Thermodynamic optimization of the CoZr system
中冷后回热式布雷顿-逆布雷顿联合循环性能优化

中冷后回热式布雷顿-逆布雷顿联合循环性能优化倪栋梁;陈林根;张泽龙;孙丰瑞【摘要】对中冷后回热式布雷顿-逆布雷顿联合循环构型进行有限时间热力学分析和优化,推导出了燃料燃烧放热流率、循环净功率、循环热效率和各个部件由于流动不可逆性产生的压力损失与顶循环压气机进口相对压力损失的函数关系.给出了循环净功率的分析和优化结果,以及在燃油消耗和尺寸约束条件下循环热效率的分析和优化结果.通过数值计算,详细分析了各主要设计参数对循环最优性能的影响.研究发现,存在最佳的中冷压比、压气机1进口相对压力损失、压气机3的压比和总压比,使循环功率获得最优值;在燃油消耗和装置尺寸的约束下,存在最佳的中冷压比、压气机1进口相对压力损失和总压比,使循环效率获得最优值;中冷过程能有效提高循环的功率,回热对循环功率影响很小.【期刊名称】《热力透平》【年(卷),期】2016(045)003【总页数】7页(P189-195)【关键词】中冷回热;布雷顿循环;逆布雷顿循环;联合循环;有限时间热力学;热力学优化【作者】倪栋梁;陈林根;张泽龙;孙丰瑞【作者单位】中国卫星海上测控部,无锡214431;海军工程大学动力工程学院,武汉430033;海军工程大学动力工程学院,武汉430033;海军工程大学动力工程学院,武汉430033;海军工程大学动力工程学院,武汉430033【正文语种】中文【中图分类】TK121998年,Radcenco等[1]建立了单轴简单开式布雷顿循环构型的有限时间热力学(FTT)模型,分别给出了各部件处相对压力损失、燃料燃烧放热率和排放到环境的乏气的放热率、循环净功率和热效率的表达式,分析了压气机入口相对压力损失对循环功率和热效率的影响,发现存在最佳的压气机相对进口压力损失,使循环输出功率达到最佳值。
在运用FTT研究开式布雷顿循环的过程中,优化原理[2-3]主要是通过调节压气机进口相对压力损失(从流体力学角度考虑实际上是调节质量流率),使循环功率、循环效率等性能指标达到最大值。
Advanced Thermodynamics

Advanced ThermodynamicsThermodynamics is a branch of physics that deals with the relationships between heat, work, and energy. It is a fundamental concept in the study of engineering, physics, chemistry, and other related fields. Advanced thermodynamics delves deeper into the complexities of these relationships, exploring moreintricate systems and phenomena. In this response, we will discuss thesignificance of advanced thermodynamics, its applications, and its impact on various industries and technologies. One of the key aspects of advanced thermodynamics is the study of non-ideal systems. While ideal systems provide a simplified model for understanding thermodynamic processes, many real-world systems deviate from ideal behavior. Advanced thermodynamics allows us to analyze and predict the behavior of non-ideal systems, taking into account factors such as interactions between molecules, phase transitions, and chemical reactions. This deeper understanding is crucial for the design and optimization of complex industrial processes, such as chemical manufacturing, power generation, and refrigeration. Furthermore, advanced thermodynamics plays a critical role in the development of sustainable energy technologies. By studying the thermodynamic properties of different energy sources, such as solar, wind, and biomass, researchers can assess their efficiency and potential for large-scale implementation. This knowledge is essential for the transition towards renewable energy sources, as it allows us to maximize energy conversion and minimize environmental impact. Additionally, advanced thermodynamics contributes to the advancement of energy storage systems, which are essential for balancing supply and demand in a renewable energy grid. In the field of materials science, advanced thermodynamics is instrumental in the design and characterization of novel materials with tailored properties. By understanding the thermodynamic driving forces behind phase transformations, alloy formation, and microstructural evolution, researchers can develop materials with specific mechanical, electrical, or magnetic properties. This has numerous applications, from the development of high-performance structural materials for aerospace and automotive industries to the design of advanced functional materials for electronics and energy storage. Moreover, advanced thermodynamics has a profound impact on the field of biophysicsand biotechnology. By applying thermodynamic principles to biological systems, researchers can gain insights into the behavior of complex biomolecular assemblies, such as proteins, nucleic acids, and membranes. This knowledge is invaluable for understanding biological processes at the molecular level, as well as for the design of biopharmaceuticals and biotechnological processes. Additionally, advanced thermodynamics is crucial for the development of cryopreservation techniques, which are essential for the long-term storage of biological materials, such as cells and tissues, for medical and research purposes. In conclusion, advanced thermodynamics is a multifaceted field with far-reaching implications.Its significance extends beyond traditional engineering disciplines, encompassing areas such as sustainable energy, materials science, and biotechnology. By delving into the complexities of non-ideal systems, advanced thermodynamics provides the foundation for the design of efficient industrial processes and the development of innovative technologies. Its impact on various industries and technologies underscores the importance of continued research and education in this field, as we strive towards a more sustainable and technologically advanced future.。
大庆外围低产低渗油田抽油机井电参法推演示功图现场试验

管理·实践/Management&Practice1现状截止2020年底,某外围采油厂油井开井6403口,其中抽油机井5923口,占开井数的92.5%,机采方式以抽油机为主。
目前,对抽油机井进行监测或诊断工况,主要是通过示功图判断井下工况,示功图是连接抽油机井地面系统和井下系统的关键节点,分析方法成熟,标准统一,实用性强,多年来油井示功图始终是判断油井运行状况不可或缺的手段之一。
但是,目前现场测试示功图,还存在以下几方面问题:1)通常示功图测试录取周期为每月1次,录取周期相对较长。
2)当载荷、工况变差时,问题发现不及时性,容易错过最佳清防蜡及问题核实日期,导致问题恶化,严重时会影响产量。
3)工人测试劳动强度大,生产效率低,数据的准确性得不到保证。
4)目前安装变频配电箱的抽油机井逐年增多,其频率变化直接影响了运行冲次,由于现场调参比较方便,导致现场示功图测试分析不能够及时。
5)还存在零点易漂移失真问题,同时需要定期标定[1]。
目前随着油田信息技术的进步,智能数字化管控平台已经成为各油田的发展,不仅可以实时诊断和分析抽油机井工况,而且降低工人劳动强度,既提高了经济效益,又注重社会效益的长远发展。
因此,通过抽油机井电参法示功图的现场应用,利用油井上易测得的电动机功率参数,实现了电参与示功图的实时转换和同步采集,减少了手持示功图仪器现场操作的不安全因素,同时还降低了前线工人测试工作量。
2电参法反演示功图基本原理2.1主要功能模块组成目前,在现有不停机间抽控制配电箱基础上,进行改造添加了几个主要模块,实现电参示功图的现场试验:智能控制处理器、曲柄位置传感器、电动机转速传感器以及三相电参监测传感器[2],主要功能模块安装位置见图1。
图1主要功能模块安装位置智能控制处理器:属于核心元器件,主要用于同时根据同步采集的电动机转速、电参和曲柄位大庆外围低产低渗油田抽油机井电参法推演示功图现场试验卢成国王秋实(大庆油田有限责任公司第八采油厂)摘要:抽油机示功图是判断抽油机井工况的重要手段之一,方法虽然成熟但需要耗费大量人力和物力,并且故障诊断自动化程度低,尤其还不能及时进行反映,因此需要一种新的测试方法迫在眉睫。
Thermal Science and Engineering
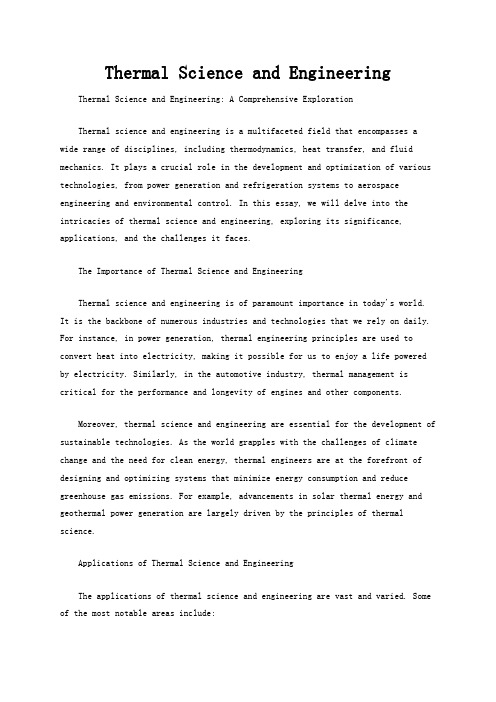
Thermal Science and Engineering Thermal Science and Engineering: A Comprehensive ExplorationThermal science and engineering is a multifaceted field that encompasses a wide range of disciplines, including thermodynamics, heat transfer, and fluid mechanics. It plays a crucial role in the development and optimization of various technologies, from power generation and refrigeration systems to aerospace engineering and environmental control. In this essay, we will delve into the intricacies of thermal science and engineering, exploring its significance, applications, and the challenges it faces.The Importance of Thermal Science and EngineeringThermal science and engineering is of paramount importance in today's world. It is the backbone of numerous industries and technologies that we rely on daily. For instance, in power generation, thermal engineering principles are used to convert heat into electricity, making it possible for us to enjoy a life powered by electricity. Similarly, in the automotive industry, thermal management is critical for the performance and longevity of engines and other components.Moreover, thermal science and engineering are essential for the development of sustainable technologies. As the world grapples with the challenges of climate change and the need for clean energy, thermal engineers are at the forefront of designing and optimizing systems that minimize energy consumption and reduce greenhouse gas emissions. For example, advancements in solar thermal energy and geothermal power generation are largely driven by the principles of thermal science.Applications of Thermal Science and EngineeringThe applications of thermal science and engineering are vast and varied. Some of the most notable areas include:1. Power Generation: Thermal power plants, which rely on the Rankine cycle or other thermodynamic cycles, are a primary source of electricity for many countries. These plants use the principles of heat transfer and thermodynamics to convertheat into mechanical work, which is then converted into electricity.2. Refrigeration and Air Conditioning: The refrigeration and air conditioning industry relies heavily on thermal engineering principles to design and optimize systems that provide cooling and dehumidification. These systems are crucial for maintaining comfortable living and working environments, as well as preserving perishable goods.3. Aerospace Engineering: In the aerospace industry, thermal management is critical for the performance and safety of aircraft and spacecraft. Thermal engineers design systems to manage the heat generated by engines, avionics, and other components, ensuring that temperatures remain within safe operating limits.4. Environmental Control: Thermal engineering plays a vital role in designing and optimizing systems for environmental control, such as heating, ventilation,and air conditioning (HVAC) systems. These systems are essential for maintaining comfortable and healthy indoor environments, as well as reducing energy consumption and emissions.Challenges in Thermal Science and EngineeringDespite the significant contributions of thermal science and engineering to various industries, the field faces several challenges. Some of the most pressing issues include:1. Energy Efficiency: As the world seeks to reduce its dependence on fossil fuels and minimize energy consumption, thermal engineers are tasked withdeveloping more efficient systems. This requires a deep understanding of thermodynamics, heat transfer, and fluid mechanics, as well as innovative design approaches.2. Material Selection: The selection of appropriate materials for thermal systems is crucial for their performance and longevity. Engineers must consider factors such as thermal conductivity, strength, and corrosion resistance when choosing materials for components like heat exchangers, pipes, and insulation.3. Environmental Impact: The environmental impact of thermal systems is a growing concern. Engineers must strive to minimize the emissions and waste generated by these systems, while also considering the lifecycle of materials and components.4. Advanced Simulation and Modeling: As thermal systems become more complex, the need for advanced simulation and modeling tools increases. These tools help engineers predict the performance of systems and identify potential issues before they arise, reducing the time and cost associated with design and testing.The Future of Thermal Science and EngineeringThe future of thermal science and engineering looks promising, with numerous opportunities for growth and innovation. As the world continues to face the challenges of climate change and the need for sustainable energy, thermal engineers will play a pivotal role in developing and optimizing technologies that meet these demands.Advancements in materials science, nanotechnology, and computational methods will likely drive the future of thermal engineering. These advancements will enable the development of more efficient, reliable, and environmentally friendly systems, paving the way for a more sustainable future.In conclusion, thermal science and engineering is a critical field that underpins many of the technologies we rely on today. Its applications are vast, and its importance cannot be overstated. As we look to the future, the challenges faced by thermal engineers will only grow, but so too will the opportunities for innovation and advancement. By embracing these challenges and leveraging thelatest technologies, thermal engineers can help shape a more sustainable and efficient world for generations to come.。
MolecularThermodynamicsPDF:分子热力学的PDF
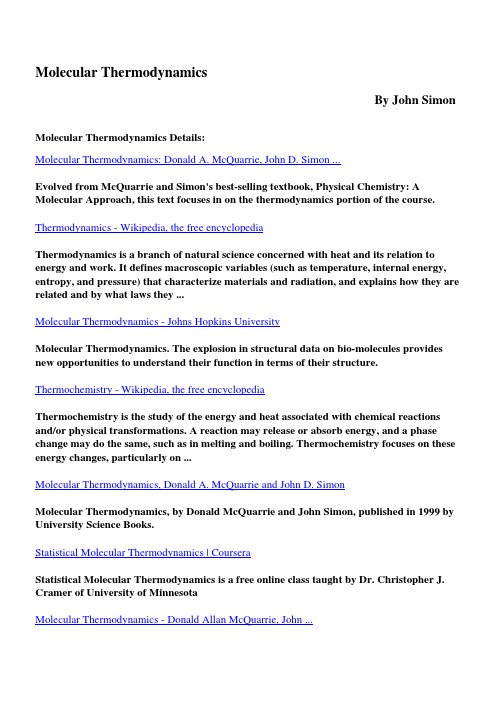
Molecular ThermodynamicsBy John SimonMolecular Thermodynamics Details:Molecular Thermodynamics: Donald A. McQuarrie, John D. Simon ...Evolved from McQuarrie and Simon's best-selling textbook, Physical Chemistry: A Molecular Approach, this text focuses in on the thermodynamics portion of the course. Thermodynamics - Wikipedia, the free encyclopediaThermodynamics is a branch of natural science concerned with heat and its relation to energy and work. It defines macroscopic variables (such as temperature, internal energy, entropy, and pressure) that characterize materials and radiation, and explains how they are related and by what laws they ...Molecular Thermodynamics - Johns Hopkins UniversityMolecular Thermodynamics. The explosion in structural data on bio-molecules provides new opportunities to understand their function in terms of their structure. Thermochemistry - Wikipedia, the free encyclopediaThermochemistry is the study of the energy and heat associated with chemical reactions and/or physical transformations. A reaction may release or absorb energy, and a phase change may do the same, such as in melting and boiling. Thermochemistry focuses on these energy changes, particularly on ...Molecular Thermodynamics, Donald A. McQuarrie and John D. SimonMolecular Thermodynamics, by Donald McQuarrie and John Simon, published in 1999 by University Science Books.Statistical Molecular Thermodynamics | CourseraStatistical Molecular Thermodynamics is a free online class taught by Dr. Christopher J. Cramer of University of MinnesotaMolecular Thermodynamics - Donald Allan McQuarrie, John ...Evolved from McQuarrie and Simon's best-selling Physical Chemistry: A Molecular Approach, this text follows a similar path by first covering the principles of quantum mechanics before engaging those ideas in the subsequent development of thermodynamics. Although many of the chapters are similar ...Introduction to Molecular ThermodynamicsIntroduction to Molecular Thermodynamics. by Robert M. Hanson and Susan Green, St. OlafCollege.enquiries:******************.Publisher'swebsiteforthisbookMolecular Thermodynamics (Physical chemistry monograph series ...Molecular Thermodynamics (Physical chemistry monograph series) [Richard E. Dickerson] on . *FREE* shipping on qualifying offers.Molecular Thermodynamics — ITQB - Universidade Nova de LisboaMolecular Thermodynamics of Liquids and Liquid Solutions, in particular, studies of Ionic Liquids and ionic liquid-containing systems constitute the main activity.Molecular thermodynamics of fluid-phase equilibria - J. M ...Molecular Thermodynamics of Fluid-Phase Equilibria, Third Edition is a systematic, practical guide to interpreting, correlating, and predicting thermodynamic properties used in mixture-related phase-equilibrium calculations. Completely updated, this edition reflects the growing maturity of ...Molecular thermodynamics - definition of Molecular ...ther·mo·chem·is·try (thûr m-k m-str) n. The chemistry of heat and heat-associated chemical phenomena. ther mo·chem i·cal (--k l) adj. ther mo·chem ist n.Prausnitz Group Home Page - University of California, BerkeleyMolecular Thermodynamics John M. Prausnitz. Professor, University of California, Berkeley Faculty Senior Scientist, Lawrence Berkeley National LaboratorymThermoWait for more... ... The website is going through major upgrade ,, wait for the new look soon :)Molecular thermodynamics - HmolpediaAn encyclopedia of topics on the thermodynamics, chemistry, and physics of human existence.Molecular Thermodynamics of Fluid-Phase Equilibria / Edition ...The classic guide to mixtures, completely updated with new models, theories, examples, and data. Efficient separation operations and many other chemical processes depend upon a thorough understanding of the properties of gaseous and liquid mixtures. Molecular Thermodynamics of Fluid-Phase ...Molecular Thermodynamics of Fluid-Phase EquilibriaThermodynamics Home - Chemical Engineering - Thermodynamics: Molecular Thermodynamics of Fluid-Phase Equilibria by Rudiger N. Lichtenthaler, Edmundo Gomes De Azevedo, John M. Prausnitz, Edmundo G. De AzevedoMolecular thermodynamics definition of Molecular ...thermochemistry [¦th?r·m??kem·?·str?] (physical chemistry) The measurement, interpretation, and analysis of heat changes accompanying chemical reactions and changes in state.Molecular Thermodynamics by Donald A. McQuarrie — Reviews ...Molecular Thermodynamics has 7 ratings and 1 review. Jacob said: This books is incredible. It's extremely comprehensive. If you love physical chemistry a...Introduction to Molecular Thermodynamics, by Robert M. Hanson ...Introduction to Molecular Thermodynamics, by Robert M. Hanson and Susan Green , University Science Books, copyright 2008Molecular Thermodynamics of Fluid Phase Equilibria - 3rd Edition2Molecular Thermodynamics of Fluid Phase Equilibria - 3rd Edition2 - Free ebook download as PDF File (.pdf), Text file (.txt) or read book online for free.Molecular thermodynamics (Book, 1999) []Get this from a library! Molecular thermodynamics. [Donald A McQuarrie; John D Simon]Molecular Thermodynamics - Download for freeMolecular Thermodynamics download from FileSnail - 3188189138905X pdf, Thermodynamics Processes an rar, FilePost DepositFiles and RapidShare files. Molecular Thermodynamics of Fluid Phase Equilibria (Prausnitz ...Molecular Thermodynamics of Fluid Phase Equilibria (Prausnitz- 2th Edition ) - Free ebook download as Text file (.txt), PDF File (.pdf) or read book online for free. k Molecular thermodynamics (Open Library)Molecular thermodynamics by Richard Earl Dickerson, 1969,W. A. Benjamin edition, in EnglishMolecular thermodynamics - definition of Molecular ...Molecular thermodynamics. Molecular Time Scale Generalized Langevin Equation Molecular Typing by Reverse Dot-Blotting Molecular Typing Working Group Molecular Unit Cell Approximation molecular velocity Molecular vibration Molecular Vibrational SpectrometerCenter for Molecular & Engineering ThermodynamicsThe University of Delaware’s Thomas H. Epps, III, is one of 30 early-career engineers nationwide invited to attend the 2013 European Union-United States Frontiers of Engineering Symposium to be held Nov. 21-23 in Chantilly, France.ChemE | Thermodynamics and Molecular ComputationsThermodynamics and Molecular Computations. Thermodynamics governs processes as diverse as chemical production, bioreaction, creation of advanced materials, protein separation, and environmental treatment.Molecular Thermodynamics Mcquarrie : ?g Rt K Q. Molecular ...pdf document tagged with molecular thermodynamics mcquarrie : ?G RT K Q. user guide pdf categorized in molecular thermodynamics mcquarrieMolecular Thermodynamics of Fluid-Phase Equilibria by John M ...Molecular Thermodynamics of Fluid-Phase Equilibria has 7 ratings and 1 review. Theclassic guide to mixtures, completely updated with new models, theorie...。
电解水制氢系统效率的统一描述
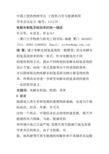
中国工程热物理学会工程热力学与能源利用学术会议论文编号:111178电解水制氢系统效率的统一描述张后程,林国星,陈金灿*(厦门大学物理与机电工程学院,福建厦门 361005)(Tel: 0592-*******, Email: jcchen@) 摘要:建立电解水制氢系统的一般模型,给出电解水制氢系统效率的统一表式。
针对电解池内不同的废热利用方式,提出不同构型的电解水制氢系统的设计方案,由统一表式直接导出不同系统的效率。
并以固体氧化物电解水制氢系统为例计算系统的效率。
所得结论对进一步研究电解水制氢系统性能有一定的指导意义。
关键词:电解水制氢;构型;效率0 前言能源是人类生存和发展的重要物质基础, 也是当今国际政治、经济、军事、外交关注的一个热点。
中国经济社会持续快速发展, 离不开能源的有力保障。
当前,能源危机和环境污染已日益严峻,发展可再生能源已成为各国学者关注的焦点。
由于太阳能、风能、地热能等可再生能源的间歇性和不易储存及运输等特点,迫切需要一种高效清洁的能源载体作为可再生能源和用户之间的桥梁。
氢能以其清洁,高效的特点被公认是未来最具潜力的理想能源载体。
制氢的方法主要有:化石燃料制氢、电解水制氢、热化学制氢及光催化制氢等等[1-5]。
由于水资源较丰富,只要合理使用电能,电解水制氢是倍受青睐的制氢途径。
若利用太阳能、核能、风能及地热能等清洁能源作为电解水制氢的动力源,则电解水制氢是大规模制氢的有效途径[6-9]。
制氢过程实际上是将不易控制的、分散的低品位能量转化成易控制的、可存储的高品位能源。
深入了解电解水分离氢和氧反应机理及伴随的电化学、热力学过程,对于提高电解水制氢系统的性能具有重要理论指导意义。
然而,在讨论电解水制氢系统的性能时,如何定义系统的产氢效率存在着一些理论分歧[10-12]。
如Ni 等[10] 在讨论高温电解水制氢系统性能时忽视了热能与电能的本质区别;Martinez-Frias 等[11] 在讨论天然气水蒸气重整制氢系统性能时虽然注意到热能与电能的区别,但同时又包含了一个与系统性能无关的随机量。
Fe-Co-(Nb,Zr)相平衡的实验测定与热力学计算的开题报告

Fe-Co-(Nb,Zr)相平衡的实验测定与热力学计算的开题报告摘要:本文介绍了一项关于Fe-Co-(Nb,Zr)相平衡的研究。
本研究的目的是通过实验测定和热力学计算来确定这一相图的各个组成部分。
在实验方面,我们使用了高温反应烧结法,并通过X射线衍射和扫描电子显微镜来分析样品的结构和成分。
在热力学方面,我们使用CALPHAD(计算热力学相图)方法来计算这一相图。
我们的研究结果表明,这个系统中Fe-Co相图主要是由一些相互转化的硅化物和碳化物相组成。
而Nb-Zr在这个系统中是以固溶体的形式存在的。
我们的实验测定和热力学计算结果非常吻合,这一研究为我们进一步理解这个系统提供了重要的信息。
关键词:相平衡;Fe-Co;Nb-Zr;实验测定;热力学计算1.研究背景Fe-Co合金是一种重要的材料,具有许多应用领域,包括磁学、电子学和结构材料等。
而Nb-Zr合金在核能和航空航天等领域也有着非常广泛的应用。
因此,在Fe-Co-(Nb,Zr)系统的研究中,理解其相平衡对于这两种合金的应用都将是非常有意义的。
虽然以前已经有关于Fe-Co-Nb系统的相平衡的研究,但是在加入Zr后,这个系统的相平衡仍然存在很多未知的问题,因此有必要进行更深入的研究。
2.研究方法在实验方面,我们使用了高温反应烧结法。
我们根据不同的原料组成和摩尔比混合Fe、Co、Nb、Zr和一些其他的添加剂(如脱氧剂)来制备样品。
我们使用X射线衍射和扫描电子显微镜来分析样品的结构和成分。
在热力学计算方面,我们使用CALPHAD(计算热力学相图)方法来计算这个系统的相图。
我们使用了Thermo-Calc软件和Ternary Assisted Optimization(TAO)方法。
我们使用了参考稳定相的热力学数据以及实验数据来对这个系统的相平衡进行计算。
3.预计研究结果我们预计通过实验测定和热力学计算来确定这个系统的相平衡。
我们预计这个系统中的Fe-Co相图将由一些相互转化的硅化物和碳化物相组成。
二氧化碳燃烧前捕获技术
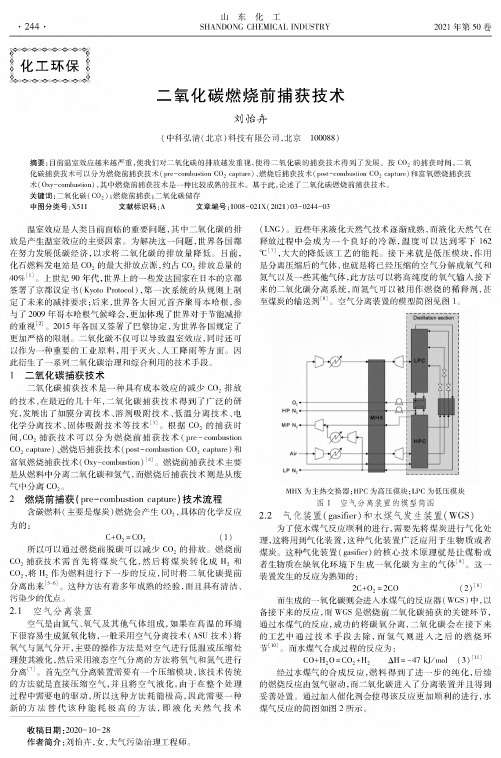
山东化工SHANDONG CHEMICAL INDUSTRY・244・2021年第50卷化工:I二氧化碳燃烧前捕获技术刘怡卉(中科弘清(北京)科技有限公司,北京100088)摘要:目前温室效应越来越严重,使我们对二氧化碳的排放越发重视,使得二氧化碳的捕获技术得到了发展。
按C°2的捕获时间,二氧化碳捕获技术可以分为燃烧前捕获技术(pre-ombustion C°2capture)(燃烧后捕获技术(post-combustion C°2capture)和富氧燃烧捕获技术(Oxa-combustion),燃烧前捕获技术比较成熟的技术。
基,论述了二氧化碳燃烧前捕获技术。
关键词:二氧化碳(C°2);燃烧前捕获;二氧化碳储存中图分类号:X511文献标识码:A文章编号:1008-021X(2021)03-0244-03室效目的题,氧化碳的排放是产室效应的因素。
为题,世界都在努力发展低碳经济,以求将二氧化碳的排放量降低。
目,化石燃料发C°2的大排放点源,C°2排放总量的40%⑴。
上世纪90,世界上的发在日本的京都签署了京都议(Kyota Protocol),!统的从规则上制了未来的求;后,世界各大国元聚哈根,参了2009哈根气候峰会,更体现了世界对于节能的重视2015又签署了巴黎协定,为世界规定了更加严格的限制。
二氧化碳不仅可以导致温室效应,同时还可作为的工业原料,火、人工降。
因此衍生了氧化碳治理和利用的技术手段。
1二氧化碳捕获技术氧化碳捕获技术有效应的减少C°2排放的技术,在的几十年,二氧化碳捕获技术了的研究,发展出了如技术、溶剂吸附技术、技术、电化学技术、固体吸附技术等技术⑶。
根据C°2的捕获时间,C°2捕技术可为燃烧前捕获技术(pw-combustion C°2capture)燃烧后捕获技术(pot-conbuwmn C°2capture)和富氧燃烧捕获技术!Oxy-ombustion)⑷。
- 1、下载文档前请自行甄别文档内容的完整性,平台不提供额外的编辑、内容补充、找答案等附加服务。
- 2、"仅部分预览"的文档,不可在线预览部分如存在完整性等问题,可反馈申请退款(可完整预览的文档不适用该条件!)。
- 3、如文档侵犯您的权益,请联系客服反馈,我们会尽快为您处理(人工客服工作时间:9:00-18:30)。
CALPHAD:Computer Coupling of Phase Diagrams and Thermochemistry34(2010)200–205Contents lists available at ScienceDirectCALPHAD:Computer Coupling of Phase Diagrams andThermochemistryjournal homepage:/locate/calphadThermodynamic optimization of the Co–Zr systemA.Durga,K.C.Hari Kumar∗Department of Metallurgical and Materials Engineering,Indian Institute of Technology Madras,Chennai-600036,Indiaa r t i c l e i n f o Article history:Received17August2009 Received in revised form4February2010Accepted27February2010 Available online23March2010 Keywords:CalphadOptimizationCobaltZirconiumAb initioPhase diagram a b s t r a c tThe constitutional and thermochemical information of the Co–Zr system is modelled using the Calphad method to obtain a reliable thermodynamic description of the system.Appropriate sublattice models are chosen to describe the Gibbs energy of all stable phases of the system.The Gibbs energies of the disordered BCC_A2and the ordered CoZr(BCC_B2)are coupled using the order–disorder model within the framework of sublattice formalism.Results from ab initio calculations are used to aid the optimization of Gibbs energy descriptions of CoZr3,CoZr2,and BCC_B2phases.Calculated phase diagram and thermochemical data are compared with the experimental data.©2010Elsevier Ltd.All rights reserved.1.IntroductionKnowledge of phase diagram and thermochemical properties of the Co–Zr system is very important since these alloying elements are used in several materials of technological importance.For example,Co and Zr are part of many metallic glass-forming systems like Zr–Co–Al[1]and Fe–Co–Zr–Mo–W–B[2].These alloying elements are also present in certain superalloys[3]and high-melting carbides[4].The metastable phase Co5Zr is a hard magnetic phase[5],whose properties are yet to be fully studied. Hence,a complete thermodynamic description of the Co–Zr system would prove invaluable to develop alloys for new applications and also provide better understanding of the implications of these alloying elements in existing alloys.The Co–Zr system has10equilibrium phases:Liquid,FCC_A1 (αCo),Co11Zr2(γ),Co23Zr6(δ),Laves_C15( ),BCC_B2(ζ),CoZr2(η), CoZr3(ω),BCC_A2(βZr)and HCP_A3( Co,αZr).Several versions of the Co–Zr phase diagram have been reported[6–13].Pechin et al.[6]were the first to report a complete phase diagram, followed by Bataleva et al.[8].The former diagram is shown in Fig.1,reproduced from[14].In[8],the CoZr3phase is shown to be forming peritectically from liquid andβZr,whereas this phase is absent in the phase diagram proposed by[6].Chart and Putland[10]were the first to calculate the Co–Zr phase diagram,based on constitutional data from[6–8,15,16]and∗Corresponding author.E-mail address:kchkumar@iitm.ac.in(K.C.Hari Kumar).some estimated entropy and enthalpy values for the intermediate phases,but without using any experimental thermochemical data. Saunders and Miodownik[11]modelled the system using limited thermochemical data and assumed all the intermediate phases to be stoichiometric.Bratberg and Jansson[12]calculated the phase diagram,but not all intermediate phases were included their work.Recently,Liu et al.[13]optimized the system using stoichiometric models for the Gibbs energy of intermediate phases, except for the Laves_C15phase.However,they have not used all the thermochemical data available in the literature for the optimization,although their own experimental phase diagram data were employed.Hence,there is a need to reassess the system.2.Experimental data2.1.Crystallographic dataCrystallographic data for the equilibrium phases in the system are given in Table1.The crystal structure of the Co11Zr2phase has not yet been well established,in spite of a recent attempt[17]to do so.The Co23Zr6phase,earlier[6]reported to be CoZr4phase was later established[18]as having a Mn23Th6-type structure.Two structure types are reported for CoZr3[19]viz.Re3B and Ni3Sn.In this study,Re3B is taken as the prototype of CoZr3since the Ni3Sn structure is reported only by Bataleva et al.[20].Also,Bataleva et al. later reported[8]it as having an Re3B-type structure.0364-5916/$–see front matter©2010Elsevier Ltd.All rights reserved. doi:10.1016/j.calphad.2010.02.006A.Durga,K.C.Hari Kumar/CALPHAD:Computer Coupling of Phase Diagrams and Thermochemistry34(2010)200–205201 Table1Fig.1.Co–Zr phase diagram as reported in[14].2.2.Constitutional dataMost comprehensive works on the phase diagram are reported by two groups[6,8].Using metallography,X-ray diffraction, thermal analysis,and electrical resistivity measurement,Pechin et al.[6]investigated the system and determined the invariant temperatures and solidus temperatures at various compositions. Bataleva et al.[8]reported liquidus temperatures,temperatures of invariant reactions involving liquid and the homogeneity ranges of intermediate phases.They also reported the presence of a CoZr3 phase which was not identified by[6].Studies by Dobromyslov et al.[21]suggests that the CoZr3phase is metastable.According to[22],the homogeneity of Laves_C15phase does not exceed 33.3at.%Zr,which is slightly lower than that suggested by[8]. Recently,Liu et al.[13]used EPMA and DTA to determine the invariant reaction temperatures and phase boundaries.They have established CoZr3as a stable phase,but with a homogeneity range lower than that suggested by[8].According to[13],the homogeneity range of Laves_C15phase is25–34at.%pared to the invariant temperatures involving liquid,congruent melting points of Laves_C15,BCC_B2,and CoZr2are imprecise.Thermal analysis data of[6]indicate that the solidus temperature for an alloy close to the ideal stoichiometry of Laves_C15is around 1812K.This should be close to its congruent melting point. However,in the published phase diagram it appears to be placed around1863K.Bataleva et al.[8]have determined this temperature to be1833K.Hence,in the present study,the melting point of Laves_C15is considered to be between1812–1833K. Similarly,data of[6]indicate that the congruent melting point of BCC_B2should be above1578K,which is the temperature of the invariant reaction Liquid↔Laves_C15+BCC_B2.Melting point of CoZr2reported by[6,8]differs by about33K.2.3.Thermochemical dataExperimental enthalpies of formation of all the intermedi-ate phases,except Co11Zr2and CoZr3,are available in literature[23–28].The enthalpies of the mixing of liquid in the compositionrange0–30at.%Zr at1873K were measured using calorimetry[29].The calculated values of enthalpies of formation of some of theintermediate phases by Colinet et al.[30]are close to experimen-tally determined values.Wang et al.[31]derived an expression forthe mixing enthalpy of the liquid phase by fitting a curve to theexperimental data from Lück et al.[29].The sources of experimental data used in the optimization arelisted in Table2.3.Theoretical predictionsIn addition to the experimental data,some theoretical predic-tions are also used in the data set for optimization.Ohodnickiet al.[32]have calculated energies of the formation of Co23Zr6,Laves_C15,BCC_B2,CoZr2,and CoZr3from first principles.In thepresent work,the enthalpies of the formation of CoZr3and Co11Zr2are calculated using Miedema’s model[33]with improved param-eters for Zr[34],since these values have not been determined ex-perimentally.To check the reliability of the predicted enthalpyvalues by Miedema’s method,we have also calculated the en-thalpies of formation of other compounds and compared themwith experimental data.The predicted values are very close to theexperimental ones.In the present work,we have also used theCASTEP[35]program to perform DFT-based ab initio calculation ofenergy of formation of CoZr3(see Section5).In addition to this,theexcess entropies of liquid are generated using Tanaka’s model[36]from the enthalpies of mixing[29].These are used to constrain theexcess entropy parameters of the liquid phase during optimization.4.Gibbs energy modelsThe Gibbs energy parameters for the pure elements are takenfrom the SGTE compilation[37].Liquid phase and terminalsolid solutions FCC_A1and HCP_A3are modelled as randomsubstitutional solutions using the Redlich–Kister[38]expressionfor excess Gibbs energy.The magnetic contribution to Gibbsenergy[39]is included for terminal solid solutions.The phases Co11Zr2and Co23Zr6are modelled as stoichiometricphases.All non-stoichiometric intermediate phases are modelledusing appropriate sublattice models[40,41],taking into accounttheir crystallography and composition range of existence.TheLaves_C15phase is modelled using the commonly used two-sublattice model(Co%,Zr)2(Co,Zr%)1,with the constraints on Gibbs energies of the end-members as suggested by[42],thatmakes it equivalent to the Wagner–Schottky model[43].The CoZr2phase has a Al2Cu-type structure and hence a two-sublattice model(Co%,Zr)1(Zr)2is used,where Co is the major202 A.Durga,K.C.Hari Kumar/CALPHAD:Computer Coupling of Phase Diagrams and Thermochemistry34(2010)200–205 Table2Table3Fig.2.The calculated Co–Zr phase diagram.constituent in the first sublattice.Note that this type of phase isalso known to have non-stoichiometry in systems such as Al–Cu,Ni–Ta.The prototype structure of CoZr3phase is Re3B.In a single unitcell containing4formula units of CoZr3,4equivalent lattice sitesare occupied by Co,another4by Zr and the remaining8by Zr.Hence,this phase is modelled using a3-sublattice model withmixing in the first two sublattices to account for the homogeneityrange.The model is given as:(Co%,Zr)1(Co,Zr%)1(Zr)2,where Co is the major constituent in the first sublattice and Zr in the secondsublattice.Choice of such a model is further justified since in othersystems(B–Re and Fe–Zr),where such a structure type exists thereis well-established non-stoichiometry for the phase.The terminal solid solution BCC_A2and the intermedi-ate phase BCC_B2are modelled using the two-sublattice or-der–disorder(A2–B2)model[44].The A2phase is modelled as (Co,Va,Zr%)1(Va)3and the B2phase as(Co%,Va,Zr)0.5(Co,Va, Zr%)0.5(Va)3.5.Ab-initio calculationsFor the CoZr3phase,whose structure is well established,but no experimental enthalpy data is available,we haveattemptedparison of the calculated phase diagram with experimental data. to calculate the energy of formation using the ab initio program CASTEP[35].Perdew–Burke–Ernzerhof(PBE)GGA[45]and ultrasoft pseudopotentials are used in this work for all the ab initio calculations.An optimum cut-off energy and an optimum number of k-points are determined by performing single-point energy calculations,varying the two parameters one at a time.The two parameters are chosen such that the calculated energy has an accuracy of10−5eV/atom.With these two parameters fixed, the geometry optimization is carried out to obtain the energy and configuration of the compound at0K.Parameters for convergence control of geometry optimization are set at5×10−6eV/atom for total energy,0.01eV/Åfor maximum force,0.02GPa for stress, and5×10−4Åfor displacement.The geometry optimization is performed,starting with the primitive unit cell of the crystal structure.To calculate the energy of formation of the CoZr3phase, the energy of Co and Zr in their ground-state HCP structure should also be known.Hence,geometry optimization is done for Co and Zr also.Considering the CoZr2sublattice model,there are2end-members namely Co:Zr and Zr:Zr.The first one corresponds to the required stable structure of the compound and the second one is a hypothetical compound.Hence,the parameter◦G CoZr2Zr:Zr corresponding to Zr:Zr is calculated.Similarly,total energies of theA.Durga,K.C.Hari Kumar/CALPHAD:Computer Coupling of Phase Diagrams and Thermochemistry34(2010)200–205203 Table4Table4(continued)simple cubic(Pearson symbol:cP1)form of Co and Zr were also calculated.These values were used in fixing the values of Gibbs energy parameters◦G BCC_B2Co:Va:Va,◦G BCC_B2Va:Co:Va,◦G BCC_B2Zr:Va:Va,and◦G BCC_B2Va:Zr:Va.The results obtained from ab initio calculations are indicated in Eqs.(1)–(4)and shown in Table3.E CoZr3=(E(CoZr3)−2·E(Co)/2−6·E(Zr)/2)8=−0.23512eV/atom=−22658J/mol≈∆f H CoZr3(1)◦G CoZr2Zr:Zr=3×GHSERZr+3×29016J/mol(2)◦G BCC_B2Co:Va:Va=◦G BCC_B2Va:Co:Va=(GHSERCo+107100)∗0.5(3)◦G BCC_B2Zr:Va:Va=◦G BCC_B2Va:Zr:Va=(GHSERZr+88400)∗0.5.(4) 6.OptimizationThe optimization was carried out using the PARROT mod-ule[46]of Thermo-Calc[47].A critical review of the experimental data was made prior to the preparation of the input file for opti-mization.While weighting the data,due consideration was given to experimental technique,purity of the starting materials,etc. The enthalpy of formation of CoZr3obtained from ab initio cal-culations(Eq.(1))was used as an input in the optimization withan error of±10%.The term◦G CoZr2Zr:Zrwas fixed according to Eq.(2).In the description of BCC_A2,the parameter◦G BCC_A2Va:Vawas chosen to have a large positive value of+30·T J/mol,as per the exist-ing practice[48].The parameter0L BCC_A2∗,Va:Vawas adjusted such that the melting points of the pure elements in the BCC_A2state are same as the values obtained using the SGTE data.The parameters ◦G BCC_B2Co:Va:Va,◦G BCC_B2Va:Co:Va,◦G BCC_B2Zr:Va:Va,and◦G BCC_B2Va:Zr:Vawere constrained ac-cording to Eqs.(3)and(4).During optimization,the liquid param-eters were optimized first,followed by the terminal solid solutions and subsequently all the intermediate phases.Final optimization was done including all phases and data simultaneously,until the sum of squares of error did not show any appreciable change.7.Results and discussionOptimized Gibbs energy functions are listed in Table4.The phase diagram calculated using the description is shown in Fig.2. The comparison of calculated phase diagram with experimental data from literature is shown in Fig.3.Considering uncertainties in the experimental data,there is reasonable agreement between the experimental and calculated phase diagrams.Enthalpies of formation of intermediate phases of the system are listed in Table5.There is good agreement between the experimental, Miedema and ab initio values of enthalpies of formation of intermediate phases with the calculated values.In Fig.4the enthalpy of mixing of liquid is plotted as a function of composition. The invariant reaction temperatures are listed in Table6.The strength of the present work is substantially more thermochemical data used for the optimization than by[13].Our optimization is somewhat more biased towards thermochemical204 A.Durga,K.C.Hari Kumar /CALPHAD:Computer Coupling of Phase Diagrams and Thermochemistry 34(2010)200–205Table 5Comparison between experimental/theoretical and optimized values of enthalpies of formation of intermediate phases (J /mol of atoms).Mole Fraction, Zr[29]E n t ha l p y o f M i x i n g , k J /m o l-40-30-20-100.20.40.60.8CoZrFig.4.Calculated and experimental values of enthalpy of mixing of liquid.data,whereas [13]relied more on the constitutional information.This is a system where we believe that thermochemical data are relatively more reliable than the phase diagram data.During the optimization we noticed that any attempt to force fit the invariant and congruent melting temperatures lead to poor fit to the enthalpy of formation of intermediate phases.Note that purity of starting materials in most phase diagram investigation were not very good ([6]:Co 99.6%,Zr 99.94%,[8]:Co:99.9%,Zr 99.9%,[13]:Co 99.8%,Zr 99.7%).8.ConclusionsConstitutional and thermochemical data concerning the Co–Zr system available in the literature were assessed carefully and optimized using the Calphad method with the aid of the software package Thermo-Calc.Results of ab initio calculations and Miedema’s method were combined with the experimental data and used in the optimization.A reasonable agreement between the experimental and calculated phase diagram is obtained.AnTable 6optimal set of parameters leading to a reliable thermodynamic description of the Co–Zr system is presented.AcknowledgementComputational support (High Performance Cluster Computer)provided by P.G.Senapathy Centre for Computational Resources,Indian Institute of Technology Madras,Chennai,India,is gratefully acknowledged.Appendix.Supplementary dataSupplementary data associated with this article can be found,in the online version,at doi:10.1016/j.calphad.2010.02.006.References[1]Y.Wang,X.Zhang,J.Qiang,Q.Wang,D.Wang,D.Li,et al.,Compositionoptimization of the Al–Co–Zr bulk metallic glasses,Scr.Mater.12(2004)1275–1278.[2]D.Y.Liu,W.S.Sun,A.M.Wang,H.F.Zhang,Z.Q.Hu,Preparation,thermalstability and magnetic properties of Fe–Co–Zr–Mo–W–B bulk metallic glass,J.Alloys Compd.370(2004)249–253.[3]D.J.Costin,J.B.Ford,Cobalt-base superalloy,United States Patent 3881918,May 1975.A.Durga,K.C.Hari Kumar/CALPHAD:Computer Coupling of Phase Diagrams and Thermochemistry34(2010)200–205205[4]R.G.Bourdeau,Cubic boron nitride and metal carbide tool bit,United StatesPatent4342595,August1982.[5]T.Saito,Microstructures and magnetic properties of Co–Zr system alloys,Mater.Trans.44(9)(2003)1713–1716.[6]W.H.Pechin,D.E.Williams,rsen,The zirconium–cobalt alloy system,Trans.ASM57(1964)464–473.[7]R.P.Elliot,Constitution of Binary Alloys,First Supplement,McGraw-Hill BookCompany,New York,USA,1965.[8]C.K.Bataleva,V.V.Kuprina,V.V.Burnasheva,V.Y.Markiv,G.N.Ronami,S.M.Kuznetsova,Phase diagram of the cobalt–zirconium system,Vest.Mosk.Univ.,Khim.11(5)(1970)557–561.[9]C.B.Alcock,K.T.Jacob,S.Zador,Zirconium:physico-chemical properties of itscompounds and alloys,International Atomic Energy Agency,Vienna,1976.[10]T.Chart,F.Putland,A thermodynamically calculated phase diagram for theCo–Cr–Zr system,Calphad3(1)(1979)9–18.[11]N.Saunders,A.P.Miodownik,Thermodynamic aspects of amorphous phasetransformation,J.Mater.Res.1(1)(1986)38–46.[12]J.Bratberg,B.Jansson,Thermodynamic evaluation of the C–Co–W–Hf–Zrsystem for cemented carbides applications,J.Phase Equilib.Diffus.27(3)(2006)213–219.[13]X.J.Liu,H.H.Zhang,C.P.Wang,K.Ishida,Experimental determination andthermodynamic assessment of the phase diagram in the Co–Zr system,J.AlloysCompd.482(1–2)(2009)99–105.[14]T.B.Massalski(Ed.),Binary Alloy Phase Diagrams,ASM International,MaterialsPark,OH,USA,1990.[15]F.A.Shunk,Constitution of Binary Alloys,Second Supplement,McGraw-HillBook Company,New York,USA,1969.[16]M.Hansen,K.Anderko,Constitution of Binary Alloys,McGraw-Hill BookCompany,New York,USA,1958.[17]G.V.Ivanova,N.N.Shchegoleva,A.M.Gabay,Crystal structure of Zr2Co11hardmagnetic compound,J.Alloys Compd.432(2007)135–141.[18]Y.B.Kuz’ma,kh,Y.V.Voroshkolov,B.I.Stadnyk,Phase equilibria in theternary systems Zr–Co–C and Nb–Fe–C,Neorg.Mater.1(1965)1112.[19]P.Villars,L.D.Calvart,Pearson’s Handbook of Crystallographic Data forIntermetallic Phases,vol.2,ASM International,Materials Park,OH,USA,1991.[20]V.V.Kuprina,S.K.Bataleva,I.G.Sokolova,Investigation of alloys of thezirconium–cobalt alloy system,Neorg.Mater.1(9)(1965)1416–1419.[21]A.V.Dobromysylov,N.V.Kazantseva,Formation of metastableω-phase inZr–Fe,Zr–Co,Zr–Ni,and Zr–Cu alloys,Scr.Mater.37(5)(1997)615–620.[22]J.H.Zhu,C.T.Liu,Defect structures in ZrCo2laves phase,Acta Mater.48(9)(2000)2339–2347.[23]J.C.Gachon,M.Dirand,J.Hertz,The enthalpies of formation of the intermediatephases Co0.38Zr0.67,Co0.5Zr0.5,Co0.67Zr0.33and Co0.8Zr0.2by direct reactioncalorimetry at high temperature,J.Less-Common Met.85(1)(1982)1–9.[24]J.C.Gachon,J.Hertz,Enthalpies of formation of binary phases in the systemsFeTi,FeZr,CoTi,CoZr,NiTi,and NiZr,Calphad7(1)(1983)1–12.[25]P.V.Gomozov,V.Y.Zasypalov,B.M.Mogutnov,Enthalpies of formation ofintermetallic compunds with CsCl structure(CoTi,CoZr,CoAl,NiTi),Russ.J.Phys.Chem.60(8)(1986)1122–1124.[26]R.Klein,P.A.G.O’Hare,I.Jacob,Standard molar enthalpies of formation ofalloys in the pseudobinary system Zr(Al x Co1−x)2at the temperature298.15K,J.Alloys Compd.261(1997)204–208.[27]Q.Guo,O.J.Kleppa,Standard enthalpies of formation of some alloys formedbetween group IV elements and group VIII elements,determined by high-temperature direct synthesis calorimetry:II.Alloys of(Ti,Zr,Hf)with(Co,Ni), J.Alloys Compd.269(1–2)(1998)181–186.[28]O.Y.Sidorov,O.Y.Esin,P.V.Gel’d,Enthalpies of formation of zirconium alloyswith iron,cobalt,nickel,and copper,Rasplavy3(3)(1989)28–33.[29]R.Lück,H.Wang,B.Predel,Kalorimetrische bestimmung der mischungsen-thalpie flüssiger cobalt–zrirconim–legierungen,Z.Anorg.Allg.Chem.619(3) (1993)447–452.[30]C.Colinet,A.Pasturel,P.Hicter,Trends in cohesive energy of transition metalalloys,Calphad9(1)(1985)71–99.[31]H.Wang,R.Lück, B.Predel,Thermodynamic calculations in quaternarytransition metal systems,J.Alloys Compd.191(1)(1993)43–49.[32]P.R.Ohodnicki Jr.,N.C.Cates,ughlin,M.E.McHenry,M.Widom,Ab initiotheoretical study of magnetization and phase stability of the(Fe,Co,Ni)23B6 and(Fe,Co,Ni)23Zr6structures of Cr23C6and Mn23Th6prototypes,Phys.Rev.B78(14)(2008)144414.[33]F.R.De Boer,R.Boom,W.C.M.Mattens,A.Miedema,A.Niessen,Cohesion inMetals,North-Holland,Amsterdam,1988.[34]Xing-Qiu Chen,R.Podlouckya,Miedema’s model revisited:the parameter forTi,Zr,and Hf,Calphad30(3)(2006)266–269.[35]S.J.Clark,M.D.Segall,C.J.Pickard,P.J.Hasnip,M.J.Probert,K.Refson,et al.,Firstprinciples methods using castep,Z.Krist.220(5–6)(2005)567–570.[36]T.Tanaka,N.A.Gokcen,Z.Morita,T.Iida,Thermodynamic relationshipbetween enthalpy and excess entropy in liquid binary alloys,Z.Metallkd.84(3)(1993)192–199.[37]A.T.Dinsdale,SGTE data for pure elements,Calphad15(4)(1991)317–425.[38]O.Redlich,A.T.Kister,Algebraic representation of thermodynamic propertiesand the classification of solutions,Ind.Eng.Chem.40(2)(1948)345–348. [39]M.Hillert,M.Jarl,A model for alloying effects in ferromagnetic metals,Calphad2(3)(1978)227–238.[40]M.Hillert,L.I.Staffanson,The regular solution model for stoichiometric phasesand ionic melts,Acta Chem.Scand.24(1970)3618–3626.[41]B.Sundman,J.Ågren,A regular solution model for phases with severalcomponents and sublattices suitable for computer applications,J.Phys.Chem.Solids42(4)(1981)297–301.[42]I.Ansara,N.Dupin,H.L.Lukas,B.Sundman,Applications of thermodynamicsin the synthesis and processing of materials,in:P.Nash(Ed.),Proc.Materials Week’94,TMS,Warrendale,PA,1994,pp.273–283.(in Rosemont).[43]C.Wagner,Thermodynamics of Alloys,Addison Wesley Publishing CompanyInc.,Massachussetts,USA,1952.[44]I.Ansara,P.Willemin,B.Sundman,Thermodynamic modeling of orderedphases in the Ni–Al system,Acta Metall.36(1988)977–982.[45]J.P.Perdew,K.Burke,M.Ernzerhof,Generalized gradient approximation madesimple,Phys.Rev.Lett.77(1996)3865.[46]B.Jansson,Ph.D.Thesis,Division of Physical Metallurgy,Royal Institute ofTechnology,Stockholm,Sweden,1984.[47]B.Sundman,B.Jansson,J.O.Andersson,The thermo-calc databank system,Calphad9(2)(1985)153–190.[48]H.L.Lukas,S.G.Fries, B.Sundman,Computational Thermodynamics—TheCalphad Method,Cambridge University Press,New York,2007.。