Directional CdS nanowires fabricated by chemical bath deposition
CdS纳米带的合成及其自组装机理

s e mb l y . Th e n a n o r i b b o n s a r e u s u a l l y 5 0 -4 0 0 n r n wi d e ,1 O 一2 0 D i n t h i c k,a n d s e v e r a l t o t e n s o f mi c r o me t e r s l o n g .Th e a t o mi c
TEM ,F T— I R ,EDS,Z e t a p o t e n t i a l a n d f l u o r e s c e n c e s p e c t r a a n d t h e s e l ba s s e mb l y me c h a n i s m wa s d i s c u s s e d .Th e r e s u l t s s h o we d
i n t h e i n i t i a l Cd Te .I n a d d i t i o n,s t r o n g d i p o l e - d i p o l e i n t e r a c t i o n s a r e b e l i e v e d t O b e t h e ma i n d r i v i n g f o r c e o f n a n o p a r t i c l e s e l  ̄a s —
强烈的偶极一偶极作用力是纳 米粒子 自组装 的主要驱 动力 。纳米 带的宽度 为 5 0  ̄4 0 0 n l n, 厚度 为 1 0  ̄2 0 n m, 长度 为几微米到 几十微米。终产物 中 C d 、 S元 素的原子百分 比接近 1: 1 , 而T e的含 量几乎为 0 , 说明T e 2 一 基 本上被 S z 一所取代。
表面功能化CdS纳米微粒的制备

二 水 合 乙酸镉 , 基 乙醇 ( ) 硫脲 和 N, 巯 ME , N一 二 甲基 甲酰胺 ( DMF) 都是分 析 纯试 剂 , 用前 没 有 使
进 一步 纯化 。 一氨基 一1 1 一邻 菲 罗啉 ( h n 按 5 ,0 Ap e )
如D MF和二 甲亚砜( MS 中有很好的分散性 。 D O)
的C S纳米 微粒 , d 通过 I 测试 显示 , p e R A h n接枝 在C S纳 米微粒 的表 面 。 d 功能 化 后的C S纳 米微 粒 呈现 d
单分 散分 布 ,X RD谱 图表 明功 能化 的 AP e - C S微粒 和纯 的 C S纳米 微 粒相 比较 , 粒仍 然 是立 方 hn d d 微 晶的结 构且 粒径 没有发 生 改变仍 保持 3 n —5 m。 关键词 : h n C S纳 米微 粒 ; Ap e ; d 配体交 换 法 : 功能 化 的
Ma n 6 T—I 红 外光 谱仪 ; ime s g a5 0F R Se n D一 5 0 0 5X一射线 衍射 仪 C K(( 一1 5 1 7 ; u ( . 4 8 ) 1 2 原料Fra bibliotek和试 剂 .
次, 乙醚 洗涤 1 , 空 室温 干燥 , 后得 到 2 9 次 真 最 . g黄 色粉 末 。得到 的 Ap e -C S纳米微 粒 在极 性 溶剂 hn d
I mm u fu e c ntl b lng o a c rm a ke no l or s e a e i fc n e r r
He 2 a d o h rc l l rt r e s wih s mio — r n t e e l a a g t t e c n u
2 结果与 讨论
2T ea ̄ re h t ( e)
◆ Nanostructures in GaAs Fabricated by Molecular Beam Epitaxy

NNanostructures in GaAs Fabricated by Molecular Beam EpitaxyLoren N. Pfeiffer, Kenneth W. West, Robert L. Willett,Hidefumi Akiyama, and Leonid P . RokhinsonWe review three novel techniques whereby the highly uniform two-dimensional films produced by molecular beam epitaxy (MBE) can be further patterned into nanowires or nanostructures having quantum confinement in all three dimensions. These techniques have the potential of greatly extending the power and utility of the MBE method. © 2005 Lucent Technologies Inc.for fabricating nanostructures from GaAs-AlGaAs MBE films that we have been recently exploring at Bell Labs.Fabrication of Nanostructures in GaAs Defined by Scanned Local Oxidation on the Surface of a Very Shallow 2D Electron or Hole SystemIn these nanostructures,the 2D carriers are de-pleted only under the locally oxidized areas of the scanned tip [3].This divides the system into several conducting 2D regions separated by insulating walls.The particular nanostructure we fabricated using this technique is the first nanodevice that is able to sort ballistic holes into their separate spin-up and spin-down components [6].This capability of sorting carriers by their spin alignment in a magnetic field is prerequisite to making the proposed new field of spin-electronics or spintronics a reality.We use the fact that,in a AlGaAs-GaAs semiconductor hetero-strucure,the spin-up and spin-down holes at the Fermi energy have,in a magnetic field,slightly dif-fering cyclotron orbits because of their differing spin-orbit interactions.This spin-separation experiment requires a host of novel nanostructure techniquesIntroductionMolecular beam epitaxy (MBE)is essentially a controlled evaporation from various elemental sources in high vacuum onto a temperature-controlled single crystal substrate.The idea of epitaxy is that in the growing layer the newly arriving atoms incorporate at the precise positions established by the immediately preceding atomic layer.This epitaxial relationship extends even to a hetero-interface where a semi-conducting GaAs layer abruptly changes to the higher bandgap more insulating material,AlAs,or to the alloy AlGaAs.Because GaAs,AlAs,and AlGaAs have sufficiently similar crystal structures to one another,MBE can produce single crystal layers of uniform and precisely controlled nano-thickness with no mis-matched chemical bonds at the hetero-interfaces.In some sense these nano-thick epitaxial films are indeed ideal two-dimensional (2D)nanostructures;however,in the remainder of this paper,we will use the word nanostructure to mean structures that have dimensions smaller than 100nm in at least two right-angle directions.Thus,all M BE films will require fur-ther processing of some kind if they are to be fabricated into nanostructures as the word is intended here.In this paper,we highlight,three of the various methodsBell Labs Technical Journal 10(3), 151–159 (2005) © 2005 Lucent Technologies Inc. Published by Wiley Periodicals,Inc. Published online in Wiley InterScience ().• DOI: 10.1002/bltj.20110because the spin-up to spin-down orbit separations typically translate to only about100nm of lateral displacement.As a first step in the nanofabrication, we designed a conceptually novel MBE quantum well structure for 2D holes that is modulation-doped from below and surface-compensated from above [7]. This allows us to reduce the quantum-well-to-sample-surface dis-tance to only 350 A, while maintaining an undoped modulation-doping setback in excess of 500 A frombelow. The valance band energy diagram versus depth into the AlGaAs-GaAs sample is shown in Figure 1.The symbol E f is the Fermi level, and the vertical axis is depth within the sample referenced to the 150Å wide GaAs quantum well located 350 Å below the sample surface. These shallow 2D quantum well structures have achieved 2D hole mobilities of 600,000 cm2/Vsec at various predetermined hole den-sities, which typically are 1.4 ϫ1011cmϪ2.The interesting thing about this structure is the extreme sensitivity of the 2D hole density to the thick-ness of the 150 Å GaAs layer between the upper Si d-doping and the sample surface. The left side of Figure1 shows the band diagram of the structure after removing 70 Å of the surface GaAs layer by GaAs con-version to gallium oxide. This small thickness change in the GaAs cap layer completely shuts off all valance band conduction in the sample by lifting the bottom of the quantum well above the Fermi level. We make use of this extreme sensitivity by installing the sample in an atomic force microscope (AFM), where a micro-tip under voltage bias is scanned in a humid atmos-phere just above the sample surface. Wherever the tip is scanned, the surface becomes locally oxidized in a strip 300 Å wide and about 100 Å deep. This effectively moves the surface states 100 Å closer to the quantum well in the strip-oxidized region, which is enough to locally lift the quantum well above the Fermi level of the 2D hole gas (HG) and cause the well to fully deplete everywhere the oxida-tion probe was scanned.The surface oxidation walls below which the 2D HG is depleted are shown in the 5 m m ϫ5 m m inset of Figure 2, where region 1 is the injector, the white (top) Panel 1.Abbreviations,Acronyms,and Terms1D—One-dimensional2D—Two-dimensionalAFM—Atomic force microscopeHG—Hole gasMBE—Molecular beam epitaxyPMMA—Polymethyl methacrylateSEM—Scanning electron micrographTEM—Transmission electron micrographFigure 1.Poisson-Schrodinger calculations of the valence band energy diagram of very shallow quantum well sample before and after 7 nm of surface oxidation.152Bell Labs Technical Journaland gray (bottom) curves are the trajectories of the two spins in a perpendicular magnetic field, and V3–V4 is the detector voltage plotted in the main figure. The magnetic focusing doublet at Bൿϭ0.18T (Figure 3) shows a separation of 36 mT. This corresponds to a measured spin up-to-down orbit separation of only 120nm. A separate experiment using an additional 3.3T in-plane B-field caused the higher peak in the doublet to disappear, demonstrating preferential selec-tion for spin-down 2D holes [6].The scanned-oxide fabrication techniques em-ployed here are very general. Because they allow any shallow 2D conducting quantum layer to be divided into various nanoscale conducting regions separated by highly insulating quantum barriers, they raise exciting possibilities for applying quantum effects to useful devices. For example, to make a quantum-scale wire with macroscopic contact pads, one would simply have the oxidizing tip draw two insulating oxide barriers in an hourglass shape with an extended waist area. The large portions of the hourglass be-come the electrical contact pads, and the extended waist becomes the quantum wire under study. The final point to note is that these scanned oxide tech-niques can readily be combined with conventional integrated circuit patterning, so that the large-scale areas are defined by the usual lithographic and chem-ical techniques, leaving only the more specialized quantum and nanoscale regions to be defined by the oxide-scanned probe.Fabrication of Nanoscale Metal Wires Definedby Nanomolds Fabricated from GaAs-AlGaAs MBE LayersThis technique exploits the special strength of molecular beam epitaxy for fabricating GaAs and AlGaAs layers whose thicknesses are uniform on the micro-scale. The initial process steps for the fabrication are summarized in Figure 4. First the MBE process is used to grow alternating layers of nanoscale-thick GaAs and AlGaAs, and then the GaAs substrate is re-moved from the MBE machine and cleaved in the open atmosphere exposing the [110] cleavage sur-faces that contain a cross-section of the various layers.Figure 3.Demonstration of spin selection of 2D holes at the0.18T magnetic focusing peak using an additionalin-plane magnetic field.Bell Labs Technical Journal153Then, using a chemical etch that preferentially attacks GaAs compared to AlGaAs, the GaAs layers and sub-strate are etched-back to obtain the grooved structure shown in the upper part of the figure. The lower part of the figure is a scanning electron micrograph (SEM) showing the cleaved edge of an actual GaAs-AlGaAs sample with five pairs of nanogrooves ranging in width from 3 nm to 50 nm.The next process step is an optional deposition and patterning of polymethyl methacrylate (PMMA) photoresist shown in light gray in Figure 5, followed by the deposition of the evaporated metal, shown in white, that will form the nanowires. The final step is an ion beam etch directed at a low angle with respect to the [110] cleave-surface. This does not affect the metal wire protected by the deep AlGaAs-walled trench, but it does remove the excess metal depositedon top of the AlGaAs separation layers, thus assuring that the metal nanowires are electrically isolated from the rest of the structure. Electrical contact to the nanowires is formed by means of metal contact strips lying normal to the wires and defined using lithogra-phy and metal liftoff. This procedure is conventional except for the fact that is must be done on the 500 m m wide cleaved edge. Figure 6shows the contacts; the unseen nanowire or wires run horizontally between the contact strips within the wider AlGaAs separation barriers shown as a dark contrast stripe 2.5 m m from the edge of the sample.Figure 4.Scanning electron micrograph of the air-cleaved edge of an AlGaAs-GaAs multilayer after a selective chemical etch that removes only the GaAs wellsto a50nm depth.Figure 5.Process diagram showing metal deposition and ion-etch removal steps needed in forming metal nanowires in the GaAs grooves on the air-cleaved sample edge. Figure 6.154Bell Labs Technical JournalBell Labs Technical Journal 155Using these techniques, we have fabricated con-tinuous metal nanowires of AuPd that are several microns in length and as narrow as 3 nm in cross-section [4]. These wires are remarkably robust. They have survived repeated cycling to cryogenic temper-atures and DC current densities of up to 109A/cm 2before becoming discontinuous.The data points on Figure 7plot room tempera-ture for terminal-resistance measurements of various AuPd nanowires made by this method versus the measured cross-sectional area of the wire. The solid line is the resistance expected for the various wires based on a calculation of the wire area and the meas-ured 2D resistivities of AuPd films code-posited with the wires. The generally good agreement between the two establishes that we are repeatedly able to make metal wires with uniform cross sections as small as 15 nm 2, which corresponds to the designed wire width of 3 nm.The inset of Figure 7demonstrates that at the small-est wire diameters achieved with this technique,we already see significant quantum corrections to the wire conductivity at 4.2K.Shown here is magneto-resistance data for AuPd metal wires 88nm (top),20nm (center),and 5nm (lower)in diameter.In quantum sized metal wires at low temperatures,pair-loops of time-reversed electron scattering trajectories can contribute coherently to the wire conductance [5].Magnetic flux suppresses the contribution of such loops.Thus,at zero magnetic field the quantum coherent scat-tering electron trajectories contribute;however,as the magnet field is raised,the quantum coherence is sup-pressed and the wire resistance rises for the smallest wire by several percent.As the wire diameters become even smaller and the temperature is lowered further,such quantum coherence effects are expected to grow substantially.Fabrication of a Nanostructure Laser from a Single Quantum WireThis nanostructure is fabricated entirely by MBE in a three-step process that we have named “cleaved-edge overgrowth” [1]. As shown in Figure 8, the first step is a conventional MBE growth of a GaAs quan-tum well in the (100) crystal plane sandwiched between two AlGaAs barriers. The substrate wafer is then removed from the MBE machine and thinned from the backside to about 90 m m total thickness. It is then cleaved in air into square pieces 10 mm on side,with additional tick marks at the site of a future cleave to be done in the MBE. These pieces are mounted using Ga solder against a low tantalum wall in the MBE machine so that the top edge of each piece faces the source furnaces. When the MBE is again brought to the proper growth conditions, a mechanical arm (step 2) sweeps across the freely standing pieces,cleaving them at the pre-established tick marks and thereby exposing a fresh (110) crystalline surface.Step 3 is started within a few seconds as the MBE source shutters are opened and the initiation of the second MBE growth begins on the newly exposed (110) cleaved edge.If the second MBE growth is also a quantum well followed by an AlGaAs barrier, then we will have fabricated a nanostructure with two quantum wells that intersect at right angles. The intersection of two such wells has been shown to be a one-dimensional (1D) wire-like trap for electrons, for holes, and forThe inset demonstrates quantum corrections observed at 4.2 K in nanowires of width 5 nm (black trace), 20 nm A [nm 108Ϫ40B [T]48Figure 7.Data plot of resistance per unit length of nanowires versus their cross-sectional area.156Bell Labs Technical JournalFigure 8.Schematic diagram of the cleaved-edge overgrowth molecular beam epitaxy process used in this case to fabricate intersecting quantum wells.Figure 9.Formation of a quantum wire at the right-angle intersection of two quantum wells.excitons [8]. Figure 9illustrates the probability contours for finding a bound electron or bound hole at such an intersection. The binding of any of these particles to the line-like intersecton is completely quantum mechanical in nature. Its origin is that only at the T-intersection can the quantum mechanical wavefunction lower its energy by speading out into three possible lobes.Having established how we can form a quantum wire by cleaved-edge-overgrowth M BE,it now only remains to show how we embedded our wire into an appropriate optical cavity that comprises the first laser that operates from the quantum wire ground state.This is shown in Figure 10.The laser consists of a T-shaped optical cavity with a 1000nm wide optical stem of AlGaAs at 35%Al fraction,intersecting an optical cross-arm 111nm wide of AlGaAs with Al frac-tion 10%.As may be seen in the figure this optical T-structure is itself embedded in an AlGaAs alloy of Al fraction 50%.The optical wire-like confinement modes are shown as gray contours in the figure.The excitonic quantum wire consists of a 14nm wide AlGaAs stem well with a 7%Al alloy fraction,intersecting a cross-arm of GaAs 6nm wide.The confinement contours forWaveguidephoton Quantum wire electronFigure 10.Schematic diagram of the structure of a single wire laser.excitons at the quantum-wire intersection are shown in black.Figure 11is a transmission electron micrograph (TEM) of a very similar T-laser structure fabricated in our laboratory with multiple stem wells and multiple excitonic wires. The T-intersections and the cross-arm for optical confinement are clearly visible.An example of the photoluminescence (PL) uni-formity of our single quantum wire along its length is shown in Figure 12. These are edge-emitting lasers typically 0.5 mm long expected to laser-emit along the quantum wire axis, with Au mirrors on the air-cleaved end-facets. This PL data was taken by scan-ning a 0.5m m dia-micro-focused excitation spot in 0.5m m steps along the entire length of the quantum wire. The wire PL at 1.581 eV is very sharp at 0.9 meV linewidth, and very uniform along the entire wire except for a very few localized excitonic states pre-sumably caused by local fluctuations in the quantum wire width. The wider PL emission at 1.635 eV is due to excitation of the stem well.This laser has multiple T-wire intersections, five of whichare seen in the micrograph.Figure 11.Transmission electron micrograph showing part of the cross section of an actual quantum wire T-laser.Figure13shows the laser emission characteristics of our single-quantum-wire edge-emitting laser[2].These characteristics were obtained by optical pumping alongBell Labs Technical Journal157158Bell Labs Technical Journalthe length of the wire at 4.2K for a 5mm long single quantum wire laser with air-cleaved facets that were Au-coated to form mirrors.Notice that the optical pump-ing threshold power to initiate lasing is only 5mW.Notice further that we are able to raise the optical pump-ing power by more than a factor of 50above this value and that,even at this extreme pumping level,we ob-tain an energy stable laser output with a tiny shift of 1.8meV compared to threshold.We believe this demon-strates that our quantum wire laser is significantly more stable in emission energy versus pump power than are corresponding 2D quantum well lasers.At this time, the weaknesses of these 1D lasers are that:•They are complicated to fabricate in large num-bers, and•They operate only below about 100 K, becauseof the small quantum wire confinement energy compared to the confinement in the nearby stem and cross-arm quantum wells.There is ongoing research in our laboratory to address these issues.References[1] A. R. Goñi, L. N. Pfeiffer, K. W . West, A.Pinczuk, H. U. Baranger, and H. L. Stormer,“Obsevation of Quantum Wire Formation at Intersecting Quantum Wells,” Appl. Phys. Lett.,61 (1992), 1956–1958.[2]Y. Hayamizu, M. Yoshita, S. Watanabe, H.Akiyama, L. N. Pfeiffer, and K. W . West, “Lasing from a Single-Quantum Wire,” Appl. Phys.Lett., 81:26 (2002), 4937–4939.[3]R. Held, T. Heinzel, A. P . Studerus, K. Ensslin,and M. Holland, “Semiconductor Quantum Point Contact Fabricated by Lithography with an Atomic Force Microscope,” Appl. Phys. Lett.,71:18 (1997), 2689–2691.Other scans using 5 m intervals demonstrate that the entire 0.5 mm laser nanowire is continuous with a PL line width of 0.9 meV FWHM.1.56Photon energy (eV)1.58 1.60 1.62 1.64Figure 12.A photoluminescence scan using a 1 m excitation spot moving at 0.5 m intervals for 25 m along a single T-wire.The normalized output spectra are shown for inputpowers ranging from the 5.2 mW threshold to 260 mW.1.5681.570 1.572Photon energy (eV)1.574 1.576260210170130100826652423326211713108.36.65.2⌬E 1(mW)Figure 13.Lasing spectra of the single T-wire at T ؍5K.Bell Labs Technical Journal 159[4]D. Natelson, R. W . Willett, K. W . West, and L. N.Pfeiffer, “Fabrication of Extremely Narrow Metal Wires,” Appl. Phys. Lett., 77:13 (2000)1991–1993.[5]D. Natelson, R. W . Willett, K. W . West, and L. N.Pfeiffer, “Geometry-Dependent Dephasing in Small Metallic Wires,” Phys. Rev. Lett .,86:9(2001), 1821–1824.[6]L. P . Rokhinson, V . Larkina, Y. B. Lyanda-Geller,L. N. Pfeiffer, and K. W . West, “Spin Separation in Cyclotron Motion,” Phys. Rev. Lett., 93:14(2004), 146601-1–146601-4.[7]L. P . Rokhinson, D. C. Tsui, L. N. Pfeiffer, and K.W . West, “AFM Local OxidationNanopatterning of a High Mobility Shallow 2D Hole Gas,” Superlattices Microst., 32:2 (2002),99–102.[8]W . Wegscheider, L. N. Pfeiffer, M. M. Dignam,A. Pinczuk, K. W . West, S. L. McCall, and R.Hull, “Lasing from Excitations in Quantum Wires,” Phys. Rev. Lett., 71:24 (1993),4071–4074.(Manuscript approved June 2005)LOREN N. PFEIFFER is a distinguished member oftechnical staff in the Semiconductor Physics Research Department at Bell Labs in Murray Hill, New Jersey. He received his B.S. degree in physics from the University of Michigan in Ann Arbor and his Ph.D., also in physics,from The Johns Hopkins University in Baltimore,Maryland. A world-renowned leader in the field of molecular beam epitaxy (MBE) technology, he is currently focusing on high-purity growth ofGaAs/AlGaAs quantum well heterostructures. His devices have been proven to exhibit the highest electron mobility ever reported for any semiconductor. Hisprofessional interests include ultra-clean semiconductor growth using MBE, as well as studies of quantum wires,quantum dots, and other nanoscale structures with novel properties. Dr. Pfeiffer is a fellow of both theAmerican Physical Society and the Johns Hopkins Society of Scholars, and he is the recipient of the 2004McGroddy Prize of the American Physical Society.KENNETH W. WEST is a member of technical staff inthe Semiconductor Physics Research Department at Bell Labs in Murray Hill,New Jersey. He is currently working on the growth and characterization of novelsemiconductor structures. His professionalinterests include development of new molecular beamepitaxy processes for high-purity semiconductor structures. He holds a B.S. degree in physics from Bucknell University in Lewisburg, Pennsylvania.ROBERT L. WILLETT is a member of technical staff inthe Semiconductor and Physics Research Department at Bell Labs in Murray Hill,New Jersey, where he conducts research on condensed matter systems. Hereceived an S.B degree in physics fromthe Massachusetts Institute of Technology (MIT) in Cambridge, and M.D. from the University of California at San Francisco, and a Ph.D., also in physics, from MIT.A fellow of the American Physical Society, Dr. Willett was a co-recipient of the 2002 Oliver Buckley Prize.His professional interests are in the physics of lower dimensional electron systems and nanostructures.HIDEFUMI AKIYAMA is a visiting scientist (consultant)in the Semiconductor Physics Research Department at Bell Labs in Murray Hill,New Jersey, where he works ondevelopment, characterization, and optical investigation of T-shaped quantum-wirelasers. He received B.S. and Ph.D. degrees in physics from the University of Tokyo in Japan. Dr. Akiyama has been an associate professor in the Institute for Solid State Physics (ISSP) at the University of Tokyo since 1996.LEONID P .ROKHINSON is a professor in the Departmentof Physics at Purdue University in West Lafayette,Indiana.He holds a Ph.D.in physics from the State University ofNew York at Stony Brook.Dr.Rokhinson,who is professionally interested in thephysics of low dimensional systems,was the recipient of the 2004National Science Foundation Career Award.N。
毕业设计(论文)反相胶束制备纳米CdS及可见光降解有机有毒污染物

毕业设计(论文)题目:反相胶束制备纳米CdS及可见光降解有机有毒污染物目录摘要 (1)前言 (2)正文 (5)1 选题背景 (5)1.1课题来源 (5)1.2课题目的及意义 (5)1.3 国内外研究进展 (6)2 方案论证 (7)2.1 CdS的反相胶束合成法 (10)3 过程论述 (11)3.1 实验主要仪器和试剂 (11)3.2 实验方法 (12)4 结果分析 (14)4.1 XRD分析 (14)4.2 CdS的电化学性质 (14)4.3 CdS光催化活性对比 (16)4.4催化降解MG吸收光谱变化 (19)4.5羟基自由基(.OH)的测定 (20)4.6 H2O2跟踪测定 (20)4.7MG的深度矿化(氧化) (22)4.8降解MG红外光谱分析 (23)5 小结 (23)致谢 (24)参考文献 (24)28 1反相胶束制备纳米CdS及可见光降解有机有毒污染物摘要:本文采用CTAB/正丁醇/正庚烷/水四元反相体系和直接沉淀法制备纳米CdS。
在可见光照射下,以CdS活化分子氧光催化降解孔雀绿(malachite green MG)为探针反应,探讨了反相体系不同水量对制备的纳米CdS光催化活性的影响,确定最佳水量ω([H2O]/[表面活性剂])为25时,制备的纳米CdS光催化活性最高,且反相胶束法制备的CdS活性明显高于直接沉淀法制备的CdS。
利用X-射线衍射仪(XRD)对CdS的晶型、尺寸进行了初步表征,表征结果显示,反相胶束法和直接沉淀法制备的CdS 均为立方闪锌矿型,水量25的CdS平均粒径约为9nm。
以CdS可见光激发催化降解MG,通过分析紫外-可见光谱(UV-Vis)、红外光谱(FTIR)和总有机碳(TOC)的测定,发现在可见光( ≥420nm)和pH=7的条件下,以水量25制备的CdS在70min内可以使MG褪色完全,反应30h后MG的矿化率达到50%以上。
采用循环伏安法(CV)和电化学交流阻抗法(EIS)研究了纳米CdS修饰电极的电化学行为,纳米CdS修饰电极对H2O2电化学还原有明显的催化作用。
nano fabrication

iron nanowire array
Synthesis of Nano Particles
Synthesized Alumina particles.
Chemical Reactions
Metal Nano Particles
Semiconductor Nano Particles
In general, precursors, reduction reagents, surface modification are needed during the formation of nano particles
Imprint mold with 10nm diameter pillars
10nm diameter holes imprinted in PMMA
10nm diameter metal dots fabricated by NIL
Imprint Lithography
Molecular Circuits Fabricated by Imprinting (HP)
Optical Applications
Quantum Dots made of CdSe can be used to replace traditional dye molecules.
Self-Assembled C-Nanotubes
S. Fan et al, Nanotechnology. 14, 1118 (2003)
E-beam system Resist E-beam/Resist Resist Interaction
E-beam wavelength
λ in Å, E is accelerating voltage of electron in V For E=30 kV, λ = 0.07 Å For E=300 kV, λ = 0.022 Å
CdS纳米粒子及其制备方法[发明专利]
![CdS纳米粒子及其制备方法[发明专利]](https://img.taocdn.com/s3/m/28c9a9b203d276a20029bd64783e0912a3167c7f.png)
(19)中华人民共和国国家知识产权局(12)发明专利申请(10)申请公布号 (43)申请公布日 (21)申请号 201810605499.7(22)申请日 2018.06.13(71)申请人 安徽师范大学地址 241002 安徽省芜湖市弋江区九华南路189号科技服务部(72)发明人 夏云生 罗荣 马明柔 韦妹妹 张冰洁 凌云云 汪宜 朱慧 王标 (74)专利代理机构 北京润平知识产权代理有限公司 11283代理人 张苗(51)Int.Cl.C09K 11/56(2006.01)B82Y 40/00(2011.01)C01G 11/02(2006.01)(54)发明名称CdS纳米粒子及其制备方法(57)摘要本发明公开了一种CdS纳米粒子及其制备方法,该制备方法包括:1)将动物蛋白、阳离子表面活性剂于水中进行进行第一接触反应;2)在保护气的存在下,将镉源添加至反应体系中并将体系的pH调至碱性进行第二接触反应,然后将硫源添加至反应体系中进行第三接触反应以制得CdS纳米粒子。
通过该方法制得的CdS纳米粒子具有优异的荧光效应,同时该制备方法具有操作简便、条件温和和原料易得的优点。
权利要求书1页 说明书5页 附图7页CN 108913126 A 2018.11.30C N 108913126A1.一种CdS纳米粒子的制备方法,其特征在于,包括:1)将动物蛋白、阳离子表面活性剂于水中进行进行第一接触反应;2)在保护气的存在下,将镉源添加至反应体系中并将体系的pH调至碱性进行第二接触反应,然后将硫源添加至反应体系中进行第三接触反应以制得所述CdS纳米粒子。
2.根据权利要求1所述的制备方法,其中,在步骤1)中,相对于0.3-0.4g的所述动物蛋白,所述阳离子表面活性剂的用量为2-5μmol,所述水的用量为5-10mL。
3.根据权利要求1所述的制备方法,其中,在步骤1)中,所述第一接触反应满足以下条件:反应温度为45-60℃,反应时间为8-20min。
微纳加工技术新进展
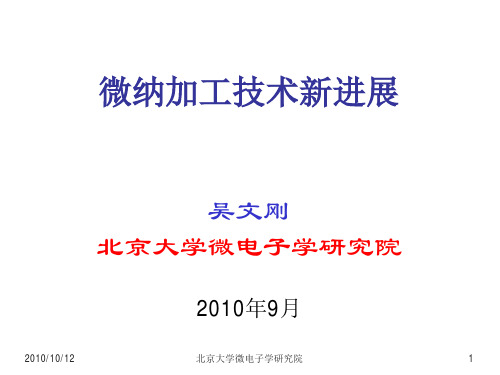
液态Ga
离子源
Needle for
assisting gas
静电透镜
聚焦离子束
2010/10/12
北京大学微电子学研究院
33
(2)
2010/10/12
北京大学微电子学研究院
34
(3)
Direct-write FIB milling
Ga+
2010/10/12
北京大学微电子学研究院
Ga+
35
(4)
微纳加工技术新进展
吴文刚
北京大学微电子学研究院
2010年9月
2010/10/12
北京大学微电子学研究院
1
Think smaller
2010/10/12
北京大学微电子学研究院
2
MEMS领域
微纳加工技术发展的重要特点
2010/10/12
Nano on MEMS → NEMS
MEMS/CMOS → on-chip
• Cost of EUV startup ~50-60 million
- Masks can cost up $88K
2010/10/12
北京大学微电子学研究院
5
Electron Beam Lithography
Advantages
• Very accurate control of pattern with
• Low cost for a next-generation
technology (No need for small λ laser
sources and optics)
• High cost in master mold, but all other
水热法制备二氧化锰及在过氧化氢传感器中的应用
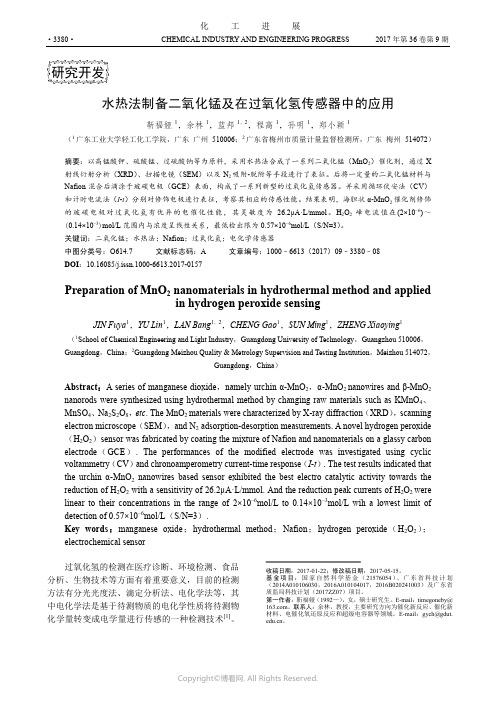
CHEMICAL INDUSTRY AND ENGINEERING PROGRESS 2017年第36卷第9期·3380·化 工 进展水热法制备二氧化锰及在过氧化氢传感器中的应用靳福娅1,余林1,蓝邦1,2,程高1,孙明1,郑小颖1(1广东工业大学轻工化工学院,广东 广州 510006;2广东省梅州市质量计量监督检测所,广东 梅州 514072) 摘要:以高锰酸钾、硫酸锰、过硫酸钠等为原料,采用水热法合成了一系列二氧化锰(MnO 2)催化剂,通过X 射线衍射分析(XRD )、扫描电镜(SEM )以及N 2吸附-脱附等手段进行了表征。
后将一定量的二氧化锰材料与Nafion 混合后滴涂于玻碳电极(GCE )表面,构成了一系列新型的过氧化氢传感器。
并采用循环伏安法(CV )和计时电流法(I -t )分别对修饰电极进行表征,考察其相应的传感性能。
结果表明,海胆状α-MnO 2催化剂修饰的玻碳电极对过氧化氢有优异的电催化性能,其灵敏度为26.2μA·L/mmol 。
H 2O 2峰电流值在(2×10–6)~(0.14×10–3)mol/L 范围内与浓度呈线性关系,最低检出限为0.57×10–6mol/L (S/N=3)。
关键词:二氧化锰;水热法;Nafion ;过氧化氢;电化学传感器中图分类号:O614.7 文献标志码:A 文章编号:1000–6613(2017)09–3380–08 DOI :10.16085/j.issn.1000-6613.2017-0157Preparation of MnO 2 nanomaterials in hydrothermal method and appliedin hydrogen peroxide sensingJIN Fuya 1,YU Lin 1,LAN Bang 1,2,CHENG Gao 1,SUN Ming 1,ZHENG Xiaoying 1(1School of Chemical Engineering and Light Industry ,Guangdong University of Technology ,Guangzhou 510006,Guangdong ,China ;2Guangdong Meizhou Quality & Metrology Supervision and Testing Institution ,Meizhou 514072,Guangdong ,China )Abstract :A series of manganese dioxide ,namely urchin α-MnO 2,α-MnO 2 nanowires and β-MnO 2 nanorods were synthesized using hydrothermal method by changing raw materials such as KMnO 4、MnSO 4、Na 2S 2O 8,etc . The MnO 2 materials were characterized by X-ray diffraction (XRD ),scanning electron microscope (SEM ),and N 2 adsorption-desorption measurements. A novel hydrogen peroxide (H 2O 2)sensor was fabricated by coating the mixture of Nafion and nanomaterials on a glassy carbon electrode (GCE ). The performances of the modified electrode was investigated using cyclic voltammetry (CV )and chronoamperometry current-time response (I -t ). The test results indicated that the urchin α-MnO 2 nanowires based sensor exhibited the best electro catalytic activity towards the reduction of H 2O 2 with a sensitivity of 26.2μA·L/mmol. And the reduction peak currents of H 2O 2 were linear to their concentrations in the range of 2×10–6mol/L to 0.14×10–3mol/L wih a lowest limit of detection of 0.57×10–6mol/L (S/N=3).Key words :manganese oxide ;hydrothermal method ;Nafion ;hydrogen peroxide (H 2O 2);electrochemical sensor过氧化氢的检测在医疗诊断、环境检测、食品分析、生物技术等方面有着重要意义,目前的检测方法有分光光度法、滴定分析法、电化学法等,其中电化学法是基于待测物质的电化学性质将待测物化学量转变成电学量进行传感的一种检测技术[1]。
- 1、下载文档前请自行甄别文档内容的完整性,平台不提供额外的编辑、内容补充、找答案等附加服务。
- 2、"仅部分预览"的文档,不可在线预览部分如存在完整性等问题,可反馈申请退款(可完整预览的文档不适用该条件!)。
- 3、如文档侵犯您的权益,请联系客服反馈,我们会尽快为您处理(人工客服工作时间:9:00-18:30)。
Journal of Crystal Growth 246(2002)108–112Directional CdS nanowires fabricated by chemicalbath depositionHui Zhang,Xiangyang Ma,Jin Xu,Junjie Niu,Jian Sha,Deren Yang*State Key Lab of Silicon Materials,Zhejiang University,310027Hangzhou,People’s Republic of ChinaReceived 15March 2002;accepted 14September 2002Communicated by L.F.SchneemeyerAbstractDirectional CdS nanowires have been fabricated by using chemical bath deposition (CBD)and porous anodic aluminum oxide (AAO)template.X-ray diffraction and selected area electron diffraction show that the nanowires are hexagonal polycrystalline in nature.Transmission electron microscopy (TEM)reveals that the diameters of nanowires are about 60nm.Furthermore,the high-resolution TEM illustrates the lattice images of {002},{101}and {100}planes in the nanowires.The directional growth of nanowires is verified by scanning electron microscopy.It is believed that the ion-by-ion mechanism dictates the CBD of CdS nanowires within the pores of AAO template.r 2002Elsevier Science B.V.All rights reserved.PACS:81.05.Dz;81.05.YsKeywords:B1.Nanomaterials;B2.Semiconducting II–VI materials1.IntroductionOne-dimensional nanostructural materials have attracted much attention over the years due to their fundamental importance and potential appli-cations in the nanodevices [1–8].It is well known that CdS is a typical wide band gap II–VI semiconductor having a band gap 2.42eV at room temperature.It has many commercial or potential applications in light-emitting diodes,solar cells,or other photoelectric devices.Recently,CdS nano-wires have been fabricated by the electrodepositionand the chemical solution transport (CST)meth-ods [9–16].In this paper,we have synthesized hexagonal polycrystalline CdS nanowires using an alternative method,i.e.CBD on porous anodic aluminum oxide (AAO)template.The basic idea behind this method is,on one hand,to make use of the nanosized pores in AAO for the confinement of growth of nanowires across the diameter,on the other hand,to take advantage of the orderly pores in the AAO template for the directional growth of CdS nanowires along the pared with electrodeposition and CST,CBD has the advantages of simplicity,high efficiency and low cost,moreover,CBD is a well proved process for the preparation of CdS films [17–23].*Corresponding author.Tel.:+86-571-8795-1667;fax:+86-571-8795-2322.E-mail address:mseyang@ (D.Yang).0022-0248/02/$-see front matter r 2002Elsevier Science B.V.All rights reserved.PII:S 0022-0248(02)01900-02.ExperimentA pure Al plate(99.99%)was annealed at5001C for2h in vacuumto formtexture,then degreased in acetone.Subsequently,it was anodized at161C in oxalic acid solution at a constant voltage of42V for3h.As a result an anodic oxide layer was formed.The top part of this layer was disorderly, which was removed in a mixture of phosphoric acid and chromic acid.After this removal,the textured Al plate was anodized again for10h under the conditions identical to those for thefirst anodizing step.Then the backside Al layer of the specimen was removed in a saturated CuCl2 solution.The bottom part of the membrane was removed by exposure to phosphoric acid at301C for90min,finally,the anodized aluminum oxide (AAO)in porous structure was formed and dried at301C.Because the diameters of pores(channels) of AAO lie in several tens of nanometers range. CdS nanowires were deposited into the AAO template by reacting0.02M CdCl2,0.05M (NH2)2CS and0.05M NH4Cl in an aqueous solution using NH3ÁH2O as a complexing agent. The pH of the solution was adjusted to11using NH3ÁH2O and the bath temperature was801C. The chemical deposition lasted for20min,then the sample was achieved and washed with deionized water.After drying in a601C oven,the sample was annealed at3001C for1h under N2atmosphere.The phase of CdS nanowires incorporated into the AAO templates was characterized by powder X-ray diffraction(XRD),with Cu K a radiation. Transmission electron microscopy(TEM)with energy dispersive X-ray(EDAX)was applied to determine the morphology and composition.To obtain the specimen for TEM observation,AAO template was dissolved in1M NaOH at601C for 1h with ultrasonic vibration,thus the CdS nanowires were dispersed uniformly in the solu-tion.Then,a small drop of the solution was dipped on a Cu grid covered with carbonfilm.The directional growth of CdS nanowires was checked by scanning electron microscope(SEM).3.Results and discussionFig.1shows the XRD spectrumfor the CdS nanowires incorporated into the AAO template. As marked in thefigure,the spectrum consists of two sets of peaks.According to the standard JCPDS cards,one set corresponds to the hexago-nal structure with a nominal composition of CdS, and the other to g-Al2O3.Fig.2shows the typical TEM image of the CdS nanowires.It can be seen that this nanowire has a relatively straight morphology and a diameter of about60nm,which corresponds to the diameter of the pores within the template used.The lowerleft Fig.1.XRD pattern of the CdS nanowires incorporated into AAO template.H.Zhang et al./Journal of Crystal Growth246(2002)108–112109inset in Fig.2is an image of the multi CdS nanowires with diameter nearly the same as the single CdS nanowire.The upper right inset in Fig.2gives a selected area electron diffraction (SAED)pattern.In this pattern,the first and second diffraction spots correspond to the {002}and {004}planes with an interplanar spacing ofabout 3.34and 1.62(A,respectively,while the first and second diffraction circles correspond to the {110}and {203}planes with an interplanarspacing of about 2.13and 1.33(A,respectively.The above result indicates that the CdS nanowires are hexagonal polycrystalline in nature.The EDAX spectrumof the CdS nanowire is shown in Fig.3.The very strong peaks for Cd and S are found in the spectrum.Quantitative analysis shows that the CdS nanowires are deficient in S in terms of composition,probably due to the volatility of S.The O peak may be originated fromthe oxidation of CdS nanowires exposed to the air.The C and Cu peaks come from the copper grid used to support the CdS nanowires.Fig.4shows the high-resolution transmission electron microscopy (HRTEM)images of the middle and fringe parts in a single CdSnanowire.Fig.2.TEM image of a typical CdS nanowire.The upper right inset is the corresponding SAED pattern;the lower left inset is an image of multi-CdSnanowires.Fig.3.The EDAX spectrumof a single CdS nanowire.H.Zhang et al./Journal of Crystal Growth 246(2002)108–112110Fig.4(a)reveals that in the middle of the nanowire,the main lattice planes are {002}and {100}with spacings of about 0.34and 0.36nm,respectively.Fig.4(b)shows that in the fringe of the nanowire,the main crystal plane is {101}with a lattice spacing of about 0.32nm.There-fore,the CdS nanowires have been again proved to be polycrystalline in nature with the major planes of {002},{100}and {101},which is in accordance with the XRD pattern shown in Fig.1.Fig.5shows the SEM image of the cross-section of the sample.The directional growth of CdS nanowires was found,because of the confined growth of the nanowires in the orderly pores of an AAO template.For the CdS thin films growth,two mechanisms have been put forward [24,25].One is cluster-by-cluster mechanism described as following:in the ammonia-thiourea system,S 2Àions are released by the alkaline hydrolysis of thiourea and Cd 2+ions are released by dissociation of the corresponding ammonia complexes.As soon as the product of the free S 2Àand Cd 2+ion concentrations exceeds the solubility product of CdS,the precipitation of CdS takes place.The other is ion-by-ion mechanism which consists of the following three steps:(1)the reversible adsorption on the substrate surface of dihydroxo-diamino cadmium,(2)the adsorption of thiourea by the formation of a metastable complex,(3)the formation of CdS and the site regeneration by the metastable complex decom-position.For the formation of CdS nanowires,it is believed to be dictated by ion-by-ion mechanism,because the CdS clusters formed in the solution via cluster-by-cluster mechanism are difficult to enter into the pores of AAO template.4.ConclusionsIn summary,we have synthesized the hexagonal polycrystalline CdS nanowires into the AAO templates using chemical bath deposition,whichFig.5.Directional growth of CdS nanowires revealed by cross-sectionalSEM.Fig.4.HRTEM images of a single CdS nanowire at different positions:(a)middle and (b)fringe.H.Zhang et al./Journal of Crystal Growth 246(2002)108–112111is much simpler than other methods such as electrodeposition and CST.SEM indicates that CdS nanowires grow directionally due to the confinement of AAO pores.The diameters of the CdS nanowires are about60nmderived fromthe TEM.HRTEM images clearly show the lattice image of{002},{101}and{100}planes of the nanowires.It is expected that the diameter of CdS nanowires can be further minimized by reducing the diameter of the pores in the AAO template. Furthermore,CBD method is also believed to be appropriate for the growth of other semiconductor nanowires.AcknowledgementsThe authors would like to appreciate the financial supports of863project No. (2001AA513023)and Zhejiang Provincial Natural Science Fund(No.601092).References[1]S.Iijima,Nature354(1991)56.[2]H.Dai,E.W.Wang,Y.Z.Lu,S.Fan,C.M.Lieber,Nature375(1995)769.[3]W.Han,S.Fan,Q.Li,Y.Hu,Science277(1997)1287.[4]J.R.Heath,F.K.Le Goues,Chem.Phys.Lett.208(1993)263.[5]Z.F.Ren,Z.P.Huang,J.W.Xu,J.H.Wang,P.Bush,M.P.Siegal,P.N.Provencio,Science282(1998)1105.[6]M.S.Fuhrer,J.Nygard,L.Shih,M.Forero,Young-GuiYoon,M.S.C.Mazzoni,Hyoung Joon Choi,Science288 (2000)494.[7]J.Kong,N.R.Franklin,C.Zhou,M.G.Chapline,S.Peng,K.Cho,H.Dai,Science287(2000)622.[8]J.Hone,B.Batlogg,Z.Benes,A.T.Johnson,J.E.Fischer,Science289(2000)1730.[9]D.Routkevich,T.Bigioni,M.Moskovits,J.Ming Xu,J.Phys.Chem.100(1996)14037.[10]J.Zhang,X.Yang,D.Wang,S.Li,Yi Xie,Younan Xia,Yitai Qian,Adv.Mater.12(2000)1348.[11]D.Xu,Y.Xu,D.Chen,G.Guo,L.Gui,Y.Tang,Adv.Mater.12(2000)520.[12]D.Routkevitch,T.L.Haslett,L.Ryan,T.Bigioni,C.Douketis,M.Moskovits,Chem.Phys.210(1996)343.[13]D.Xu,Y.Xu,D.Chen,G.Guo,L.Gui,Y.Tang,Chem.Phys.Lett.325(2000)340.[14]Y.Li,J.Wan,Z.Gu,Mater.Sci.Eng.A286(2000)106.[15]J.Suh,J.Lee,Chem.Phys.Lett.281(1997)384.[16]J.H.Zhan,X.G.Yang,S.D.Li,D.W.Wang,Y.Xie,Y.T.Qian,J.Crystal Growth220(2000)231.[17]G.Sasikala,P.Thilakan,C.Subramanian,Sol.EnergyMater.Sol.Cells62(2000)275.[18]J.G.Vazquez-Luna,R.B.Lopez Flores,M.Rubin-Falfan,L.Del,C.Gomez-Pavon,J.Crystal Growth187(1998) 380.[19]I.O.Oladeji,L.Chow,J.R.Liu,W.K.Chu, A.N.P.Bustamante, C.Fredricksen, A.F.Schulte,Thin Solid Films359(2000)154.[20]A.Zehe,J.G.Vazquez Luna,Sol.Energy Mater.Sol.Cells68(2000)217.[21]J.Herreo,M.T.Gutierrez,C.Guillen,J.M.Dona,M.A.Martinez,A.M.Chaparro,R.Bayon,Thin Solid Films 361–362(2000)28.[22]Y.Nosaka,K.Yamaguchi,H.Miyama,H.Hayashi,Chem.Lett.87(1988)605.[23]M.A.Martinez,C.Guillen,J.Herrero,Appl.Surf.Sci.140(1999)182.[24]J.M.Dona,J.Herrero,J.Electrochem.Soc.144(1997)4081.[25]R.Ortega-Borges, D.Lincol,J.Electrochem.Soc.140(1993)3464.H.Zhang et al./Journal of Crystal Growth246(2002)108–112 112。