICER so that CREB, CREM and ATF1 are able to interact with the co-activators like CBP and p300. Hence, under physiological conditions, ICER induction is a transient phenomenon that allows cAMP signaling to return to the basal state. In contrast, prolonged or inappropriate induction of ICERelicits pathological consequences (Ref.18). Similarly, phosphorylation of NK-KappaB (Nuclear Factor-KappaB) by PKA is necessary for transcriptional activation and interaction with CBP. PKA modulates the activity of transcription factors, such as nuclear receptors and HMG (High Mobility Group)-containing proteins, influencing their dimerization or DNA-binding properties.A peculiar example is the mechanism by which PKA regulates Gli3 (Gli-Kruppel Family Member-3) under the influence from Hedgehog signaling. Function of Gli3 is similar to that of Drosophila gene CI (Cubitus Interruptus) activity. In this case phosphorylation stimulates a specific cleavage of Gli3 which transforms the protein from an activator to a repressor. However, proteins like PKIs (Protein Kinase Inhibitors) and Mep1B (Meprin-A-Beta) down regulate PKA activity to prevent aberrant gene expression (Ref.13 & 16).Normally, the level of intracellular cAMP is regulated by the balance between the activities of two types of enzymes; ACs and the cyclic nucleotide PDEs chiefly in response to hormones and neurotransmitters. The cAMP signaling is involved in controlling exocytotic events in polarized epithelial cells with implication for Diabetes Insipidus, Hypertension, Gastric ulcers, Thyroid disease, Diabetes Mellitus, and Asthma. Heterologous sensitization of cAMP signaling contributes to fundamental physiological processes such as the timing of circadian rhythms, sexual behavior, and neurotransmitter crosstalk, and also to neurological disorders such as substance abuse and drug-induced Dyskinesias (Ref.19). This provide insight into the mechanisms of addiction and many other central nervous system disorders reflective of altered neuronal cell functioning. Besides it also represent very likely roles for ACs and open up avenues for therapeutics targeting ACs that may prove useful in the development of male contraceptives (Ref.1 & 20).References:1. Watts VJ,Neve KA.Sensitization of adenylate cyclase by Galpha i/o-coupled receptors.Pharmacol. Ther. 2005 Jun;106(3):405-21.2. Jaiswal BS,Conti M.Calcium regulation of the soluble adenylyl cyclase expressed in mammalian spermatozoa.Proc. Natl. Acad. Sci. U S A. 2003 Sep 16;100(19):10676-81.3. Baillie GS,Houslay MD.Arrestin times for compartmentalised cAMP signalling and phosphodiesterase-4 enzymes.Curr. Opin. Cell Biol. 2005 Apr;17(2):129-34.4. Pullar CE,Isseroff RR.Cyclic AMP mediates keratinocyte directional migration in an electric field.J. Cell Sci. 2005 May 1;118(Pt 9):2023-34.5. Bie B,Peng Y,Zhang Y,Pan ZZ.cAMP-mediated mechanisms for pain sensitization during opioid withdrawal.J. Neurosci. 2005 Apr 13;25(15):3824-32.6. Cumbay MG,Watts VJ.Galphaq potentiation of adenylate cyclase type 9 activity through a Ca2+/calmodulin-dependent pathway.Biochem. Pharmacol. 2005 Apr 15;69(8):1247-56.7. Yamada RX,Matsuki N,Ikegaya Y.cAMP differentially regulates axonal and dendritic development of dentate granule cells.J. Biol. Chem. 2005 Nov 11;280(45):38020-8.8. Dessauer CW,Nguyen BT.Relaxin stimulates multiple signaling pathways: activation of cAMP,PI3K,and PKCzeta in THP-1 cells.Ann. N Y. Acad. Sci. 2005 May;1041:272-9.9. Leblais V,Jo SH,Chakir K,Maltsev V,Zheng M,Crow MT,Wang W,Lakatta EG,Xiao RP.Phosphatidylinositol 3-kinase offsets cAMP-mediated positive inotropic effect via inhibiting Ca2+ influx incardiomyocytes.Circ. Res. 2004 Dec 10;95(12):1183-90.10. Kim SS,Choi JM,Kim JW,Ham DS,Ghil SH,Kim MK,Kim-Kwon Y,Hong SY,Ahn SC,Kim SU,Lee YD,Suh-Kim H.cAMP induces neuronal differentiation of mesenchymal stem cells via activation of extracellular signal-regulated kinase/MAPK.Neuroreport. 2005 Aug 22;16(12):1357-61.11. Kiermayer S,Biondi RM,Imig J,Plotz G,Haupenthal J,Zeuzem S,Piiper A.Epac Activation Converts cAMP from a Proliferative into a Differentiation Signal in PC12 Cells.Mol. Biol. Cell. 2005 Oct 5.12. Malbon CC,Tao J,Wang HY.AKAPs (A-kinase anchoring proteins) and molecules that compose their G-protein-coupled receptor signalling complexes.Biochem. J. 2004 Apr 1;379(Pt 1):1-9.13. Tasken K,Aandahl EM.Localized effects of cAMP mediated by distinct routes of protein kinase A.Physiol. Rev. 2004 Jan;84(1):137-67.14. Dumaz N,Marais R.Integrating signals between cAMP and the RAS/RAF/MEK/ERK signalling pathways.FEBS J. 2005 Jul;272(14):3491-504.15. Chin PC,Majdzadeh N,D'Mello SR.Inhibition of GSK3beta is a common event in neuroprotection by different survival factors.Brain. Res. Mol. Brain Res. 2005 Jun 13;137(1-2):193-201.16. Taylor SS,Kim C,Vigil D,Haste NM,Yang J,Wu J,Anand GS.Dynamics of signaling by PKA.Biochim. Biophys. Acta. 2005 Sep 22.17. Goeckeler ZM,Wysolmerski RB.Myosin phosphatase and cofilin mediate cAMP/cAMP-dependent protein kinase-induced decline in endothelial cell isometric tension and myosin II regulatory light chain phosphorylation.J. Biol. Chem. 2005 Sep 23;280(38):33083-95.18. Ding B,Abe J,Wei H,Xu H,Che W,Aizawa T,Liu W,Molina CA,Sadoshima J,Blaxall BC,Berk BC,Yan C.A positive feedback loop of phosphodiesterase 3 (PDE3) and inducible cAMP early repressor (ICER) leads tocardiomyocyte apoptosis.Proc. Natl. Acad. Sci. U S A. 2005 Oct 11;102(41):14771-6.19. Shankar DB,Cheng JC,Sakamoto KM.Role of cyclic AMP response element binding protein in human leukemias.Cancer. 2005 Nov 1;104(9):1819-24.20. Linder JU,Schultz JE.The class III adenylyl cyclases: multi-purpose signalling modules.Cell. Signal. 2003 Dec;15(12):1081-9.。