毕设英文翻译英文版
毕业设计外文翻译_英文版
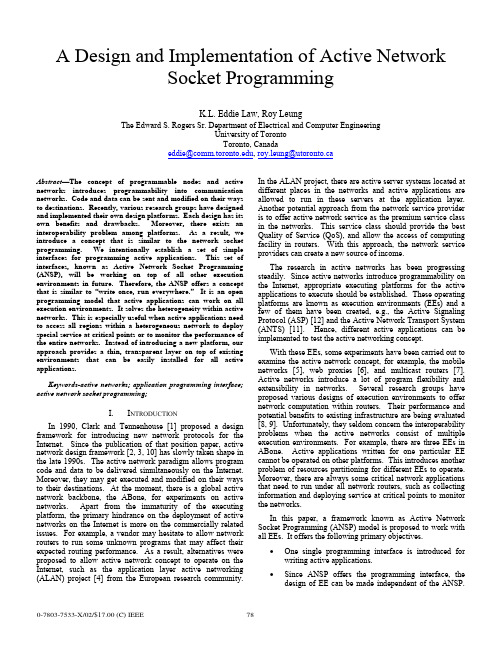
A Design and Implementation of Active NetworkSocket ProgrammingK.L. Eddie Law, Roy LeungThe Edward S. Rogers Sr. Department of Electrical and Computer EngineeringUniversity of TorontoToronto, Canadaeddie@, roy.leung@utoronto.caAbstract—The concept of programmable nodes and active networks introduces programmability into communication networks. Code and data can be sent and modified on their ways to destinations. Recently, various research groups have designed and implemented their own design platforms. Each design has its own benefits and drawbacks. Moreover, there exists an interoperability problem among platforms. As a result, we introduce a concept that is similar to the network socket programming. We intentionally establish a set of simple interfaces for programming active applications. This set of interfaces, known as Active Network Socket Programming (ANSP), will be working on top of all other execution environments in future. Therefore, the ANSP offers a concept that is similar to “write once, run everywhere.” It is an open programming model that active applications can work on all execution environments. It solves the heterogeneity within active networks. This is especially useful when active applications need to access all regions within a heterogeneous network to deploy special service at critical points or to monitor the performance of the entire networks. Instead of introducing a new platform, our approach provides a thin, transparent layer on top of existing environments that can be easily installed for all active applications.Keywords-active networks; application programming interface; active network socket programming;I. I NTRODUCTIONIn 1990, Clark and Tennenhouse [1] proposed a design framework for introducing new network protocols for the Internet. Since the publication of that position paper, active network design framework [2, 3, 10] has slowly taken shape in the late 1990s. The active network paradigm allows program code and data to be delivered simultaneously on the Internet. Moreover, they may get executed and modified on their ways to their destinations. At the moment, there is a global active network backbone, the ABone, for experiments on active networks. Apart from the immaturity of the executing platform, the primary hindrance on the deployment of active networks on the Internet is more on the commercially related issues. For example, a vendor may hesitate to allow network routers to run some unknown programs that may affect their expected routing performance. As a result, alternatives were proposed to allow active network concept to operate on the Internet, such as the application layer active networking (ALAN) project [4] from the European research community. In the ALAN project, there are active server systems located at different places in the networks and active applications are allowed to run in these servers at the application layer. Another potential approach from the network service provider is to offer active network service as the premium service class in the networks. This service class should provide the best Quality of Service (QoS), and allow the access of computing facility in routers. With this approach, the network service providers can create a new source of income.The research in active networks has been progressing steadily. Since active networks introduce programmability on the Internet, appropriate executing platforms for the active applications to execute should be established. These operating platforms are known as execution environments (EEs) and a few of them have been created, e.g., the Active Signaling Protocol (ASP) [12] and the Active Network Transport System (ANTS) [11]. Hence, different active applications can be implemented to test the active networking concept.With these EEs, some experiments have been carried out to examine the active network concept, for example, the mobile networks [5], web proxies [6], and multicast routers [7]. Active networks introduce a lot of program flexibility and extensibility in networks. Several research groups have proposed various designs of execution environments to offer network computation within routers. Their performance and potential benefits to existing infrastructure are being evaluated [8, 9]. Unfortunately, they seldom concern the interoperability problems when the active networks consist of multiple execution environments. For example, there are three EEs in ABone. Active applications written for one particular EE cannot be operated on other platforms. This introduces another problem of resources partitioning for different EEs to operate. Moreover, there are always some critical network applications that need to run under all network routers, such as collecting information and deploying service at critical points to monitor the networks.In this paper, a framework known as Active Network Socket Programming (ANSP) model is proposed to work with all EEs. It offers the following primary objectives.• One single programming interface is introduced for writing active applications.• Since ANSP offers the programming interface, the design of EE can be made independent of the ANSP.This enables a transparency in developing andenhancing future execution environments.• ANSP addresses the interoperability issues among different execution environments.• Through the design of ANSP, the pros and cons of different EEs will be gained. This may help design abetter EE with improved performance in future.The primary objective of the ANSP is to enable all active applications that are written in ANSP can operate in the ABone testbed . While the proposed ANSP framework is essential in unifying the network environments, we believe that the availability of different environments is beneficial in the development of a better execution environment in future. ANSP is not intended to replace all existing environments, but to enable the studies of new network services which are orthogonal to the designs of execution environments. Therefore, ANSP is designed to be a thin and transparent layer on top of all execution environments. Currently, its deployment relies on automatic code loading with the underlying environments. As a result, the deployment of ANSP at a router is optional and does not require any change to the execution environments.II. D ESIGN I SSUES ON ANSPThe ANSP unifies existing programming interfaces among all EEs. Conceptually, the design of ANSP is similar to the middleware design that offers proper translation mechanisms to different EEs. The provisioning of a unified interface is only one part of the whole ANSP platform. There are many other issues that need to be considered. Apart from translating a set of programming interfaces to other executable calls in different EEs, there are other design issues that should be covered, e.g., • a unified thread library handles thread operations regardless of the thread libraries used in the EEs;• a global soft-store allows information sharing among capsules that may execute over different environmentsat a given router;• a unified addressing scheme used across different environments; more importantly, a routing informationexchange mechanism should be designed across EEs toobtain a global view of the unified networks;• a programming model that should be independent to any programming languages in active networks;• and finally, a translation mechanism to hide the heterogeneity of capsule header structures.A. Heterogeneity in programming modelEach execution environment provides various abstractions for its services and resources in the form of program calls. The model consists of a set of well-defined components, each of them has its own programming interfaces. For the abstractions, capsule-based programming model [10] is the most popular design in active networks. It is used in ANTS [11] and ASP [12], and they are being supported in ABone. Although they are developed based on the same capsule model, their respective components and interfaces are different. Therefore, programs written in one EE cannot run in anther EE. The conceptual views of the programming models in ANTS and ASP are shown in Figure 1.There are three distinct components in ANTS: application, capsule, and execution environment. There exist user interfaces for the active applications at only the source and destination routers. Then the users can specify their customized actions to the networks. According to the program function, the applications send one or more capsules to carry out the operations. Both applications and capsules operate on top of an execution environment that exports an interface to its internal programming resources. Capsule executes its program at each router it has visited. When it arrives at its destination, the application at destination may either reply it with another capsule or presents this arrival event to the user. One drawback with ANTS is that it only allows “bootstrap” application.Figure 1. Programming Models in ASP and ANTS.In contrast, ASP does not limit its users to run “bootstrap” applications. Its program interfaces are different from ANTS, but there are also has three components in ASP: application client, environment, and AAContext. The application client can run on active or non-active host. It can start an active application by simply sending a request message to the EE. The client presents information to users and allows its users to trigger actions at a nearby active router. AAContext is the core of the network service and its specification is divided into two parts. One part specifies its actions at its source and destination routers. Its role is similar to that of the application in ANTS, except that it does not provide a direct interface with the user. The other part defines its actions when it runs inside the active networks and it is similar to the functional behaviors of a capsule in ANTS.In order to deal with the heterogeneity of these two models, ANSP needs to introduce a new set of programming interfaces and map its interfaces and execution model to those within the routers’ EEs.B. Unified Thread LibraryEach execution environment must ensure the isolation of instance executions, so they do not affect each other or accessThe authors appreciate the Nortel Institute for Telecommunications (NIT) at the University of Toronto to allow them to access the computing facilitiesothers’ information. There are various ways to enforce the access control. One simple way is to have one virtual machine for one instance of active applications. This relies on the security design in the virtual machines to isolate services. ANTS is one example that is using this method. Nevertheless, the use of multiple virtual machines requires relatively large amount of resources and may be inefficient in some cases. Therefore, certain environments, such as ASP, allow network services to run within a virtual machine but restrict the use of their services to a limited set of libraries in their packages. For instance, ASP provides its thread library to enforce access control. Because of the differences in these types of thread mechanism, ANSP devises a new thread library to allow uniform accesses to different thread mechanisms.C. Soft-StoreSoft-store allows capsule to insert and retrieve information at a router, thus allowing more than one capsules to exchange information within a network. However, problem arises when a network service can execute under different environments within a router. The problem occurs especially when a network service inserts its soft-store information in one environment and retrieves its data at a later time in another environment at the same router. Due to the fact that execution environments are not allowed to exchange information, the network service cannot retrieve its previous data. Therefore, our ANSP framework needs to take into account of this problem and provides soft-store mechanism that allows universal access of its data at each router.D. Global View of a Unified NetworkWhen an active application is written with ANSP, it can execute on different environment seamlessly. The previously smaller and partitioned networks based on different EEs can now be merging into one large active network. It is then necessary to advise the network topology across the networks. However, different execution environments have different addressing schemes and proprietary routing protocols. In order to merge these partitions together, ANSP must provide a new unified addressing scheme. This new scheme should be interpretable by any environments through appropriate translations with the ANSP. Upon defining the new addressing scheme, a new routing protocol should be designed to operate among environments to exchange topology information. This allows each environment in a network to have a complete view of its network topology.E. Language-Independent ModelExecution environment can be programmed in any programming language. One of the most commonly used languages is Java [13] due to its dynamic code loading capability. In fact, both ANTS and ASP are developed in Java. Nevertheless, the active network architecture shown in Figure 2 does not restrict the use of additional environments that are developed in other languages. For instance, the active network daemon, anted, in Abone provides a workspace to execute multiple execution environments within a router. PLAN, for example, is implemented in Ocaml that will be deployable on ABone in future. Although the current active network is designed to deploy multiple environments that can be in any programming languages, there lacks the tool to allow active applications to run seamlessly upon these environments. Hence, one of the issues that ANSP needs to address is to design a programming model that can work with different programming languages. Although our current prototype only considers ANTS and ASP in its design, PLAN will be the next target to address the programming language issue and to improve the design of ANSP.Figure 2. ANSP Framework Model.F. Heterogeneity of Capsule Header StructureThe structures of the capsule headers are different in different EEs. They carries capsule-related information, for example, the capsule types, sources and destinations. This information is important when certain decision needs to be made within its target environment. A unified model should allow its program code to be executed on different environments. However, the capsule header prevents different environments to interpret its information successfully. Therefore, ANSP should carry out appropriate translation to the header information before the target environment receives this capsule.III. ANSP P ROGRAMMING M ODELWe have outlined the design issues encountered with the ANSP. In the following, the design of the programming model in ANSP will be discussed. This proposed framework provides a set of unified programming interfaces that allows active applications to work on all execution environments. The framework is shown in Figure 2. It is composed of two layers integrated within the active network architecture. These two layers can operate independently without the other layer. The upper layer provides a unified programming model to active applications. The lower layer provides appropriate translation procedure to the ANSP applications when it is processed by different environments. This service is necessary because each environment has its own header definition.The ANSP framework provides a set of programming calls which are abstractions of ANSP services and resources. A capsule-based model is used for ANSP, and it is currently extended to map to other capsule-based models used in ANTSand ASP. The mapping possibility to other models remains as our future works. Hence, the mapping technique in ANSP allows any ANSP applications to access the same programming resources in different environments through a single set of interfaces. The mapping has to be done in a consistent and transparent manner. Therefore, the ANSP appears as an execution environment that provides a complete set of functionalities to active applications. While in fact, it is an overlay structure that makes use of the services provided from the underlying environments. In the following, the high-level functional descriptions of the ANSP model are described. Then, the implementations will be discussed. The ANSP programming model is based upon the interactions between four components: application client , application stub , capsule , and active service base.Figure 3. Information Flow with the ANSP.•Application Client : In a typical scenario, an active application requires some means to present information to its users, e.g., the state of the networks. A graphical user interface (GUI) is designed to operate with the application client if the ANSP runs on a non-active host.•Application Stub : When an application starts, it activates the application client to create a new instance of application stub at its near-by active node. There are two responsibilities for the application stub. One of them is to receive users’ instructions from the application client. Another one is to receive incoming capsules from networks and to perform appropriate actions. Typically, there are two types of actions, thatare, to reply or relay in capsules through the networks, or to notify the users regarding the incoming capsule. •Capsule : An active application may contain several capsule types. Each of them carries program code (also referred to as forwarding routine). Since the application defines a protocol to specify the interactions among capsules as well as the application stubs. Every capsule executes its forwarding routine at each router it visits along the path between the source and destination.•Active Service Base : An active service base is designed to export routers’ environments’ services and execute program calls from application stubs and capsules from different EEs. The base is loaded automatically at each router whenever a capsule arrives.The interactions among components within ANSP are shown in Figure 3. The designs of some key components in the ANSP will be discussed in the following subsections. A. Capsule (ANSPCapsule)ANSPXdr decode () ANSPXdr encode () int length ()Boolean execute ()New types of capsule are created by extending the abstract class ANSPCapsule . New extensions are required to define their own forwarding routines as well as their serialization procedures. These methods are indicated below:The execution of a capsule in ANSP is listed below. It is similar to the process in ANTS.1. A capsule is in serial binary representation before it issent to the network. When an active router receives a byte sequence, it invokes decode() to convert the sequence into a capsule. 2. The router invokes the forwarding routine of thecapsule, execute(). 3. When the capsule has finished its job and forwardsitself to its next hop by calling send(), this call implicitly invokes encode() to convert the capsule into a new serial byte representation. length() isused inside the call of encode() to determine the length of the resulting byte sequence. ANSP provides a XDR library called ANSPXdr to ease the jobs of encoding and decoding.B. Active Service Base (ANSPBase)In an active node, the Active Service Base provides a unified interface to export the available resources in EEs for the rest of the ANSP components. The services may include thread management, node query, and soft-store operation, as shown in Table 1.TABLE I. ACTIVE SERVICE BASE FUNCTION CALLSFunction Definition Descriptionboolean send (Capsule, Address) Transmit a capsule towards its destination using the routing table of theunderlying environment.ANSPAddress getLocalHost () Return address of the local host as an ANSPAddress structure. This isuseful when a capsule wants to check its current location.boolean isLocal (ANSPAddress) Return true if its input argument matches the local host’s address andreturn false otherwise.createThread () Create a new thread that is a class ofANSPThreadInterface (discussed later in Section VIA “Unified Thread Abstraction”).putSStore (key, Object) Object getSStore (key) removeSStore (key)The soft-store operations are provided by putSStore(), getSSTore(), and removeSStore(), and they put, retrieve, and remove data respectively. forName (PathName) Supported in ANSP to retrieve a classobject corresponding to the given path name in its argument. This code retrieval may rely on the code loading mechanism in the environment whennecessary.C. Application Client (ANSPClient)boolean start (args[])boolean start (args[],runningEEs) boolean start (args[],startClient)boolean start (args[],startClient, runningEE)Application Client is an interface between users and the nearby active source router. It does the following responsibilities.1. Code registration: It may be necessary to specify thelocation and name of the application code in some execution environments, e.g., ANTS. 2. Application initialization: It includes selecting anexecution environment to execute the application among those are available at the source router. Each active application can create an application client instance by extending the abstract class, ANSPClient . The extension inherits a method, start(), to automatically handle both the registration and initialization processes. All overloaded versions of start() accept a list of arguments, args , that are passed to the application stub during its initialization. An optional argument called runningEEs allows an application client to select a particular set of environment variables, specified by a list of standardized numerical environment ID, the ANEP ID, to perform code registration. If this argument is not specified, the default setting can only include ANTS and ASP. D. Application Stub (ANSPApplication)receive (ANSPCapsule)Application stubs reside at the source and destination routers to initialize the ANSP application after the application clients complete the initialization and registration processes. It is responsible for receiving and serving capsules from the networks as well as actions requested from the clients. A new instance is created by extending the application client abstract class, ANSPApplication . This extension includes the definition of a handling routine called receive(), which is invoked when a stub receives a new capsule.IV. ANSP E XAMPLE : T RACE -R OUTEA testbed has been created to verify the design correctnessof ANSP in heterogeneous environments. There are three types of router setting on this testbed:1. Router that contains ANTS and a ANSP daemonrunning on behalf of ASP; 2. Router that contains ASP and a ANSP daemon thatruns on behalf of ANTS; 3. Router that contains both ASP and ANTS.The prototype is written in Java [11] with a traceroute testing program. The program records the execution environments of all intermediate routers that it has visited between the source and destination. It also measures the RTT between them. Figure 4 shows the GUI from the application client, and it finds three execution environments along the path: ASP, ANTS, and ASP. The execution sequence of the traceroute program is shown in Figure 5.Figure 4. The GUI for the TRACEROUTE Program.The TraceCapsule program code is created byextending the ANSPCapsule abstract class. When execute() starts, it checks the Boolean value of returning to determine if it is returning from the destination. It is set to true if TraceCapsule is traveling back to the source router; otherwise it is false . When traveling towards the destination, TraceCapsule keeps track of the environments and addresses of the routers it has visited in two arrays, path and trace , respectively. When it arrives at a new router, it calls addHop() to append the router address and its environment to these two arrays. When it finally arrives at the destination, it sets returning to false and forwards itself back to the source by calling send().When it returns to source, it invokes deliverToApp() to deliver itself to the application stub that has been running at the source. TraceCapsule carries information in its data field through the networks by executing encode() and decode(), which encapsulates and de-capsulates its data using External Data Representation (XDR) respectively. The syntax of ANSP XDR follows the syntax of XDR library from ANTS. length() in TraceCapsule returns the data length, or it can be calculated by using the primitive types in the XDRlibrary.Figure 5. Flow of the TRACEROUTE Capsules.V. C ONCLUSIONSIn this paper, we present a new unified layered architecture for active networks. The new model is known as Active Network Socket Programming (ANSP). It allows each active application to be written once and run on multiple environments in active networks. Our experiments successfully verify the design of ANSP architecture, and it has been successfully deployed to work harmoniously with ANTS and ASP without making any changes to their architectures. In fact, the unified programming interface layer is light-weighted and can be dynamically deployable upon request.R EFERENCES[1] D.D. Clark, D.L. Tennenhouse, “Architectural Considerations for a NewGeneration of Protocols,” in Proc. ACM Sigcomm’90, pp.200-208, 1990. [2] D. Tennenhouse, J. M. Smith, W. D. Sicoskie, D. J. Wetherall, and G. J.Minden, “A survey of active network research,” IEEE Communications Magazine , pp. 80-86, Jan 1997.[3] D. Wetherall, U. Legedza, and J. Guttag, “Introducing new internetservices: Why and how,” IEEE Network Magazine, July/August 1998. [4] M. Fry, A. Ghosh, “Application Layer Active Networking,” in ComputerNetworks , Vol.31, No.7, pp.655-667, 1999.[5] K. W. Chin, “An Investigation into The Application of Active Networksto Mobile Computing Environments”, Curtin University of Technology, March 2000.[6] S. Bhattacharjee, K. L. Calvert, and E. W. Zegura, “Self OrganizingWide-Area Network Caches”, Proc. IEEE INFOCOM ’98, San Francisco, CA, 29 March-2 April 1998.[7] L. H. Leman, S. J. Garland, and D. L. Tennenhouse, “Active ReliableMulticast”, Proc. IEEE INFOCOM ’98, San Francisco, CA, 29 March-2 April 1998.[8] D. Descasper, G. Parulkar, B. Plattner, “A Scalable, High PerformanceActive Network Node”, In IEEE Network, January/February 1999.[9] E. L. Nygren, S. J. Garland, and M. F. Kaashoek, “PAN: a high-performance active network node supporting multiple mobile code system”, In the Proceedings of the 2nd IEEE Conference on Open Architectures and Network Programming (OpenArch ’99), March 1999. [10] D. L. Tennenhouse, and D. J. Wetherall. “Towards an Active NetworkArchitecture”, In Proceeding of Multimedia Computing and Networking , January 1996.[11] D. J. Wetherall, J. V. Guttag, D. L. Tennenhouse, “ANTS: A toolkit forBuilding and Dynamically Deploying Network Protocols”, Open Architectures and Network Programming, 1998 IEEE , 1998 , Page(s): 117 –129.[12] B. Braden, A. Cerpa, T. Faber, B. Lindell, G. Phillips, and J. Kann.“Introduction to the ASP Execution Environment”: /active-signal/ARP/index.html .[13] “The java language: A white paper,” Tech. Rep., Sun Microsystems,1998.。
毕业设计英文翻译(英文)
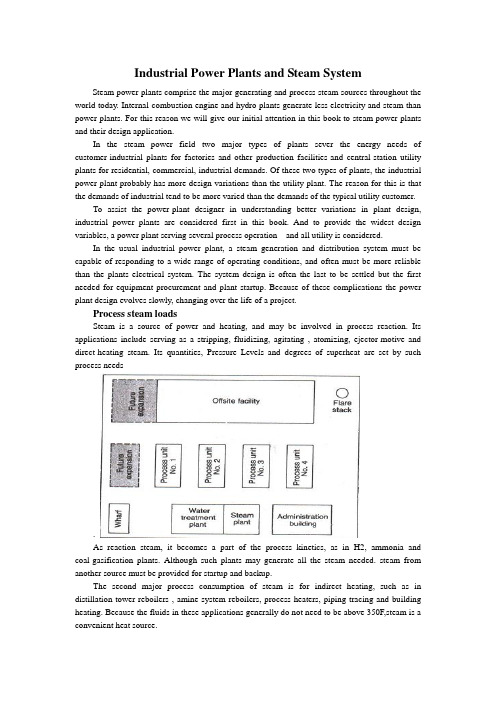
Industrial Power Plants and Steam SystemSteam power plants comprise the major generating and process steam sources throughout the world today. Internal-combustion engine and hydro plants generate less electricity and steam than power plants. For this reason we will give our initial attention in this book to steam power plants and their design application.In the steam power field two major types of plants sever the energy needs of customer-industrial plants for factories and other production facilities-and central-station utility plants for residential, commercial, industrial demands. Of these two types of plants, the industrial power plant probably has more design variations than the utility plant. The reason for this is that the demands of industrial tend to be more varied than the demands of the typical utility customer.To assist the power-plant designer in understanding better variations in plant design, industrial power plants are considered first in this book. And to provide the widest design variables, a power plant serving several process operation and all utility is considered.In the usual industrial power plant, a steam generation and distribution system must be capable of responding to a wide range of operating conditions, and often must be more reliable than the plants electrical system. The system design is often the last to be settled but the first needed for equipment procurement and plant startup. Because of these complications the power plant design evolves slowly, changing over the life of a project.Process steam loadsSteam is a source of power and heating, and may be involved in process reaction. Its applications include serving as a stripping, fluidizing, agitating , atomizing, ejector-motive and direct-heating steam. Its quantities, Pressure Levels and degrees of superheat are set by such process needs.As reaction steam, it becomes a part of the process kinetics, as in H2, ammonia and coal-gasification plants. Although such plants may generate all the steam needed. steam from another source must be provided for startup and backup.The second major process consumption of steam is for indirect heating, such as in distillation-tower reboilers , amine-system reboilers, process heaters, piping tracing and building heating. Because the fluids in these applications generally do not need to be above 350F,steam is a convenient heat source.Again, the quantities of steam required for the services are set by the process design of the facility. There are many options available to the process designer in supplying some of these low-level heat requirements, including heat-exchange system , and circulating heat-transfer-fluid systems, as well as system and electricity. The selection of an option is made early in the design stage and is based predominantly on economic trade-off studies.Generating steam from process heat affords a means of increasing the overall thermal efficiency of a plant. After providing for the recovery of all the heat possible via exchanges, the process designer may be able to reduce cooling requirements by making provisions for the generation of low-pressure(50-150 psig)steam. Although generation at this level may be feasible from a process-design standpoint, the impact of this on the overall steam balance must be considered, because low-pressure steam is excessive in most steam balances, and the generation of additional quantities may worsen the design. Decisions of this type call close coordination between the process and utility engineers.Steam is often generated in the convection section of fired process heaters in order to improve a plant’s thermal efficiency. High-pressure steam can be generated in the furnace convection section of process heater, which have radiant heat duty only.Adding a selective –catalytic-reduction unit for the purpose of lowing NOx emissions may require the generation of waste-heat steam to maintain correct operating temperature to the catalytic-reduction unit.Heat from the incineration of waste gases represents still another source of process steam. Waste-heat flues from the CO boilers of fluid-catalytic crackers and from fluid-coking units, for example, are hot enough to provide the highest pressure level in a steam system.Selecting pressure and temperature levelsThe selecting of pressure and temperature levels for a process steam system is based on:(1)moisture content in condensing-steam turbines,(2)metallurgy of the system,(3)turbine water rates,(4)process requirements ,(5)water treatment costs, and(6)type of distribution system.Moisture content in condensing-steam turbines---The selection of pressure and temperature levels normally starts with the premise that somewhere in the system there will be a condensing turbine. Consequently, the pressure and temperature of the steam must be selected so that its moisture content in the last row of turbine blades will be less than 10-13%. In high speed, a moisture content of 10%or less is desirable. This restriction is imposed in order to minimize erosion of blades by water particles. This, in turn, means that there will be a minimum superheat for a given pressure level, turbine efficiency and condenser pressure for which the system can be designed.System mentallurgy- A second pressure-temperature concern in selecting the appropriate steam levels is the limitation imposed by metallurgy. Carbon steel flanges, for example, are limited to a maximum temperature of 750F because of the threat of graphite (carbides) precipitating at grain boundaries. Hence, at 600 psig and less, carbon-steel piping is acceptable in steam distribution systems. Above 600 psig, alloy piping is required. In a 900- t0 1,500-psig steam system, the piping must be either a r/2 carbon-1/2 molybdenum or a l/2 chromium% molybdenum alloyTurbine water rates - Steam requirements for a turbine are expressed as water rate, i.e., lb of steam/bph, or lb of steam/kWh. Actual water rate is a function of two factors: theoretical water rate and turbine efficiency.The first is directly related to the energy difference between the inlet and outlet of a turbine, based on the isentropic expansion of the steam. It is, therefore, a function of the turbine inlet and outlet pressures and temperatures.The second is a function of size of the turbine and the steam pressure at the inlet, and of turbine operation (i.e., whether the turbine condenses steam, or exhausts some of it to an intermediate pressure level). From an energy stand point, the higher the pressure and temperature, the higher the overall cycle efficiency. _Process requirements - When steam levels are being established, consideration must be given to process requirements other than for turbine drivers. For example, steam for process heating will have to be at a high-enough pressure to prevent process fluids from leaking into the steam. Steam for pipe tracing must be at a certain minimum pressure so that low-pressure condensate can be recovered.Water treatment costs - The higher the steam pressure, the costlier the boiler feedwater treatment. Above 600 psig, the feedwater almost always must be demineralized; below 600 psig, soft,ening may be adequate. It may have to be of high quality if the steam is used in the process, such as in reactions over a catalyst bed (e.g., in hydrogen production).Type of distribution system - There are two types of systems: local, as exemplified by powerhouse distribution; and complex, by wluch steam is distributed to many units in a process plant. For a small local system, it is not impractical from a cost standpoint for steam pressures to be in the 600-1,500-psig range. For a large system, maintaining pressures within the 150-600-psig range is desirable because of the cost of meeting the alloy requirements for higher-pressure steam distribution system.Because of all these foregoing factors, the steam system in a chemical process complex or oil refinery frequently ends up as a three-level arrangement. The highest level, 600 psig, serves primarily as a source of power. The intermediate level, 150 psig, is ideally suitable for small emergency turbines, tracing off the plot, and process heating. The low level, normally 50 psig, can be used for heating services, tracing within the plot, and process requirements. A higher fourth level normally not justified, except in special cases as when alarge amount ofelectric power must be generated.Whether or not an extraction turbine will be included in the process will have a bearing on the intermediate-pressure level selected, because the extraction pressure should be less than 50% of the high-pressure level, to take into account the pressure drop through the throttle valve and the nozzles of the high-pressure section of' the turbine.Drivers for pumps and compressorsThe choice between a steam and an electric driver for a particular pump or compressor depends on a number of things, including the operational philosophy. In the event of a power failure, it must be possible to shut down a plant orderly and safely if normal operation cannot be continued. For an orderly and safe shutdown, certain services must be available during a power failure: (1) instrument air, (2) cooling water, (3) relief and blow down pump out systems, (4) boiler feedwater pumps, (5) boiler fans, (6) emergency power generators, and (7) fire water pumps.These services are normally supplied by steam or diesel drivers because a plant's steam or diesel emergency system is considered more reliable than an electrical tie-line.The procedure for shutting down process units must be analyzed for each type of processplant and specific design. In general, the following represent the minimum services for which spare pumps driven by steam must be provided: column reflux, bottoms and purge-oil circulation, and heater charging. Most important is to maintain cooling; next, to be able to safely pump the plant's inventory into tanks.Driver selection cannot be generalized; a plan and procedure must be developed for each process unit.The control required for a process is at times another consideration in the selection of a driver. For example, a compressor may be controlled via flow or suction pressure. The ability to vary driver speed, easily obtained with a steam turbine, may be basis for selecting a steam driver instead of a constant-speed induction electric motor. This is especially important when the molecular weight of the gas being compressed may vary, as in catalytic-cracking and catalytic-reforming processes.In certain types of plants, gas flow must be maintained to prevent uncontrollable high-temperature excursions during shutdown. For example, hydrocrackers are purged of heavy hydrocarbon with recycle gas to prevent the exothermic reactions from producing high bed temperatures. Steam-driven compressors can do this during a power failure.Each process operation must be analyzed from such a safety viewpoint when selecting drivers for critical equipment. The size of a relief and blowdown system can be reduced by installing steam drivers. In most cases, the size of such a system is based on a total power failure. If heat-removal powered by steam drivers, the relief system can be smaller. For example, a steam driver will maintain flow in the pump-around circuit for removing heat from a column during a power failure, reducing the relief load imposed on the flare system.Equipment support services (such as lubrication and sea-oil systems for compressors) that could be damaged during a loss of power should also be powered by steam drivers.Driver size can also be a factor. An induction electric motor requires large starting currents - typically six times the normal load. The drop in voltage caused by the startup of such a motor imposes a heavy transient demand on the electrical distribution system. For this reason, drivers larger than 10,000 hp are normally steam turbines, although synchronous motors as large as 25,000 hp are used.The reliability of life-support facilities - e.g., building heat, potable water, pipe tracing, emergency lighting-during power failures is of particular concern mates. In such a case, at least one boiler should be equipped with steam-driven auxiliaries to provide these services.Lastly, steam drivers are also selected for the purpose of balancing steam systems and avoiding large amounts of letdown between steam levels. Such decisions regarding drivers are made after the steam balances have been refined and the distribution system has been fully defined. There must be sufficient flexibility to allow balancing the steam system under all operating conditions.Selecting steam driversAfter the number of steam drivers and their services have been established, the utility, or process engineer will estimate the steam consumption for making the steam balance.The standard method of doing this is to use the isentropic expansion of steam correeted for turbine efficiency.Actual steam consumption by a turbine is determined via:SR = (TSR)(bhp)/EHere, SR = actual steam rate, lb/h; TSR = theoretical steam rate, lb/hr/bhp ; bhp = turbine brake horsepower; and E = turbine efficiency.When exhaust steam can be used for process heating, the highest thermodynamic efficiency can be achieved by means of backpressure turbines. Large drivers, which are of high efficiency and require low theoretical steam rates, are normally supplied by the high-pressure header, thus minimizing steam consumption.Small turbines that operate only in emergencies can be allowed to exhaust to atmosphere. Although their water rates are poor, the water lost in short-duration operations may not represent a significant cost. Such turbines obviously play a small role in steam balance planning.Constructing steam balancesAfter the process and steam-turbine demands have been established, the next step is to construct a steam balance for the chemical complex or oil refinery. A sample balance is shown in Fig. 1-4. It shows steam production and consumption, the header systems, letdown stations, and boiler plant. It illustrates a normal (winter) case.It should be emphasized that there is not one balance but a series, representing a variety of operating modes. The object of the balances is to determine the design basis for establishing boiler she, letdown stations and deaerator capacities, boiler feedwater requirements, and steam flows in various parts of the system.The steam balance should cover the following operating modes: normal, all units operating; winter and summer conditions; shutdown of major units; startup of major units; loss of largest condensate source; power failure with flare in service; loss of large process steam generators; and variations in consumption by large steam users.From 50 t0 100 steam balances could be required to adequately cover all the major impacts on the steam system of a large complex.At this point, the general basis of the steam system design should have been developed by the completion of the following work:1. All significant loads have been examined, with particular attention focused on those for which there is relatively little design freedom - i.e., reboilers, sparing steam for process units, large turbines required because of electric power limitation and for shutdown safety.2. Loads have been listed for which the designer has some liberty in selecting drivers. These selections are based on analyses of cost competitiveness.3. Steam pressure and temperature levels have been established.4. The site plan has been reviewed to ascertain where it is not feasible to deliver steam or recover condensate, because piping costs would be excessive.5. Data on the process units are collected according to the pressure level and use of steam - i.e., for the process, condensing drivers and backpressure drivers.6. After Step 5, the system is balanced by trial-and-error calculations or computerized techniques to determine boiler, letdown, deaerator and boiler feedwater requirements.7. Because the possibility of an electric power failure normally imposes one of the major steam requirements, normal operation and the eventuality of such a failure must both be investigated, as a minimum.Checking the design basisAfter the foregoing steps have been completed, the following should be checked:Boiler capacity - Installed boiler capacity would be the maximum calculated (with an allowance of l0-20% for uncertainties in the balance), corrected for the number of boilers operating (and on standby).The balance plays a major role in establishing normal-case boiler specifications, both number and size. Maximum firing typically is based on the emergency case. Normal firing typically establishes the number of boilers required, because each boiler will have to be shut down once a year for the code-required drum inspection. Full-firing levels of the remaining boilers will be set by the normal steam demand. The number of units required (e.g., three 50% units, four 33%units, etc.) in establishing installed boiler capacity is determined from cost studies. It is generally considered double-jeopardy design to assume that a boiler will be out of service during a power failure.Minimum boiler turndown - Most fuel-fired boilers can be operated down to approximately 20% of the maximum continuous rate. The maximum load should not be expected to be below this level.Differences between normal and maximum loads –If the maximum load results from an emergency (such as power failure), consideration should be given to shedding process steam loads under this condition in order to minimize in- stalled boiler capacity. However, the consequences of shedding should be investigated by the process designer and the operating engineers to ensure the safe operation of the entire process.Low-level steam consumption - The key to any steam balance is the disposition of low-level steam. Surplus low-level steam can be reduced only by including more condensing steam turbines in the system, or devising more process applications for it, such as absorption refrigeration for cooling process streams and ranking-cycle systems for generating power. In general, balancing the supply and consumption of low-level steam is a critical factor in the design of the steam system.Quantity of steam at pressure-reducing stations - Because useful work is not recovered from the steam passing through a pressure-reducing station, such flow should be kept at a minimum. In the Fig. 1-5 150/50-psig station, a flow of only 35,000 lb/h was established as normal for this steam balance case (normal, winter). The loss of steam users on the 50-psig systems should be considered, particularly of the large users, because a shutdown of one may demand that the 150/50-psig station close off beyond its controllable limit. If this happened, the 50-psig header would be out of control, and an immediate-pressure buildup in the header wouldbegin, setting off the safety relief valves.The station's full-open capacity should also be checked to ensure that it can make up any 50-psig steam that may be lost through the shutdown of a single large 50-psig source (a turbine sparing a large electric motor, for example}. It would be undesirable for the station to be sized so that it opens more than 80%. In some cases, range ability requirements may dictate two valves (one small and one large).Intermediate pressure level - If large steam users or suppliers may come on stream or go off steam, the normal(day-to-day) operation should be checked. No such change in normal operation should result in a significant upset (e.g.,relief valves set off, or the system pressure control lost).If a large load is lost, the steam supply should be reduced by the letdown-station. If the load suddenly increases, the 600/150-psig station must be able of supplying the additional steam. If steam generated via the process disappears, the station must be capable of making up theload. If150-psig steam is generated unexpectedly, the 600/150-psig station must be able to handle the cutback.The important point here is that where the steam flow could rise t0 700,000 lb/h, this flow should be reduced by a cutback at the 600/150-psig station, not by an increase in the flow to the lower-pressure level, because this steam would have nowhere to go. The normal (600/150-psig) letdown station must be capable of handling some of the negative load swings, even though, overall, this letdown needs to be kept to a minimum.On the other hand, shortages of steam at the 150-psig level can be made up relatively easily via the 600/150-psig station. Such shortages are routinely small in quantity or duration, or both-(startup, purging, electric drive maintenance, process unit shutdown, etc.)High-pressure level - Checking the high-pressure level is generally more straightforward because rate control takes place directly at the boilers. Firing can be increased or lowered to accommodate a shortage or surplus.Typical steam-balance casesThe Fig. 1-4 steam balance represents steady-state condition, winter operation, all process units operating, and no significant unusual demands for steam.An analysis similar to the foregoing might also be required for the normal summertime case, in which a single upset must not jeopardize control but the load may be less (no tank heating, pipe tracing, etc.)The balance representing an emergency (e.g., loss of electric power) is significant. In this case, the pertinent test point is the system's ability to simply weather the upset, not to maintain normal, stable operation. The maximum relief pressure that would develop in any of the headers represents the basis for sizing relief valves. The loss of boiler feed water or condensate return, or both, could result in a major upset, or even a shutdown.Header pressure control during upsetsAt the steady-state conditions associated with the multiplicity of balances, boiler capacity can be adjusted to meet user demands. However, boiler load cannot be changed quickly to accommodate a sharp upset. Response rate is typically limited to 20% of capacity per minute. Therefore, other elements must be relied on to control header pressures during transient conditions.The roles of several such elements in controlling pressures in the three main headers during transient conditions are listed in Table l-3. A control system having these elements will result in a steam system capable of dealing with the transient conditions experienced in moving from one balance point to another.Tracking steam balancesBecause of schedule constraints, steam balances and boiler size are normally established early in the design stage. These determinations are based on assumptions regarding turbine efficiencies, process steam generated in waste-heat furnaces, and other quantities of steam that depend on purchased equipment. Therefore, a sufficient number of steam balances should be tracked through the design period to ensure that the equipment purchased will satisfy the original design concept of the steam system.This tracking represents an excellent application for a utility data-base system and a system linear programming model. During the course of the mechanical design of a large "grass roots" complex, 40 steam balances were continuously updated for changes in steam loads via such an application.Cost tradeoffsTo design an efficient but least-expensive system, the designer ideally develops a total minimum-cost curve – which incorporates all the pertinent costs related to capital expenditures, installation, fuel, utilities, operations and maintenance-and performs a cost study of the final system. However, because the designer is under the constraint of keeping to a project schedule, major, highly expensive equipment must be ordered early in the project, when many key parts of the design puzzle are not available (e.g., a complete load summary, turbine water rates, equipment efficiencies and utility costs).A practical alternative is to rely on comparative-cost estimates, as are conventionally used in assisting with engineering decision points. This approach is particularly useful in making early equipment selections when fine-tuning is not likely to alter decisions, such as regarding the number of boilers required, whether boilers should be shop-fabricated or field-erected, and the practicality of generating steam from waste heat or via cogeneration.The significant elements of a steam-system cost-comparative study are costs for: equipment and installation; ancillaries (i.e., miscellaneous items required to support the equipment,such as additional stacks, upgraded combustion control, more extensive blowdown facilities, etc.); operation(annual); maintenance (annual); and utilities.The first two costs may be obtained from in-house data or from vendors. Operational and maintenance costs can be factored from the capital cost for equipment based on an assessment of the reliability of the purchased equipment.Utility costs are generally the most difficult to establish at an early stage because sources frequently depend on the site of the plant. Some examples of such costs are: purchased fuel gas - $5.35/million Btu, raw water - $0.60/1,000 gal, electricity - $0.07{kWh, and demineralized boiler feedwater -$1.50/1,000 gal. The value of steam at the various pressureLevels can be developed [5J.Let it be further assumed that the emergency balance requires 2,200,000 lb/h of steam (all boilers available). Listed in Table 1 4 are some combinations of boiler installations that meet the design conditions previously stipulated.Table l-4 indicates that any of the several combinations of power-boiler number and size could meet both normal and emergency demand. Therefore, a comparative-cost analysis would be made to assist in making an early decision regarding the number and size of the power boilers.(Table l-4 is based on field-erected, industrial-type boiler Conventional sizing of this type of boiler might range from 100,000 lb/h through 2,000,000 lb/h for each.)An alternative would be the packaged boiler option (although it does not seem practical at this load level. Because it is shop-fabricated, this type of boiler affords a significant saving in terms of field installation cost. Such boilers are available up to a nominal capacity of 100,000 lb/h, with some versions up t0 250,000 lb7h.Selecting turbine water rate i.e., efficiency) represents another major cost concern. Beyond the recognized payout period (e.g., 3 years), the cost of drive steam can be significant in comparison with the equipment capital cost. The typical 30% efficiency ofthe medium-pressure backpressure turbine can be boosted significantly.Driver selections are frequently made with the help of cost-tradeoff studies, unless overriding considerations preclude a drive medium. Electric pump drives are typically recommended on the basis of such studies.Steam tracing has long been the standard way of winterizing piping, not only because of its history of successful performance but also because it is an efficient way to use low-pressure steam.Design consideratonsAs the steam system evolves, the designer identifies steam loads and pressure levels, locates steam loads, checks safety aspects, and prepares cost-tradeoff studies, in order to provide low-cost energy safely, always remaining aware of the physical entity that will arise from the design.How are design concepts translated into a design document? And what basic guidelines will ensure that the physical plant will represent what was intended conceptually?Basic to achieving these ends is the piping and instrument diagram (familiar as the P&ID). Although it is drawn up primarily for the piping designers benefit, it also plays a major role in communicating to the instrumentation designer the process-control strategy, as well as in conveying specialty information to electrical, civil, structural, mechanical and architectural engineers. It is the most important document for representing the specification of the steam。
毕业设计外文翻译模板
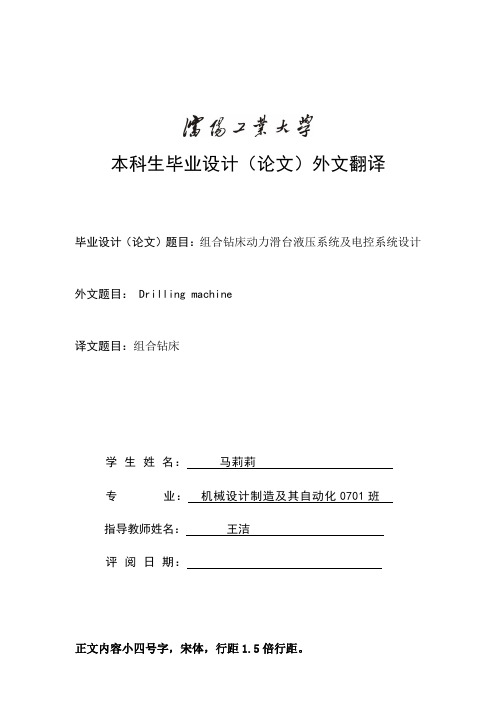
本科生毕业设计(论文)外文翻译毕业设计(论文)题目:组合钻床动力滑台液压系统及电控系统设计外文题目: Drilling machine译文题目:组合钻床学生姓名:马莉莉专业:机械设计制造及其自动化0701班指导教师姓名:王洁评阅日期:正文内容小四号字,宋体,行距1.5倍行距。
The drilling machine is a machine for making holes with removal of chips and it is used to create or enlarge holes. There are many different types of drilling machine for different jobs, but they can be basically broken down into two categories.The bench drill is used for drilling holes through raw materials such as wood, plastic and metal and gets its name because it is bolted to bench for stability so that larger pieces of work can be drilled safely. The pillar drill is a larger version that stands upright on the floor. It can do exactly the same work as the bench drill, but because of its size it can be used to drill larger pieces of materials and produce bigger holes. Most modern drilling machines are digitally automated using the latest computer numerical control (CNC) technology.Because they can be programmed to produce precise results, over and over again, CNC drilling machines are particularly useful for pattern hole drilling, small hole drilling and angled holes.If you need your drilling machine to work at high volume, a multi spindle drill head will allow you to drill many holes at the same time. These are also sometimes referred to as gang drills.Twist drills are suitable for wood, metal and plastics and can be used for both hand and machine drilling, with a drill set typically including sizes from 1mm to 14mm. A type of drill machine known as the turret stores tools in the turret and positions them in the order needed for work.Drilling machines, which can also be referred to as bench mounted drills or floor standing drills are fixed style of drills that may be mounted on a stand or bolted to the floor or workbench. A drilling machine consists of a base, column, table, spindle), and drill head, usually driven by an induction motor.The head typically has a set of three which radiate from a central hub that, when turned, move the spindle and chuck vertically, parallel to the axis of the column. The table can be adjusted vertically and is generally moved by a rack and pinion. Some older models do however rely on the operator to lift and re clamp the table in position. The table may also be offset from the spindles axis and in some cases rotated to a position perpendicular to the column.The size of a drill press is typically measured in terms of swing which can be is defined as twice the throat distance, which is the distance from the centre of the spindle to the closest edge of the pillar. Speed change on these drilling machines is achieved by manually moving a belt across a stepped pulley arrangement.Some drills add a third stepped pulley to increase the speed range. Moderndrilling machines can, however, use a variable-speed motor in conjunction with the stepped-pulley system. Some machine shop drilling machines are equipped with a continuously variable transmission, giving a wide speed range, as well as the ability to change speed while the machine is running.Machine drilling has a number of advantages over a hand-held drill. Firstly, it requires much less to apply the drill to the work piece. The movement of the chuck and spindle is by a lever working on a rack and pinion, which gives the operator considerable mechanical advantage.The use of a table also allows a vice or clamp to be used to position and restrain the work. This makes the operation much more secure. In addition to this, the angle of the spindle is fixed relative to the table, allowing holes to be drilled accurately and repetitively.Most modern drilling machines are digitally automated using the latest computer numerical control (CNC) technology. Because they can be programmed to produce precise results, over and over again, CNC drilling machines are particularly useful for pattern hole drilling, small hole drilling and angled holes.Drilling machines are often used for miscellaneous workshop tasks such as sanding, honing or polishing, by mounting sanding drums, honing wheels and various other rotating accessories in the chuck. To add your products click on the traders account link above.You can click on the links below to browse for new, used or to hire a drilling machine.Drilling machines are used for drilling, boring, countersinking, reaming, and tapping. Several types are used in metalworking: vertical drilling machines, horizontal drilling machines, center-drilling machines, gang drilling machines, multiple-spindle drilling machines, and special-purpose drilling machines.Vertical drilling machines are the most widely used in metalworking. They are used to make holes in relatively small work-pieces in individual and small-lot production; they are also used in maintenance shops. The tool, such as a drill, countersink, or reamer, is fastened on a vertical spindle, and the work-piece is secured on the table of the machine. The axes of the tool and the hole to be drilled are aligned by moving the workpiece. Programmed control is also used to orient the workpiece and to automate the operation. Bench-mounted machines, usually of the single-spindle type, are used to make holes up to 12 mm in diameter, for instance, in instrument-making.Heavy and large workpieces and workpieces with holes located along a curved edge are worked on radial drilling machines. Here the axes of the tool and the hole to be drilled are aligned by moving the spindle relative to the stationary work-piece.Horizontal drilling machines are usually used to make deep holes, for instance, in axles, shafts, and gun barrels for firearms and artillery pieces.Center-drilling machines are used to drill centers in the ends of blanks. They are sometimes equipped with supports that can cut off the blank before centering, and in such cases they are called center-drilling machines. Gang drilling machines with more than one drill head are used to produce several holes at one time. Multiple-spindle drilling machines feature automation of the work process. Such machines can be assembled from several standardized, self-contained heads with electric motors and reduction gears that rotate the spindle and feed the head. There are one-, two-, and three-sidedmultiple-spindle drilling machines with vertical, horizontal, and inclined spindles for drilling and tapping. Several dozen such spindles may be mounted on a single machine. Special-purpose drilling machines, on which a limited range of operations is performed, are equipped with various automated devices.Multiple operations on workpieces are performed by various combination machines. These include one- and two-sided jig boring machines,drilling-tapping machines (usually gang drilling machines with reversible thread-cutting spindles), milling-type drilling machines and drilling-mortising machines used mainly for woodworking, and automatic drilling machines.In woodworking much use is made of single- and multiple-spindle vertical drilling machines, one- and two-sided, horizontal drilling machines (usually with multiple spindles), and machines equipped with a swivel spindle that can be positioned vertically and horizontally. In addition to drilling holes, woodworking machines may be used to make grooves, recesses, and mortises and to remove knots.英文翻译指导教师评阅意见。
毕业设计英文翻译
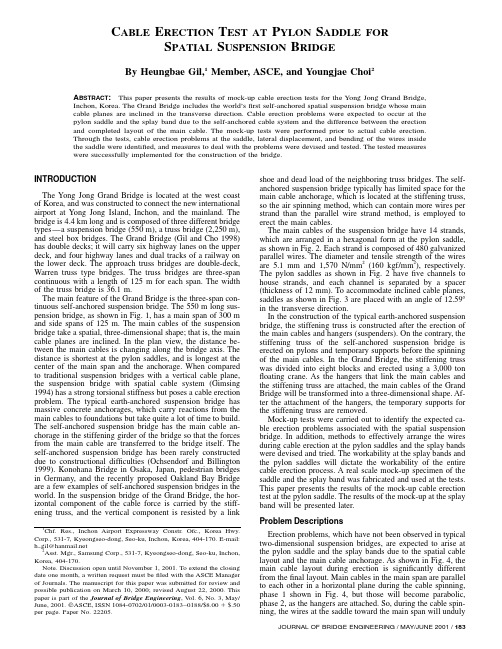
INTRODUCTION
The Yong Jong Grand Bridge is located at the west coast of Korea, and was constructed to connect the new international airport at Yong Jong Island, Inchon, and the mainland. The bridge is 4.4 km long and is composed of three different bridge types—a suspension bridge (550 m), a truss bridge (2,250 m), and steel box bridges. The Grand Bridge (Gil and Cho 1998) has double decks; it will carry six highway lanes on the upper deck, and four highway lanes and dual tracks of a railway on the lower deck. The approach truss bridges are double-deck, Warren truss type bridges. The truss bridges are three-span continuous with a length of 125 m for each span. The width of the truss bridge is 36.1 m.
shoe and dead load of the neighboring truss bridges. The selfanchored suspension bridge typically has limited space for the main cable anchorage, which is located at the stiffening truss, so the air spinning method, which can contain more wires per strand than the parallel wire strand method, is employed to erect the main cables.
(完整版)_毕业设计英文翻译_及格式

毕业设计(论文)英文翻译题目专业班级姓名学号指导教师职称200年月日The Restructuring of OrganizationsThroughout the 1990s, mergers and acquisitions a major source of corporate restructuring, affecting millions of workers and their families. This form of restructuring often is accompanied by downsizing. Downsizing is the process of reducing the size of a firm by laying off or retiring workers early. The primary objectives of downsizing are similar in U.S. companies and those in other countries:●cutting cost,●spurring decentralization and speeding up decision making,●cutting bureaucracy and eliminating layers of especially they did five years ago. One consequence of this trend is that today’s managers supervise larger numbers of subordinates who report directly to them. In 1990, only about 20 percent of managers supervise twelve or more people and 54 percent supervised six or fewer.Because of downsizing, first-line managers quality control, resources, and industrial engineering provide guidance and support. First-line managers participate in the production processes and other line activities and coordinate the efforts of the specialists as part of their jobs. At the same time, the workers that first-line managers supervise are less willing to put up with authoritarian management. Employees want their jobs to be more creative, challenging, fun, and satisfying and want to participate in decisions affecting their work. Thus self-managed work teams that bring workers and first-line managers together to make joint decisions to improve the way they do their jobs offer a solution to both supervision and employee expectation problems. When you ’t always the case. Sometimes entire divisions of a firm are simply spun off from the main company to operate on their own as new, autonomous companies. The firm that spun them off may then become one of their most important customers or suppliers. That AT&T “downsized” the old Bell Labs unit, which is now known as Lucent Technologies. Now, rather than - return is free to enter into contracts with companies other than AT&T. this method of downsizing is usually called outsourcing.Outsourcing means letting other organizations perform a needed service andor manufacture needed parts or products. Nike outsources the production of its shoes to low-cost plants in South Korea and China and imports the shoes for distribution in North America. These same plants also ship shoes to Europe and other parts of Asia for distribution. Thus today’s managers face a new challenge: t o plan, organize, lead, and control a company that may as a modular corporation. The modularcorporation is most is most common in three industries: apparel, auto manufacturing, and electronics. The most commonly out-sourced function is production. By out sourcing production, a company can switch supplier best suited to a customer’s needs.Decisions about what to outsource and what to keep in- to contract production to another company is a sound business decision to contract production to another company is a sound business decision, at least for U.S. manufacturers. It appears to the unit cost of production by relieving the company of some overhead, and it frees the company to allocate scarce resources to activities for which the company examples of modular companies are Dell Computer, Nike, Liz Claiborne fashions, and ship designer Cyrix.As organizations downsize and outsource functions, they become flatter and smaller. Unlike the behemoths of the past, the new, smaller firms are less like autonomous fortresses and more like nodes in a net work of complex relationships. This approach, called the network form of organization, involves establishing strategic alliances among several entities.In Japan, cross-ownership and alliances among firms-called keiretsu-both foreign and U.S. auto parts producers. It also owns 49 percent of Hertz, the car rental company that is also a major customer. Other alliances include involvement in several research consortia. In the airline industry, a common type of alliance is between an airline and an airframe manufacture. For example, Delta recently agreed to buy all its aircraft from Boeing. Boeing Airlines. Through these agreements, Boeing guarantees that it will be able to sell specified models of its aircraft and begin to adapt their operations to the models they will be flying in the future. Thus both sides expect to reap benefits from these arrangements for many years.Networks forms of organizations are prevalent in access to the universities and in small, creative organizations. For example, the U.S. biotechnology industry is characterized by network of relationships between new biotechnology firms dedicated to research and new products development and established firms in industries that can use these new products, such as pharmaceuticals. In return for sharing technical information with the larger firms, the smaller firms gain access to their partners’ resources for product testing, marketing, and distribution. Big pharmaceutical firms such as Merk or Eli Lily gain from such partnerships because the smaller firms typically development cycle in the larger firms.Being competitive increasingly requires establishing and managing strategic alliances with other firms. In a strategic alliance, two or more firms agree to cooperate in a venture that is expected to benefit both firms.企业重组整个20世纪90年代中,合并和收购一直是企业重组的主要起源,影响着千百万的工人和他们的家庭。
毕业设计英文翻译
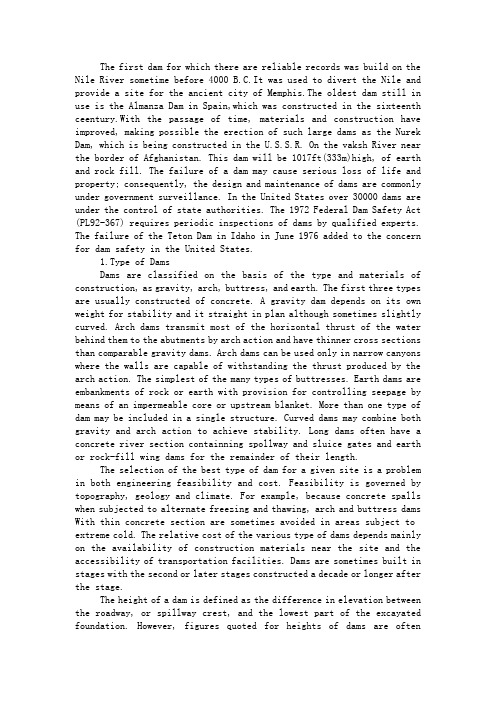
The first dam for which there are reliable records was build on the Nile River sometime before 4000 B.C.It was used to divert the Nile and provide a site for the ancient city of Memphis.The oldest dam still in use is the Almanza Dam in Spain,which was constructed in the sixteenth ceentury.With the passage of time, materials and construction have improved, making possible the erection of such large dams as the Nurek Dam, which is being constructed in the U.S.S.R. On the vaksh River near the border of Afghanistan. This dam will be 1017ft(333m)high, of earth and rock fill. The failure of a dam may cause serious loss of life and property; consequently, the design and maintenance of dams are commonly under government surveillance. In the United States over 30000 dams are under the control of state authorities. The 1972 Federal Dam Safety Act (PL92-367) requires periodic inspections of dams by qualified experts. The failure of the Teton Dam in Idaho in June 1976 added to the concern for dam safety in the United States.1.Type of DamsDams are classified on the basis of the type and materials of construction, as gravity, arch, buttress, and earth. The first three types are usually constructed of concrete. A gravity dam depends on its own weight for stability and it straight in plan although sometimes slightly curved. Arch dams transmit most of the horizontal thrust of the water behind them to the abutments by arch action and have thinner cross sections than comparable gravity dams. Arch dams can be used only in narrow canyons where the walls are capable of withstanding the thrust produced by the arch action. The simplest of the many types of buttresses. Earth dams are embankments of rock or earth with provision for controlling seepage by means of an impermeable core or upstream blanket. More than one type of dam may be included in a single structure. Curved dams may combine both gravity and arch action to achieve stability. Long dams often have a concrete river section containning spollway and sluice gates and earth or rock-fill wing dams for the remainder of their length.The selection of the best type of dam for a given site is a problem in both engineering feasibility and cost. Feasibility is governed by topography, geology and climate. For example, because concrete spalls when subjected to alternate freezing and thawing, arch and buttress dams With thin concrete section are sometimes avoided in areas subject to extreme cold. The relative cost of the various type of dams depends mainly on the availability of construction materials near the site and the accessibility of transportation facilities. Dams are sometimes built in stages with the second or later stages constructed a decade or longer after the stage.The height of a dam is defined as the difference in elevation between the roadway, or spillway crest, and the lowest part of the excayated foundation. However, figures quoted for heights of dams are oftendetemined in other ways. Frequently the height is taken net height above the old riverbed.。
毕业设计翻译定稿 英汉对照(绝版)
A Comparison of AASHTO Bridge Load Rating Methods Authors:Cristopher D. Moen, Ph.D., P.E., Virginia Tech, Blacksburg, VA, cmoen@Leo Fernandez, P.E., TranSystems, New York, NY, lafernandez@INTRODUCTIONThe capacity of an existing highway bridge is traditionally quantified with a load rating factor. This factor, when multiplied by the design live load magnitude, describes the total live load a bridge can safely carry. The load rating factor, RF, is related to the capacity of the controlling structural component in the bridge, C, and the dead load D and live load L applied to that component with the equation:L DC RF -=(1)Visual bridge inspections provide engineers with information to quantify the degradation in structural integrity of a bridge (i.e., the reduction in C). The trends in RF over time can be employed by bridge owners to make decisions regarding bridge maintenance and replacement. For example, when a bridge is first constructed, RF=1.3 means that a bridge can safely carry 1.3 times the weight of its design live load (i.e., that C-D, the existing capacity after accounting for dead load, is 1.3 times the design live load L). If the RF decreases to 0.8 after 20 years of service, deterioration of the primary structural components has most likely occurred and rehabilitation or replacement should be considered.Equation (1) is a simple idea, but C, D, and L can be highly variable and difficult to characterize depending upon the bridge location, bridge type, daily traffic flow, structural system (e.g., simple or continuous span) and choice of constructionmaterials (e.g. steel, reinforced or prestressed concrete, composite construction). The American Association of State Highway and Transportation Officials (AASHTO) Manual for Condition Evaluation of Bridges (MCEB) provides a formal load rating procedure to assist engineers in the evaluation of existing bridges [AASHTO 1994 with interims through 2003]. The MCEB provides two load rating methods, one based on an allowable stress approach (ASR) and another based on a load factor approach (LFR). Both the ASR and LFR methods are consistent with the design loading and capacity calculations outlined in the AASHTO Standard Specification for the Design of Highway Bridges [AASHTO 2002]. Recently momentum has shifted towards a probabilistic-based bridge design approach with the publication of the AASHTO LRFD Bridge Design Specifications [AASHTO 2007]. Bridges designed with this code have a uniform probability of failure (i.e., a uniform reliability). The AASHTO Manual for Condition Evaluation and Load and Resistance Factor Rating (LRFR) of Highway Bridges [AASHTO 2003] extends this idea of uniform reliability from LRFD to the load rating of existing bridges and is currently the recommended load rating method (over the ASR and LFR methods) by the Federal Highway Administration (FHWA).The transition from ASR and LFR to LRFR bridge load rating methodology represents a positive shift towards a more accurate and rational bridge evaluation strategy. Bridge owners are optimistic that the LRFR load rating methodology will improve bridge safety and economy, but they are also currently dealing with the tough questions related to its implementation. Why do ASR, LFR, and LRFR methods produce different load rating factors for the same bridge? Should we change the posting limit on a bridge if the LRFR rating is lower than the MCEB ratings? What are the major philosophical differences between the three methods? It is the goal of this paper to answer some of these questions (and at the same time dispel common myths) with a succinct summary of the history of the three methods. A comparison of the LFR and LRFR methods for a typical highway bridge will also bepresented, with special focus on the benefits inherent in the rational, probabilistic approach of the LRFR load rating method. This paper is also written to serve as an introduction to load rating methodologies for students and engineers new to the bridge evaluation field.S UMMARY OF EXISTING LITERATURESeveral reports have been published which summarize the development of AASHTO design and load rating methodologies. FHWA NHI Report 07-019 is an excellent historical reference describing the evolution of AASHTO live loadings (including the HS20-44 truck) and load factor design [Kulicki 2007b]. NCHRP Report 368 describes the development of the AASHTO LRFD design approach[Nowak 1999], and is supplemented by the NCHRP Project No. 20-7/186 report[Kulicki 2007a] with additional load factor calibration research. NCHRP Report 454 documents the calibration of the AASHTO LRFR load factors [Moses 2000], and NCHRP Web Document 28 describes the implementation of the LRFR load rating method [NCHRP 2001]. The NCHRP Project 20-7/Task 122 report supplements Web Document 28 with a detailed comparison of the LRFR and LFD load rating approaches [Mertz 2005].AASHTO A LLOWABLE STRESS RATING METHODThe Allowable Stress Rating (ASR) method is the most traditional of the three load rating methods, primarily because the performance of a bridge is evaluated under service conditions in the load rating equation [AASHTO 1994]:)1(21l L A D A C RF +-= (2) C is calculated with a “working stress” approach where the capacity of the primary structural members is limited to a proportion of the assumed failure stress (e.g., 0.55F y for structural steel in tension and 0.3f’c for concrete in compression.) Consistent with the service level approach, the demand dead load D and live load Lare unfactored, i.e. A 1=1.0 and A 2=1.0.The uncertainty in the strength of the bridge is accounted for in the ASR approach by limiting the applied stresses, but the variability in the demand loads is neglected. For example, dead load on a bridge has a relatively low variability because the dimensional tolerances of the primary structural members (e.g., a hot-rolled steel girder) are small [Nowak 2000]. Vehicular traffic loads on a bridge have a higher uncertainty because of varying traffic volume (annual average daily truck traffic or ADTT) and varying types of vehicular traffic (e.g., primarily trucks on an interstate or primarily cars on a parkway). The ASR method also does not consider redundancy of a bridge (e.g., continuous or simple spans, hammerhead piers or multiple column bents) or the amplified uncertainty in the capacity of aging structural members versus newly constructed members. The ASR method’s treatment of capacity and demand results in load rating factors lacking a uniform level of reliability (i.e., a uniform probability of failure) across all types of highway bridges. For example, with the ASR method, two bridges can have RF=2 even though one bridge carries a high ADTT with a non-redundant superstructure (higher probability of failure) while the other bridge carries a low AADT with a redundant superstructure (lower probability of failure).AASHTO L OAD F ACTOR R ATING METHODIn contrast to the ASR method’s service load approach to load rating, the AASHTO Load Factor Rating (LFR) method evaluates the capacity of a bridge at its ultimate limit state . The LFR load rating factor equation is:12(1)nR A D RF A L I φ-=+ (3) where the capacity C of the bridge in (2) has been replaced with φ R n , the predicted strength of the controlling structural component in the bridge. R n is the nominal capacity of the structural component and φ is a strength reduction factor which accounts for the uncertainty associated with the material properties,workmanship, and failure mechanisms (e.g., shear, flexure, or compression). For example, φ is 0.90 for the flexural strength of a concrete beam and 0.70 for a concrete column with transverse ties [AASHTO 2002]. The lower φ for the concrete column means that there is more uncertainty inherent in the structural behavior and strength prediction for a concrete column than for a concrete beam. The dead load factor A 1 is 1.3 to account for unanticipated permanent load and A 2 is either 1.3 or2.17, defining a live load envelope ranging from an expected design level (Inventory) to an extreme short term loading (Operating) [AASHTO 1994].The LFR method is different from the ASR method because it calculates the load rating factor RF by quantifying the potential for failure of a bridge (and associated loss of life and property) instead of quantifying the behavior of a bridge in service . The LFR method is similar to the ASR method in that it does not account for the influence of redundancy on the reliability of a bridge. Also, the load factors A 1 and A 2 are defined without a formal reliability analysis (i.e., they are not derived by considering probability distributions of capacity and demand) and therefore do not produce rating factors consistent with a uniform probability of failure.AASHTO L OAD AND R ESISTANCE F ACTOR R ATING METHODThe AASHTO Load and Resistance Factor Rating (LRFR) method evaluates the existing capacity of a bridge using structural reliability theory [Melchers 1999; Nowak 2000]. The LRFR rating factor equation is similar in form to (2) and (3):(1)c s n DC DW L R DC DW RF LL IM ϕϕϕγγγ--=+ (4) where ϕc is a strength reduction factor that accounts for the increased variability in the member strength of existing bridges when compared to new bridges [Moses 1987]. The factor ϕs addresses the failure of structural systems and penalizes older non-redundant structures with lower load ratings [Ghosn 1998]. The dead load factors γDC and γDW have been separated in LRFR to account for a lower variability indead load for primary structural components DC (e.g., columns and beams) and a higher variability for bridge deck wearing surfaces DW.Another important difference between the LRFR method and the ASR and LFR methods is the use of the HL93 notional design live load, which is a modern update to the HS20-44 notional load first implemented in 1944 [Kulicki 2007b] (notional in this case means that the design live load is not meant to represent actual truck traffic but instead is a simplified approximation intended to conservatively simulate the influence of live load across many types of bridge spans). The HL93 loading produces live load demands which are more consistent with modern truck traffic than the HS20-44 live load. The HL93 design loading combines the HS20-44 truck with a uniform load and also considers the load case of a tandem trailer with closely spaced axles and relatively high axle loads (in combination with a uniform load) [AASHTO 2007]. The design tandem load increases the shear demand on shorter bridges and produces, in combination with the design lane load, a live load effect greater than or equal to the AASHTO legal live load Type 3, Type 3S2, and Type 3-3 vehicles [AASHTO 1994].AASHTO LFR VS. LRFR LOAD RATING COMPARISONA parameter study is conducted in this section to explore the differences between the AASHTO LFR and LRFD load rating methods. The ASR method is not included in the study because it evaluates the live load capacity of a bridge at service levels, which makes it difficult to compare against the ultimate limit state LFR and LRFR methods (also note that the ASR method employs less modern “working stress” methods for calculating member capacities than LFR and LRFR). A simple span multi-girder bridge with steel girders and a composite concrete bridge deck is considered. The flexural capacity of an interior girder is assumed to control the load rating. AASHTO legal loads are employed in the study to provide a consistent live loading between the rating methods (although the impact factor and live loaddistribution factor for the controlling girder will be different for LFR and LRFR methods).The LFR load rating equation in (3) is rewritten as:u 12LFR LFD LFD M A D RF A B I L-= (5) where M u is the LFD flexural capacity of the composite girder (φ is implicit in the calculation of M u ), B LFD is the live load distribution factor for an interior girder[AASHTO 1994]:5.5LFD S B = (6) and the live load impact factor I LFD is [AASHTO 1994]:501125LFD I =++ (7) The span length of the bridge is denoted as . A 1 and A 2 are chosen as 1.3 in this study to compare the LFR Operating rating with the LRFR rating method (the intent of the LRFR legal load rating is to provide a single rating level consistent with the LFD Operating level [AASHTO 2003]).The LRFR equation in (4) is rewritten to be consistent with (5):u2c s DC D LFR L LRFD LRFD M M RF B I Lϕϕγγ-= (8) Where B LRFD is the live load distribution factor for moment in an interior girder[AASHTO 2007]0.60.230.075()()()9.512g LRFD sK S S B t =+ (9) and I LRFD , the live load impact factor, is 1.33 [AASHTO 2007]. M D is the dead load moment assuming that the dead load effects from a wearing surface and utilities are zero (i.e., DW is zero) and γDC is 1.25. M u is assumed equivalent in (5) and (8) because the LFD and LRFD prediction methods for the flexural capacity of composite girders are founded on a common structural basis [Tonias 2007]. The term K g /12 t s 3 in (9) is assumed equal to 1 as suggested by the LRFD specification forpreliminary design [AASHTO 2007] (this approximation reduces the number of variables in the parameter study). The term LL in (4), i.e. the LRFD lane loading, is approximated by 2L in (8). This conversion from lane loading to wheel line loading allows for the cancellation of L (i.e., the live load variable) when (8) and (5) are formulated as a ratio:(10)Rearranging the term M u in (10) leads to:(11)The relationship between the LRFR and LFR load rating equations, as described in (11), is explored in Figure 1 to Figure 4. M D/M u is assumed as 0.30 for the bridge span lengths considered in this study. Equation (11) varies only slightly (a maximum of 5%) when M D/M u ranges between 0.10 to 0.50 because the LFR and LRFR dead load factors are similar, i.e. γDC=1.25 and A1=1.3. Figure 1 demonstrates that the LRFR legal load rating is less than the LFD Operating rating for both short and long single span bridges (the span range is 20 ft. to 200 ft. in this study). This is consistent with the findings of NCHRP Web Document 28, which demonstrates that the LRFR legal load rating is lower than the LFD Operating rating but higher than the LFD Inventory rating [NCHRP 2001]. RF LRFR increases for longer span lengths because the live load distribution factor B LRFD in (9) decreases with increasing . RF LRFR also increases as the girder spacing, S, increases (S ranges from 3 ft. to 7 ft. in Figure 1) because the LRFD live load distribution factor B LRFD decreases relative to the LFD live load distribution factor B LFD for larger girder spacings.FIGURE 1-COMPARISON OF LRFR AND LFR (OPERATING) LEGAL LOAD RATING FACTORS FOR FLEXURE IN AN INTERIOR GIRDER OF A SIMPLE SPAN MULTI-GIRDER COMPOSITE BRIDGEThe volume of traffic is directly accounted for in the LRFR load rating method by considering the Average Daily Truck Traffic (ADTT) (this is an improvement over the LFR method which does not account for frequency of bridge usage when calculating RF). Figure 2 highlights the variability of the LRFR legal load rating with ADTT. RF LRFR is approximately 30% greater for a lightly traveled bridge (ADTT≤100) when compared to a heavily traveled bridge (ADTT≥5000), and the LRFR load rating trends toward the LFD Operating load rating for lightly traveled bridges.FIGURE 2 - INFLUENCE OF ANNUAL DAILY TRUCK TRAFFIC ON THE LRFR LEGAL LOAD RATING FACTOR (S=4 FT.)The factors ϕs and ϕc account for system redundancy and the increased uncertainty from bridge deterioration in the LRFR load rating method respectively (this is an important update to the LFR rating method which assumes one level of uncertainty for all bridge types and bridge conditions). Figure 3 demonstrates that RF LRFR decreases by approximately 30% as the bridge condition deteriorates from good to poor. Bridges with a small number of girders (e.g., 3 or 4 girders) are considered to be more susceptible to catastrophic collapse, which is reflected in the lower RF LRFR load rating factors in Figure 4.FIGURE 3 –INFLUENCE OF CONDITION FACTOR ϕs ON THE LRFR LOAD RATING FACTOR (S=4 FT.)FIGURE 4 - INFLUENCE OF SYSTEM FACTOR ϕc ON LRFR LOAD RATING FACTOR (S=4 FT.)D ISCUSSIONThe LRFR load rating method represents an important step in the evolution of bridge evaluation strategies. The method is calibrated to produce a uniform level of reliability across all existing highway bridges (i.e., a uniform probability of failure) and is an improvement over the ASR and LFR methods because it allows bridge owners to account for traffic volume, system redundancy, and the increased uncertainty in the predicted strength of deteriorating bridge components. The LRFR load rating method can be used as a foundation for the development of more accurate performance-based bridge evaluation strategies in the future, where bridge owners directly calculate the existing capacity (or reliability) with in service data from a structural health monitoring network and make maintenance decisions based on relationships between corrosion, structural capacity, and repair or replacement costs.Reliability-based cost models have been proposed, for example [Nowak 2000]: T I F F C C C P =+ (12)Where CT is the total cost of the bridge over its lifetime, CI is the initial cost, CF is the failure cost of the bridge (which could include rehabilitation costs), and PF is the failure probability of the bridge. As PF increases (i.e., as the bridge deteriorates over time), the total cost CT increases, which ties the reliability of the bridge to economy and provides a metric from which to optimize maintenance decisions and minimum rehabilitation costs in a highway system. The continued evolution of bridge evaluation strategies depends on improved methods for evaluating the structural capacity of bridges and defining correlation between corrosion in bridges, strength loss, and failure rates [ASCE 2009].The AASHTO LRFR load rating method is a step forward in bridge evaluation strategy when compared to the ASR and LFR methods because it is calibrated to produce a uniform reliability across all existing highway bridges. The LRFR method provides factors which account for the volume of traffic on the bridge, the redundancy of the superstructure, and the increased uncertainty in structural capacity associated with a deteriorating structure. The flexural LRFR load rating factor for an interior steel composite girder in a multi-girder bridge is up to 40% lower than the LFR Operating load rating over a span range of 20 ft. to 200 ft. and for girder spacings between 3 ft. and 7 ft. The LRFR flexural load rating factor increases for longer span lengths and larger girder spacings, influenced primarily by the LRFD live load distribution factor.A CKNOWLEDGEMENTSThe authors are grateful for the guidance provided by Bala Sivakumar in the organization of this paper. The authors also wish to thank Kelley Rehm and Bob Cullen at AASHTO for their help identifying historical references pertaining to AASHTO live load vehicles and design procedures.R EFERENCESAASHTO, Manual for Condition Evaluation of Bridges, Second Edition, with 1995, 1996, 1998, 2000, 2001, and 2003 Revisions, AASHTO, Washington, D.C., 1994.AASHTO, Standard Specifications for Highway Bridges, 17th Edition, AASHTO, Washington, D.C., 2002.AASHTO, Manual for Condition Evaluation and Load and Resistance Factor Rating (LRFR) of Highway Bridges, AASHTO, Washington, D.C., 2003.AASHTO, LRFD Bridge Design Specifications, 4th Edition, AASHTO, Washington, D.C., 2007.ASCE, "ASCE/SEI-AASHTO Ad-Hoc Group on Bridge Inspection, Rehabilitation, and Replacement White Paper on Bridge Inspection and Rating", ASCE Journal of Bridge Engineering, 14(1), 2009, 1-5.Ghosn, M., Moses, F., NCHRP Report 406: Redundancy in Highway Bridge Superstructures, TRB, National Research Council, Washington, D.C., 1998.Kulicki, J.M., Prucz, Zolan, Clancy, Chad M., Mertz, Dennis R., Updating the Calibration Report for AASHTO LRFD Code (Project No. NCHRP 20-7/186), AASHTO, Washington, DC, 2007a.Kulicki, J.M., Stuffle, Timothy J., Development of AASHTO Vehicular Loads (FWHA NHI 07-019), Federal Highway Administration, National Highway Institute (NHNI-10), 2007b.Melchers, R.E., Structural Reliability Analysis and Prediction, John Wiley and Sons, New York, 1999.Mertz, D.R., Load Rating by Load and Resistance Factor Evaluation Method (NCHRP Project 20-07/Task 122), TRB, National Research Council, Washington DC, 2005.Moses, F., NCHRP Report 454: Calibration of Load Factors for LRFR BridgeEvaluation, TRB, National Research Council, Washington, D.C., 2000.Moses, F., Verma, D., NCHRP Report 301: Load Capacity Evaluation of Existing Bridges, TRB, National Research Council, Washington, D.C., 1987.NCHRP, Manual for Condition Evaluation and Load Rating of Highway Bridges Using Load and Resistance Factor Philosophy (NCHRP Web Document 28), TRB, National Research Council, Washington DC, 2001.Nowak, A.S., NCHRP Report 368: Calibration of LRFD Bridge Design Code, TRB, National Research Council, Washington D.C., 1999.Nowak, A.S., Collins, Kevin R., Reliability of Structures, McGraw Hill, New York, 2000.Tonias, D.E., Zhao, J.J., Bridge Engineering: Design, Rehabilitation, and Maintentance of Modern Highway Bridges, McGraw-Hill, New York, 2007.AASHTO关于桥梁荷载等级评定方法的比较作者:Cristopher D. Moen,Ph.D.,P.E.,Virginia Tech,Blacksburg,VA,cmoen@Leo Fernandez,P.E.,TranSystems,New York,NY,lafernandez@绪论:现有的高速公路桥梁的承载能力是用传统单一荷载等级因数定量化的。
毕业设计英文翻译
毕业设计英文翻译Graduation DesignAbstract:This graduation design aims to propose a solution for improving the efficiency of waste management in urban areas. The current waste management system suffers from various problems such as inadequate collection practices, lack of recycling infrastructure, and improper disposal of hazardous waste. To address these issues, this design proposes the implementation of a comprehensive waste management system, which includes improved collection practices, the establishment of recycling centers, and the introduction of stricter waste disposal regulations. The design also includes the development of a mobile application to facilitate communication between residents and waste management authorities. This design offers a feasible and sustainable solution to improve waste management in urban areas.Introduction:Waste management has become an increasingly important issue for urban areas due to the rapid growth of population and industrial activities. The current waste management system in many cities is unable to cope with the increasing volume of waste being generated. This has led to numerous problems such as environmental pollution, health hazards, and the inefficient utilization of resources. Therefore, there is a need for an improved waste management system that can effectively address these issues. Methodology:This design proposes several key strategies to improve wastemanagement in urban areas. Firstly, there needs to be an improvement in waste collection practices. This can be achievedby implementing a more efficient collection schedule and ensuring that waste collection trucks cover all areas of the city. Additionally, separate collection bins for recyclable and non-recyclable waste should be provided to encourage recycling.Secondly, it is essential to establish recycling centers in strategic locations throughout the city. These centers will act as collection points for recyclable materials and will also provide education and awareness programs to promote recycling among residents. Furthermore, partnerships with recycling companies should be established to ensure the proper processing and utilization of recyclable materials.Lastly, stricter waste disposal regulations should be introduced to discourage improper disposal of hazardous waste. This can be achieved by imposing fines and penalties on individuals and businesses that do not comply with waste disposal regulations. Additionally, educational campaigns should be conducted to raise awareness about the dangers of improper waste disposal and to promote responsible waste management practices.Results and Discussion:The proposed waste management system, if implemented, will have several benefits. Firstly, it will contribute to a cleaner and healthier environment by reducing pollution and preventing the spread of diseases. Secondly, the establishment of recycling centers will promote resource conservation and reduce the dependence on raw materials. Finally, the introduction of stricterwaste disposal regulations will ensure proper handling of hazardous waste, thus protecting human health and the environment.Conclusion:In conclusion, the implementation of a comprehensive waste management system is necessary to address the shortcomings of the current waste management system in urban areas. This design proposes several key strategies such as improved collection practices, the establishment of recycling centers, and the introduction of stricter waste disposal regulations. The design also includes the development of a mobile application to enhance communication between residents and waste management authorities. By implementing these strategies, this design offers a feasible and sustainable solution to improve waste management in urban areas.。
毕业设计中英文翻译
1 Introduction and scope1.1 Aims of the ManualThis Manual provides guidance on the design of reinforced and prestressed concrete building structures. Structures designed in accordance with this Manual will normally comply with DD ENV 1992-1-1: 19921 (hereinafter referred to as EC2).1.2 Eurocode systemThe structural Eurocodes were initiated by the European Commission but are now produced by the Comité Européen de Normalisation (CEN) which is the European standards organization, its members being the national standards bodies of the EU and EFTA countries,e.g. BSI.CEN will eventually publish these design standards as full European Standards EN (Euronorms), but initially they are being issued as Prestandards ENV. Normally an ENV has a life of about 3 years to permit familiarization and trial use of the standard by member states. After formal voting by the member bodies, ENVs are converted into ENs taking into account the national comments on the ENV document. At present the following Eurocode parts have been published as ENVs but as yet none has been converted to an EN:DD ENV 1991-1-1: Basis of design and actions on structures (EC1)DD ENV 1992-1-1: Design of concrete structures (EC2)DD ENV 1993-1-1: Design of steel structures (EC3)DD ENV 1994-1-1: Design of composite steel and concrete structures (EC4)DD ENV 1995-1-1: Design of timber structures (EC5)DD ENV 1996-1-1: Design of masonry structures (EC6)DD ENV 1997-1-1: Geotechnical design (EC7)DD ENV 1998-1-1: Earthquake resistant design of structures (EC8)DD ENV 1999-1-1: Design of aluminium alloy structures (EC9)Each Eurocode is published in a number of parts, usually with ‘General rules’ and ‘Rules for buildings’ in Part 1. The various parts of EC2 are:Part 1.1 General rules and rules for buildings;Part 1.2 Supplementary rules for structural fire design;Part 1.3 Supplementary rules for precast concrete elements and structures;Part 1.4 Supplementary rules for the use of lightweight aggregate concrete;Part 1.5 Supplementary rules for the use of unbonded and external prestressing tendons;Part 1.6 Supplementary rules for plain or lightly reinforced concrete structures;Part 2.0 Reinforced and prestressed concrete bridges;Part 3.0 Concrete foundations;Part 4.0 Liquid retaining and containment structures.All Eurocodes follow a common editorial style. The codes contain ‘Principles’ and‘Application rules’. Principles are general statements, definitions, requirements and sometimes analytical models. All designs must comply with the Principles, and no alternative is permitted. Application rules are rules commonly adopted in design. They follow the Principles and satisfy their requirements. Alternative rules may be used provided that compliance with the Principles can be demonstrated.Some parameters in Eurocodes are designated by | _ | , commonly referred to as boxed values. The boxed values in the Codes are indicative guidance values. Each member state is required to fix the boxed value applicable within its jurisdiction. Such information would be found in the National Application Document (NAD) which is published as part of each ENV.There are also other purposes for NADs. NAD is meant to provide operational information to enable the ENV to be used. For certain aspects of the design, the ENV may refer to national standards or to CEN standard in preparation or ISO standards. The NAD is meant to provide appropriate guidance including modifications required to maintain compatibility between the documents. Very occasionally the NAD might rewrite particular clauses of the code in the interest of safety or economy. This is however rare.1.3 Scope of the ManualThe range of structures and structural elements covered by the Manual is limited to building structures that do not rely on bending in columns for their resistance to horizontal forces and are also non-sway. This will be found to cover the vast majority of all reinforced and prestressed concrete building structures. In using the Manual the following should be noted:• The Manual has been drafted to comply with ENV 1992-1-1 together with the UK NAD• Although British Standards have been referenced as loading codes in Sections 3 and 6,to comply with the UK NAD, the Manual can be used in conjunction with other loading codes • The structures are braced and non-sway• The concrete is of normal weight• The structure is predominantly in situ• Prestressed concrete members have bonded or unbonded internal tendons• The Manual can be used in conjunction with all commonly used materials in construction; however the data given are limited to the following:– concrete up to characteristic cylinder strength of 50N/mm2 (cube strength 602N/mm)– high-tensile reinforcement with characteristic strength of 4602N/mm– mild-steel reinforcement with characteristic strength of 2502N/mm– prestressing tendons with 7-wire low-relaxation (Class 2) strands• High ductility (Class H) has been assumed for:– all ribbed bars and grade 250 bars, and– ribbed wire welded fabric in wire sizes of 6mm or over• Normal ductility (Class N) has been assumed for plain or indented wire welded fabric.For structures or elements outside this scope EC2 should be used.1.4 Contents of the ManualThe Manual covers the following design stages:• gene ral principles that govern the design of the layout of the structure• initial sizing of members• estimating of quantities of reinforcement and prestressing tendons• final design of members.2 General principlesThis section outlines the general principles that apply to both initial and final design of both reinforced and prestressed concrete building structures, and states the design parameters that govern all design stages.2.1 GeneralOne engineer should be responsible for the overall design, including stability, and should ensure the compatibility of the design and details of parts and components even where some or all of the design and details of those parts and components are not made by the same engineer.The structure should be so arranged that it can transmit dead, wind and imposed loads in a direct manner to the foundations. The general arrangement should ensure a robust and stable structure that will not collapse progressively under the effects of misuse or accidental damage to any one element.The engineer should consider engineer site constraints, buildability2, maintainability and decommissioning.The engineer should take account of his responsibilities as a ‘Designer’ under the Construction (Design & Management) Regulations.32.2 StabilityLateral stability in two orthogonal directions should be provided by a system of strongpoints within the structure so as to produce a braced non-sway structure, in which the columns will not be subject to significant sway moments. Strongpoints can generally be provided by the core walls enclosing the stairs, lifts and service ducts. Additional stiffness can be provided by shear walls formed from a gable end or from some other external or internal subdividing wall. The core and shear walls should preferably be distributed throughout the structure and so arranged that their combined shear centre is located approximately on the line of the resultant in plan of the applied overturning forces. Where this is not possible, the resulting twisting moments must be considered when calculating the load carried by each strongpoint. These walls should generally be of reinforced concrete not less than 180mm thick to facilitate concreting, but they may be of 215mm brickwork or 190mm solid blockwork properly tied and pinned up to the framing for low- to medium-rise buildings.Strongpoints should be effective throughout the full height of the building. If it is essential for strongpoints to be discontinuous at one level, provision must be made to transfer the forces toother vertical components.It is essential that floors be designed to act as horizontal diaphragms, particularly if precast units are used.Where a structure is divided by expansion joints each part should be structurally independent and designed to be stable and robust without relying on the stability of adjacent sections.2.3 RobustnessAll members of the structure should be effectively tied together in the longitudinal, transverse and vertical directions.A well-designed and well-detailed cast-in situ structure will normally satisfy the detailed tying requirements set out in subsection 5.11.Elements whose failure would cause collapse of more than a limited part of the structure adjacent to them should be avoided. Where this is not possible, alternative load paths should be identified or the element in question strengthened.2.4 Movement jointsMovement joints may need to be provided to minimize the effects of movements caused by, for example, shrinkage, temperature variations, creep and settlement.The effectiveness of movement joints depends on their location. Movement joints should divide the structure into a number of individual sections, and should pass through the whole structure above ground level in one plane. The structure should be framed on both sides of the joint. Some examples of positioning movement joints in plan are given in Fig. 2.1.Movement joints may also be required where there is a significant change in the type of foundation or the height of the structure. For reinforced concrete frame structures in UK conditions, movement joints at least 25mm wide should normally be provided at approximately 50m centres both longitudinally and transversely. In the top storey and for open buildings and exposed slabs additional joints should normally be provided to give approximately 25m spacing. Joint spacing in exposed parapets should be approximately 12m.Joints should be incorporated in the finishes and in the cladding at the movement joint locations.2.5 Fire resistance and durabilityFor the required period of fire resistance (prescribed in the Building Regulations), the structure should:• have adequate loadbearing capacity• limit the temperature rise on the far face by sufficient insulation, and• have sufficient integrity to prevent the formation of crack s that will allow the passage of fire and gases.Fig. 2.1 Location of movement jointsThe design should take into account the likely deterioration of the structure and its components in their environment having due regard to the anticipated level of maintenance. The following inter-related factors should be considered:• the required performance criteria• the expected environmental conditions• the composition, properties and performance of materials• the shape of members and detailing• the quality of workmanship• any protective measure• the likely maintenance during the intended life.Concrete of appropriate quality with adequate cover to the reinforcement should be specified. The above requirements for durability and fire resistance may dictate sizes for members greater than those required for structural strength alone.。
毕设英语翻译(英文和译文都有)
Wear 225–229 Ž1999. 354–367Wear of TiC-coated carbide tools in dry turningC.Y .H. Lim ) , S.C. Lim, K.S. LeeDepartment of Mechanical and Production Engineering, National Uni Õersity of Singapore, 10 Kent Ridge Crescent, Singapore 119260, SingaporeAbstractThis paper examines the flank and crater wear characteristics of titanium carbide ŽTiC .-coated cemented carbide tool inserts during dry turning of steel workpieces. A brief review of tool wear mechanisms is presented together with new evidence showing that wear of the TiC layer on both flank and rake faces is dominated by discrete plastic deformation, which causes the coating to be worn through to the underlying carbide substrate when machining at high cutting speeds and feed rates. Wear also occurs as a result of abrasion, as well as cracking and attrition, with the latter leading to the wearing through of the coating on the rake face under low speed conditions. When moderate speeds and feeds are used, the coating remains intact throughout the duration of testing. Wear mechanism maps linking the observed wear mechanisms to machining conditions are presented for the first time. These maps demonstrate clearly that transitions from one dominant wear mechanism to another may be related to variations in measured tool wear rates. Comparisons of the present wear maps with similar maps for uncoated carbide tools show that TiC coatings dramatically expand the range of machining conditions under which acceptable rates of tool wear might be experienced. However, the extent of improvement brought about by the coatings depends strongly on the cutting conditions, with the greatest benefits being seen at higher cutting speeds and feed rates. q 1999 Elsevier Science S.A. All rights reserved.Keywords: Wear mechanisms; Wear maps; TiC coatings; Carbide tools1. IntroductionIn the three decades since the commercial debut of coated cutting tools, these tools have gained such popular- ity that today’s metal cutting industry has come to rely almost exclusively on them. This success stems from the spectacular improvements in tool performance and cutting economies that the coatings are able to bring to traditional high-speed-steel and cemented carbide tools w 1x . At pre- sent, the most common group of coated tools consists of various combinations of titanium nitride ŽTiN ., titanium carbide ŽTiC ., titanium carbonitride ŽTiCN . and aluminium selection of the appropriate tool and machining conditions for a particular application, but also assist engineers andscientists in their development of new tool andcoating materials.This work investigates the wear of coated cementedcarbide tool inserts during dry turning under a wide range ofmachining conditions. Although the current trend is towardsthe use of multilayer coatings, an understanding of the wearcharacteristics of the individual constituent mate- rials wouldbenefit the development of such multilayers. TiC ischosen in this study, since it often forms theimportant base layer in multilayer-coatings due to its lowoxide ŽAl 2 O 3 ., deposited in a multilayer manner onto thermal mismatch with cemented carbide substratesw 5x . cemented carbide substrates. The wear behaviour of coated tools has understandably been the subject of much research but in most instances, the focus appears to be limited to relatively narrow ranges ofmachining conditions Žsee for example, Refs. w 2–4x ..There seems to be a lack of overviews on the wearcharacteristics of different coated tools throughoutthe entire range of their recommended cutting conditions.Such information would not only contribute to a more informedThe flank and crater wear characteristics of TiC-coated tools will be examined, and the methodology of wear maps will be applied to explore the ways in which these tools may be used more effectively.2. Experimental detailsA series of experiments was carried out in accordance with the International Standard ISO 3685-1977 ŽE.test for single-point turning tools w6x. Commerciallyavailable) Corresponding author. Tel.: q65-874-8082; fax: q65-779-1459;e-mail: mpelimc@.sg TiC-coated tool inserts of geometry ISO SNMN 120408 from Sumitomo’s AC720 coated grade were used in these0043-1648r99r$ - see front matter q 1999 Elsevier Science S.A. All rights reserved. PII: S 0 0 4 3 - 1 6 4 8 Ž9 8 .0 0 3 6 6 - 4C.Y.H. Lim et al.r W ear 225–229 (1999) 354–367 355Table 1Tool geometry used in the turning testsBack rake angley68Side rake angley68End clearance angle68Side clearance angle68End cutting edge angle158Side cutting edge angle158Nose radius0.8 mmtests. The cemented carbide substrates belonged to the ISO application group P20–P30 and these had been coated with TiC to an average thickness of 8.5 mm. Knoop microhard- ness indentation testing on the TiC coating with a load of 50 gf indicated a mean hardness of 2678 kg r mm2 . The workpiece material, a hot-rolled medium carbon steel ŽAISI 1045 equivalent.with an average hardness of 89 HRB, was used in its as-received condition. A toolholder of designation ISO CSBNR 2525M12 was employed to achieve the specified cutting geometry. Details of this tool geometry and the test configuration adopted during the turning tests may be found respectively in Table 1 and Fig.23. The chipbreaker, which formed part of the clamping mechanism of the toolholder, was fully wound back during the tests to prevent it from supporting the chip and shorten- ing the contact length.A total of 13 sets of various combinations of cutting speed and feed rate were selected for the tests, with the aim of adequately covering the recommended range of machining conditions for coated carbide tools w7–9x. The choice of these 13 conditions was also influenced in part by the need to explore the wear behaviour under certain machining conditions for which no wear data were avail- able from the open literature. This was to ensure the proper tool wear w10x. A value of 2 mm was chosen, based on the average depth of cut used in the machining tests of other researchers whose data were extracted for the wear maps. No cutting fluid was used in these experiments, as stipu- lated in ISO 3685-1977 ŽE.w6x. Each insert was tested for a total of 20 min or until catastrophic failure, whichever occurred first. The period of 20 min was chosen to limit the amount of work material consumed, while at the same time corresponding to the average tool life of between 10 to 20 min seen in industrial practice.Flank and crater wear were monitored at regular inter- vals throughout the machining experiments. The locations of these wear regions on the tool are shown in Fig. 24. According to ISO 3685-1977 ŽE. w6x, flank and crater wear were measured by the width of the flank wear land, VB, and the depth of the crater, KT, respectively. These mea- surements are illustrated in Fig. 25. It has been shown previously w11x that the rates of flank and crater wear may be more meaningfully portrayed by the dimensionless pa- rameters of VB and KT per unit cutting distance. These quantities are more conveniently represented by log wŽVB or KT.rŽcutting distance.x, and the experimental wear rates from the present tests are given in Table 2.3. Tool wear mechanismsSeveral studies on the mechanisms of flank and crater wear in TiC-coated carbide tools may be found in the open literature Žsee for example, Refs. w4,12,13x., but in each case, the tools were tested under a relatively narrow range of machining conditions. In this work, the worn tools were examined using scanning electron microscopy ŽSEM., after removing adherent work material by immersion in concen-construction of the wear maps later. These conditions are trated hydrochloricacid ŽHCl.. A number of metallo-listed in Table 2. The depth of cut was kept constant since it has been shown that this parameter has little effect ongraphic sections through the centre of thecrater and normal to the cutting edge were also made. TheobservedTable 2Machining conditions and experimental tool wear ratesSet Speed Žm r min.Feed Žmm r rev.Flank wear rate Crater wear rateŽVB.rŽDistance.log 10 ŽŽVB.rŽKT.rŽDistance.log 10 ŽŽKT.rŽDistance..ŽDistance..1 32.3 0.06 9.60 =10y82 207.5 0.06 3.04 =10y83 404.0 0.06 1.57 =10y74 103.9 0.2 3.03 =10y85 186.5 0.2 2.04 =10y86 302.9 0.2 6.09 =10y87 98.3 0.3 3.81 =10y88 193.0 0.3 2.39 =10y89 316.9 0.3 1.12 =10y710 31.7 0.4 9.78 =10y811 349.2 0.4 2.37 =10y712 150.1 0.5 2.33 =10y813 241.0 0.5 4.05 =10y8y7.0 1.32 =10y8y7.5 1.08 =10y9y6.8 5.65 =10y9y7.5 7.22 = 10y 10y7.7 6.79 = 10y 10y7.2 4.73 =10y9y7.4 1.27 =10y9y7.6 1.50 =10y9y7.0 1.17 =10y7y7.0 1.18 =10y8y6.6 5.72 =10y7y7.6 1.88 =10y9y7.4 5.93 =10y9y7.9y9.0y8.2y9.1y9.2y8.3y8.9y8.8y6.9y7.9y6.2y8.7y8.2C.Y.H. Lim et al.r W ear 225–229 (1999) 354–367 356appear plastically deformed in the direction of workpiecerotation ŽFig. 1b.. As cutting continues to the end of the20-min test, a ‘ridge-and-furrow’ topography isformedŽFig. 1c.. This ridge-and-furrow appearance has previouslybeen reported w4,13,14x, and the mechanismresponsibleFig. 1. Topography of flank face Ža.when new, Žb.after 3 mins, and Žc.after 20 mins of cutting at 103.9 m r min and 0.2 mm r rev,showing original dimple-like surface features being worn bydiscrete plastic deformation to give a ridge-and-furrow appearance.wear mechanisms are discussed below, with an attempt tocorrelate the current findings with published reportsin order to present a better picture of tool wear across a widerrange of cutting conditions.3.1. Flank wear mechanismsThe unworn TiC coating on the flank face of the newtool shown in Fig. 1a exhibits ‘dimple-like’ surface fea-tures. After 3 min of machining, however, these featuresC.Y.H. Lim et al.r W ear 225–229 (1999) 354–367357was termed ‘discrete plastic deformation’ since the depth ofthe gradual thinning of the coating worn by discrete plastic deforma- tion. deformation is limited to 1 mm or less of the coating surfacew4x, as seen in Fig. 1c.It has been demonstrated that during machining, highcompressive stresses and intimate contact between theatomically clean surfaces of the newly-machined work- pieceand the flank face of TiC-coated carbide tools results inseizure over much of the tool–work contact area w15x. ŽItshould be pointed out that the term ‘seizure’used in themachining context differs somewhat from the usual tribo-logical understanding in which the real and nominal con- tactareas are equal..However, cutting is able to continue as thework material moves by shear in the layers of the workadjacent to this interface w16x. Such conditions gener- ate highshear stresses on the tool surface that plastically deform and‘smear’ the original dimple-like features of the coating in thedirection of workpiece rotation. With time, these deformeddimples flow and merge into the ridge- and-furrowtopography shown in Fig. 1c. It has been proposed that thisprocess culminates in the ductile frac- ture of tiny fragmentsof the coating, which are then swept away by the passingwork w4x.Discrete plastic deformation gradually reduces thethickness of the TiC coating during machining. The cross-sectional view of the flank wear land in Fig. 2 shows a‘depression’ in the coating worn by discrete plastic defor-mation. As wear progresses, localized areas of the underly-ing carbide substrate become exposed ŽFig. 3a., and even-tually merge into a continuous band of exposed substrate ŽFig.3b., an observation shared by several other workers w4,17–20x.Fig. 2. Section through flank wear land after 20 min of cutting at 150.1m r min and 0.5 mm r rev, showing a ‘depression’ in the TiC coating due toC.Y.H. Lim et al.r W ear 225–229 (1999) 354–367 358Fig. 3. Flank wear land showing coating removal by discrete plastic deformation, Ža.beginning with the appearance of holes and voids, and Žb. followed by the merging of voids to form a continuous band of exposed substrate. deformation around the tool edge w22x. This may contribute to cracking due to the inability of the brittle TiC layer to conform to this deformation. Although cracking of the coating does not directly result in flank wear, the cracks compromise the integrity of the coating and may become preferential sites for coating removal. Fig. 4 shows an example of how tiny fragments of the coating have been ‘ plucked out’ in the vicinity of cracks. This could acceler- ate coating wear and hasten the exposure of the substrate.The severity of cracking and attrition appears to depend on the cutting speed and feed rate. At speeds below 40 m r min, no signs of cracking or attrition are seen. At moderate speeds and feeds, a few fine cracks are visible, but attrition is not evident in most cases. Cracks become more abundant at high speeds and feeds, accompanied by slight attrition of the coating. These observations lend support to the earlier suggestion that cracking is related to the compressive deformation of the tool edge. Higher cutting speeds increase tool temperatures, thus causing greater softening of the tool nose, while a higher feed imposes higher compressive stresses on the tool edge. Both factors result in greater deformation of the tool nose, which increases the extent of cracking. However, under the conditions of the present tests, cracking and attrition of the TiC coating on the flank does not appear to play a dominant role in tool wear. There is also no evidence to support the suggestion that hard coating fragments re- moved via the attrition process contribute significantly to wear by abrasion w20x.Flank wear of TiC-coated carbide tools has frequently been attributed to the dominance of abrasive wear Žsee for example, Refs. w12,14,20,23x.. It seems unlikelythoughRidge-and-furrow wear surfaces are seen under all the conditions used in the present tests, but they appear more pronounced at higher cutting speeds and feed rates. It has been shown that increases in speed and feed lead to a rise in temperatures at the tool flank w21x. This causes the TiC layer to soften w22x, rendering it more susceptible to plastic deformation. In tools tested at high speeds and feeds, the coating is worn away rapidly by discrete plastic deforma- tion in a matter of minutes, exposing the carbide substrate beneath.Other features observed on the worn tool flanks are fine cracks parallel to the tool edge and perpendicular to the direction of workpiece rotation ŽFig. 4.. These are found on all tools except those tested at very low cutting speeds Žless than 40 m r min.. Careful examination of new tools shows that such cracks are not present prior to testing. Furthermore, the cracks are confined to the flank wear land, the only region that is in contact with the workpiece during machining. These findings suggest that the cutting process is responsible for the formation of these cracks. It is believed that the high shear stresses that cause discrete plastic deformation, also lead to cracking within theC.Y.H. Lim et al.r W ear 225–229 (1999) 354–367359TiC coating. In addition, the high compressive loads imposed on the tool edge during cutting are known to cause bulk that abrasion would be a major mechanism of coating wear since the TiC coating has a hardness equal to that of hard inclusions in the workpiece w22x. There is also little evi- dence of deep abrasion grooves on the coating, which might indicate the dominance of abrasive wear. At low magnifications, the ridge-and-furrow topography of dis-Fig. 4. Flank wear land showing fine cracks in the TiC coating, and the attrition of coating particles in the vicinity of the cracks when cutting at 316.9 m r min and 0.3 mm r rev.C.Y.H. Lim et al.r W ear 225–229 (1999) 354–367 360crete plastic deformation could perhaps be mistaken for evidence of abrasion and it is usually only upon closer examination that the difference between the two mecha- nisms may be discerned.On some tools, however, there appear to be faint grooves scratched on the surface of the coating. The tool shown in Fig. 5 has been tested at a very low cutting speed and feed rate. The worn surface appears smooth with numerous shallow but sharp grooves. These grooves do not resemble the coarser ridge-and-furrow features of discrete plastic deformation. Even on surfaces that do exhibit ridge-and- furrow formation, such as the one in Fig. 6, faint sharp lines may also be seen on top of the ridges. It is possible that these grooves are the result of abrasion by favourably-oriented inclusions in the workpiece thatareFig. 6. Flank wear land after 20 min of cutting at 207.5 m r min and 0.06 able to plough into the TiC coating w 24x . Abrasion is mm r rev, showing sharp, shallow abrasion grooves on top of ridge-and- probably significant only at lower speeds and feeds, where discrete plastic deformation involves very small strains and the wear rate is low w 4x . At higher speeds and feeds, the effect that such abrasion has on the overall wear of the coating on the flank face is likely to be small since the grooves are very shallow, especially when compared with the depth of the furrows formed by discrete plastic defor- mation. TiC has been found to be the most resistant tofurrow surface. in Fig. 7. The growth in the exposed areas of substrate through such a chipping mechanism has also been reported elsewhere w 12,25x . Examination of the tools in their as-machined condition show that regions where the substrate have become ex- abrasion among the common coating materialsŽsee for posed are completely covered by work material, which example, Refs. w 13,14,20x ., so it is hardly surprising that abrasion is not a major wear mechanism in the present investigation.For most of the tools, the TiC coatings remained intact throughout the entire duration of the experiment. Only a few tools tested under high speed or high feed conditions suffered coating loss through discrete plastic deformation and attrition. Once an area of substrate has been exposed, the passing work ‘impinges’ on the lower border of the coating adjacent to these exposed areas of substrate, and slowly chips away at the coating, causing the flank wear land to grow downwards. This process leaves a very uneven border at the bottom of the flank wear land, as seenFig. 5. Flank wear land after 20 min of cutting at 32.3 m r min and 0.06 mm r rev, showing sharp, shallow grooves due to abrasion.C.Y.H. Lim et al.r W ear 225–229 (1999) 354–367361may be removed only by dissolving in acid. This suggeststhat there has been intimate contact between the workmaterial and the exposed carbide substrate during machin-ing. Removal of the adherent work material reveals a smoothtopography with ridges and grooves on some of thecarbide grains ŽFig. 8.. This feature has been com- monlyassociated with diffusion wear in cemented carbide toolsw22,26x. However, it is also likely that the discrete plasticdeformation mechanism observed on the TiC coat- ing,continues to wear away the substrate as well. Such a processcould also contribute to the smoothly worn appear- ance.Fig. 7. Flank wear land after 20 min of cutting at 207.5 m r min and 0.06mm r rev, showing the uneven border between the TiC coating and theexposed carbide substrate as a result of chipping of the coating.C.Y.H. Lim et al.r W ear 225–229 (1999) 354–367 362features on the coating have been deformed in the chip flowdirection. These observations suggest that discrete plasticdeformation is also a dominant mechanism in coat- ing wearon the rake face. Similar to the case of flank wear earlier,discrete plastic deformation on the rake face be- comes morepronounced as cutting speed and feed rate are raised. Thesefindings agree with a previous suggestion that theseverity of this wear process is governed by temperature andshear stress, which rise with increasingspeed and feed w4x. The coating on the rakeface isFig. 8. Flank wear land after 2 min of cutting at 404 m r min and 0.06mm r rev, showing a smooth topography, as well as carbide grains withrandomly-oriented ridges and grooves.3.2. Crater wear mechanismsThe ridge-and-furrow topography associated with dis-crete plastic deformation on the flank face is also seen on therake face. In the comparison of a new and a worn tool inFig. 9, it is apparent that the originaldimple-likeFig. 9. Topography of rake face Ža. when new, and Žb. after 3 min of cuttingat 302.9 m r min, 0.2 mm r rev, showing original dimple-likesurface features being worn by discrete plastic deformation togive a ridge-and-furrow appearance.C.Y.H. Lim et al.r W ear 225–229 (1999) 354–367363gradually worn to expose the carbide substrate in a mannersimilar to that seen on the flank face.The micrograph in Fig. 10 shows extensive cracking onthe rake face parallel to the cutting edge and perpendicular tothe chip flow direction. In the vicinity of these cracks, tinypieces of the TiC coating have been plucked out, leavingjagged uneven edges. The cracks here resemble the cracksseen on the flank face. It is believed that in moving the chipover the tool under a condition of seizure w15x, the high-shearstresses generated on the tool surface causes the coating tofracture. Unlike the case of flank wear in which cracking ismore severe at higher speeds and feeds, this phenomenon isobserved on the rake face only at low cuttingspeeds. This may be explained by considering the differenttemperatures experienced on the rake face at low speeds andat high speeds. At low speeds, the temperature during cuttingis lower and the hardness of the TiC coating remainsrelatively high; the coating is therefore likely to be brittle andmore susceptible to cracking. With rising speed, the highertemperatures cause the hardness of the TiC coating to dropw22x, and the coating becomes more duc- tile. Consequently,the shear stresses imposed on the coat- ing surface result indiscrete plastic deformation of the coating rather thancracking.The cracking of the TiC coating on the rake facefacilitates removal of the coating by attrition. The view of therake face at a low magnification in Fig. 11 shows large areaswhere the coating has been removed by such anFig. 10. Rake face after cutting for 20 min at 32.3 m r min and 0.06 mm r rev,showing cracks parallel to the cutting edge and perpendicular to chip flowdirection.C.Y.H. Lim et al.r W ear 225–229 (1999) 354–367364Fig. 11. Rake face after cutting for 20 min at 32.3 m r min and 0.06 mm r rev, showing large areas where the TiC coating has been removed via cracking and attrition.attrition process. The extensive attrition at low speeds and feeds, could also be caused by a less laminar and more intermittent flow of the chip over the rake face. Sudden and local tensile stresses imposed by the unevenly flowing work material would tear away fragments of the coating. Uneven flow of the chip is associated with the occurrenceFig. 13. Crater bottom after 30 s of cutting at 241 m r min and 0.5 mm r rev, showing a smooth topography, as well as carbide grains with randomly-oriented ridges and grooves.The TiC coating is worn away fairly rapidly at high speeds and feeds via discrete plastic deformation. When this happens, the crater quickly fills with work material. Dissolving the adherent work or examining the tool in cross-section reveals a deep crater. Careful study of the crater in cross-section shows the occasional protrudingof a built-up-edge ŽBUE .. Although no adherent BUE was carbide at the tool –work interface ŽFig. 12.. This is afound on any tool after cutting, this does not rule out its occurrence during machining. An earlier report described the formation of a BUE when machining at speeds be- tween 15 m r min and 40 m r min w 27x . The same study also noted that the adhesion between the BUE and the coated tool edge is very weak, and the BUE is readily loosened during metallographic preparations. Here, a comparison of the surface finish of the workpiece after machining at two different speeds Ž32.3 m r min and 103.9 m r min . reveals that the workpiece is a lot rougher at the lower speed: 6.35 characteristic often associated with diffusion wear of car- bide tools, in which it is believed that carbides that are less soluble in the work material are left protruding while the surrounding tool material is worn away at a faster rate w 26x . However, the discrete plastic deformation mechanism could also account for such features, since the softer matrix would be deformed to a greater extent and worn away more quickly than the harder carbides. Removing the work material in the crater with acid reveals a smooth topogra- phy with carbide grains that have randomly-orientedmm Ra as compared to 2.46 mm Ra. A poor surface finish grooves on their surfaces ŽFig. 13.. This appearance ishas been found to be a good indication of the formation of BUE during machining w 22x .very similar to that of the flank wear land shown in Fig. 8.4. Wear maps for TiC-coated carbide toolsWear maps are useful tools for presenting the overall behaviour of wearing systems in a more meaningful andcomplete fashion w 28–30x . Research on metalsC.Y.H. Lim et al.r W ear 225–229 (1999) 354–367365 w31–34x,Fig. 12. Section through crater after cutting for 30 s at 307.8 m r min and 0.6 mm r rev, showing protruding carbide grains at the tool–work inter- face. ceramics Žsee for example, Ref. w35x., and some cutting tools w36–38x has shown that such maps facilitate the study and understanding of the relationships between measured wear rates and the dominant wear mechanisms over a wide range of operating conditions. The wear-map approach is adopted in this work to examine the wear characteristics of the TiC-coated carbide tools.The construction of a wear map first requires the exten- sive gathering of wear data from the technical literature for the particular wear system of interest. In this case, infor- mation relating to flank and crater wear of TiC-coated carbide tools during dry turning of steel workpieces wasC.Y.H. Lim et al.r W ear 225–229 (1999) 354–367366Fig. 14. Map for flank wear of TiC-coated carbide inserts during dry turning. The regions where the different ranges of wear rates are observed are shaded accordingly. The safety zone is the region where flank wear rates are the lowest; the considerably larger least-wear regime is also indicated.collected. The axes of the map are then decided: usually two Žsometimes three . operating parameters of the system zone Ž) y 6.9.. On the crater wear map, the wear rates span a wider range than in the case of flank wear, and are selected to form a plane Žor space . within whichthese are divided into five regimes: the safety zone Ž- empirical wear data are presented. Here, the same axes as y 8.5., a least-wear zone Žy 8.0 to y 8.4., and three other those employed previouslyw 11,36 –38x , namely,cuttinghigher-wear regions Ž) y 7.9..speed Žin m r min . and feed rate Žin mm r rev ., are used. 4.1. Wear-rate mapsMaps showing the flank and crater wear rates of TiC- coated carbide tools are given in Figs. 14 and 15. These maps, which have been introduced earlier w 38x , are based on the results of the present cutting tests, together with similar data from 35 other sources. The boundaries on the maps define different regions within which wear rates of similar ranges of values are contained. Three major wear regions are demarcated on the flank wear map; these are: the safety zone Ždimensionless wear rates - y 7.5., the moderate-wear region Žy 7.0 to y 7.4., and the high-wearThe boundaries on the wear maps reflect the influence of cutting speed and feed rate on the wear of the inserts. Under high speed –high feed conditions, the protective TiC coating is worn away quickly to expose the substrate, which has a much lower wear resistance, and thus gives rise to the higher wear rates seen. The increased wear rate at the low speeds is probably due to the presence of a BUE occurring at those speeds. Information on the wear of TiC-coated tools in the speed range of BUE formation is scanty since such low speeds are not within the normal machining range of these tools. It is believed however, that the BUE that forms on TiC-coated inserts during low-speed machining is very unstable w 27x . The unstable nature of the BUE causes it to break off and reform over and over again,。
- 1、下载文档前请自行甄别文档内容的完整性,平台不提供额外的编辑、内容补充、找答案等附加服务。
- 2、"仅部分预览"的文档,不可在线预览部分如存在完整性等问题,可反馈申请退款(可完整预览的文档不适用该条件!)。
- 3、如文档侵犯您的权益,请联系客服反馈,我们会尽快为您处理(人工客服工作时间:9:00-18:30)。
72页Machine Tools Objectived.Machine tools are the main engines of the manufacturing industry. This chapter covers a few of the details that are common to all classes of machine tools discussed in this book. After completing the chapter, the reader will be able to>understand the classification of the various machine tools used in manufacturing industries.>identify the differences between generating and forming of surfaces. > identify various methods used to generate different types of surfaces. >distinguish between the different accuracies and surface finishes that are achievable with different machine tools.>understand the different components of the machine tools and their functions.>learn about the different support structures used in the machine tools. >understand the various actuation systems that are useful to generate the required surfaces.>Learn the different types of guideways used in the machine tools.>understand the work holding requirements.3.1 INTRODUCTIONThe earliest known machine tools are the Egyptian foot-operated lathes.These machine tools were developed essentially to allow for the introduction of accuracy in manufacturing.A machine tool is defined as one which while holding the cutting tools, would be able to remove metal from a workpiece in order to generate the requisite job of given size, configuration, and finish. It is different from a machine, which is essentially a means of converting the source of power from one form to the other. The machine tools are the mother machines since without them, no components can be produced in their finished form. They are very old and the industrial revolution owes its success to them.A machine tool is required to provide support to the workpiece and cutting tools as well as provide motion to one or both of them in order to generate the required shape on the workpiece. The form generated depends upon the type of machine tool used.In the last two centuries, the machine tools have been developed substantially. The machine tool versatility has grown to cater to the varied needs Of the new inventors coming with major developments. For example,James Watt's steam engine could be proven only after a satisfactory method was found to bore the engine cylinder with a boring bar by Wilkinson around 1775.A machine tool is designed to perform certain primaryfunctions,but the extent to which it can be exploited to perform secondary functions is a measure of its flexi bility.Generally,the flexibility of the machine tool is inc reased by the use of secondary functional attachments,s uch as radius or spherical turning attachment for a cent re lathe.Alternatively,to improve productivity,special atta chments are added,which also reduce the flexibility.3.2CLASSIFICATION OF MACHINE TOOLSThere are many ways in which the machine tools can be classified.One such classification based on the produc tion capability and application is shown below:1.General purpose machine tools(GPM)are those designed to perform a variety of machining operations on a wide range of components.By the very nature of generalisation,the general purpose machine tools are thou gh capable of carrying out a variety of tasks,would no t be suitable for large production,since the setting time for any given operation is large.Thus,the idle time on the general purpose machine tools is more and the mac hine utilisation is poor.The machine utilisation may be termed as the percentage of actual machining or chip g enerating time to the actual time available.This is much lower for the general purpose machine tools.They m ay also be termed as the basic machine tools. Further,skilled operators would be required to run the general purpose machine tools.Hence,their utility is in job shops,such as catering to small batch and large v ariety job production,where the requirement is versatility rather than production capability.Examples are lathe,shaper,and milling machine.2Production machine tools are those where a number of functions of the machine tools are automated such t hat the operator skill required to produce the component is reduced.Also,this would help in reducing the idle t ime of the machine tool,thus improving the machine ut ilisation.It is also possible that a general purpose machi ne tool may be converted into a production machine to ol by the utilisation of jigs and fixtures for holding the workpiece.These have been developed from the basic m achine tools.Some examples are capstan lathes,turret la thes,automats,and multiple spindle drilling ma chines. The setting time for a given job is more.Also,tooling design for a given job is more time consuming and ex pensive.Hence the production machine tools can only beused for large volume production.3.Special purpose machine tools(SPM)are those mac hine tools in which the setting operation for the job a nd tools is practically eliminated and complete automationi s achieved.ms greatly reduces the actual manufacturing t ime of a component and helps in the reduction of cos ts.These tools are used for mass manufacturing.These machine tools are expensive compared to the general pur pose machines since they are specifically designed for the given application,and are restrictive in their application c apabilities.Examples are cam shaft grinding machine,conn ecting rod twin boring machine,and piston turning lathe.4.Single purpose machine tools are those,which are designed specifically for doing a single operation ona class of jobs or on a single job.These tools ha ve thehighest amount of automation and are used for really high rates of production.These are used specifically for one product only,and thus have the least flexibili ty.However,these do not require any manual interven tion andare most cost effective.Examples are transfer linescomposed of unit heads for completely machining any given product.The application of the above four types can be shown graphically in Fig. 3.1.Fig. 3.1Application of machine tools based on the capability. 3.3GENERATING AND FORMINGGenerally,the component shape is produced in machine tools by two different techniques,generating and forming. Generating is the technique in which the required pr ofile is obtained by manipulating the relative motionsof the workpiece and the cutting tool edge.Thus,the obtained contour would not be identical to the shape of the cutting tool edge.This is generally used for a majority of the general profiles required.The type of surface generated depends on the primary motion ofthe workpiece as well as the secondary or feed motio n of the cutting tool.For example,when the workpiece is rotated and a single point tool is moved along a straight line paralle l to the axis ofrotation of the workpiece,a helical s urface is generated,as shown in Fig. 3.2(a).If the pitch of the helix or feed rate is extremely small,or the surface generated may be approximated to a cylin der.This is carried out in ladles and is called turning or cylindrical turning.Fig. 3.2Generating and forming of surfaces by machine tools.An alternate method of obtaining the given profile is called forming in which,the shape of the cutting toolis impressed upon the workpiece,as shown in fig. 3.2 (b).Thus,the accuracy Of the obtained shape dependupon the accuracy of the form of the tool used.However,many of the machine tool operations areactually combinations of the above two.For example. when a dove tail is cut,the actual profile is obtained by sweeping the angular cutter along the straight line. Thus,it involves forming(angular cutter profile)and gene rating(sweeping along a line),as shown in Fig. 3.3.Fig3.3Generation of surface.3.4METHODS OF GENERATING SURFACESFig. 3.4Classification of machine tools using single point cuttingtools.A large number of surfaces can be generated or formed with the help of the motions given to the tooland the workpiece.The shape of the tool also makesa very important contribution to the final surface obtaine d Basically,there are two types of motions given in a machine tool.The primary motion given to the workpiece or cutting tool constitutes the cutting speed,which cause s a relative motion between the tool and workpiece suc h that the face of the cutting tool approaches the mat erial to be ually,the primary motion consum es most of the cutting power.The secondary motion is one which feeds the tool relatively past the workpiece. The combination of the primary and secondary motions is responsible for the generation of specific surfaces.Someti mes,there would be a tertiary movement in between thecuts for specific surfaces.A classification of machine tools based on the motions is shown in Fig. 3.4,for single point tools,an d Fig. 3.5for multi-point tools.In the case of job rot ation,cylindrical surfaces would be generated,as shown i n Fig. 3.6,when a tool is fed in a direction parallelto the axis of rotation.When the feeding direction is not parallel to the axis of rotation,complex surfaces, such as cones(Fig. 3.7),or contours(Fig. 3.8)can begenerated.The tools used in the above cases are of si ngle point.If the tool motion is perpendicular to the a xis of rotation,a plane surface would be generated,as shown in Fig. 3.9.However,if a cutting tool of a giv en form is fed in a direction perpendicular to the axis of rotation,also called plunge cutting,a contour surface of revolution would be obtained,as shown in Fig. 3.10.Fig. 3.5Classification of machine tools using multi-point cutting tools. Plane surface generation in shaping Plane surfaces can be generated when the job or tool reciprocates for the primary motion,as shown in Fig. 3.11,without any rota tion.With the multi-point tools generally plane surfaces aregene rated,as shown in Fig. 3.12.However,in this situation, a combination of forming and generating,is used to get a variety of complex surfaces,which are otherwise i mpossible to get through the single-point tool operations. Some typical examples are the spur gear hobbing and spiral milling of formed cavities.3.5ACCURACY AND FINISH ACHIEVABLEIt is necessary to select a given machine tool or m chining operation for a job such that it is the lowest cost option.There are various operations possible for a given type of surface and each one has its own charac teristics in terms of possible accuracy,surface finish,and cost.This selection is made at the time of process pla nning.The obtainable accuracy for various types of machi ne tools is shown in Table 3.1.The surface finish expe cted from the various processes is shown in Fig. 3.13.The values presented in Table 3.1and Fig. 3.13areonly a rough guide.The actual values greatly vary depe nding on the condition of the machine tool,the cutting tool used,and the various cutting process parameters.80Manufacturing TechnologyBASIC ELEMENTS OF MACHINE TOOLS3.6 BASIC ELEMENTS OF MACHINE TOOLSThe various components that are present in all the mac hine tools may be identified as follows:•Work holding device to hold the workpiece in the correct orientation to achieve the required in manufacturin g,for example chuck.•Tool holding device to hold the cutting tool in the correct position with respect to the workpiece,and provi de enough holding force to counteract the cutting forces acting on the tool,example tool•Work motion mechanism to provide the necessary sp eed to the workpiece for generating the surface,example head stock.•Tool motion mechanism to provide the various motio ns needed for the tool in conjunction with workpiece m otion in order to generate the required surface profiles, example carriage.•Support structure to support all the mechanisms sho wn above,and maintain their relative position with respe ct to each other,and allow for relative movement betw een the various parts to obtain the*requisite part pr ofile and accuracy,example bed.The type of device or mechanism used varies depending on the type of machine tool and the function it is expected to serve.In this chapter,someof the more common elements would be discussed.How ever,further details may be found in the chapters wher e the actual machine tools are discussed.The various motions that need to be provided in the machine tool are cutting speed and feed.The range of speed and feed rates to be provided in a given machi ne tool depends on the capability of the machine tool and the range of work materials that are expected to be processed.Basically,the actual speed and feed chosen depends upon the•work material,•required production rate,•required surface finish,and•expected accuracy.The drive units in a machine tool are expected to provide the required speed and convert the rotational sp eed into linear motion.Details of these may be foundin books dealing with machine tool design.3.7 SUPPORT STRUCTURESThe broad categories of support structures found in vario us machine tools are shown in Fig. 3.14.They may be classified as beds(horizontal structures)or columns(vertic al structures).The main requirements of the support structure are •Rigidity•Accuracy of guideways•Impact resistance•Wear resistanceBed provides a support for all the elements presentin a machine tool.It also provides the true relative po sitions Of all units in machine tools.Some of these un its may be sliding on the bed or fixed.For the purpo se Of sliding,accurate guideways are provided.Bed weig ht is approximately half the total weight of the machine tool.The basic construction of a bed is like a box,to provide the highest possible rigidity with low weight.To increase the rigidity,the basic box structure is added wi th various types of ribs,as shown in Fig. 3.15.The a ddition of ribs complicates the manufacturing process for the beds.Beds are generally constructed using cast iron or alloy c ast iron consisting of alloying elements,such as nickel,c hromium,and molybdenum.With cast iron,because of t he intricate designs of the beds,the casting defects may not be fully eliminated.Alloy steel structure is also used for making beds. The predominant manufacturing method used is welding.T he following advantages can be claimed for steel constru ction:(a)With steels,the wall thickness can be reduced .Thus,greater strength and stiffness for the same weight would be possible with alloy steel bed construction.(b)Walls of different thicknesses can be conveniently welded.Whereas in casting,this would create problems.(c)Repair of welded structures would be easier.(d)Large machining allowances would have to be provi ded for casting to remove the defects and hard Concrete is also tried as bed material.Its choice is ma inly because of the large damping capacity.For precision machine tools and measuring machines,granite is also us ed as the bed material.The major types of bed styles used in the machine tools are shown in Fig. 3.16.。