电气工程外文翻译
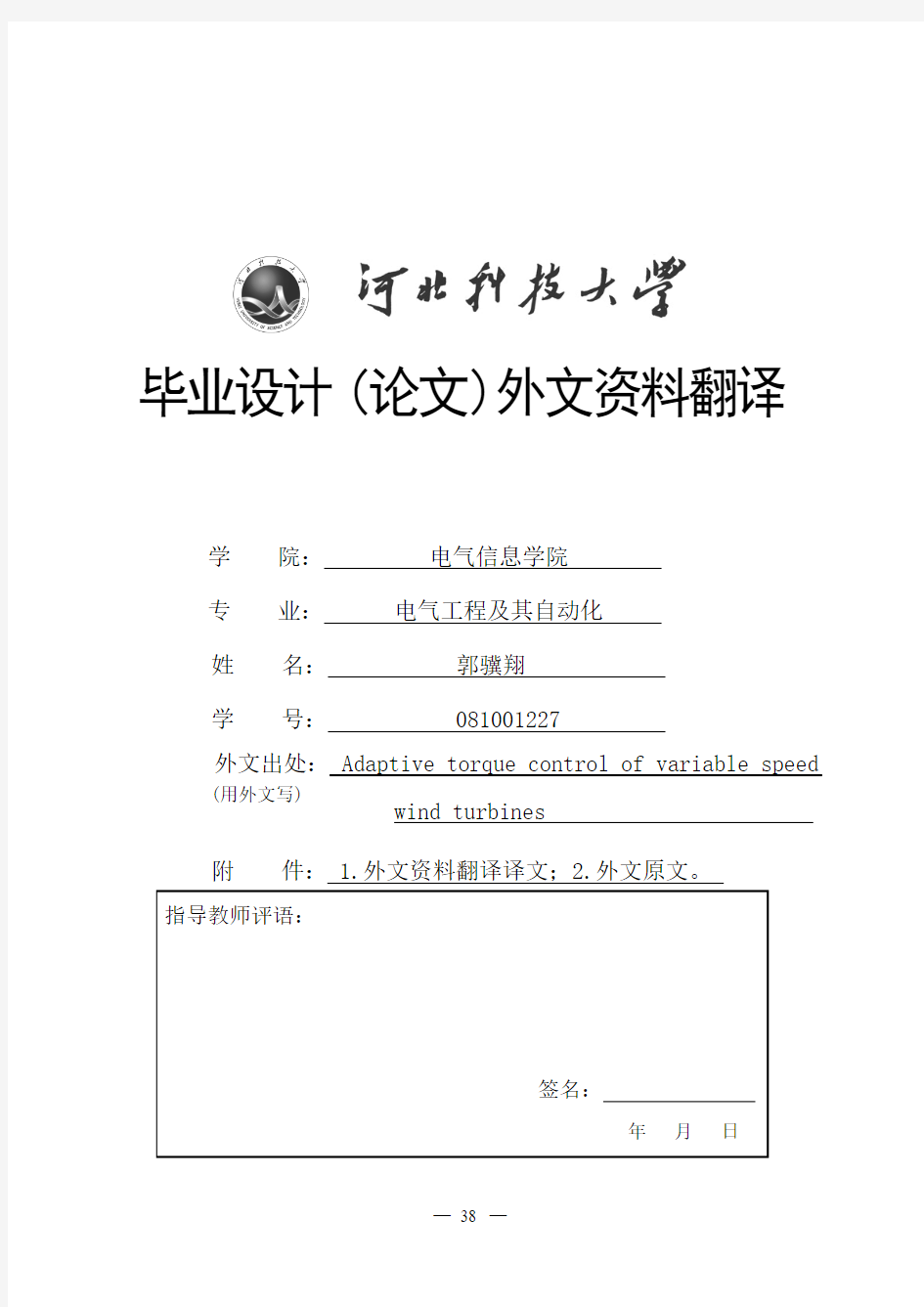
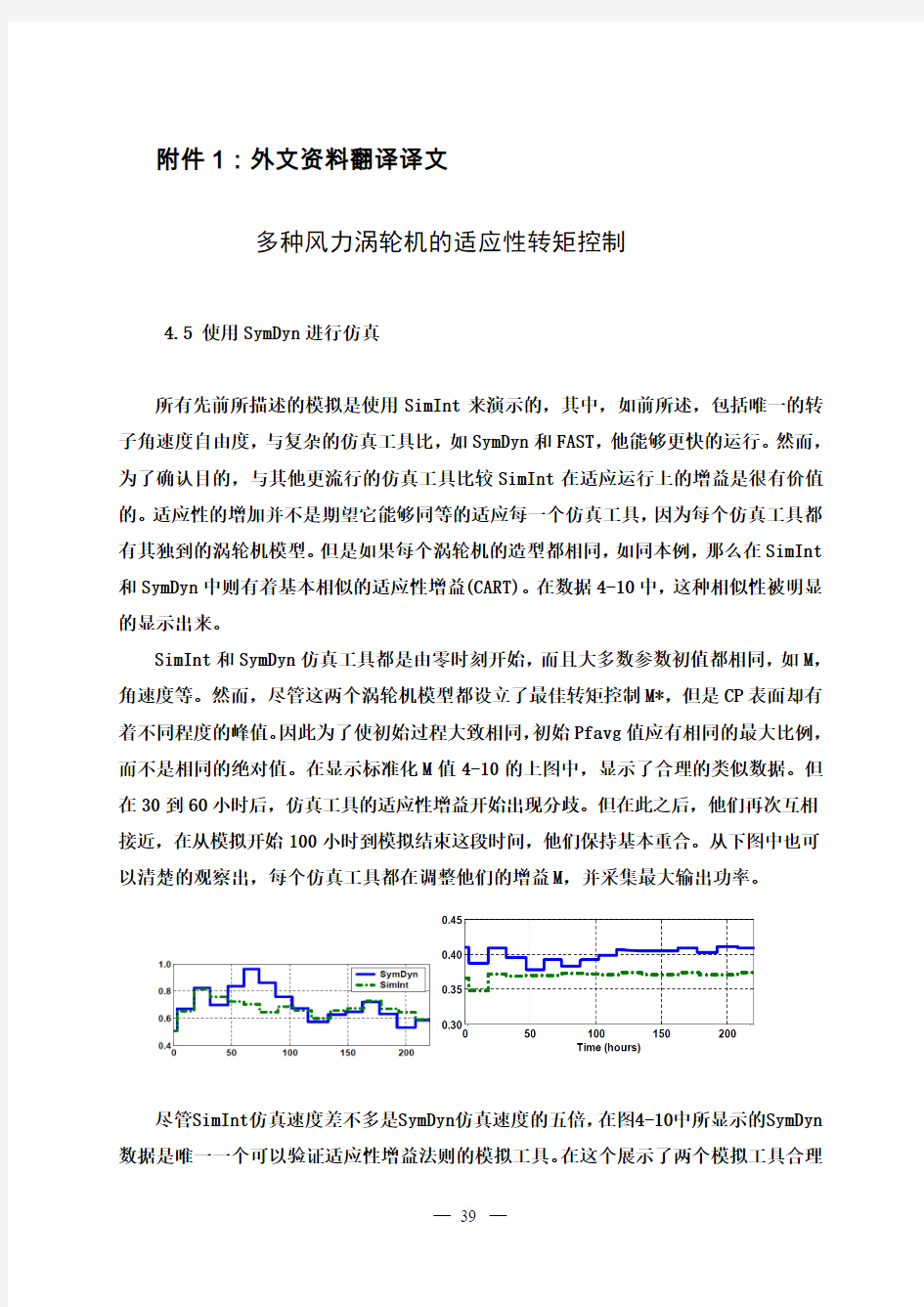
毕业设计(论文)外文资料翻译
学院:电气信息学院
专业:电气工程及其自动化
姓名:郭骥翔
学号: 081001227
外文出处: Adaptive torque control of variable speed
wind turbines 附件: 1.外文资料翻译译文;2.外文原文。
指导教师评语:
签名:
年月日(用外文写)
附件1:外文资料翻译译文
多种风力涡轮机的适应性转矩控制
4.5 使用SymDyn进行仿真
所有先前所描述的模拟是使用SimInt来演示的,其中,如前所述,包括唯一的转子角速度自由度,与复杂的仿真工具比,如SymDyn和FAST,他能够更快的运行。然而,为了确认目的,与其他更流行的仿真工具比较SimInt在适应运行上的增益是很有价值的。适应性的增加并不是期望它能够同等的适应每一个仿真工具,因为每个仿真工具都有其独到的涡轮机模型。但是如果每个涡轮机的造型都相同,如同本例,那么在SimInt 和SymDyn中则有着基本相似的适应性增益(CART)。在数据4-10中,这种相似性被明显的显示出来。
SimInt和SymDyn仿真工具都是由零时刻开始,而且大多数参数初值都相同,如M,角速度等。然而,尽管这两个涡轮机模型都设立了最佳转矩控制M*,但是CP表面却有着不同程度的峰值。因此为了使初始过程大致相同,初始Pfavg值应有相同的最大比例,而不是相同的绝对值。在显示标准化M值4-10的上图中,显示了合理的类似数据。但在30到60小时后,仿真工具的适应性增益开始出现分歧。但在此之后,他们再次互相接近,在从模拟开始100小时到模拟结束这段时间,他们保持基本重合。从下图中也可以清楚的观察出,每个仿真工具都在调整他们的增益M,并采集最大输出功率。
尽管SimInt仿真速度差不多是SymDyn仿真速度的五倍,在图4-10中所显示的SymDyn 数据是唯一一个可以验证适应性增益法则的模拟工具。在这个展示了两个模拟工具合理
的相同之处的实验后,验证了SimInt可以作为测试增益法则的实验的仿真工具。然而,不像SimInt,SymDyn除了分析发电机转速外,还可以分析其他额外的自由度。因此,对SymDyn仿真数据的分析是为了确定适应性增益法则是否会给涡轮机带来可能的额外压力。这种分析是下一部分的主题。
4.6 对涡轮机原件的压力仿真
除了涡轮机转速外,SymDyn还能模拟许多其他的自由度,比如转动轴角速度,叶片转动角,风塔扭转角度,塔方,前角等。对这些自由度的建模能力是SymDyn有效的控制设计和测试的关键。在使用SymDyn的适应性控制器测试中,要回答的问题是:当涡轮机在被标准的非适应性发电机转矩控制器控制时(公式2.1),适应性发电机转矩控制器(公式2.14)是否会引起额外的本应缺失的涡轮机暂态压力。更长远的稳定状态负荷的变化会在5.3中进一步讨论,但使用这些仿真工具长时间分析它是很难做到的,并且并不在这部分的考虑范围内。然而,当每次适应性发生的时候,一个突然的电机转矩变化可以激起一些不知数目的模式。因此,这部分会集中讨论在适应性发生前后很快采集到的SymDyn数据。
从SymDyn的试验中看来,适应性转矩控制增益M不会引起上述自由度增加额外的压力。接下来的三个表格中(图4-11到图4-13),为两种在SymDyn仿真在图4-10中发生的不同的适应性提供的数据样本,它包括三个自由度(传动轴扭转,扇叶转动弯曲,风塔两侧弯曲)。表4-3为每个例子提供了一些相关信息。每个适应性涉及M增加的变化,但变化的方向并未影响其他适应应力的性质研究。此外,转子转速大约相同时也是如此。CART的最大转速是4.37弧度每秒,所以在2.9到3.0弧度内在区域2中进行操作是比较好的。最后,当转子转速下降时4号适应度发生,当转速上升时7号适应度发生。转子加速度与风速加速度密切相关,在一般情况下,更大的风速会对涡轮机部件造成更大的压力。
在SymDyn所能提供的建模能力的自由度中,转动轴扭角是与发电机紧密相连的自由度之一,因为它连接着发电机转子。因此对转矩控制适应增益最重要的影响预计会在轴上观察到,事实也确实如此。图4-11展现了两种适应性的轴扭角。
在图4-11的上图中,4号适应性中很显然,传动轴出现对适应性的不良反应发生在有垂直线标记的时刻1084.51。然而,由于一些原因,这种不良反应并不令人担忧,因为它第一次出现。首先,由适应性减少所引起的震荡发生在短短几秒内,表明这可能是一个稳定的模式。其次,最大震荡的震角约为0.3度,即使在下图中的适应时间中,这仍是小于在下图的明显震荡。这表明,适应性不会造成比环境变化所产生的影响更大。最后,在仿真中,适应性是看做同步发生的,或者相差0.01秒。在真正的涡轮机例子中,它不太可能迅速改变发动机转矩。所以真正的涡轮轴不会观察到如此巨大的跃变。因此,当对一个真正的涡轮机实现适应控制器时,要密切关注轴扭转角的震荡。但这并不代表着就不去执行这个操作。
图4-12展现了在适应性执行前后15秒的每个叶片的震角。“震”是指平面的叶片的弯曲方向。对于水平涡轮机,叶片弯曲通常发生在风向叶片施加升力的方向。从图4-11可以发现,在7号适应度时间内发生的环境条件变化比4号适应度时间内剧烈的多,因而叶片扭角更大而且承受更多的变化。为7号适应度所绘制的叶片扭角确实在适应性之后有所增加,但是它的平均增加可以认为是更快的旋转转子所引起的。基于这些有代表性的数据,突然地适应性不会引起对叶片旋转瓣片的不必要的压力。然而,应当指出,对于发生在宣传平面和对旋转轴扭转角震动更敏感的叶片扭角,在SymDyn中无法仿真。
注意在图4-13中这两个适应的最终图,展现了塔两侧的弯曲角。可以发现,发电机转矩增益的两种适应性在这种模式下没有得到一致的效果,在这个例子中,在适应性开始前振幅继续下降,然而在其他例子中则有上升的趋势。塔弯曲的主要因素是环境变化和转子转速,首先因为塔的固有频率与区域2中的转子转速向对应(大约2.7弧度每秒)。事实上,这种转子转速在4号适应性的最后几秒种达到,这可能可以解释为什么震荡会
增加。总结起来,在SymDyn仿真的所有自由度中,似乎只有传动轴扭转会被适应控制器的适应性增益所激发,而这种激发在一个真正的涡轮机中会少的明显。在其他没有绘制的可仿真的自由度中(塔前后扭角和塔扭角)展示了,像塔两侧扭角图示一样,对于适应性没有一致的响应。
使用SymDyn和SimInt工具,适应性增益法则被仿真测试了。SimInt已经测试了一些关注的领域,包括非零偏航误差,不准确的测风和改变的参数。我们也已经将SymDyn 和SimInt的仿真结果做了比较,并发现基本是相似的。SymDyn的输出为涡轮机部件一些新的压力做了测试。我们建议适应性法则现在已经可以再真正的CART上进行测试了。
附件2:外文原文(复印件)
红外数据通信技术外文翻译文献
红外数据通信技术外文翻译文献(文档含中英文对照即英文原文和中文翻译) Infrared Remote Control System Abstract Red outside data correspondence the technique be currently within the scope of world drive extensive usage of a kind of wireless conjunction technique, drive numerous hardware and software platform support. Red outside the transceiver product have cost low, small scaled turn, the baud rate be quick, point to point SSL, be free from electromagnetism thousand Raos
etc. characteristics, can realization information at dissimilarity of the product fast, convenience, safely exchange and transmission, at short distance wireless deliver aspect to own very obvious of advantage. Along with red outside the data deliver a technique more and more mature, the cost descend, red outside the transceiver necessarily will get at the short distance communication realm more extensive of application. The purpose that design this system is transmit customer’s operation information with infrared rays for transmit media, then demodulate original signal with receive circuit. It use coding chip to modulate signal and use decoding chip to demodulate signal. The coding chip is PT2262 and decoding chip is PT2272. Both chips are made in Taiwan. Main work principle is that we provide to input the information for the PT2262 with coding keyboard. The input information was coded by PT2262 and loading to high frequent load wave whose frequent is 38 kHz, then modulate infrared transmit dioxide and radiate space outside when it attian enough power. The receive circuit receive the signal and demodulate original information. The original signal was decoded by PT2272, so as to drive some circuit to accomplish customer’s operation demand. Keywords: Infrared dray;Code;Decoding;LM386;Red outside transceiver 1 Introduction 1.1 research the background and significance Infrared Data Communication Technology is the world wide use of a wireless connection technology, by the many hardware and software platforms supported. Is a data through electrical pulses and infrared optical pulse switch between the wireless data transceiver technology.
通信工程专业英语翻译
通信工程专业英语翻译 JXTA is a crystallization by Sun company's chief scientist Bill Joy's more than twenty years of brewing."JXTA technology is a platform for Network programming and calculation.To solve the modern distribution calculation especially peer-to-peer (Peer to Peer, P2P) in the calculation of the problem".[1] JXTA research project,which will provide a new framework that make the user more convenient to access to connect on the Internet's personal computer resources, thus further expand Internet 's space. At the same time JXTA is also the Sun's "ONE Internet" strategic continuance, and will take a more positive attitude to compete with the .net strategy of Microsoft and Hailstorm plan . JXTA agreement defines a set of six agreement based on XML, the organization of node into node group, release and found some resources, communication and mutual monitoring provides standardized method.(Endpoint Routing Protocol,ERP) is used for node found routing.To send a message to other nodes, and through the potential firewall and connection.(Rendezvous Protocol,RVP) s used for the nodes in the group to spread information.(Peer Resolver Protocol,PRP) is Used to one or more points to send general inquiries, and receive the response of inquiries.
PLC控制下的电梯系统外文文献翻译、中英文翻译、外文翻译
PLC控制下的电梯系统 由继电器组成的顺序控制系统是最早的一种实现电梯控制的方法。但是,进入九十年代,随着科学技术的发展和计算机技术的广泛应用,人们对电梯的安全性、可靠性的要求越来越高,继电器控制的弱点就越来越明显。 电梯继电器控制系统故障率高,大大降低了电梯的可靠性和安全性,经常造成停梯,给乘用人员带来不便和惊忧。且电梯一旦发生冲顶或蹲底,不但会造成电梯机械部件损坏,还可能出现人身事故。 可编程序控制器(PLC)最早是根据顺序逻辑控制的需要而发展起来的,是专门为工业环境应用而设计的数字运算操作的电子装置。鉴于其种种优点,目前,电梯的继电器控制方式己逐渐被PLC控制所代替。同时,由于电机交流变频调速技术的发展,电梯的拖动方式己由原来直流调速逐渐过渡到了交流变频调速。因此,PLC控制技术加变频调速技术己成为现代电梯行业的一个热点。 1. PLC控制电梯的优点 (1)在电梯控制中采用了PLC,用软件实现对电梯运行的自动控制,可靠性大大提高。 (2)去掉了选层器及大部分继电器,控制系统结构简单,外部线路简化。 (3)PLC可实现各种复杂的控制系统,方便地增加或改变控制功能。 (4) PLC可进行故障自动检测与报警显示,提高运行安全性,并便于检修。 (5)用于群控调配和管理,并提高电梯运行效率。 (6)更改控制方案时不需改动硬件接线。 2.电梯变频调速控制的特点 随着电力电子技术、微电子技术和计算机控制技术的飞速发展,交流变频调速技术的发展也十分迅速。电动机交流变频调速技术是当今节电、改善工艺流程以提高产品质量和改善环境、推动技术进步的一种主要手段。变频调速以其优异的调速性能和起制动平稳性能、高效率、高功率因数和节电效果,广泛的适用范围及其它许多优点而被国内外公认为最有发展前途的调速方式 交流变频调速电梯的特点 ⑴能源消耗低 ⑵电路负载低,所需紧急供电装置小 在加速阶段,所需起动电流小于2.5倍的额定电流。且起动电流峰值时间短。由于起动电流大幅度减小,故功耗和供电缆线直径可减小很多。所需的紧急供电
机械设计设计外文文献翻译、中英文翻译、外文翻译
机械设计 摘要:机器是由机械装置和其它组件组成的。它是一种用来转换或传递能量的装置,例如:发动机、涡轮机、车辆、起重机、印刷机、洗衣机、照相机和摄影机等。许多原则和设计方法不但适用于机器的设计,也适用于非机器的设计。术语中的“机械装置设计”的含义要比“机械设计”的含义更为广泛一些,机械装置设计包括机械设计。在分析运动及设计结构时,要把产品外型以及以后的保养也要考虑在机械设计中。在机械工程领域中,以及其它工程领域中,所有这些都需要机械设备,比如:开关、凸轮、阀门、船舶以及搅拌机等。 关键词:设计流程设计规则机械设计 设计流程 设计开始之前就要想到机器的实际性,现存的机器需要在耐用性、效率、重量、速度,或者成本上得到改善。新的机器必需具有以前机器所能执行的功能。 在设计的初始阶段,应该允许设计人员充分发挥创造性,不要受到任何约束。即使产生了许多不切实际的想法,也会在设计的早期,即在绘制图纸之前被改正掉。只有这样,才不致于阻断创新的思路。通常,还要提出几套设计方案,然后加以比较。很有可能在这个计划最后决定中,使用了某些不在计划之内的一些设想。 一般的当外型特点和组件部分的尺寸特点分析得透彻时,就可以全面的设计和分析。接着还要客观的分析机器性能的优越性,以及它的安全、重量、耐用性,并且竞争力的成本也要考虑在分析结果之内。每一个至关重要的部分要优化它的比例和尺寸,同时也要保持与其它组成部分相协调。 也要选择原材料和处理原材料的方法。通过力学原理来分析和实现这些重要的特性,如那些静态反应的能量和摩擦力的最佳利用,像动力惯性、加速动力和能量;包括弹性材料的强度、应力和刚度等材料的物理特性,以及流体润滑和驱动器的流体力学。设计的过程是重复和合作的过程,无论是正式或非正式的进行,对设计者来说每个阶段都很重要。 最后,以图样为设计的标准,并建立将来的模型。如果它的测试是符合事先要
通信工程项目毕业材料外文翻译
用于多跳认知无线电网络的分布式网络编码控制信道 Alfred Asterjadhi等著 1 前言 大多数电磁频谱由政府机构长期指定给公司或机构专门用于区域或国家地区。由于这种资源的静态分配,许可频谱的许多部分在许多时间和/或位置未使用或未被充分利用。另一方面,几种最近的无线技术在诸如IEEE802.11,蓝牙,Zigbee之类的非许可频段中运行,并且在一定程度上对WiMAX进行操作;这些技术已经看到这样的成功和扩散,他们正在访问的频谱- 主要是2.4 GHz ISM频段- 已经过度拥挤。为了为这些现有技术提供更多的频谱资源,并且允许替代和创新技术的潜在开发,最近已经提出允许被许可的设备(称为次要用户)访问那些许可的频谱资源,主要用户未被使用或零星地使用。这种方法通常被称为动态频谱接入(DSA),无线电设备发现和机会性利用未使用或未充分利用的频谱带的能力通常称为认知无线电(CR)技术。 DSA和CR最近都引起了无线通信和网络界的极大关注。通常设想两种主要应用。第一个是认知无线接入(CW A),根据该认知接入点,认知接入点负责识别未使用的许可频谱,并使用它来提供对次用户的接入。第二个应用是我们在这个技术中研究的应用,它是认知自组织网络(CAN),也就是使用 用于二级用户本身之间通信的无许可频谱,用于诸如点对点内容分发,环境监控,安全性等目的,灾难恢复情景通信,军事通信等等。 设计CAN系统比CW A有更多困难,主要有两个原因。第一是识别未使用的频谱。在CW A中,接入点的作用是连接到互联网,因此可以使用简单的策略来推断频谱可用性,例如查询频谱调节器在其地理位置的频谱可用性或直接与主用户协商频谱可用性或一些中间频谱经纪人另一方面,在CAN中,与频谱调节器或主要用户的缺乏直接通信需要二级用户能够使用检测技术自己识别未使用的频谱。第二个困难是辅助用户协调媒体访问目的。在CW A中存在接入点和通常所有二级用户直接与之通信(即,网络是单跳)的事实使得直接使用集中式媒体接入控制(MAC)解决方案,如时分多址(TDMA)或正交频分多址(OFDMA)。相反,预计CAN将跨越多跳,缺少集中控制器;而对于传统的单通道多跳自组织网络而言,这个问题的几个解决方案是已知的,因为假设我们处理允许设备访问的具有成
伺服电机外文文献翻译
伺服电机 1. 伺服电机的定义 伺服电动机又称执行电动机,在自动控制系统中,用作执行元件,把所收到的电信号转换成电动机轴上的角位移或角速度输出。分为直流和交流伺服电动机两大类,其主要特点是,当信号电压为零时无自转现象,转速随着转矩的增加而匀速下降。伺服电机在伺服系统中控制机械元件运转的发动机. 是一种补助马达间接变速装置。伺服电机可使控制速度, 位置精度非常准确。将电压信号转化为转矩和转速以驱动控制对象。转子转速受输入信号控制,并能快速反应,在自动控制系统中作执行元件,且具有机电时间常数小、线性度高、始动电压低等特点。 2. 伺服电机工作原理 1.伺服主要靠脉冲来定位,基本上可以这样理解,伺服电机接收到1 个脉冲,就会旋转1 个脉冲对应的角度,从而实现位移,因为,伺服电机本身具备发出脉冲的功能,所以伺服电机每旋转一个角度,都会发出对应数量的脉冲,这样,和伺服电机接受的脉冲形成了呼应,或者叫闭环,如此一来,系统就会知道发了多少脉冲给伺服电机,同时又收了多少脉冲回来,这样,就能够很精确的控制电机的转动,从而实现精确的定位,可以达到0.001mm有刷电机成本低,结构简单,启动转矩大,调速范围宽,控制容易,需要维护,但维护方便(换碳刷),产生电磁干扰,对环境有要求。无刷电机体积小,重量轻,出力大,响应快,速度高,惯量小,转动平滑,力矩稳定。控制复杂,容易实现智能化,其电子换相方式灵活,可以方波换相或正弦波换相。电机免维护,效率很高,运行温度低,电磁辐射很小,长寿命,可用于各种环境。 2. 交流伺服电机也是无刷电机,分为同步和异步电机,目前运动控制中一般都用同步电机,它的功率范围大,可以做到很大的功率。大惯量,最高转动速度低,且随着功率增大而快速降低。因而适合做低速平稳运行的应用。 3. 永磁交流伺服电动机简介 20 世纪80 年代以来,随着集成电路、电力电子技术和交流可变速驱动技术的发展,永磁交流伺服驱动技术有了突出的发展,各国著名电气厂商相继推出各自的交流伺服电动机和伺服驱动器系列产品并不断完善和更新。交流伺服系统已成为当代高性能伺服系统的主要发展方向,使原来的直流伺服面临被淘汰的危机。90 年代以后,世界各国已经商品化了的交流伺服系统是采用全数字控制的正弦
外文翻译中文
运作整合 供应链协作的首要问题是提高运作整合的程度。供应链协作课达到的好处,直接关系到捕捉效率之间的职能的企业,以及全国的企业,构成了国内或国际供应链。本章重点阐述的挑战,一体化管理,由研究为什么一体化创造价值,并通过详列的挑战,双方的企业集成和供应链整合。必不可少的供应链流程是确定的。注意的是,然后向信息技术提供,以方便集成化供应链规划。本章最后审查了定价。在最后的分析,定价的做法和政府是至关重要的供应链的连续性。 为什么整合创造价值 基本的优点与挑战的综合管理介绍了在第1章。进一步解释整合管理的重要性,有用的指出客户都至少有三个角度的价值。 传统的角度来看,价值是经济价值。第二个价值的角度来看,是市场价值。 实现双方经济和市场价值是很重要的客户。然而,越来越多的企业认识到商业上的成功也取决于第三个角度来看,价值,被称为关联性。 物流一体化目标 为实现物流一体化的供应链背景下,6个业务目标必须同时取得:( 1 )响应,( 2 )差额减少,( 3 )库存减少,( 4 )托运巩固,( 5 )质量,( 6 )生命周期支持。的相对重要性,每个直接关系到公司的物流战略。 响应 一公司的工作能力,以满足客户的要求,及时被称为反应。作为一再指出,信息技术是促进反应为基础的战略,允许业务的承诺被推迟到最后可能时间,其次是加速投放。实施对应策略服务,以减少库存承诺或部署在预期客户的需求。响应服务转向业务重点从预测未来的需求,以容纳顾客对快速订单到出货的基础上。理想的情况是,在一个负责任的系统中,库存是没有部署,直到客户承诺。支持这样的承诺,公司必须有物流的属性,库存的可用性和及时交付,一旦客户订单收到。 差异减少 所有经营领域的物流系统很容易受到差额。方差结果从未能履行任何预期的层面后勤业务不如预期。举例来说,毫不拖延地在客户订单处理,意想不到的干扰,以便选择,抵港货物损坏,在客户的位置,和/或未能提供在适当的位置上的时间,所有创造无计划的差异,在订单到交货周期。一个共同的解决办法,以保障对不利的差异是使用库存安全库存,以缓冲行动。这亦是共同使用的首选运输,以克服意想不到的差异延误交货计划。这种做法,鉴于其相关的成本高,可以尽量减少使用资讯科技,以维持积极的物流控制。向程度的差异是最小化,物流的生产力将提高。因此,差异减少,消除系统中断,是一个基本的目标,综合物流管理。 库存减少 要达到的目标,库存减少,一个综合物流系统必须控制资产的承诺,并把速度。资产的承诺,是财政的价值部署清单。把速度,反映了利率,这是充实库存随着时间的推移。高转率,再加上预期的库存供货,平均资产用于库存正在迅速而有效利用,这就是整体资产承诺支持一个综合运作减至最低。 库存能够而且确实方便可取的好处这是很重要的要请记住。库存是至关重要的实现规模经济,在制造业和采购。目的是要减少和管理存货,以尽可能最低的水平,同时实现整体供应链绩效的目标。
5G无线通信网络中英文对照外文翻译文献
5G无线通信网络中英文对照外文翻译文献(文档含英文原文和中文翻译)
翻译: 5G无线通信网络的蜂窝结构和关键技术 摘要 第四代无线通信系统已经或者即将在许多国家部署。然而,随着无线移动设备和服务的激增,仍然有一些挑战尤其是4G所不能容纳的,例如像频谱危机和高能量消耗。无线系统设计师们面临着满足新型无线应用对高数据速率和机动性要求的持续性增长的需求,因此他们已经开始研究被期望于2020年后就能部署的第五代无线系统。在这篇文章里面,我们提出一个有内门和外门情景之分的潜在的蜂窝结构,并且讨论了多种可行性关于5G无线通信系统的技术,比如大量的MIMO技术,节能通信,认知的广播网络和可见光通信。面临潜在技术的未知挑战也被讨论了。 介绍 信息通信技术(ICT)创新合理的使用对世界经济的提高变得越来越重要。无线通信网络在全球ICT战略中也许是最挑剔的元素,并且支撑着很多其他的行业,它是世界上成长最快最有活力的行业之一。欧洲移动天文台(EMO)报道2010年移动通信业总计税收1740亿欧元,从而超过了航空航天业和制药业。无线技术的发展大大提高了人们在商业运作和社交功能方面通信和生活的能力无线移动通信的显著成就表现在技术创新的快速步伐。从1991年二代移动通信系统(2G)的初次登场到2001年三代系统(3G)的首次起飞,无线移动网络已经实现了从一个纯粹的技术系统到一个能承载大量多媒体内容网络的转变。4G无线系统被设计出来用来满足IMT-A技术使用IP面向所有服务的需求。在4G系统中,先进的无线接口被用于正交频分复用技术(OFDM),多输入多输出系统(MIMO)和链路自适应技术。4G无线网络可支持数据速率可达1Gb/s的低流度,比如流动局域无线访问,还有速率高达100M/s的高流速,例如像移动访问。LTE系统和它的延伸系统LTE-A,作为实用的4G系统已经在全球于最近期或不久的将来部署。 然而,每年仍然有戏剧性增长数量的用户支持移动宽频带系统。越来越多的
双闭环直流调速系统外文翻译
对直流电机的速度闭环控制系统的设计 钟国梁 机械与汽车工程学院华南理工大学 中国,广州510640 电子邮件:zhgl2chl@https://www.360docs.net/doc/0118744912.html, 机械与汽车工程学院 华南理工大学 中国,广州510640 江梁中 电子邮件:jianglzh88@https://www.360docs.net/doc/0118744912.html, 该研究是由广州市科技攻关项目赞助(No.2004A10403006)。(赞助信息) 摘要 本文介绍了直流电机的速度控制原理,阐述了速度控制PIC16F877单片机作为主控元件,利用捕捉模块的特点,比较模块和在PIC16F877单片机模数转换模块将触发电路,并给出了程序流程图。系统具有许多优点,包括简单的结构,与主电路同步,稳定的移相和足够的移相范围,10000步控制的角度,对电动机的无级平滑控制,陡脉冲前沿,足够的振幅值,设定脉冲宽度,良好的稳定性和抗干扰性,并且成本低廉,这速度控制具有很好的实用价值,系统可以容易地实现。 关键词:单片机,直流电机的速度控制,控制电路,PI控制算法
1.简介 电力电子技术的迅速发展使直流电机的转速控制逐步从模拟转向数字,目前,广泛采用晶闸管直流调速传动系统设备(如可控硅晶闸管,SCR )在电力拖动控制系统对电机供电已经取代了笨重的F-D 发电机电动机系统,尤其是单片机技术的应用使速度控制直流电机技术进入一个新阶段。在直流调速系统中,有许多各种各样的控制电路。单片机具有高性能,体积小,速度快,优点很多,价格便宜和可靠的稳定性,广泛的应用和强劲的流通,它可以提高控制能力和履行的要求实时控制(快速反应)。控制电路采用模拟或数字电路可以实现单片机。在本文中,我们将介绍一种基于单片机PIC16F877单片机的直流电机速度控制系统的分类。 2.直流电机的调速原理 在图1中,电枢电压a U ,电枢电流a I ,电枢回路总电阻a R ,电机 常数a C 和励磁磁通Φ,根据KVL 方程,电机的转速为 Φ-= a a a a C R I U n a pN C 60= a a a a U R I U ≈- )1(63.0)(84.0)1()1()()1()(10--+-=--+-=k e k e k T k e a k e a k T k T d d d d i l T T Tf Kp a T T Kp a +==+ =10)1(
通信工程移动通信中英文对照外文翻译文献
中英文翻译 附件1:外文资料翻译译文 通用移动通信系统的回顾 1.1 UMTS网络架构 欧洲/日本的3G标准,被称为UMTS。 UMTS是一个在IMT-2000保护伞下的ITU-T 批准的许多标准之一。随着美国的CDMA2000标准的发展,它是目前占主导地位的标准,特别是运营商将cdmaOne部署为他们的2G技术。在写这本书时,日本是在3G 网络部署方面最先进的。三名现任运营商已经实施了三个不同的技术:J - PHONE 使用UMTS,KDDI拥有CDMA2000网络,最大的运营商NTT DoCoMo正在使用品牌的FOMA(自由多媒体接入)系统。 FOMA是基于原来的UMTS协议,而且更加的协调和标准化。 UMTS标准被定义为一个通过通用分组无线系统(GPRS)和全球演进的增强数据
技术(EDGE)从第二代GSM标准到UNTS的迁移,如图。这是一个广泛应用的基本原理,因为自2003年4月起,全球有超过847万GSM用户,占全球的移动用户数字的68%。重点是在保持尽可能多的GSM网络与新系统的操作。 我们现在在第三代(3G)的发展道路上,其中网络将支持所有类型的流量:语音,视频和数据,我们应该看到一个最终的爆炸在移动设备上的可用服务。此驱动技术是IP协议。现在,许多移动运营商在简称为2.5G的位置,伴随GPRS的部署,即将IP骨干网引入到移动核心网。在下图中,图2显示了一个在GPRS网络中的关键部件的概述,以及它是如何适应现有的GSM基础设施。 SGSN和GGSN之间的接口被称为Gn接口和使用GPRS隧道协议(GTP的,稍后讨论)。引进这种基础设施的首要原因是提供连接到外部分组网络如,Internet或企业Intranet。这使IP协议作为SGSN和GGSN之间的运输工具应用到网络。这使得数据服务,如移动设备上的电子邮件或浏览网页,用户被起诉基于数据流量,而不是时间连接基础上的数据量。3G网络和服务交付的主要标准是通用移动通信系统,或UMTS。首次部署的UMTS是发行'99架构,在下面的图3所示。 在这个网络中,主要的变化是在无线接入网络(RAN的)CDMA空中接口技术的引进,和在传输部分异步传输模式作为一种传输方式。这些变化已经引入,主要是为了支持在同一网络上的语音,视频和数据服务的运输。核心网络保持相对不变,主要是软件升级。然而,随着目前无线网络控制器使用IP与3G的GPRS业务支持节点进行通信,IP协议进一步应用到网络。 未来的进化步骤是第4版架构,如图4。在这里,GSM的核心被以语音IP技术为基础的IP网络基础设施取代。 海安的发展分为两个独立部分:媒体网关(MGW)和MSC服务器(MSS)的。这基本上是打破外连接的作用和连接控制。一个MSS可以处理多个MGW,使网络更具有扩展性。 因为现在有一些在3G网络的IP云,合并这些到一个IP或IP/ ATM骨干网是很有意义的(它很可能会提供两种选择运营商)。这使IP权利拓展到整个网络,一直到BTS(基站收发信台)。这被称为全IP网络,或推出五架构,如图五所示。在HLR/ VLR/VLR/EIR被推广和称为HLR的子系统(HSS)。 现在传统的电信交换的最后残余被删除,留下完全基于IP协议的网络运营,并
外文翻译通信工程英文的毕业设计论文
编号: 毕业设计(论文)外文翻译 (译文) 学院:信息与通信学院 专业:通信工程 学生姓名: 学号: 指导教师单位:信息与通信学院 姓名: 职称: 2014年 2 月9 日 Radio network planning process and methods for WCDMA Abstract This paper describes the system dimensioning and the radio network planning methodology for a third generation WCDMA system. The applicability of each method is demonstrated using examples of likely system scenarios. The challenges of
modeling the multiservice environment are described and the implications to the system performance simulations are introduced. Keywords: Telecommunication network planning, Mobile radio- communication, Code division multiple access, Wide band transmission, Multiple service network, Dimensioning, Simulator, Static model, Dynamic model, Cellular network. Resume(?) Contents I.Introduction II. Initial planning, system dimensioning III. Detailed planning process IV. Example dimensioning case and verification of dimensioning with static simulations V. Comparison of a static to a dynamic network simulator VI. Conclusion INTRODUCTION As the launch of third generation technology approaches, operators are forming strategies for the deployment of their networks. These strategies must be supported by realistic business plans both in terms of future service demand estimates and the requirement for investment in network infrastructure. Evaluating the requirement for network infrastructure can be achieved using system dimensioning tools capable of assessing both the radio access and the core network components. Having found an attractive business opportunity, system deployment must be preceded by careful network planning. The network planning tool must be capable of accurately modeling the system behaviour when loaded with the expected traffic profile. The third generation cellular systems will offer services well beyond the capabilities of today's networks. The traffic profile, as well as the radio access technology itself form the two most significant challenges when dimensioning and planning a WCDMA based third generation system. The traffic profile describes the mixture of services being used by the population of users. There are also specific system functionalities which must be modelled including fast power control and soft handover. In order to accurately predict the radio coverage the system eatures associated with WCDMA must be taken into account in the network modeling process. Especially the channel characterization, and interference control mechanisms in the case of any CDMA system must be considered. In WCDMA network multiple services co-exist. Different services (voice, data) have different processing gains, Eb/N0 performance and thus different receiver SNR requirements. In addition to those the WCDMA coverage depends on the load characterization, hand over parameterization, and power control effects. In current second generation systems' coverage planning processes the base station sensitivity is constant and the coverage threshold is the same for each base station. In the case of WCDMA the coverage threshold is dependent on the number of users and used bit rates in all cells, thus it
电力电子技术的发展及应用
均是精品,欢迎下载学习!!! 电力电子技术的发展及应用 朱磊1侯振义1张开2 (空军工程大学电讯工程学院陕西西安710077) (南京理工大学动力工程学院江苏南京210000) 摘要:本文通过介绍电力电子技术的发展及应用,阐述了电力电子技术在国民经济中的重要作用,结合国家政策,描绘出我国电力电子行业的大好前景。 关键词:电力电子技术功率器件逆变能源 电力电子技术,又称功率电子技术。它主要研究各种电力电子器件,以及这些电力电子器件所构成的各种各样高效地完成对电能的变换和控制的电路或装置。它既是电子学在强电(高电压、大电流)或电工领域的一个分支,又是电工学在弱电(低电压、大电流)或电子领域的一个分支,总之是强弱电相结合的新学科。 1 电力电子技术的发展 电力电子技术的发展与功率器件的发展密切相关,1948年普通晶体管的发明引起了电子工业革命,1957年第一只晶闸管的问世,为电力电子技术的诞生奠定了基础。 1.1 电力电子技术的晶闸管时代 由于大功率硅整流器能够高效率的把工频交流电转变为直流电,因此在60年代和70年代,大功率硅整流管和晶闸管的开发与应用得到大发展,这一时期称之为电力电子技术的晶闸管时代。 1.2 电力电子技术的逆变时代 20世纪70年代,随着自关断器件的出现,电力电子技术进入了逆变时代。七十年代出现了世界范围的能源危机,交流电机变频调速因节能效果显著而迅速发展。在70年代到80年代,随着变频调速装置的普及,大功率逆变用的晶闸管、巨型功率晶体管(GTR)和门极可关断晶闸管(GT0)成为当时电力电子器件的主角。这时的电力电子技术已经能够实现整流和逆变,但工作频率较低,仅局限在中低频范围内。 1.3现代电力电子时代 80年代末期和90年代初期发展起来的以功率MOSFET和IGBT为代表的集高频、高压和大电流于一身的功率半导体复合器件,使以低频技术处理问题为主的传统电力电子学,向以高频技术处理问题为主的现代电力电子学转变创造了条件,表明传统电力电子技术已经进入现代电力电子时代。 这一时期,各种新型器件应用大规模集成电路技术,向复合化、模块化的方向发展,使得器件及结构紧凑、体积缩小,并且能够综合了不同器件的优点。在性能上,器件的容量不断增大,工作频率不断提高,目前,市场化的碳化硅器件达(3500V\1200A),智能功率模块达到(1200V\800A),在斩波器的PWM开关频率可达1MHz。 这一时期,各种新的控制方法得到了广泛应用,特别是现代电力电子技术越来越多地运用了人工智能技术。在所有人工智能学科中,神经网络将对电力电子学产生的影响最大,利用混合人工智能技术(神经一模糊,神经一遗传,神经一模糊一遗传,模糊一遗传)开发强大的智能控制以及估计方法,单个神经模糊专用集成芯片能承担无传感器矢量控制,且具有在线故障诊断和容错控制能力。基于人工智能的模糊控制在参数变化和负载转矩扰动的非线性反馈系统中可能可以提供最好的鲁棒性,在故障监测和故障耐力控制中将会起到越来越重要的作用。 2电力电子的技术应用 随着科技的不断发展和人们要求的不断提高,电力电子技术的应用越来越广泛。当今世界先进工业国家正处于由“工业经济”模式向“信息经济”模式转变的时期。电力电子技术作为信息产业与传统产业之间的桥梁,是在非常广泛的领域内改造传统产业、支持高新技术发展的基础。因此,电力电子技术将在国民经济中扮演着越来越重要的角色。
外文翻译中文版(完整版)
毕业论文外文文献翻译 毕业设计(论文)题目关于企业内部环境绩效审计的研究翻译题目最高审计机关的环境审计活动 学院会计学院 专业会计学 姓名张军芳 班级09020615 学号09027927 指导教师何瑞雄
最高审计机关的环境审计活动 1最高审计机关越来越多的活跃在环境审计领域。特别是1993-1996年期间,工作组已检测到环境审计活动坚定的数量增长。首先,越来越多的最高审计机关已经活跃在这个领域。其次是积极的最高审计机关,甚至变得更加活跃:他们分配较大部分的审计资源给这类工作,同时出版更多环保审计报告。表1显示了平均数字。然而,这里是机构间差异较大。例如,环境报告的数量变化,每个审计机关从1到36份报告不等。 1996-1999年期间,结果是不那么容易诠释。第一,活跃在环境审计领域的最高审计机关数量并没有太大变化。“活性基团”的组成没有保持相同的:一些最高审计机关进入,而其他最高审计机关离开了团队。环境审计花费的时间量略有增加。二,但是,审计报告数量略有下降,1996年和1999年之间。这些数字可能反映了从量到质的转变。这个信号解释了在过去三年从规律性审计到绩效审计的转变(1994-1996年,20%的规律性审计和44%绩效审计;1997-1999:16%规律性审计和绩效审计54%)。在一般情况下,绩效审计需要更多的资源。我们必须认识到审计的范围可能急剧变化。在将来,再将来开发一些其他方式去测算人们工作量而不是计算通过花费的时间和发表的报告会是很有趣的。 在2000年,有62个响应了最高审计机关并向工作组提供了更详细的关于他们自1997年以来公布的工作信息。在1997-1999年,这62个最高审计机关公布的560个环境审计报告。当然,这些报告反映了一个庞大的身躯,可用于其他机构的经验。环境审计报告的参考书目可在网站上的最高审计机关国际组织的工作组看到。这里这个信息是用来给最高审计机关的审计工作的内容更多一些洞察。 自1997年以来,少数环境审计是规律性审计(560篇报告中有87篇,占16%)。大多数审计绩效审计(560篇报告中有304篇,占54%),或组合的规律性和绩效审计(560篇报告中有169篇,占30%)。如前文所述,绩效审计是一个广泛的概念。在实践中,绩效审计往往集中于环保计划的实施(560篇报告中有264篇,占47%),符合国家环保法律,法规的,由政府部门,部委和/或其他机构的任务给访问(560篇报告中有212篇,占38%)。此外,审计经常被列入政府的环境管理系统(560篇报告中有156篇,占28%)。下面的元素得到了关注审计报告:影响或影响现有的国家环境计划非环保项目对环境的影响;环境政策;由政府遵守国际义务和承诺的10%至20%。许多绩效审计包括以上提到的要素之一。 1本文译自:S. Van Leeuwen.(2004).’’Developments in Environmental Auditing by Supreme Audit Institutions’’ Environmental Management Vol. 33, No. 2, pp. 163–1721
通信工程专业Code-division-multiple-access码分多址大学毕业论文外文文献翻译及原文
毕业设计(论文)外文文献翻译 文献、资料中文题目:码分多址 文献、资料英文题目:Code division multiple access 文献、资料来源: 文献、资料发表(出版)日期: 院(部): 专业: 班级: 姓名: 学号: 指导教师: 翻译日期:2017.02.14
外文原文 Code division multiple access Code division multiple access (CDMA) is a channel access method used by various radio communication technologies. It should not be confused with the mobile phone standards called cdmaOne, CDMA2000 (the 3G evolution of cdmaOne) and WCDMA (the 3G standard used by GSM carriers), which are often referred to as simply CDMA, and use CDMA as an underlying channel access method. One of the concepts in data communication is the idea of allowing several transmitters to send information simultaneously over a single communication channel. This allows several users to share a band of frequencies (see bandwidth). This concept is called multiple access. CDMA employs spread-spectrum technology and a special coding scheme (where each transmitter is assigned a code) to allow multiple users to be multiplexed over the same physical channel. By contrast, time division multiple access (TDMA) divides access by time, while frequency-division multiple access (FDMA) divides it by frequency. CDMA is a form of spread-spectrum signalling, since the modulated coded signal has a much higher data bandwidth than the data being communicated. Steps in CDMA Modulation Each user in a CDMA system uses a different code to modulate their signal. Choosing the codes used to modulate the signal is very important in the performance of CDMA systems. The best performance will occur when there is good separation between the signal of a desired user and the signals of other users. The separation of the signals is made by correlating the received signal with the locally generated code of the desired user. If the signal matches the desired user's code then the correlation function will be high and the system can extract that signal. If the desired user's code has nothing in common with the signal the correlation should be as close to zero as