外文翻译---利用冶金渣副产物去除酸性矿山废水中的污染物
治理酸性矿山废水的方法
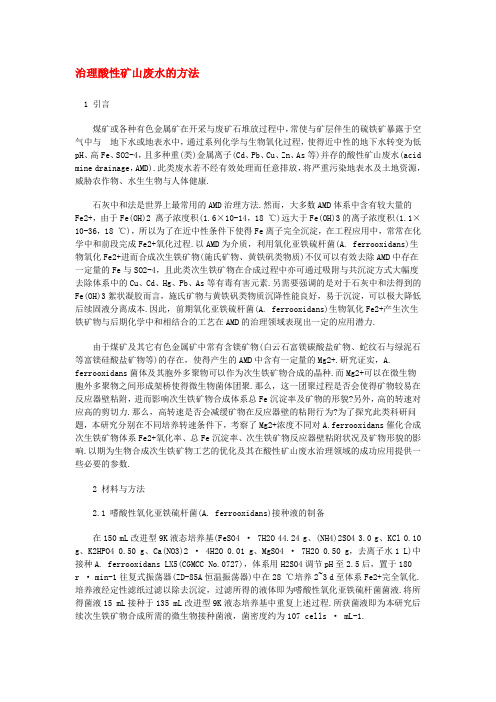
治理酸性矿山废水的方法1 引言煤矿或各种有色金属矿在开采与废矿石堆放过程中,常使与矿层伴生的硫铁矿暴露于空气中与地下水或地表水中,通过系列化学与生物氧化过程,使得近中性的地下水转变为低pH、高Fe、SO2-4,且多种重(类)金属离子(Cd、Pb、Cu、Zn、As等)并存的酸性矿山废水(acid mine drainage,AMD).此类废水若不经有效处理而任意排放,将严重污染地表水及土地资源,威胁农作物、水生生物与人体健康.石灰中和法是世界上最常用的AMD治理方法.然而,大多数AMD体系中含有较大量的Fe2+,由于Fe(OH)2 离子浓度积(1.6×10-14,18 ℃)远大于Fe(OH)3的离子浓度积(1.1×10-36,18 ℃),所以为了在近中性条件下使得Fe离子完全沉淀,在工程应用中,常常在化学中和前段完成Fe2+氧化过程.以AMD为介质,利用氧化亚铁硫杆菌(A. ferrooxidans)生物氧化Fe2+进而合成次生铁矿物(施氏矿物、黄铁矾类物质)不仅可以有效去除AMD中存在一定量的Fe与SO2-4,且此类次生铁矿物在合成过程中亦可通过吸附与共沉淀方式大幅度去除体系中的Cu、Cd、Hg、Pb、As等有毒有害元素.另需要强调的是对于石灰中和法得到的Fe(OH)3絮状凝胶而言,施氏矿物与黄铁矾类物质沉降性能良好,易于沉淀,可以极大降低后续固液分离成本.因此,前期氧化亚铁硫杆菌(A. ferrooxidans)生物氧化Fe2+产生次生铁矿物与后期化学中和相结合的工艺在AMD的治理领域表现出一定的应用潜力.由于煤矿及其它有色金属矿中常有含镁矿物(白云石富镁碳酸盐矿物、蛇纹石与绿泥石等富镁硅酸盐矿物等)的存在,使得产生的AMD中含有一定量的Mg2+.研究证实,A. ferrooxidans菌体及其胞外多聚物可以作为次生铁矿物合成的晶种.而Mg2+可以在微生物胞外多聚物之间形成架桥使得微生物菌体团聚.那么,这一团聚过程是否会使得矿物较易在反应器壁粘附,进而影响次生铁矿物合成体系总Fe沉淀率及矿物的形貌?另外,高的转速对应高的剪切力.那么,高转速是否会减缓矿物在反应器壁的粘附行为?为了探究此类科研问题,本研究分别在不同培养转速条件下,考察了Mg2+浓度不同对A.ferrooxidans催化合成次生铁矿物体系Fe2+氧化率、总Fe沉淀率、次生铁矿物反应器壁粘附状况及矿物形貌的影响.以期为生物合成次生铁矿物工艺的优化及其在酸性矿山废水治理领域的成功应用提供一些必要的参数.2 材料与方法2.1 嗜酸性氧化亚铁硫杆菌(A. ferrooxidans)接种液的制备在150 mL改进型9K液态培养基(FeSO4 · 7H2O 44.24 g、(NH4)2SO4 3.0 g、KCl 0.10 g、K2HPO4 0.50 g、Ca(NO3)2 · 4H2O 0.01 g、MgSO4 · 7H2O 0.50 g,去离子水1 L)中接种A. ferrooxidans LX5(CGMCC No.0727),体系用H2SO4调节pH至2.5后,置于180r · min-1往复式振荡器(ZD-85A恒温振荡器)中在28 ℃培养2~3 d至体系Fe2+完全氧化.培养液经定性滤纸过滤以除去沉淀,过滤所得的液体即为嗜酸性氧化亚铁硫杆菌菌液.将所得菌液15 mL接种于135 mL改进型9K液态培养基中重复上述过程.所获菌液即为本研究后续次生铁矿物合成所需的微生物接种菌液,菌密度约为107 cells · mL-1.2.2 生物合成次生铁矿物试验在一系列250 mL锥形瓶中分别盛放制备好的A. ferrooxidans LX5接种液15 mL,①加入浓缩10倍的改进型9K液体培养基(Mg2+浓度为480 mg · L-1,以MgSO4 · 7H2O形式加入)15 mL,后补充去离子水至溶液总体积为150 mL,使得体系Mg2+浓度为48mg · L-1(记作“Mg2+-48 mg · L-1”体系);②其它试验设计同处理①,而体系Mg2+设计浓度为4.8 mg · L-1(记作“Mg2+-4.8 mg · L-1”体系).用H2SO4将上述pH调至2.50,分别将混合液在28 ℃,180 r · min-1或100 r · min-1条件下振荡培养,每个处理设置3个重复.每12 h监测体系pH值,且从体系均匀取样1 mL,过0.45 μm滤膜,测定滤液Fe2+及总Fe浓度,进而计算Fe2+氧化率及总Fe沉淀率.待体系Fe2+氧化完全后将不同体系产生的矿物沉淀用定性滤纸收集,酸化的去离子水(pH=2.0)洗3次,再用去离子水洗涤2次后,在50 ℃环境中烘干,分析矿物的矿相及形貌.2.3 测定方法溶液pH用PHS-3C型酸度计测定,Fe2+与总Fe浓度采用邻菲罗啉比色法进行分析. t 时刻Fe2+氧化率=(C (Fe2+)0-C (Fe2+)t)/ C (Fe2+)0×100%,式中,C (Fe2+)0与C (Fe2+)t 分别为反应初始和反应t小时体系Fe2+浓度.t时刻总Fe沉淀率=(C (TFe)0-C (TFe)t)/ C (TFe)0×100%,式中C (TFe)0与C (TFe)t分别为反应初始和反应t小时体系总Fe浓度.次生铁矿物矿相用X射线衍射仪(XRD,MiniFles II,日本理学)测定,测试工作条件为:管电压30 kV,管电流15 mA,扫描区间10~70°(2θ),步长0.02°,Cu靶(弯晶单色器).次生铁矿物形貌采用热场发射扫描电子显微镜(SEM,JSM-7001F)观察,工作距离(样品表面到物镜的距离)9.7 mm,加速电压5.0 kV.3 结果与分析3.1 不同培养转速下Mg2+对生物合成次生铁矿物体系pH的影响Fe2+生物氧化为Fe3+是一个能使体系pH升高的过程,后续Fe3+水解产生次生铁矿物却是pH降低的过程.180 r · min-1或100 r · min-1的培养转速下,“Mg2+-4.8 mg · L-1”与“Mg2+-48 mg · L-1”两生物合成次生铁矿物体系pH随时间的变化情况如图 1所示.可以得出,转速对体系Fe2+生物氧化至Fe3+及后续Fe3+水解过程有明显的影响.当培养转速为180 r · min-1时,“Mg2+-4.8 mg · L-1”生物合成次生铁矿物体系pH首先从0 h的~2.50升高至12 h的 ~2.65,后逐渐降低至48 h的~2.07.“Mg2+-48 mg · L-1”体系在此培养转速条件下,pH首先从0 h的~2.50升高至12 h的 ~2.66,后逐渐降低至48 h的~2.12.然而,当培养转速为100 r · min-1时,体系pH下降速度相对缓慢,“Mg2+-4.8 mg · L-1”体系与“Mg2+-48 mg · L-1”体系pH在12 h均达到~2.69,后逐渐分别降低至72 h的~2.21与~2.17.图 1 A. ferrooxidans催化合成次生铁矿物体系不同培养转速条件下pH变化情况整体而言,不同培养转速下,“Mg2+-4.8 mg · L-1”与“Mg2+-48 mg · L-1”体系pH变化差异主要表现在pH下降阶段.然而,“Mg2+-4.8 mg · L-1”与“Mg2+-48 mg · L-1”两体系pH降低快慢趋势却随培养转速的不同而不尽相同.在培养转速为180 r · min-1条件下,“Mg2+-4.8 mg · L-1”体系pH下降速度要快于“Mg2+-48 mg · L-1”体系,而当培养转速为100 r · min-1 时,后者pH下降速度却快于前者.3.2 不同培养转速下Mg2+对生物合成次生铁矿物体系Fe2+氧化率的影响体系Fe2+有效氧化是后续Fe3+水解产生次生铁矿物的前提条件.本研究中,“Mg2+-4.8 mg · L-1”与“Mg2+-48 mg · L-1”两生物合成次生铁矿物体系在180 r · min-1或100 r · min-1的培养转速下,Fe2+氧化率随时间的变化趋势见图 2.图 2 A. ferrooxidans催化合成次生铁矿物体系不同培养转速条件下Fe2+氧化率变化趋势从图 2可以得出,当培养转速为180 r · min-1 时,“Mg2+-4.8 mg · L-1”与“Mg2+-48 mg · L-1”两体系均可在48 h内实现Fe2+完全氧化.而当转速降低至100 r · min-1 时,两体系Fe2+完全氧化时间要延长至72 h.然而,任一体系次生铁矿物合成过程中,Fe2+氧化率随培养时间均呈现“S型”变化趋势.例如,“Mg2+-4.8 mg · L-1”体系在180 r · min-1的培养条件下,Fe2+氧化率在前12 h仅为9.1%,12~36 h 培养过程中,Fe2+氧化率聚增至97%,体系培养至48 h时,Fe2+氧化完全.同时可以发现,当培养转速为180 r · min-1时,“Mg2+-4.8 mg · L-1”与“Mg2+-48 mg · L-1”两体系Fe2+氧化率在0~12 h与36~48 h“首尾”培养期间并不存在明显差异.而在12~36 h 过程中,“Mg2+-4.8 mg · L-1”体系Fe2+氧化率要明显快于“Mg2+-48 mg · L-1”体系.例如,在培养至24 h时,“Mg2+-4.8 mg · L-1”体系Fe2+氧化率达到78.2%,而“Mg2+-48 mg · L-1”体系仅为50.8%.同样,当培养转速为100 r · min-1时,“Mg2+-4.8 mg · L-1”与“Mg2+-48 mg · L-1”两体系Fe2+氧化率在0~24 h与60~72 h“首尾”期间亦不存在明显差异.差异主要集中表现在24~60 h的培养过程中,然而,与180 r · min-1培养条件所得结果不同,此时“Mg2+-4.8 mg · L-1”体系Fe2+氧化率却稍慢于“Mg2+-48 mg · L-1”体系.例如,在36 h与48 h 时,“Mg2+-4.8 mg · L-1”体系Fe2+氧化率分别为33.2%与61.9%,而相应的“Mg2+-4.8mg · L-1”体系Fe2+氧化率却分别为40.0%与77.3%.3.3 不同培养转速下Mg2+对生物合成次生铁矿物体系总Fe沉淀率的影响一般而言,次生铁矿物合成体系总Fe沉淀率越高,意味着较多的Fe参与体系次生铁矿物的合成.所以,总Fe沉淀率变化情况能够直接反应体系次生铁矿物合成能力.此研究中,“Mg2+-4.8 mg · L-1”与“Mg2+-48 mg · L-1”两生物合成次生铁矿物体系在180 r · min-1或100 r · min-1培养转速下,总Fe沉淀率随时间变化趋势如图 3所示.图 3 A. ferrooxidans催化合成次生铁矿物体系不同培养转速条件下总Fe沉淀率变化趋势从图 3可以得出,当培养转速为180 r · min-1 时,“Mg2+-4.8 mg · L-1”与“Mg2+-48 mg · L-1”两体系培养前12 h总Fe沉淀率几乎可以忽略,而在后期培养过程中,两体系总Fe沉淀率均呈现逐渐增加趋势,然而增加的幅度却不尽一致.“Mg2+-4.8 mg · L-1”体系在12~48 h培养过程中,总Fe沉淀率从1.0%增加至37.4%.而“Mg2+-4.8 mg · L-1”体系在相应时间,总Fe沉淀率仅从1.0%增加至31.7%.前者总Fe沉淀率较后者提高近18.0%.而当培养转速为100 r · min-1 时,上述两矿物合成体系在培养前24 h总Fe沉淀率亦可以忽略,培养后期总铁沉淀率逐渐增加.培养至72 h 体系Fe2+完全氧化时刻,“Mg2+-4.8 mg · L-1”与“Mg2+-48 mg · L-1”两体系总Fe沉淀率分别为21.3%与23.0%,前者较后者降低7.4%.需要说明的是,相同矿物合成体系,高转速培养更利于总Fe沉淀转化为次生“Mg2+-4.8 mg · L-1”与“Mg2+-48 mg · L-1”两体系在180 r · min-1铁矿物.图 3结果表明,条件下培养至Fe2+完全氧化时,体系总Fe沉淀率较同体系在100 r · min-1培养条件下所得总Fe沉淀率增加了75.6%与37.8%.3.4 不同培养转速下Mg2+对生物合成次生铁矿物矿相的影响X射线衍射图谱(XRD)技术常常被用来分析矿物的矿相.本研究“Mg2+-4.8 mg · L-1”与“Mg2+-48 mg · L-1”体系在不同转速下培养,当Fe2+完全氧化时的矿物X射线衍射图谱见图 4.图 4 生物合成次生铁矿物体系亚铁完全氧化时矿物X射线衍射图谱本研究中,所有体系产生的次生铁矿物X射线衍射图谱出峰位置及相对强度近似一致.结合体系元素组成,参考矿物标准衍射图谱,发现本研究不同体系所得到的次生铁矿物衍射图谱中主要尖锐强峰(“J”标注)出峰位置及相对强度与标准图谱中黄铁矾类物质(黄钾铁矾:No.22-0827;黄铵铁矾:No.26-1014;草黄铁矾:No.31-0650)相关参数相一致.研究得到次生铁矿物特有的微弱宽峰(“S标注”)与标准图谱中标准施氏矿物类物质(No.47-1775)相关参数近似一致.故可以判定,本研究所有体系产生的次生铁矿物均应为黄铁矾与施氏矿物共存的混合物.3.5 不同培养转速下Mg2+对生物合成次生铁矿物存在形态的影响本研究中“Mg2+-4.8 mg · L-1”与“Mg2+-48 mg · L-1”两处理体系Fe2+完全氧化时刻,体系次生铁矿物实际形态见图 5.由图 5可以很直观的看出,当培养转速为180r · min-1且体系Fe2+完全氧化时,“Mg2+-4.8 mg · L-1”体系产生的约1.21 g的次生铁矿物均匀分散在培养液中.而“Mg2+-48 mg · L-1”体系矿物总产量为0.98 g,且约70%次生铁矿物却牢固粘附于摇瓶底部.当培养转速为100 r · min-1且体系Fe2+完全氧化时,“Mg2+-4.8 mg · L-1”与“Mg2+-48 mg · L-1”两处理体系产生的次生铁矿物均全部粘附于摇瓶底部,矿物产生量分别为0.66 g与0.74 g.图 5 合成次生铁矿物合成体系Fe2+完全氧化时矿物的存在形态(左: Mg2+-4.8 mg · L-1;右:Mg2+-48 mg · L-1,其它元素组成与9K培养基相同)不同体系次生铁矿物的存在状态在一定意义上决定着体系pH、Fe2+氧化率及总Fe沉淀率的变化趋势.前人研究证实,体系中存在的微生物细胞壁结构或胞外多聚物,与前期合成的次生铁矿物可以作为“晶种”加速矿物的后期合成,另外,研究表明,次生铁矿物对A. ferrooxidans存在一定量的吸附.本实验180 r · min-1培养条件,“Mg2+-4.8 mg · L-1”体系合成的矿物均匀分散于体系中,体系均匀分散的微生物菌体与前期合成的次生铁矿物均有利于后期矿物的进一步合成,进而加速体系总Fe沉淀率的增加及pH下降.而当体系Mg2+为48 mg · L-1时,体系合成的部分矿物不断粘附于摇瓶底部,前期合成的次生矿物及其所吸附的微生物菌体无法较好的为后期矿物合成提供模板,进而减缓体系总Fe沉淀率增加及pH的下降趋势.另外,粘附于摇瓶底部的矿物由于吸附固定一定量的A. ferrooxidans,进而减弱微生物对体系Fe2+的氧化能力,且这一推论在本研究3.2节部分结果中被很好验证.本实验100 r · min-1培养条件下,“Mg2+-4.8 mg · L-1”与“Mg2+-48 mg · L-1”两处理体系产生的次生铁矿物均全部粘附于摇瓶底部.所以此时对体系pH、Fe2+氧化率及总Fe沉淀率变化起主导作用的不再是矿物的存在状态,而是体系Mg2+的相对含量.Mg2+是微生物细胞内酶的重要成分,亦是A. ferrooxidans生长(或其生长培养基)必需元素之一,其含量的增加可能会在一定程度上提高A. ferrooxidans活性,进而可在一定程度上提高Fe2+氧化能力及Fe3+的水解速度,进而加速总Fe沉淀.笔者认为,本研究造成次生铁矿物在摇瓶底部粘附的主要原因是初期合成的矿物与微生物菌体或微生物胞外多聚物相互作用形成包裹体,在水力剪切条件不足的情况下逐渐沉积粘附于瓶底.本研究结果已表明,培养转速越低,矿物粘附在摇瓶底部的可能性亦越大,产生次生铁矿物量相对较少.另外,由于Mg2+可在微生物胞外多聚物间形成架桥而使得微生物菌体团聚,那么Mg2+在A. ferrooxidan胞外多聚物间的架桥作用使得A. ferrooxidans菌体团聚体增大,进而使得矿物与微生物相互作用形成更大的包裹体,以致增加矿物在瓶底沉积粘附的可能性,本研究培养转速为180 r · min-1 条件下,“Mg2+-4.8 mg · L-1”与“Mg2+-48 mg · L-1”两处理体系矿物存在形态可较好的佐证这一推断.具体参见污水宝商城资料或更多相关技术文档。
去除废水中的砷与硫酸盐 文献翻译解读

学校代码:学号:HENAN INSTITUTE OF ENGINEERING文献翻译题目去除炼铜工业废水中的砷与硫酸盐学生姓名李福举专业班级资源环境科学1241班学号201210410109院(部)资源与环境学院指导老师完成时间化学工程期刊141 (2008) 89–98去除炼铜工业废水中的砷与硫酸盐C. Ahmed Basha a,∗, S. Josephine Selvi b, E. Ramasamy c, S. Chellammal aa Central Electrochemical Research Institute, Karaikudi 630 006, Indiab R.V.S. Engineering College, Bangalore, Indiac Coimbatore Institute of Technology, Coimbatore, India摘要来自含砷矿石加工流程中的污废水可能有不同含量的三价砷,五价砷,氧离子,亚砷酸盐和砷酸盐。
现在工业采用的是砷酸铁沉淀法(铁盐法),使用这种方法产生的问题是有大量的污泥形成。
砷酸铁沉淀的有效pH范围为4—8,但是废水的pH仅为约0.6,硫酸盐的浓度会高一些。
因此,需要加入碱提高pH,使砷酸铁形成沉淀,也因此消耗了更多的碱。
化学物质的添加可能会提高总溶解固体的含量。
这项研究的目的在于去除来自冶金工业中通过电渗析,电离子交换以及电凝技术产生的废水中的砷化物(废水中包括其他重金属在内的污染物浓度范围为1000-2000mg/L)。
使用电渗析法,在电流密度为2A/dm2时,砷的去除率达到91.4%,硫酸盐达到37.1%。
使用电离子交换技术,在电流密度为3A/dm2时,砷的去除率达到58.2%,硫酸盐的去除率为72.7%。
使用电凝技术,在电流密度为1.5A/dm2时,砷的去除可达到低于原子吸收光谱仪的检出限。
通过结合电离子交换技术和电凝技术,可使提高pH所消耗的碱的量有效降至最小化。
酸性矿山废水(AMD)处理技术综述
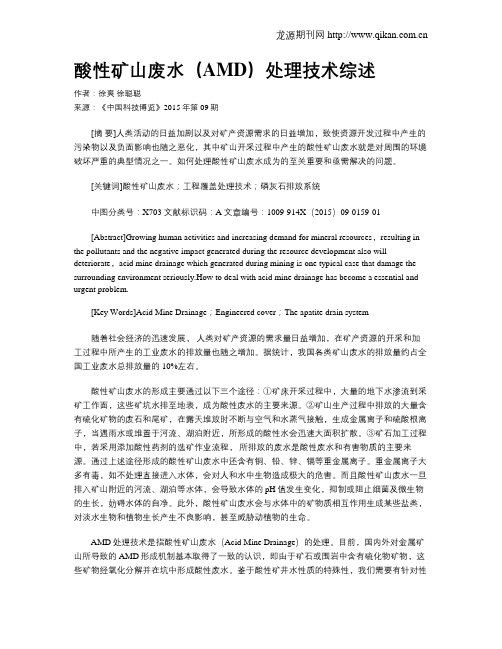
酸性矿山废水(AMD)处理技术综述作者:徐爽徐聪聪来源:《中国科技博览》2015年第09期[摘要]人类活动的日益加剧以及对矿产资源需求的日益增加,致使资源开发过程中产生的污染物以及负面影响也随之恶化,其中矿山开采过程中产生的酸性矿山废水就是对周围的环境破坏严重的典型情况之一。
如何处理酸性矿山废水成为的至关重要和亟需解决的问题。
[关键词]酸性矿山废水;工程覆盖处理技术;磷灰石排放系统中图分类号:X703 文献标识码:A 文章编号:1009-914X(2015)09-0159-01[Abstract]Growing human activities and increasing demand for mineral resources,resulting in the pollutants and the negative impact generated during the resource development also will deteriorate,acid mine drainage which generated during mining is one typical case that damage the surrounding environment seriously.How to deal with acid mine drainage has become a essential and urgent problem.[Key Words]Acid Mine Drainage;Engineered cover;The apatite drain system随着社会经济的迅速发展,人类对矿产资源的需求量日益增加,在矿产资源的开采和加工过程中所产生的工业废水的排放量也随之增加。
据统计,我国各类矿山废水的排放量约占全国工业废水总排放量的 10%左右。
酸性矿山废水的形成主要通过以下三个途径:①矿床开采过程中,大量的地下水渗流到采矿工作面,这些矿坑水排至地表,成为酸性废水的主要来源。
去除废水中的砷与硫酸盐 文献翻译解读

学校代码:学号:HENAN INSTITUTE OF ENGINEERING文献翻译题目去除炼铜工业废水中的砷与硫酸盐学生姓名李福举专业班级资源环境科学1241班学号201210410109院(部)资源与环境学院指导老师完成时间化学工程期刊141 (2008) 89–98去除炼铜工业废水中的砷与硫酸盐C. Ahmed Basha a,∗, S. Josephine Selvi b, E. Ramasamy c, S. Chellammal aa Central Electrochemical Research Institute, Karaikudi 630 006, Indiab R.V.S. Engineering College, Bangalore, Indiac Coimbatore Institute of Technology, Coimbatore, India摘要来自含砷矿石加工流程中的污废水可能有不同含量的三价砷,五价砷,氧离子,亚砷酸盐和砷酸盐。
现在工业采用的是砷酸铁沉淀法(铁盐法),使用这种方法产生的问题是有大量的污泥形成。
砷酸铁沉淀的有效pH范围为4—8,但是废水的pH仅为约0.6,硫酸盐的浓度会高一些。
因此,需要加入碱提高pH,使砷酸铁形成沉淀,也因此消耗了更多的碱。
化学物质的添加可能会提高总溶解固体的含量。
这项研究的目的在于去除来自冶金工业中通过电渗析,电离子交换以及电凝技术产生的废水中的砷化物(废水中包括其他重金属在内的污染物浓度范围为1000-2000mg/L)。
使用电渗析法,在电流密度为2A/dm2时,砷的去除率达到91.4%,硫酸盐达到37.1%。
使用电离子交换技术,在电流密度为3A/dm2时,砷的去除率达到58.2%,硫酸盐的去除率为72.7%。
使用电凝技术,在电流密度为1.5A/dm2时,砷的去除可达到低于原子吸收光谱仪的检出限。
通过结合电离子交换技术和电凝技术,可使提高pH所消耗的碱的量有效降至最小化。
大宝山矿业酸性矿山废水的污染与治理技术研究

T echnology技 术大宝山矿业酸性矿山废水的污染与治理技术研究Research on Pollution and Treatment Technology of Acid Mine Wastewater inDabaoshan Mining Industry文/廖正家echnology 技 术62T流入自然水体、用于农田灌溉都会给自然界及人类带来极大的负面影响。
AMD 的末端治理也是针对这两方面的问题进行,国内外常用的处理酸性废水中重金属的方法主要有物理法、化学法、生物化学法,除了这些传统的方法之外,近年来还出现人工湿地法、电化学处理等新技术。
2.2.1 物理法吸附法、离子交换法都属于比较常见的物理法。
吸附法结合多孔吸附材料对废水中的重金属进行处理,活性炭起到了吸附剂的作用。
离子交换法利用交换树脂可以对水中的重金属进行去除并且能够有效回收重金属。
膜分离法结合特殊薄膜选择水体的污染物通过这种方式处理水中重金属,透析、超滤、纳滤等技术都是比较常用的技术。
通过研究发现结合反渗透膜技术进行处理的重金属去除率在99.5%左右;利用纳滤膜技术对初始浓度均为5mg/L 的Ni2+和Cd2+的去除率可达98.85%和82.69%,处理效果好。
2.2.2 化学法(1)中和法是指通过往AMD 中投加碱中和剂(碱石灰、消石灰、碳酸钙等),促使废水中重金属离子与OH-反应生成难溶或不溶的氢氧化物沉淀而分离去除。
该方法具有工艺成熟简单,中和剂便宜易获取,处理效率高,效果稳定等优势,成为AMD 处理技术中最常用的方法,工程使用率占90%以上。
(2)硫化物沉淀法是基于重金属离子与硫离子之间的强亲和力,能够生成溶解度很小且稳定的硫化物的基础上,结合硫化剂核NMD 中的重金属离子发生反应形成金属硫化沉淀,通过这种方式处理重金属。
(3)生物化学法这种方式是当前比较先进的一种处理方法,具有成本低、适用性强等特点,也是非常有潜力的一种处理方法。
工业废水处理流程步骤英语
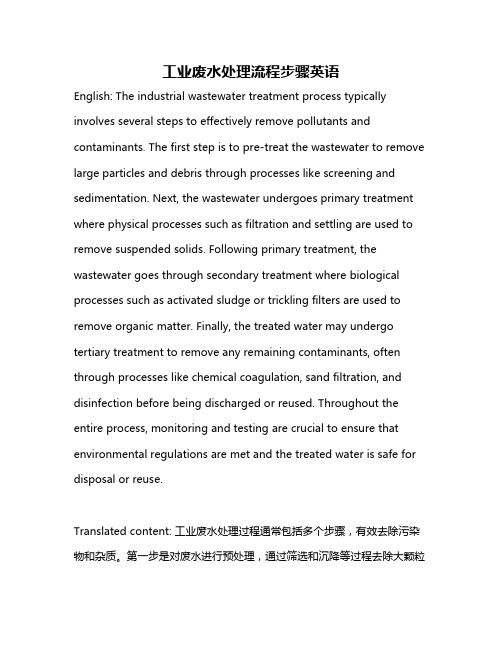
工业废水处理流程步骤英语English: The industrial wastewater treatment process typically involves several steps to effectively remove pollutants and contaminants. The first step is to pre-treat the wastewater to remove large particles and debris through processes like screening and sedimentation. Next, the wastewater undergoes primary treatment where physical processes such as filtration and settling are used to remove suspended solids. Following primary treatment, the wastewater goes through secondary treatment where biological processes such as activated sludge or trickling filters are used to remove organic matter. Finally, the treated water may undergo tertiary treatment to remove any remaining contaminants, often through processes like chemical coagulation, sand filtration, and disinfection before being discharged or reused. Throughout the entire process, monitoring and testing are crucial to ensure that environmental regulations are met and the treated water is safe for disposal or reuse.Translated content: 工业废水处理过程通常包括多个步骤,有效去除污染物和杂质。
钢渣去除酸性矿山废水中硫酸盐的机理研究
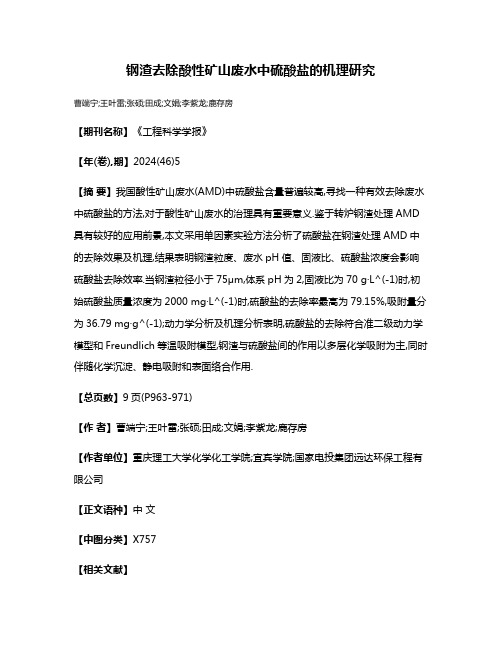
钢渣去除酸性矿山废水中硫酸盐的机理研究
曹端宁;王叶雷;张硕;田成;文娟;李紫龙;鹿存房
【期刊名称】《工程科学学报》
【年(卷),期】2024(46)5
【摘要】我国酸性矿山废水(AMD)中硫酸盐含量普遍较高,寻找一种有效去除废水中硫酸盐的方法,对于酸性矿山废水的治理具有重要意义.鉴于转炉钢渣处理AMD 具有较好的应用前景,本文采用单因素实验方法分析了硫酸盐在钢渣处理AMD中的去除效果及机理,结果表明钢渣粒度、废水pH值、固液比、硫酸盐浓度会影响硫酸盐去除效率.当钢渣粒径小于75μm,体系pH为2,固液比为70 g·L^(-1)时,初始硫酸盐质量浓度为2000 mg·L^(-1)时,硫酸盐的去除率最高为79.15%,吸附量分为36.79 mg·g^(-1);动力学分析及机理分析表明,硫酸盐的去除符合准二级动力学模型和Freundlich等温吸附模型,钢渣与硫酸盐间的作用以多层化学吸附为主,同时伴随化学沉淀、静电吸附和表面络合作用.
【总页数】9页(P963-971)
【作者】曹端宁;王叶雷;张硕;田成;文娟;李紫龙;鹿存房
【作者单位】重庆理工大学化学化工学院;宜宾学院;国家电投集团远达环保工程有限公司
【正文语种】中文
【中图分类】X757
【相关文献】
1.脱硫酸菌去除煤矿酸性废水中硫酸盐的初步研究
2.硫酸盐还原菌去除酸性矿水中金属铜离子的实验研究
3.硫酸盐还原菌法固定酸性矿山废水中重金属的研究进展
4.利用硫酸盐还原菌去除矿山废水中污染物试验研究
5.钢渣去除染料废水中酸性枣红吸附性能及机理
因版权原因,仅展示原文概要,查看原文内容请购买。
利用冶炼炉渣除去工业废水中砷锑的方法[发明专利]
![利用冶炼炉渣除去工业废水中砷锑的方法[发明专利]](https://img.taocdn.com/s3/m/e14ade0de009581b6bd9ebf0.png)
专利名称:利用冶炼炉渣除去工业废水中砷锑的方法专利类型:发明专利
发明人:孙红燕,刘卫,森维,刘贵阳,易中周,肖锐敏
申请号:CN201310539060.6
申请日:20131105
公开号:CN103553197A
公开日:
20140205
专利内容由知识产权出版社提供
摘要:本发明是一种利用冶炼炉渣除去工业废水中砷锑的方法,其步骤是在含As>10mg/L,Sb >10mg/L的工业废水中加入酸调节酸度,控制pH=0.5~4;加入冶炼炉渣和氧化剂,使冶炼炉渣在酸性、氧化气氛下与废水中的As、Sb发生化学反应或物理吸附,控制温度40~100℃,保温反应
1~3h;加入中和剂进行中和,控制pH=6~9;过滤溶液,得到的滤液和滤渣,如果滤液含As<
0.3mg/L,Sb<0.5mg/L,则除杂合格,送污水处理系统进一步净化其它杂质,如果含As>
0.3mg/L,Sb>0.5mg/L,则重复上述步骤直到合格,滤渣送渣场堆存。
本发明采用冶炼炉渣代替沉淀剂除杂,废水中的砷、锑在1~3h内快速有效脱除,脱除率接近100%,效率高成本低,滤渣晶体结构良好,容易澄清过滤且含有铁、钙、镁、铝与五价砷、五价锑组成的砷酸盐、锑酸盐,化学性质稳定。
申请人:红河学院
地址:661100 云南省红河哈尼族彝族自治州蒙自市红河学院理学院内
国籍:CN
代理机构:红河州专利事务所
代理人:朱跃平
更多信息请下载全文后查看。
- 1、下载文档前请自行甄别文档内容的完整性,平台不提供额外的编辑、内容补充、找答案等附加服务。
- 2、"仅部分预览"的文档,不可在线预览部分如存在完整性等问题,可反馈申请退款(可完整预览的文档不适用该条件!)。
- 3、如文档侵犯您的权益,请联系客服反馈,我们会尽快为您处理(人工客服工作时间:9:00-18:30)。
Removal of pollutants from acid mine wastewaterusing metallurgical by-product slagsD. Feng a,∗, J.S.J. van Deventer a, C. Aldrich ba Department of Chemical and Biomolecular Engineering, The University of Melbourne, Melbourne, Vic., 3010, Australiab Department of Chemical Engineering, University of Stellenbosch, Private Bag X1, Matieland, 7602, Stellenbosch, South AfricaReceived in revised form 8 January 2004; accepted 12 January 2004AbstractTheremoval of pollutants from acid mine drainage using metallurgicalby-product slags was studied in laboratory scale. Metallurgicalby-product furnace slags were used as sorbents for metal ions and dispersed air column flotation was employed for the solid/liquid separationof the loaded slags. Batch sorption/pH/kinetic studies were conducted using simulated Cu and Pb bearing wastewater. The calcium glasstype of slags had high surface area and porosity. Promising result was succeeded from the combined process of slag sorption/flotation on thetreatment of an acid mine drainage from a South African gold mine.© 2004 Elsevier B.V. All rights reserved.Keywords: Furnace slag; Sorption; Flotation; Wastewater treatment; Acid mine drainage1. IntroductionVarious methods exist for the removal of toxic metalions from aqueous solution, viz. ion exchange, reverse osmosis,precipitation and adsorption, among others. Adsorptionis by far the most versatile and widely used process.Activated carbon has been the standard adsorbent for thereclamation of municipal and industrial wastewaters. Owingto the high-cost of activated carbon, production of itslow-cost alternatives has been the focus of research in thisarea for years. These sorbents for the heavy metals sorptionranged from natural materials to industrial and agriculturalby-products, such as fly ash, carbonaceous material, metaloxides, zeolites, moss, hydroxides, lignin, clays, biomass,peanut hulls, pyrite fines, goethite and coral sand.Furnace slags as metallurgical by-products are beingused as fillers or in the production of slag cement. It hasbeen reported that granulated furnace slag can be convertedinto an effective adsorbent and used for the removal ofdyes [1,2] and metal ions [3,4]. Alkaline-based slags asnon-conventional sorbents for various heavy metal ionscombine ion-exchange and sorption properties with anacid-neutralising ability. Acid mine water is an unavoidableby-product of the mining and mineral industry, especiallyas far as the oxidation of sulphide minerals is concerned.Acid mine waters typically contain high concentrations ofdissolved heavy metals and sulphate and can have a highturbidity and pH values as low as 2. These conditions mayprohibit discharge of untreated acid mine waters into publicstreams, as they have a detrimental effect on aquatic plantand fish life. Similarly, ground water pollution caused by thedrainage of acid mine water is an equally serious problem.Traditionally, acid mine water is neutralised by treatmentwith lime, resulting in concomitant precipitation of iron,aluminium and other metal hydroxides. However, since theminimum solubilities for the different metals usuallyfoundin the polluted water occur at different pH values and the hydroxide precipitates are amphoteric in nature, maximumremoval efficiency of mixed metals cannot be achieved at asingle precipitation pH level. Conventional sorbents are notacceptable in such a mal-condition as acidic high-turbiditymine drainage. Slags can be used as low-cost adsorbentsand neutralising agents and viable alternatives to the combinationof much more expensive activated carbon or ionexchange resins and lime.Slags exist often in a powdered form and are mainly appliedas dispersions. Downstream of the reaction tank, asuitable solid/liquid separation is generally necessary. Flota-tion offers various advantages for the scope of separation,compared with other processes such as filtration, sedimentationor centrifugation [5] and constitutes a known method ineffluent and water treatment [6,7]. Combination of adsorptionand subsequent flotation has proven to be an effectivemethod for the removal of heavy metals from wastewaterstreams [8–10].The present study involves an examination of the sorptioncapacities of two different slags for Cu and Pb removal fromwastewater streams. Batch sorption/pH/kinetic studies wereconducted in laboratory scale using simulated Cu and Pbbearing wastewater. Flotation of slags following ion sorptionoffers an effective way for solid/liquid separation. Alsoreported is the successful use of the novel technique in thetreatment of an acid drainage from a gold mine.2. Experimental workTwo furnace slags, viz. iron making slag and steel makingslag, were obtained from Saldanha Steel South Africain the form of powder with a mean particle size of 24.5and 24.1 m, respectively. The size distribution was: 100%and100m; 90% and 45 m; 22% and 10 m; and 1.2%and 1m for the iron slag, compared to 100% and 100m;90% and 45m; 23% and 10m; and 1.5% and 1m forthe steel slag. The chemical composition of the slags expressedas oxides in mass percentage is shown in Table 1.The XRD spectra obtained by a DRON X-ray diffractometerindicated that the calcium glass was the major phase inthe two slags. The slags were washed with distilled waterto remove the adhering impurities for three times and driedat 200 ◦C. The dried slags were stored in a desiccator forExperiments.HCl and NaOH (analytical grade from Merck) were usedto adjust the solution pH. The Cu and Pb stock solutionswere prepared by dissolving their corresponding chloride ornitrate salts (CuCl2 ·5H2O and Pb(NO3)2, analytical gradefrom Merck) in distilled water. The ion concentrations instock solutions were about 5000 mg/l. A cationic flocculant (polyamine type) was obtained from Monica, South Africa.Sodium dodecyl sulphate (analytical grade from Sigma) was used as a collector in flotation.Batch sorption experiments were carried out at an ambienttemperature of about 18 ◦C on a roller (60 min−1) using1 l screwed cap plastic bottles. The sorpt ion isotherm studieswere conducted by varying the initial ion concentrations.After a contact time of 24 h, the reaction mixture was filteredthrough a 0.45 m membrane filter (Millipore) and the filtratewas analysed for ion contents. The loading of the slagswas determined by the difference of the ion contents beforeand after adsorption equilibrium. All the kinetic experimentswere carried out at a constant temperature of 18 ◦Cin a 1 l round bottomed transparent plastic reaction vesselimmersed in a water bath. The solution in the vessel wasagitated with a glass impeller at a fixed speed of 600 min−1.All the experiments were duplicated with only the averagevalues being reported.The metal ion contents in solutions were determined byVarian Inductively Coupled Plasma (ICP). The solution pHvalues were detected by CRISON Micro pH 2000. Electrokineticmeasurements for the determination of point ofzero charge (PZC) were carried out on a Laser Zeta meter(Rank Brothers, Cambridge). The surface area of the samplewas measured by BET method on Micropores (ModelASAP 2010, Micromeritics Instrument Corporation, Norcross,GA). The common inorganic anions were determinedby a DIONEX AI450 ion chromatograph with a conductingdetector. For turbidity (NTU units) measurements, a nephelometerfrom HF Instruments was used.The test flotation column was made of glass having adiameter and height of 35 and 300 mm, respectively. Thesparged air was distributed through a sinter glass with poresize 4 (10–15m). The experiments were conducted at anambient temperature of 18 ◦C. The froth was overflowedautomatically. A certain amount of surfactant was added tothe slag slurry and was allowed to condition for 2 min priorto flotation in a beakerstirred by a magnetic stirrer. In thetreatment of the acid mine drainage, the cationic flocculantwas added to the slag slurry and conditioned for 3 min priorto the addition of the collector. The flotation efficiency wasdetermined by the removal of the slags through the changeof the solution turbidity.3. Results and discussion3.1. Sorption equilibriaTwo important physico–chemical aspects for the evaluationof the sorption process as a unit operation are the equilibriaof sorption and the kinetics. Sorption equilibrium isestablished when the concentration of metal in a bulk solutionis in dynamic balance with that of the interface. Fig. 1shows typical sorption isotherms of Cu2+ and Pb2+ on thetwo slags, respectively. The slurry pH was maintained at 5.5 and the slag doses were 2 g/l.Fig. 1.Sorption isotherms of Cu2+ and Pb2+ on the slags. IS representsiron slag and SS steel slag. The maximum standard deviation was within3%.As can be seen from Fig. 1, the iron slag had a muchhigher sorption capacity for heavy metals than the steel slagand Cu2+ had a higher loading on the slags than Pb2+ interms of molar numbers.The sorption isotherms of uptake metals by the slags couldbe expressed as Langmuir isotherms. The metal sorptionconstants for the Cu2+ and Pb2+ on the slags were calculatedfrom Langmuir plots and are given in Table 2. TheLangmuir parameters, Qmax (mg/g) and K (l/mg), are relatedto saturation capacity and the sorption binding constant, respectively.The sorption data for the slags were fitted toLangmuir isotherm equations. The sorption data in respectof the both metals provided an excellent fit to the Langmuirisotherm, giving high correlation coefficients for the slags.As can be seen from Table 2, the saturation capacity ofCu2+ was 88.50 mg/g for the iron slag and 16.21 mg/g for thesteel slag. The saturation capacity of Pb2+ was 95.24 mg/gfor the iron slag and 32.26 mg/g for the steel slag. The sorptionbindingconstants of the iron slag were much higherthan those of the steel slag, in well accordance with thefact that the iron slag had a much higher sorption capacitythan the steel slag. An adsorption capacity of around 40 mgPb2+/g on a granulated blast-furnace slag was reported forthe size fraction of <0.25mm [11]. This slag had a similarcomposition to the iron slag in this study, consisting of34% SiO2, 44% CaO, 6.4% Al2O3, 2.45% MgO and 0.5%Fe2O3. However, the iron slag had a much higher adsorptioncapacity than the reported slag in the literature, likelydue to a much smaller particle size and a higher surface areafor the iron slag.Fig. 2.Dependence of the slag loading with Cu2+ and Pb2+ on equilibriumsolutionpH. IS represents iron slag and SS steel slag.3.2. Effect of pH on sorptionThe original solution concentrations were about 200 mg/l,the slag doses were 2 g/l and the contact time was 24 h. Fig. 2illustrates the pH dependence of the slag loading withCu2+and Pb2+, respectively. Clearly, the metal uptake was quitelow at low pH levels. However, with an increase in solutionpH, a significant enhancement in sorption was recorded forboth slags, with the optimum pH values for the metal sorptionby the iron and steel slags being 3.5–8.5 and 5.2–8.5,respectively. This behaviour can be explained by consideringthe surface charges of the slags which depend, to a largeextent, upon the values of PZC of silica (about 2.3) andalumina (about 8.2) [12]. The composite PZC of the adsorbentslags, as determined by electrophoretic measurements,was found to be 3.2 for the steel slag and 4.8 for the ironslag. For the iron slag, the particle surface had a positivecharge density in the range of pH < 4.8. Under these conditionsthe uptake of metals would be quite low due to electrostaticrepulsion. With increasing pH, i.e. beyond PZC ofthe iron slag (>4.8) the negative charge density on the surfaceof the iron slag increased, thereby resulting in a suddenenhancement in the metal adsorption onto the iron slag.Likewise, the steel slag had a quite low metal loading ataround pH < 3.2 and a higher metal loading at pH > 3.2.In addition, the precipitation of heavy metal ions may contributeto the higher metal loadings on the slags at highersolution pH.3.3. Sorption kineticsThe slag doses were 2 g/l and the slurry pH was maintainedat 5.5. The initial concentrations for Cu2+ and Pb2+solutions were around 200 mg/l, respectively. The sorptionkinetic result is shown in Fig. 3. The sorption equilibriumfor the steel slag could reach within 1 h, while thesorption equilibrium for the iron slag could only reachtill 24 h.Fig. 3.Sorption kinetics of Cu2+ and Pb2+ on the slags. IS representsiron slag and SS steel slag.3.4. Sorption mechanismIn view of the nature of the slags, an exchange interactionof the slag glass with thesolution can be expressed as follows[11]:=SiOCa + 2HOH → =SiOH2 + Ca2+ + 2OH− (1)It can be expected that in acid environment the above reactionwould shift to the left due to the high concentration ofhydrogen ions. The basic slags had neutralising effect followingthe above route.Different amounts of slags were added into 1 l distilledwater and stirred at 600 min−1 for 24 h. Table 3 shows t hechanges of the solution pH and Ca2+ concentrations. Clearly,the calcium ion exchanged with the hydrogen ion and wasreleased from the slags into the solutions, while the solutionpH increased. This confirms that the reaction in Eq. (1)occurred in the contact of the slags with solutions. After 24 hcontact, the calcium concentration was much lower for thesteel slag than for the iron slag at the same doses. It can beinferred that the iron slag had a much higher ion exchangeability than the steel slag, which was in agreement with thesorption equilibria. In the presence of divalent heavy metalions (M2+) in solutions, the above equation can be describedas:=SiOCa +M2+ → =SiOM + Ca2+ (2)The slags had high surface area and porosity as indicatedin Table 1, which provided the basis for metal sorption aswell. As indicated by the microstructures of the slags inFig. 4. Typical topographic image for the original iron slag by SEM. Barlength is 1 m and the magnification 10000×.Fig. 5. Typical topographic image for the original steel slag by SEM. Barlength is 1 m and the magnification 10000×.Figs. 4–6, both slags revealed porous structures and the ironslag had smaller pores than the steel slag. After sorption,some loose flocs appeared at the slag surface as indicated inFig. 6. These flocs could be alumino silicates of copper orcopper hydroxide complexes depositing on the porous slagsurface.Fig. 6. Typical topographic image for the copper loaded iron slag bySEM. Bar length is 1 m and the magnification 10000×.3.5. Slag flotationSome loose and fairly small flocs appeared in the slurryafter 24 h slag adsorption. The solid/liquid separation bysimple sedimentation was not complete in short times (upto 10 h) and required a further stage of filtration. Bothion-exchange and metal precipitation contributed to theremoval of metal ions from wastewater by the slags. Thehydroxide species of the metal ions increased the turbidityof solutions after the adsorption. In addition, the presenceof some fine slag particles could also result in an increase insolution turbidity and this can, however, be avoided by usingcoarse slag particles. In order to remove the suspendedsolids, dispersed air column flotation was employed for thesubsequent separation of loaded slags from solutions. Theinitial Cu and Pb concentrations were 200 mg/l and the slagdoses were 2 g/l. The solution pH was maintained at 5.5.After 24 h contact, the slurry was subjected to flotation atan air flowrate of 200 ml/min. The average bubble size inthe flotation column was around 0.62 mm, based on frothimage analysis. The flotation result is shown in Table 4.As can be seen from Table 4, at a dose of 15 mg/lSDS thesolution turbidity after flotation was already under 1.00 NTU(tap water turbidity) for the slag slurry systems. Both Cu2+and Pb2+ concentrations further decreased after flotationand this effect became more prominent with increasing theSDS doses. This was attributed to the complexation of heavymetal ions with the collector SDS, as confirmed in the blanktests without slags. Adsorptive slag flotation, using smallamounts of slags as carriers, was found to be very effectivefor the treatment of solutions containing heavy metals suchas copper and lead.Settling should also be an effective process leaving a clearsupernatant with the aid of a typical flocculant. Settling issimpler and relatively cheaper. However, flotation is fasterand more effective in recovering fine particles in comparisonwith settling. In the current study, fine slag powderswere used as the sorbents. Therefore, flotation was chosenas the subsequent solid/liquid separation process. In casethat coarse slag particles are used for wastewater treatment,settling process should be favourable. Sorption of pollutantsin packed columns with coarse slag particles can be efficient,despite a lower adsorption area. This will avoid theuse of the subsequent flotation or settling for solid/liquidseparation.3.6. Treatment of an acid mine drainage by flotation ofadsorptive slagsThe acid mine water used in the experiments was sampledfrom the drainage of a South African gold mine. The acidmine water had a very low pH of 2.03 and a very highsulphate concentration, as indicated in Table 5.The acid mine water is neutralised with lime in site, resultingin concomitant precipitation of iron, aluminium andother metal hydroxides. Treatment with lime requires a shortreaction period, owing to its high solubility (0.15%). However,since the minimum solubilities for the different metalsusually found in the polluted water occur at different pHvalues and the hydroxide precipitates are amphoteric in nature,maximum removal efficiency of mixed metals cannotbe achieved at a single precipitation pH level around 7. Theslags combine ion-exchange and sorption properties with anacid-neutralising ability and may offer an alternative for theremoval of metal ions from the acid drainage.Batch sorption experiments were conducted with 1 l of theacid mine drainage and the contact time was 24 h. The effectof slag doses on the removal of ions from the acid minedrainage is shown in Table 5. The slurry pH increased toneutral pH as the slag dose increased up to 30 g/l. Most of theheavy metals such as Cu, Pb, Cr were removed at an iron slagdose of 30 g/l and the residual concentrations could meet thedischarge standards (<0.05 mg/l). The iron slag had a betterperformance in heavy metal ion removal than the steel slag.The precious metal ions such as Au, Ag, Pt, Pd, Rh and Ruwere only removed to a small extent. In general, the preciousmetal ions exist in the forms of anionic complexes withchloride in the acidic environment, such as AuCl4−, PdCl62−and PtCl42−. The anionic complexes could not physicallyadsorb onto the negatively charged slag surface at pH >ZPC and could not exchange with Ca2+ in the slags. Theremoval of some of the precious metal ions may be attributedto the co-flocculation with the Fe(OH)3 flocs formed due tothe increased pH.The other anionic ions except for PO43−were also difficult to remove owing to the same reason forthe anionic precious metal complexes. PO43− was removedas low solubility calcium phosphate precipitates. Ca and Mgcould form some difficult-to-dissolve precipitates with F−,PO43− and SO42− depositing on the slag surface.After 24 h contact with 30 g/l slags, a substantial amount of loose andfairly small flocs appeared in the slag slurry.a Ion concentration in mg/l.Direct flotation by SDS was not very effective for thesolid/liquid separation. A cationic flocculant polyamineat an optimised concentration of 100 mg/l was added intothe slurry and conditioned for 3 min prior to the additionof SDS. The fine flocs and slags formed large andwell-structured aggregates with the addition of polyamine,which were readily removed by the subsequent flotationprocess. The flotation result is shown in Table 6. Obviously,the residual concentrations of all the base metal and preciousmetal ions in the solution were low enough for dischargeinto the public streams, solution pH was neutral and thea Ion concentration in mg/l.solution turbidity was under 1.0 NTU (tap water) after theslag neutralisation and sorption, flocculation and flotationprocess. The cationic flocculant not only flocculatedthe softfine particles to form large aggregates, but also served as acarrier for the removal of anions such as anionic preciousmetal complexes due to the electrostatic attraction. Afterflocculation, the aggregates were more resistant to shearingand suitable for the subsequent flotation. The iron slag had abetter performance than the steel slag for the mine drainagetreatment in terms of the final residual ion concentrations.Finally, it is believed that the concept of adsorptive slagflotation should be stressed by using the non-conventionalsorbents of metallurgical by-product slags and high capacityflotation techniques. The case study only focused on thetreatment of acid mine drainage and it can be expected thatthis process may lead to an alternative future technology inthe field of inorganic and organic contaminants removal4. ConclusionsThe iron and steel making slags were appropriate sorbentsfor heavy metal removal from aqueous solutions. Theslags combined ion-exchange and sorption properties withan acid-neutralising ability. The iron slag had a much highersorption capacity for metals than the steel slag owing to itshigher surface area, higher porosity and higher ion-exchangeeffectivelyseparate the slags, yielding very low solution turbidities.Advantages when compared with settling were observedin terms of process kinetics and quality of the supernatants.The adsorbing slag flotation is particularly attractive for theremoval of heavy metal ions from acid streams and has obtainedpromising result on the treatment of an acid minedrainage from a South African gold mine.ability. Flotation following the slag sorption could effectively References[1] V.K. Gupta, S.K. Srivastava, D. Mohan, Ind. Eng. Chem. Res. 36(1997) 2207.[2] K.R. Ramakrishna, T. Viraraghavan, Waste Manage. 17 (8) (1997)483.[3] S.K. Srivastava, V.K. Gupta, D. Mohan, J. Environ. Eng. Div. Am.Soc. Civ. Eng. 123 (1997) 461.[4] V.K. Gupta, A. Rastogi, M.K. Dwivedi, D. Mohan, Sep. Sci. Technol.32 (1997) 2883.[5] K.A. Matis, A.I. Zouboulis, I.C. Hancock, Sep. Sci. Technol. 29(1994) 1055.[6] Th.F. Zabel, in: P. Mavros, K.A. Matis (Eds.), Innovations in Flotation Technology, 1992, Kluwer Academic, Dordrecht, The Netherlands,p. 431.[7] A.I. Zouboulis, K.A. Matis, K.A. Stalidis, G.A. in: P. Mavros, K.A.Matis (Eds.), Innovations in Flotation Technology, 1992, KluwerAcademic, Dordrecht, The Netherlands, p. 475.[8] C. Aldrich, D. Feng, Minerals Eng. 13 (10–11) (2000) 1129.[9] J. Rubio, F. Tessele, Minerals Eng. 10 (7) (1997) 671.[10] A.I. Zouboulis, K.A. Kydros, K.A. Matis, Water Res. 29 (1995) 1755.[11] K.K. Panday, G. Prasad, V.N. Singh, Water Res. 19 (1985) 869.[12] S.V. Dimitrova, D.R. Mehandgiev, Water Res. 32 (11) (1998) 3289.利用冶金渣副产物去除酸性矿山废水中的污染物摘要在实验室规模,研究利用冶金副产物渣料去除酸性矿物排泄的污染物。